Abstract
Considering the biorefinery concept, the authors of this review, who belong to the Chemical Engineering Department of the University of Vigo, in the Faculty of Science, have developed their researches in this field. This review shows the work of the group in the last two decades, based on the fractionating of lignocellulosic materials (forest, industrial, and agricultural wastes) to obtain different products such as phenolic compounds with antioxidant effects, oligosaccharides with prebiotic properties, lactic acid, bioethanol, biocomposites, etc.
Teniendo en cuenta el concepto de biorrefinería, los autores de esta revisión, que pertenecen al Departamento de Ingeniería Química de la Universidad de Vigo, en la Facultad de Ciencias, han desarrollado sus investigaciones en este campo. Esta revisión muestra el trabajo del grupo en las dos últimas décadas, basado en el fraccionamiento de materiales lignocelulósicos (residuos forestales, industriales y agrícolas) para obtener diferentes productos, tales como compuestos fenólicos con efectos antioxidantes, oligosacáridos con propiedades prebióticas, ácido láctico, bioetanol, materiales compuestos, etc.
Introduction
Biorefinery, a concept analogous to petroleum refineries, is defined by the International Energy Agency Bioenergy Task 42 (2009) as the sustainable processing of biomass into a spectrum of marketable products (food, feed, materials, chemicals) and energy (fuels, power, heat).
According to the National Research Council (Citation2000), the development of biorefineries is the key for access to an integrated production of food, feed, chemicals, materials, goods, fuels, and energy of the future.
The valorization of biomass first involves a pretreatment step. It is accepted that the treatment is a key stage that determines the rest of the global biorefinery process. An ideal pretreatment must meet the following conditions: (1) simple and inexpensive operation, (2) facility to reduce particle size, (3) low energy, water and chemicals’ consumption, (4) little corrosion, (5) capable to alter the lignocellulosic material (LCM) structure, (6) with low or no loss of polysaccharides, (7) production of large amounts ofhemicelluloses’ derivatives with high added-valueproduction, (8) generation of low furfural, hydroxymethylfurfural, or phenolic acids (9) obtaining of solid residues with high cellulose content and high enzyme susceptibility, (10) production of lignin and/or derivatives of high quality, and (11) generation of little wastes (Garrote, Domínguez, & Parajó, Citation1999). In this context, autohydrolysis seems an ideal pretreatment. The optimum operating conditions of hydrothermal pretreatment should be selected taking into account thecomposition of the liquid phase, which contains monomeric and oligomeric sugars, sugar degradation products, organic acids, extractives, and phenolics, and the composition (cellulose and lignin) and susceptibility to enzymatic hydrolysis of the solid fraction.
Supplementary Figure 1 shows a general scheme of the processes that can be carried out for the fractionation of biomass to separate the main components of LCM (cellulose, hemicellulose, and lignin).
When raw materials rich in pectins, xylan, or other saccharide polymers are subjected to hydrothermal processing under selected operational conditions, mixtures of oligosaccharides are generated in the reaction medium by partial depolymerization of the mentioned polymers. If the material is rich in pectin, mixtures mainly made up of arabino-oligosaccharides, galacto-oligosaccharides and oligogalacturonides can be obtained. If a xylan-rich material is used, mixtures of substituted xylooligosaccharides (XOSs) are then produced. The use of softwoods gives as a result a mixture of galactomannans, glucomannans, and other oligosaccharides.
The liquid phase obtained is a complex mixture that requires further purification in order to produce oligosaccharides to be used as prebiotics or monomeric sugars to be used as a detoxified carbon source for bioconversion processes. Also, the phenolic compounds presented due to partial depolymerization of lignin and lignin–hemicellulose can be used due to their antioxidant properties.
The oligosaccharides from autohydrolysis liquors can be purified by solvent extraction, ionic exchange resins, or membrane processing for further applications. Membrane processing using ultra- or nano-filtration membranes allows not only the concentration of these oligosaccharides but also the removal of monosaccharides and other low molecular weight compounds undesirable for the use of these oligosaccharides as prebiotics. However, nanofiltration membranes can also be used to separate monosaccharides from sugar degradation products, such as furfural or hydroxymethylfurfural. Also, oligosaccharides from autohydrolysis liquors or the solid phase can also be treated enzymatically to obtain monomeric sugars. In both cases, these sugars can be used as carbon source for fermentation purposes to obtain bioethanol or organic compounds such as lactic acid.
Solvent extraction has been successfully applied to selectively separate non-saccharide inhibitory compounds. The waste stream generated by the solvent extraction of the hydrolyzates can be valorized, providing phenolic compounds released from the depolymerized lignin fraction, and also extractives, aliphatic fatty acids, and sugar-derived products. In order to increase the purity of these extracts, several technologies such as solvent fractionation, adsorption–desorption in gels and polymeric resins have been addressed.
Following the biorefinery scheme shown in Supplementary Figure1, in the autohydrolysis treatment, most of initial LCM is recovered as solid residue or spent solid, in proportions typically in the range from 40 to 65 kg/100 kg raw material, on dry basis. This solid residue is mainly composed of cellulose and lignin, and their structure was altered by the hydrothermal treatment that made the solid residue more susceptible to later stages of global process. An interesting option isthe production of second-generation biofuels from this solid residue. Bioethanol from LCM is considered one of the most promising sources of alternatives to fossil fuels, showing advantages as improvement of local economy, reduction in CO2 emissions, abundance, renewability, lack of dependence on other countries, and may replace up to 30% of gasoline.
On the other hand, lactic acid (or 2-hydroxypropanoic acid) is a valuable industrial chemical with a variety of applications, such as (1) acidulant, flavor, and preservative in the food industry; (2) raw material for the production of base chemicals; and (3) monomer for polymerization to biodegradable poly-lactic acid (PLA), an environmental friendly alternative to biodegradable plastics (Hofvendahl & Hähn-Hägerdal, Citation2000; John, Anisha, Nampoothiri, & Pandey, Citation2009; Vijayakumar, Aravindan & Viruthagiri, Citation2008). Lactic acid can be produced commercially by chemical and fermentative methods. The biotechnological process shows several advantages over the chemical synthesis, including the selective production of only one isomer (d(+) or l(+)) or the use of cheap raw materials such as corn cob, whey, molasses, and other LCMs (Vijayakumar et al., Citation2008). Fermentative lactic acid production from renewable resources comprises the following steps: pretreatment of substrate, hydrolysis of polysaccharides to sugars, fermentation of sugars to lactic acid, separation of bacteria and solid particles from the broth, and purification of lactic acid (Hofvendahl & Hähn-Hägerdal, Citation2000).
Besides its application as carbon source for fermentation, the solid phase obtained from the autohydrolysis reaction can be used for bio-composites’ manufacture, as autohydrolysis is one of the processes studied to modify the chemical nature and structure of fibers and improve their interaction with polymeric matrix. The bio-composites are materials formed by a polymeric matrix and a reinforcing material, in which at least one of them is derived from natural resources. The reinforcement material can be plant or wood fibers, with or without surface chemical or physical modification. Due to the hydrophilic nature of the natural fibers and the hydrophobicity of the matrix, their interaction is limited, and this fact affects the mechanical properties of the obtained composites. In order to improve the compatibility between these materials, chemical modifications of fiber surface or the use of compounds acting as compatibilizers has been studied.
Relevant studies
In this section, a review of the work carried out by the research group into the biorefinery field is shown. This has been divided in the different components of the LCMs that can be used for several applications. Supplementary Table 1 summarizes a variety of processes developed by the group in which several raw materials were studied to produce prebiotics, lactic acid, bioethanol, antioxidants, and composites.
Oligosaccharides to be used as prebiotics
A prebiotic is defined as “a selectively fermented ingredient that allows specific changes in the composition and/or activity of the gastrointestinal microbiota that confers benefits upon host wellbeing and health” (Gibson, Probert, Van Loo, Rastall, & Roberfroid, Citation2004). The most important prebiotics are the non-digestible oligosaccharides, including fructoligosaccharides and galacto-oligosaccharides, which are commercially available. Its bifidogenic capacity has been confirmed by in vivo assays with humans. Due to the influence of prebiotics on human health, there is, nowadays, a growing interest in the development of alternative and clean processes for the production of new prebiotic ingredients with added functionality. Pectic oligosaccharides and XOSs are two examples of this kind of new prebiotics.
In previous works, our research team has evaluated the effect of the operational conditions (temperature and reaction time) on the oligosaccharides (OS) production using both xylan and pectin-rich raw materials. Supplementary Tables 2 and 3 show some of the results obtained.
Simultaneously, other effects (including extractive removal and side-reactions leading to the production of monosaccharides, sugar-decomposition products and lignin depolymerization) occur in the reaction media. In order to utilize the OS as food ingredients, some undesired compounds (present in the enzymatic hydrolyzates) must be removed, thereby increasing the purity of the product. For this purpose, nanofiltration, liquid–liquid extraction, and ion exchange (using anionic and cationic resins) were useful alternatives for concentrating liquors and/or removing low-molar mass impurities, such as monosaccharides or phenolics, enabling the purification of oligosaccharide mixtures.
Following a processing scheme based on the mentioned technologies (see Vegas et al. (Citation2006) for details), a final product containing 90.7% oligosaccharides, 3.6% monosaccharides, and only 5.7% of non-volatile impurities (mainly phenolic and nitrogen-containing compounds) was produced from autohydrolysis liquors obtained using rice husks as raw material.
González-Muñoz, Domínguez, and Parajó (Citation2008) studied the depolymerization of oligosaccharides from rice husks in an enzymatic membrane reactor, using a commercial xylanase and a 2 kDa membrane, obtaining oligosaccharides with a degree of polymerization (DP) range of 2 to 4 in the permeate. The study compares two different approaches: simultaneous reaction and permeation, and combined reaction and permeation. Gullón et al. (2010) studied the manufacturing of high-purity XOSs obtained from rice husks autohydrolysis and its structural characterization. Besides, the fermentability of the purified product by fecal inocula was also evaluated to determine the potential prebiotic effect.
Gullón, González-Muñoz, and Parajó (Citation2008) fractionated XOSs from Eucalyptus globulus autohydrolysis liquors by ultrafiltration using ceramic membranes with different cut-offs. Operating at 7.5 bar with a 5 kDa membrane up to a volume concentration ratio of 5.5 led to an increase of XOSs and substituents (acetyl and uronic groups) by a factor of 3.8%, decreasing the mass fraction of monosaccharides by 55%. The structure and properties of XOSs from E. globulus were studied by Gullón, González-Muñoz, Van Gool, et al. (Citation2011). These studies confirmed the potential of the refined XOSs for prebiotic applications.
Gullón, González-Muñoz, and Parajó (Citation2011) studied the potential prebiotic effects of oligosaccharides from solid wastes obtained in the dehulling stage of malting industries, mainly made up of barley husks, spent grains, and grain fragments. Three different concentrates of XOSs (obtained as indicated in Supplementary Figure2) were fermented by fecal inocula and their prebiotic potential was confirmed by the presence of metabolic products such as succinate, lactate, formate, acetate, propionate, and butyrate.
González-Muñoz, Álvarez, Santos, and Parajó (in press) studied the solubilization of hemicelluloses from Pinus pinaster wood, determining the potential hemicellulosic sugar content that can be obtained at different operating conditions (based on the severity of the process) in non-isothermal autohydrolysis. González-Muñoz, Santos, and Parajó (Citation2011) purified oligosaccharides from P. pinaster autohydrolysis liquors by diafiltration. The autohydrolysis conditions were evaluated and the optimum to obtain a liquor with high content of oligosaccharides and low in monosaccharides was established as a isothermal process at175°C during 24.5 min. The high retention of oligosaccharides and low of monosaccharides during the diafiltration step allowed increasing the oligosaccharides to monosaccharides ratio from 4 to 17.8, obtaining a liquor with a 94.7% of oligosaccharides (with respect to non-volatile compounds).
Cellulosic fraction for second-generation bioethanol
The second-generation bioethanol production (from solid residues with low pentose content, as those produced in autohydrolysis) involves the following three steps: pretreatment of LCM for improving the susceptibility of enzymes; enzymatic hydrolysis of cellulose to glucose, and biological conversion of glucose to ethanol.
Supplementary Table 4 shows the results of the effects of the autohydrolysis on enzymatic hydrolysis. As general trend, more severe operating conditions led to a decrease in oligomer concentration in liquid phase (due to decomposition reactions) and an increase in glucose concentration in enzymatic hydrolysis. In the severest autohydrolysis conditions, a slight decrease in glucose concentration can be observed in agricultural residues, as with corn cobs, with a decrease of 29.3% of maximum glucose concentration with increasing autohydrolysis maximum temperature from 210 to 216°C (Garrote, Yáñez, Alonso, & Parajó, Citation2008), or with barley husks, with a decrease of 10.1% with increasing autohydrolysis maximum temperature from 210 to 216°C (Ares-Peón, Vila, Garrote, & Parajó, Citation2011). With a typical hardwood, Eucalytpus globulus, no decrease in maximum glucose concentration with increasing autohydrolysis temperature was observed (Romaní, Garrote, Alonso, & Parajó, Citation2010b). With these three LCMs, the cellulose to glucose conversion is close to 100% in the optimal enzymatic hydrolysis conditions. These data show that it is necessary to search intermediate operating autohydrolysis conditions leading to a global optimum (from an economic point of view), taking into account the recovery of oligomers in the liquid phase and the susceptibility of the solid residue to enzymatic conversion, without forgetting other valuable compounds, as pectin or phenolic compounds. As shown in Supplementary Table 4, these conditions are not the same. In case of hardwoods, more severe autohydrolysis conditions, up to 250°C, do not produce improvements in cellulose to glucose conversion, as it begins to degrade the cellulose during the autohydrolysis (Romaní, Garrote, Alonso, & Parajó, Citation2010a).
Simultaneous saccharification and fermentation (SSF) assays carried out with solid residue of E.globulus wood show that autohydrolysis conditions of 230°C result in the best bioethanol concentration and conversion, with an optimum value of 86.4% of cellulose to ethanol conversion (Romaní et al., Citation2010a). In order to improve the compatibility among hemicellulose-derived compounds in liquid phase and good bioethanol conversion of solid phase, the treatment of solid residue from autohydrolysis with sodium hydroxide, in the processing of Acacia dealbata (Yáñez, Romaní, Garrote, Alonso, & Parajó, Citation2009), or with organosolv using ethanol/water solutions, with E. globulus (Romaní, Garrote, López, & Parajó, Citation2011), has been investigated. With both treatments, significant improvements were achieved in the cellulose to glucose conversion and in the kinetic of enzymatic conversion.
Monomeric sugars for obtaining lactic acid
Among the fermentation processes studied, the SSF technology has been recommended as a good strategy for microbial production of value-added products, like ethanol and lactic acid from renewable biomass. The SSF process offers various advantages such as the use of a single-reaction vessel for both hydrolysis and fermentation, low processing time, reduced product inhibition, and increased productivity (Gullón, Garrote, Alonso, & Parajó, Citation2007; Marques, Santos, Gírio, & Roseiro, Citation2008; Sreenath, Moldes, Koegel, & Straub, Citation2001; Yáñez, Alonso, & Parajó, Citation2005).
In this field, our research group reported several works following the processing scheme showed in Supplementary Figure 3 (Rivas, Moldes, Domínguez, & Parajó, Citation2004; Yáñez, Alonso, & Parajó, Citation2006; Yáñez et al., Citation2009).
Rivas et al. (Citation2004) reported the SSF production of lactic acid from autohydrolyzed corn cobs in media containing cheap nutrients (spent yeast cells and corn steep liquor), cellulolytic enzymes, and Lactobacilllus rhamnosus CECT-288 cells. In SSF, experiments were carried out under a variety of operational conditions defined by three independent variables (liquor to solid ratio (6–10 g/g), enzyme to substrate ratio (5–55 FPU/g substrate), and spent yeast concentration (5–20 g/L)). The dependence of the lactic acid concentration achieved on the operational conditions was interpreted by means of an empirical model. Supplementary Table 5 shows the lactic acid concentration, productivity, and yield obtained under the selected conditions.
Yáñez et al. (2006) subjected rice husks to autohydrolysis (non-isothermal operation to reach a final temperature of 205°C, liquid to solid ratio of 8 g/g). Then, the resulting solid phase was assayed as substrate for enzymatic hydrolysis after a treatment with hydrogen peroxide. The effects of the temperature (30–80°C), reaction time (1–4 h), hydrogen peroxide charge (10–50 g/100 g autohydrolyzed solid), and liquid to solid ratio (15 and 30 g/g) were evaluated. Solid yield, solid composition, and susceptibility to enzymatic hydrolysis were assessed by means of empirical second-order mathematical models. Under selected conditions (50 g hydrogen peroxide/100 g solids, 80°C, 4 h, liquor to solid ratio of 15 g/g), high proportions of lignin were removed. When the pretreated solid was subjected to enzymatic hydrolysis (liquor to solid ratio of 15 g/g, enzyme/substrate ratio of 25 FPU/g, 48 h), 67.9% of cellulose was converted into glucose, leading to high glucose concentrations (43.5 g/L). In an additional experimental work (data no published), selected pretreated solid was subjected to SSF (see conditions and main results in Supplementary Table 5), showing the viability of the lactic process.
Recently, Yáñez et al. (Citation2009) reported a study dealing with the autohydrolysis, sodium hydroxide extraction, and enzymatic hydrolysis of A. dealbata wood. Under selected conditions, solids containing 90% cellulose were produced. When these samples were used as hydrolysis substrates, solutions containing up to 27.8 g glucose/L were obtained after 48 h of enzymatic hydrolysis, corresponding to a yield of 47.3 g glucose per 100 g autohydrolyzed solids. Some preliminary SSF experiments were carried out (data not reported, see conditions in Supplementary Table 5), and lactic acid concentrations close to 100 g/L at 60 h of the treatment were achieved.
Extractives and lignin fraction for their use as antioxidants
Solvent extraction with ethyl acetate has been successfully applied to detoxify hydrolyzates (Parajó, Domínguez, & Domínguez, Citation1997) and to refine XOS solutions (Vegas, Alonso, Domínguez, & Parajó, Citation2004), and the phenolic compounds recovered in the extract may be considered of natural structure and composition and have showed antioxidant activity comparable to that of synthetic antioxidants (Cruz, Domínguez, Domínguez, & Parajó, Citation1999, 2001; Cruz, Domínguez, & Parajó, 2004; Garrote, Cruz, Domínguez, & Parajó, Citation2003, Citation2008). The combination of these alternatives for barley husks, the solid byproduct from malting industries, has been proposed to yield a liquid phase containing oligosaccharides with prebiotic activity and low-molecular weight phenolics with antioxidant activity, and a solid phase containing cellulose and lignin (Conde, Gullón, Moure, Domínguez, & Parajó, Citation2009). In another alternative, the aqueous phases from treatments were extracted with ethyl acetate, and the soluble solids were redissolved in 80% ethanol (to obtain a more hydrophilic product), although this stage decreased the phenolic contents and radical scavenging capacities of the ethyl acetate extracts from almond shells, chestnuts burs, and grape pomace (Conde, Moure, Domínguez, & Parajó, Citation2011). This application of the ethyl acetate extracts would add commercial value to this byproduct of the refining process, providing a potentially useful approach to sustainable development.
Among phenolic compounds, alcohols, aldehydes, ketones, and benzoic and cinnamic acids have been identified. These latter ones are naturally found in fruits and vegetables and are primary responsible for the antioxidant potency of some extracts. The high-performance liquid chromatography with diode-array detection (HPLC-DAD) confirmed the presence of benzoic and cinnamic acids as major components (Conde, Gullón, et al., Citation2009; Conde, Moure, Domínguez, & Parajó, Citation2008, Citation2011; Garrote, Cruz, et al., Citation2008; Garrote, Falqué, Domínguez, & Parajó, Citation2007; Garrote, Kabel, Schols, Falqué, Domínguez, & Parajó, Citation2007; Parajó et al., 2008).
A revision of the antioxidant potential of this lignin-soluble fraction has been published (Garrote, Cruz, Moure, Domínguez, & Parajó, Citation2004), and the works on the recovery of the fraction solubilized in autohydrolysis treatments of agroindustrial, forest, and food wastes of lignocellulosic nature have been summarized (Parajó et al., Citation2008) and are now updated. Supplementary Figure 4 shows the simplified flow diagram of an integrated multiproduct processing of mild acid hydrolysis fractionated LCMs.
The major operational conditions influencing the hydrolytic process are temperature and time, which can be closely related if the treatment is performed under non-isothermal conditions. The effects of autohydrolysis temperature during the autohydrolysis of lignocellulosics are shown in Supplementary Figure 5. In a comparative study, the highest percentages of solid solubilization upon autohydrolysis (30%) were observed for corn cob and almond shells (Conde, et al., Citation2011). Supplementary Table 6 summarizes the soluble yield as weight percent of the initial solid material and the antioxidant assays reported. The radical scavenging capacity was determined using stable free radicals DPPH (α,α-diphenyl-β-picrylhydrazyl), ABTS (2,2′-azinobis (3-ethyl-benzothiazoline-6-sulfonate)), superoxide, and hydroxyl, or with the radical generator AAPH (2,2′-azobis (2-methylpropionamidine) dihydrochloride). The reducing power of the ethyl acetate extracts and the ability to protect from oil oxidation both in bulk phase and in emulsions have been confirmed, as well as the higher thermostability than synthetic antioxidants (Cruz, Conde, Domínguez, & Parajó, Citation2007).
The antimicrobial action of crude extracts was reported (Cruz et al., Citation2001). The crude extracts protected fish oil from oxidation (Moure, Pazos, Medina, Domínguez, & Parajó, Citation2007), and the addition of extracts to pineapple, apple, and orange natural juices enhanced their stability at room temperature (Conde, Citation2003). Purified extracts were active to protect the stability of sunflower oil in water/oil and in oil/water emulsions (Conde, Moure, Domínguez, Gordon, and Parajó, Citation2011).
The original phenolic content in the ethyl acetate crude extracts ranged between 25 and 35% d.w.b. (Conde, et al., Citation2011; Garrote et al., Citation2004; Parajó et al., Citation2008), and the removal of impurities using membranes, activated carbon (Soto, Moure, Domínguez, & Parajó, Citation2008), solvent fractionation (Conde et al., Citation2008), Sephadex LH-20 gels (Cruz et al., Citation2004; Cruz, Domínguez, & Parajó, Citation2005; Parajó et al., Citation2008), and polymeric resins (Conde, Moure, Domínguez, Gordon, et al., Citation2011; Conde et al., Citation2008) was addressed. Adsorption onto polymeric commercial resins and further desorption with ethanol were a promising alternative to obtain concentrates with more than 50% phenolic content, with antioxidant activity equivalent to 1.5 g Trolox per gram of extract (Conde et al. Citation2008).
Studies dealing with the detailed characterization of the purified products and fractions and their food-related applications are ongoing.
Cellulose and lignin-enriched solid for biocomposites’ manufacture
Our research group, as a partner of the INTERREG projects Naturplas I and II, contributed in the investigation to obtain composites applicable in the automotive industry and continued this topic in further research. Autohydrolyzed spent solids from E. globulus wood (denoted as AEW), rice husk (ARH), bamboo fibers (ABF), and Cytisus scoparius wood (ACW) were used as reinforcing materials in the formulation of PLA bio-composites. Autohydrolysis treatments were carried out under non-isothermal conditions in a Parr reactor following the standard heating temperature profile up to a target temperature. Selected liquor to solid ratio was 8:1 kg/kg. Treated solids were recovered by filtration, washed, oven-dried, and vacuum stored before compounding. Liquors obtained can be derived to oligosaccharides, sugars, antioxidants, or for fermentation purposes, as exposed in other paragraphs. Two different PLA were used along the experimentation in the formulation of composite materials: PLA1 NatureWorks 3051, supplied by Cargill Dow LLC, USA, and PLA2, supplied by Hycail Finland Oy, Finland. ISO 527 tensile and impact specimens (Model 1A) were obtained by extrusion-injection and tested to obtain their mechanical properties. Water absorption was determined from relative mass increase of probes when immersed in distilled water.
Supplementary Table 7 shows the impact and tensile results from tests performed to neat PLA and composites made with 30% of autohydrolyzed materials. Young's modules of reinforced composites increase with respect to the neat PLA (PLA1 for ABF and ACW, and PLA2 for AEW and ARH) by 41–74%. Lower values for Young's modules (2.96 and 2.84 GPa for ABF and ACW, respectively) were achieved when the percentage of reinforced materials was 20 (González, Campos, Cunha, Santos, & Parajó, Citation2011; González, Santos, & Parajó, Citation2011). Tensile strength presents a slight decrease in the reinforced composites except in the samples carried out with AEW. All the composites present lower values of strain at break when compared to virgin PLA. So, a more rigid and brittle material is obtained when polymer matrix is reinforced with natural fibers, and this behavior is more profound in the case of PLA1. Higher impact strength was achieved with ACW and ABF, whereas similar values were obtained with ARH and AEW.
Supplementary Figure 6 shows the water absorption for neat PLA, untreated source fibers from C. scoparius wood (SCW), ABF, and ACW-reinforced composite samples. Due to the hygroscopicity of cellulosic fibers, their addition as a reinforcement increased water absorption. As shown in Supplementary Figure 6, similar values, higher than neat PLA, were obtained with the autohydrolyzed fibers ABF and ACW. When compared with composites obtained using untreated source fibers (SCW), the autohydrolysis reduced the water absorption (González, Campos, et al., Citation2011; González, Santos, et al., Citation2011). This reduction in water absorption is ascribed to the selective removal of the more hydrophilic hemicelluloses and increase of lignin in the residual fibers. Higher percentages of reinforced fibers increased the water absorption (González, Campos, et al., Citation2011; González, Santos, et al., Citation2011).
The experimental data confirmed the potential of composites made up of PLA and autohydrolyzed fibers as an environmental friendly alternative to composites obtained from conventional petrochemical thermoplastics and glass or aramid fibers. The use of autohydrolyzed spent solids in the reinforcement of PLA composites resulted in better stiffness and impact strength, reduced strain at break, and good tensile strength.
Perspectives
This overview highlights the potential of the technologies used to valorize biomass from forest, industrial, and agricultural wastes. Different products from food additives, chemicals, composites, or biofuel have been obtained from several raw materials applying diverse procedures of reaction and purification.
tcyt_a_598949_sup_27125791.pdf
Download PDF (1.1 MB)Acknowledgments
This study has had financial support in the framework of the following Research Projects: PPQ2000-0688-C05-02, AGL2003-03596, ALG2006-05387, PGIDIT06 TAL38301PR, AGL2008-02072, CTM2009-12664, 09REM003383PR, which had partial financial support from the FEDER funds of the European Union, and Xunta Galicia (Research Project PGIDT01 PXI38302PN, PGIDT06 TAM38301PR, Red 2006-6), with partial financial support from the FEDER funds of the European Union.
References
- Alonso , J. L. , Garrote , G. , Domínguez , H. , Santos , V. and Parajó , J. C. 2009 . Lactic acid from apple pomace: A laboratory experiment for teaching valorisation of wastes . CyTA – Journal of Food , 7 : 83 – 88 .
- Ares-Peón , I. A. , Vila , C. , Garrote , G. and Parajó , J. C. 2011 . Enzymatic hydrolysis of autohydrolyzed barley husks . Journal of Chemical Technology & Biotechnology , 86 : 251 – 260 .
- Conde , E. 2003 . “ Estudio de la estabilidad de antioxidantes naturales procedentes de residuos agroindustriales ” . In Proyecto Fin de Carrera. Faculty of Sciences of Ourense, University of Vigo
- Conde , E. , Cara , C. , Moure , A. , Ruiz , E. , Castro , E. and Domínguez , H. 2009 . Antioxidant activity of the phenolic compounds released by hydrothermal treatments of olive tree pruning . Food Chemistry , 114 : 806 – 812 .
- Conde , E. , Gullón , P. , Moure , A. , Domínguez , H. and Parajó , J. C. 2009 . Fractionation of industrial solids containing barley husks in aqueous media . Food and Bioproducts Processing , 87 : 208 – 214 .
- Conde , E. , Moure , A. , Domínguez , H. , Gordon , M. H. and Parajó , J. C. 2011 . Purified phenolics from hydrothermal treatments of biomass: Ability to protect sunflower bulk oil and model food emulsions from oxidation . Journal of Agricultural and Food Chemistry, Manuscript submitted for publication ,
- Conde , E. , Moure , A. , Domínguez , H. and Parajó , J. C. 2008 . Fractionation of antioxidants from autohydrolysis of barley husks . Journal of Agricultural and Food Chemistry , 56 : 10651 – 10659 .
- Conde , E. , Moure , A. , Domínguez , H. and Parajó , J. C. 2011 . Production of antioxidants by non-isothermal autohydrolysis of lignocellulosic wastes . LWT – Food Science and Technology , 44 : 436 – 442 .
- Cruz , J. M. , Conde , E. , Domínguez , H. and Parajó , J. C. 2007 . Thermal stability of antioxidants obtained from wood and industrial wastes . Food Chemistry , 100 : 1059 – 1064 .
- Cruz , J. M. , Dominíguez , H. and Parajó , J. C. 2004 . Assessment of the production of antioxidants from winemaking waste solids . Journal of Agricultural and Food Chemistry , 52 : 5612 – 5620 .
- Cruz , J. M. , Domínguez , H. and Parajó , J. C. 2005 . Anti-oxidant activity of isolates from acid hydrolysates of Eucalyptus globulus wood . Food Chemistry , 90 : 503 – 511 .
- Cruz , J. M. , Domínguez , J. M. , Domínguez , H. and Parajó , J. C. 1999 . Solvent extraction of hemicellulosic wood hydrolysates: A procedure useful for obtaining both detoxified fermentation media and polyphenols with antioxidant activity . Food Chemistry , 67 : 147 – 153 .
- Cruz , J. M. , Domínguez , J. M. , Domínguez , H. and Parajó , J. C. 2001 . Antioxidant and antimicrobial effects of extracts from hydrolysates of lignocellulosic materials . Journal of Agricultural and Food Chemistry , 49 : 2459 – 2464 .
- Cunha , A. M. , Campos , A. R. , Cristovão , C. , Vila , C. , Santos , V. and Parajó , J. C. 2006 . Sustainable materials in automotive applications . Plastics, Rubber and Composites , 35 : 233 – 241 .
- Garrote , G. , Cruz , J. M. , Domínguez , H. and Parajó , J. C. 2003 . Valorisation of waste fractions from autohydrolysis of selected lignocellulosic materials . Journal of Chemical Technology & Biotechnology , 78 : 392 – 398 .
- Garrote , G. , Cruz , J. M. , Domínguez , H. and Parajó , J. C. 2008 . Non-isothermal autohydrolysis of barley husks: Product distribution and antioxidant activity of ethyl acetate soluble fractions . Journal of Food Engineering , 84 : 544 – 552 .
- Garrote , G. , Cruz , J. M. , Moure , A. , Domínguez , H. and Parajó , J. C. 2004 . Antioxidant activity of byproducts from the hydrolytic processing of selected lignocellulosic materials . Trends in Food Science & Technology , 15 : 191 – 200 .
- Garrote , G. , Domínguez , H. and Parajó , J. C. 1999 . Hydrothermal processing of lignocellulosic materials . HolzalsRoh – und Werkstoff , 57 : 191 – 202 .
- Garrote , G. , Falqué , E. , Domínguez , H. and Parajó , J. C. 2007 . Autohydrolysis of agricultural residues: Study of reaction byproducts . Bioresource Technology , 98 : 1951 – 1957 .
- Garrote , G. , Kabel , M. A. , Schols , H. A. , Falqué , E. , Domínguez , H. and Parajó , J. C. 2007 . Effects of Eucalyptus globulus wood autohydrolysis conditions on the reaction products . Journal of Agricultural and Food Chemistry , 55 : 9006 – 9013 .
- Garrote , G. , Yáñez , R. , Alonso , J. L. and Parajó , J. C. 2008 . Coproduction of oligosaccharides and glucose from corncobs by hydrothermal processing and enzymatic hydrolysis . Industrial and Engineering Chemistry Research, | , 47 : 1336 – 1345 .
- Gibson , G. R. , Probert , H. M. , Van Loo , J. , Rastall , R. A. and Roberfroid , M. B. 2004 . Dietary modulation of the human colonic microbiota: Updating the concept of prebiotics . Nutrition Research Reviews , 17 : 259 – 275 .
- González , D. , Campos , A. R. , Cunha , A. M. , Santos , V. and Parajó , J. C. 2011 . Manufacture of fibrous reinforcements for biodegradable biocomposites from Citysus scoparius . Journal of Chemical Technology and Biotechnology , 86 : 575 – 583 .
- González , D. , Santos , V. and Parajó , J. C. 2011 . Manufacture of fibrous reinforcements for biocomposites and hemicellulosic oligomers from bamboo . Chemical Engineering Journal , 167 ( 1 ) : 278 – 287 .
- González , J. , Cruz , J. M. , Domínguez , H. and Parajó , J. C. 2004 . Production of antioxidants from Eucalyptus globulus wood by solvent extraction of hemicellulose hydrolysates . Food Chemistry , 84 : 243 – 251 .
- González-Muñoz , M. J. , Álvarez , R. , Santos , V. and Parajó , J. C . (in press). Production of hemicellulosic sugars from Pinus pinaster wood by sequential steps of aqueous extraction and acid hydrolysis . Wood Science and Technology ,
- González-Muñoz , M. J. , Domínguez , H. and Parajó , J. C. 2008 . Depolymerization of xylan-derived products in an enzymatic membrane reactor . Journal Membrane Science , 320 : 224 – 231 .
- González-Muñoz , M. J. , Santos , V. and Parajó , J. C. 2011 . Purification of oligosaccharides obtained from Pinus pinaster hemicelluloses by diafiltration . Desalination and Water Treatment , 27 : 48 – 53 .
- Gullón , B. , Garrote , G. , Alonso , J. L. and Parajó , J. C. 2007 . Production of L-lactic acid and oligomeric compounds from apple pomace by simultaneous saccharification and fermentation: A response surface methodology assessment . Journal of Agricultural and Food Chemistry , 55 : 5580 – 5587 .
- Gullón , B. , Yáñez , R. , Alonso , J. L. and Parajó , J. C. 2010 . Production of oligosaccharides and sugars from rye straw: A kinetic approach . Bioresource Technology , 101 : 6676 – 6684 .
- Gullón , P. , González-Muñoz , M. J. and Parajó , J. C. 2008 . Membrane processing of liquors from Eucalyptus globulus autohydrolysis . Journal of Food Engineering , 87 : 257 – 265 .
- Gullón , P. , González-Muñoz , M. J. and Parajó , J. C. 2011 . Manufacture and prebiotic potential of oligosaccharides derived from industrial solid wastes . Bioresource Technology , 102 : 6112 – 6119 .
- Gullón , P. , González-Muñoz , M. J. , van Gool , M. P. , Schols , H. A. , Hirsch , J. , Ebringerova , A. and Parajó , J. C. 2010 . Production, refining, structural characterization and fermentability of rice husk xylooligosaccharides . Journal of Agricultural and Food Chemistry , 58 : 3632 – 3641 .
- Gullón , P. , González-Muñoz , M. J. , van Gool , M. P. , Schols , H. A. , Hirsch , J. , Ebringerova , A. and Parajó , J. C. 2011 . Structural features and properties of soluble products derived from Eucalyptus globulus hemicelluloses . Food Chemistry , 127 : 1798 – 1807 .
- Hofvendahl , K. and Hähn-Hägerdal , B. 2000 . Factors affecting the fermentative lactic acid production from renewable resources . Enzyme and Microbial Technology , 26 : 87 – 107 .
- IEA (International Energy Agency) . 2009 . Bioenergy Task 42 on Biorefineries , Biorefineries : Co-production of fuels, chemicals, power and materials from biomass . Retrieved from: www.biorefinery.nl/ieabioenergy-task42
- John , R. P. , Anisha , G. S. , Nampoothiri , K. M. and Pandey , A. 2009 . Direct lactic acid fermentation: Focus on simultaneous saccharification and lactic acid production . Biotechnology Advances , 27 : 145 – 152 .
- Martínez , M. , Gullón , B. , Schols , H. A. , Alonso , J. L. and Parajó , J. C. 2009 . Assessment of the production of oligomeric compounds from sugar beet pulp . Industrial and Engineering Chemistry Research , 48 : 4681 – 4687 .
- Martínez , M. , Yáñez , R. , Alonso , J. L. and Parajó , J. C. 2010 . Chemical production of pectic oligosaccharides from orange peel wastes . Industrial and Engineering Chemistry Research , 49 : 8470 – 8476 .
- Marques , S. , Santos , J. A.L. , Gírio , F. M. and Roseiro , J. C. 2008 . Lactic acid production from recycled paper sludge by simultaneous saccharification and fermentation . Biochemical Engineering Journal , 41 : 210 – 216 .
- Moure , A. , Domínguez , H. and Parajó , J. C. 2005 . Antioxidant activity of liquors from aqueous treatments of Pinus radiata wood . Wood Science and Technology , 39 : 129 – 139 .
- Moure , A. , Pazos , M. , Medina , I. , Domínguez , H. and Parajó , J. C. 2007 . Antioxidant activity of extracts produced by solvent extraction of almond shells acid hydrolysates . Food Chemistry , 101 : 193 – 201 .
- National Research Council. 2000 . Biobased industrial products, priorities for research and commercialization , Washington , DC : National Academic Press .
- Parajó , J. C. , Domínguez , H. and Domínguez , J. M. 1997 . Xylitol production from Eucalyptus wood hydrolysates extracted with organic solvents . Process Biochemistry , 32 : 599 – 604 .
- Parajó , J. C. , Domínguez , H. , Moure , A. , Díaz-Reinoso , B. , Conde , E. , Soto , M. L. and González-López , N. 2008 . Recovery of phenolic antioxidants released during hydrolytic treatments of agricultural and forest residues . Electronic Journal of Environmental, Agricultural and Food Chemistry , 7 : 3243 – 3249 .
- Rivas , B. , Moldes , A. B. , Domínguez , J. M. and Parajó , J. C. 2004 . Lactic acid production from corn cobs by simultaneous saccharification and fermentation: A mathematical interpretation . Enzyme and Microbial Technology , 34 : 627 – 634 .
- Romaní , A. , Garrote , G. , Alonso , J. L. and Parajó , J. C. 2010a . Bioethanol production from hydrothermally pretreated Eucalyptus globulus wood . Bioresource Technology , 101 : 8706 – 8712 .
- Romaní , A. , Garrote , G. , Alonso , J. L. and Parajó , J. C. 2010b . Experimental assessment on the enzymatic hydrolysis of hydrothermally pretreated Eucalyptus globulus wood . Industrial & Engineering Chemistry Research , 49 : 4653 – 4663 .
- Romaní , A. , Garrote , G. , López , F. and Parajó , J. C. 2011 . Eucalyptus globulus fractionation by autohydrolysis and delignification . Bioresource Technology , 102 : 5896 – 5904 .
- Soto , M. L. , Moure , A. , Domínguez , H. and Parajó , J. C. 2008 . Charcoal adsorption of phenolic compounds present in distilled grape pomace . Journal of Food Engineering , 84 : 156 – 163 .
- Sreenath , H. K. , Moldes , A. B. , Koegel , R. G. and Straub , R. J. 2001 . Lactic acid production by simultaneous saccharification and fermentation of alfalfa fiber . Journal of Bioscience and Bioengineering , 92 : 518 – 523 .
- Vegas , R. , Alonso , J. L. , Domínguez , H. and Parajó , J. C. 2004 . Processing of rice husk autohydrolysis liquors for obtaining food ingredients . Journal of Agricultural and Food Chemistry , 52 : 7311 – 7317 .
- Vegas , R. , Alonso , J. L. , Domínguez , H. and Parajó , J. C. 2005 . Manufacture and refining of oligosaccharides from industrial solid wastes . Industrial and Engineering Chemistry Research , 44 : 614 – 620 .
- Vegas , R. , Kabel , M. , Schols , H. A. , Alonso , J. L. and Parajó , J. C. 2008 . Hydrothermal processing of rice husks: Effects of severity on product distribution . Journal of Chemical Technology and Biotechnology , 83 : 965 – 972 .
- Vegas , R. , Luque , S. , Álvarez , J. R. , Alonso , J. L. , Domínguez , H. and Parajó , J. C. 2006 . Membrane-assisted processing of xylooligosaccharides-containing liquors . Journal of Agricultural and Food Chemistry , 54 : 5430 – 5436 .
- Vijayakumar , J. , Aravindan , R. and Viruthagiri , T. 2008 . Recent trends in the production, purification and application of lactic acid . Chemical and Biochemical Engineering Quarterly , 22 : 245 – 264 .
- Vila , C. , Campos , A. R. , Cristovão , C. , Cunha , A. M. , Santos , V. and Parajó , J. C. 2008 . Sustainable biocomposites based on autohydrolysis of lignocellulosic substrates . Composites Science and Technology , 68 : 944 – 952 .
- Yáñez , R. , Alonso , J. L. and Parajó , J. C. 2005 . Study on the suitability of untreated corrugated cardboard for D(−)-lactic acid production by SSF using Lactobacillus coryniformis subsp . Torquens. Afinidad , 62 : 295 – 301 .
- Yáñez , R. , Alonso , J. L. and Parajó , J. C. 2006 . Enzymatic saccharification of hydrogen peroxide-treated solids from hydrothermal processing of rice husks . Process Biochemistry , 41 : 1244 – 1252 .
- Yáñez , R. , Romaní , A. , Garrote , G. , Alonso , J. L. and Parajó , J. C. 2009 . Experimental evaluation of alkaline treatment as a method for enhancing the enzymatic digestibility of autohydrolysed Acacia dealbata . Journal of Chemical Technology and Biotechnology , 84 : 1070 – 1077 .
Supplementary material
Supplementary Figure 1. General scheme of a biorefinery process.
Figura adicional 1. Esquema general de un proceso de biorrefinería.
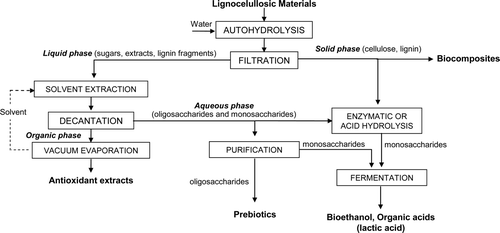
Supplementary Figure 2. Processing scheme for the purification of XOS from barley husks wastes (modified from Gullón, González-Muñoz, and Parajó, Citation2011).
Figura adicional 2. Esquema del proceso para la purificación de xilooligosacáridos (XOS) de residuos de cáscaras de cebada (modificado de Gullón, González-Muñoz, & Parajó, 2011).
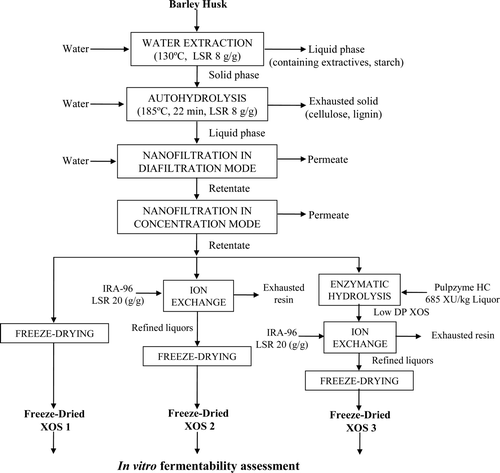
Supplementary . Processing scheme for lactic acid production from LCMs.
Figura adicional 3. Esquema de proceso de producción de ácido láctico procedente de materiales lignocelulósicos.
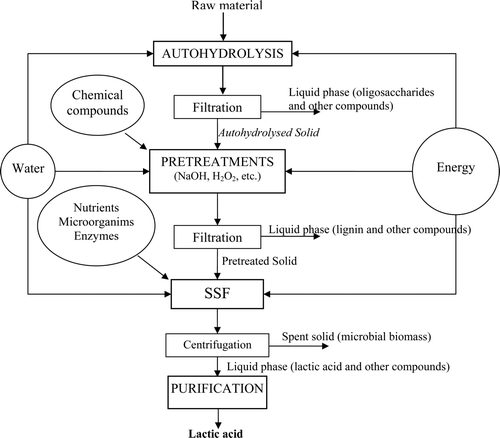
Supplementary . Flow diagram of the proposed processes for recovering the acid-soluble lignin fraction depolymerized during autohydrolysis of LCMs.
Figura adicional 4. Diagrama de flujo del proceso propuesto para la recuperación de la fracción de lignina soluble en ácido despolimerizada durante la autohidrólisis de materiales lignocelulósicos.
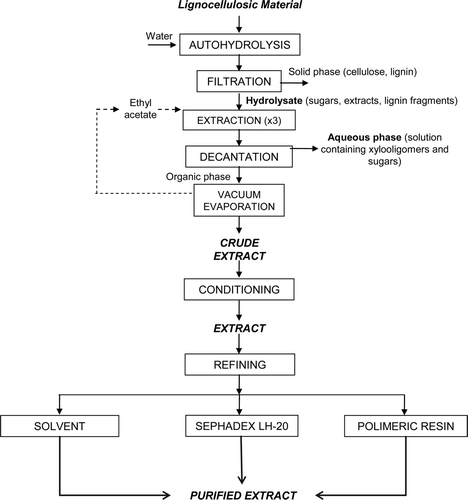
Supplementary . Effect of temperature during autohydrolytic treatment of lignocellulosic wastes (Garrote et al., Citation2003, Citation2008; Moure, Domínguez, & Parajó, Citation2005; Conde, Cara, et al., Citation2009, Conde, Moure, Domínguez, & Parajó, Citation2011).
Figura adicional 5. Efecto de la temperatura durante el tratamiento de autohidrólisis de residuos lignocelulósicos (Garrote et al., 2003, 2008; Moure, Domínguez, and Parajó, 2005; Conde, Cara, et al., 2009, Conde, Moure, Domínguez, and Parajó, 2011).
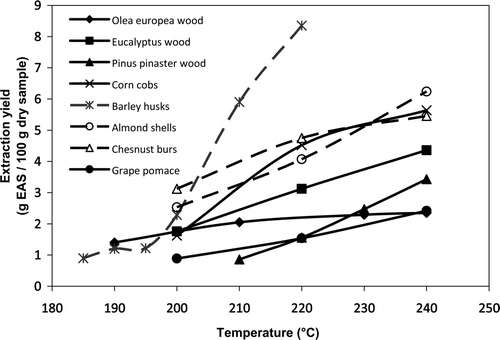