Abstract
Phycobiliproteins are pigment–protein complexes, brilliant-colored and water soluble, existing in cyanobacteria and red algae. They have a great interest in food technology, food supplements, and pharmaceutical applications. Therefore it is important to have a fast, easy, and reliable method to determine the content of phycoerythrin (PE), phycocyanin (PC), and allophycocyanin (APC) in red algae. The samples are ground, extracted in phosphate buffer (pH 6.8), centrifuged, and the fluorescence scan of the supernatant is recorded. Optimum excitation-emission wavelength intervals (Δλ) for synchronous spectrofluorimetric analysis of the three phycobiliproteins were 10 nm for PE (LD = 10.9 × 10−3 mg/l) and APC (LD = 0.5 mg/l) and 40 nm for PC (LD = 0.77 mg/l). Algae analyzed contain PC and PE.
Las ficobiliproteínas son complejos de proteínas y pigmentos, de color brillante y solubles en agua, existentes en las cianobacterias y algas rojas, de gran interés debido al gran número de aplicaciones en la tecnología de los alimentos, complementos alimenticios en la industria farmacéutica y en clínica. Por ello es necesario disponer de un método rápido, fácil y fiable para determinar el contenido de ficoeritrina, ficocianina y aloficocianina en algas rojas. Las muestras se trituran y se extraen en tampón fosfato (pH 6,8), se centrifugan y se registra el espectro espectrofluorimétrico del sobrenadante. El intervalo óptimo entre la longitud de onda de excitación y emisión (Δλ) para el análisis mediante espectrofluorimetría sincrónica de las tres ficobiliproteínas fué 10 nm para la ficoeritrina (LD = 10,9 × 10−3 mg/l) y aloficocianina (LD = 0,5 mg/l) y 40 nm para la ficocianina (LD = 0,77 mg/l). Se ha determinado la presencia de ficocianina y ficoeritrina en las algas analizadas.
Introduction
Phycobiliproteins or biliproteins are a group of proteins with covalently attached linear tetrapyrrole chromophoric groups (Básaca-Loya, Valdez, Enríquez-Guevara, Gutierrez Millán, & Burboa, Citation2009; Viskari & Colyer, Citation2003). In cyanobacteria and red algae, the phycobiliproteins are organized in supramolecular complexes, called phycobilisomes which are assembled in regular arrays on the outer surface of the thylakoid membranes (MacColl et al., Citation1999; Mishra, Shivastav, Pancha, Jain, & Mishra, Citation2010; Sekar & Chandramohan, Citation2008; Zhao & Qin, Citation2006).
Phycobiliproteins are attractive since they are not harmful to human beings if they are applied to external surface or ingested. Phycobiliproteins are chromoproteins that have anti-inflammatory, hepato-protective and antioxidant properties (Holdt & Kraan, Citation2011). Purified phycobiliproteins have a variety of uses such as colorants in food, health drinks, and coloring agent in sweet confectionary and cosmetic in Japan, Tailand, and China (Mishra et al., Citation2010). They are used most widely as fluorescent labels for cells and macromolecules in highly sensitive fluorescence techniques (Mishra et al., Citation2010; Viskari & Colyer, Citation2003).
The phycobiliproteins themselves are water-soluble, very stable at physiological pHs, and highly fluorescent proteins. They are usually divided into three separate groups based on their color and absorption properties, namely phycoerythrins (PEs), phycocyanins (PCs), and allophycocyanins (APCs). The PEs appear bright pink color, PCs are dark cobalt blue, and APCs are brighter aqua blue. The absorption maxima are 540–570, 610–620, and 650–655 nm for PE, PC, and APC, respectively (Básaca-Loya et al., Citation2009; Isailovic, Sultana, Phillips, & Yeung, Citation2006; Lawrenz & Fedewa, Citation2011; Liu, Chen, Zhang, Zhang, & Zhou, Citation2005; Liu, Su et al., 2009; Mishra et al., Citation2010; Niu, Wang, & Tseng, Citation2006; Sun, Wang, Chen, & Gong, Citation2003).
Phycoerythrin is characterized by intensive fluorescence emissions at about 575 nm. Phycocyanin is one of the most widespread phycobiliproteins in almost all phycobiliprotein-containing organisms. These blue or blue-purple-colored phycobiliprotein emit intensive red fluorescence at about 640 nm. Allophycocyanin is a minor contained phycobiliprotein species with respect to all the others, but it exists in almost red algae growing in natural environment. The phycobiliproteins are high fluorescence quantum efficiency in a broad range of pH values of neutral solution and large stokes shift (MacColl et al., Citation1999; Sun & Wang, Citation2000; Sun et al., Citation2003). Efficient excitation energy coupling among the chromophores in the phycobiliproteins trimer/hexamer and among the phycobiliproteins in the phycobilisome gives them some special spectroscopic properties superior to organic dyes. When the phycobiliproteins are isolated from the organisms, the proteins become highly fluorescent because they no longer have any nearby acceptors to which to transfer the harvested energy.
In red algae, there is a need for a quick, easy and reliable method for determining PE and PC contents. One of the most frequently cited phycobilin quantification techniques utilize the spectral properties of crude aqueous extracts to estimate the pigments content of sample tissues. The samples are ground, extracted in phosphate buffer, centrifuged, and the absorbance values or fluorescence of the supernatant corresponding to peaks and troughs of phycobiliproteins are recorded (Básaca-Loya et al., Citation2009; Denis et al., Citation2010; Liu, Chen et al., 2005; Sampath-Wiley & Neefus, Citation2007; Sun & Wang, Citation2000; Xu & Gao, Citation2008).
This paper describes a constant-wavelength synchronous scan spectrofluorimetric method for the individual determination of the three phycobiliproteins very abundant in red algae: PE, PC, and APC. It is of great interest to have fast and reliable methods for identification and quantification of phycobiliproteins in algae due to their great importance in food technology, food supplements, and pharmacological and clinical applications.
Materials and methods
Chemicals and standard solutions
Potassium dihydrogen phosphate and sodium hydrogen phosphate were from Merck (Darmstadt, Germany) and were used for the preparation of buffer phosphate (pH 6.8). Standards of B-PE (Fluka, Stinheim, Germany), C-PC (Sigma, St. Louis, MO, USA), and APC (Fluka) were supplied by Sigma-Aldrich (Steinheim, Germany). Water used for all solutions was obtained from a Milli-Q water purification system (Millipore, Bedford, MA, USA).
Stock standard solutions of PE, PC, and APC and their mixtures were prepared in buffer phosphate, pH 6.8.
Samples
In this paper six samples are analyzed of two different red seaweeds: Porphyra umbilicalis (Nori) and Palmaria palmata (Dulse). Dried samples packed in polypropylene bags of about 100 g are marketed by a factory in Galicia and were collected on the Atlantic coast (Northwest Spain).
For the extraction of phycobiliproteins from the samples, around 100 mg of sample previously ground are heavy and carefully crushed in a porcelain mortar by adding drops of phosphate buffer. The solution is transferred to a graduated test tube and make up to 10 ml with phosphate buffer. The mixture was vortexed (Ika Vortex VG3; Ika-Werke, Staufen, Germany) for 15 min and then centrifuged (Eba 12 centrifuge; Hettich, Kirchlengern, Germany) for 3 min at 1500 rpm. The phycobiliproteins were extracted from the supernatant.
For identification and quantification have been used fluorescent properties of phycobiliproteins. All spectrofluorimetric measurements were performed in a Perkin-Elmer LS-50 luminescent spectrometer (BeakonsWeld, Buckinghamshire, UK) equipped with a xenon discharge lamp, Monk-Gillieson momochromators and 1 cm quartz cuvettes with Teflon stoppers. Spectral data acquisition and processing were carried out by means of the program Fluorescence Data Manager software; the instrument was serially interfaced (RS232C) to a PC.
Working conditions
For each of the above solutions excitation spectra between 200 and 700 nm at a scan speed 240 nm/min and with excitation slit width 2.5 nm and emission slit width 5 nm were recorded. The excitation–emission wavelength differences (Δλ) were 10 and 40 nm.
The moisture content of all samples were determined by drying in an oven at 100°C for 24 h.
Results and discussion
Phycobiliproteins extraction
Phycobiliproteins extraction is usually done by grinding apparatus (porcelain mortar and pestle), and extraction times in phosphate buffer ranging from 2 to 48 h. Following extraction at 4°C, the samples were centrifuged.
Extraction is normally carried out with buffer phosphate at different pH. Phycoerythrin displays a good stability to pH variations between pH 3.5 and 9.5. In the fluorescence spectra, the emission maxima decreased about 10% along with the pH value fell from 7.0 to 4.4; at the same time, the emission wavelength red-shifted a little from 580 to 581 nm (Liu, Chen et al., 2005; Liu, Su et al., 2009; Sun & Wang, Citation2000; Sun et al., Citation2009). Phycoerythrin was found to be sensitive to heat and light in aqueous solution; PE is insoluble in acidic solution (pH 3.0) and is highly soluble and stable at pH 7.0. When phycobilisomes are exposed to dilute buffers with pH 6–7, the phycobilisomes dissociate into their components, and individual biliproteins can be identified and purified (Lawrenz & Fedewa, Citation2011; Liu, Chen et al., 2005; MacColl, Citation2004; Mishra et al., Citation2010; Sampath-Wiley & Neefus, Citation2007).
Spectrofluorimetry
Phycobiliproteins are highly fluorescent proteins with absorption coefficients and quantum yields that are superior compared with other fluorescent proteins. They exhibit a large Stokes shift which is very important (Liu, Chen et al., Citation2005; Niu et al., Citation2006; Sun et al., Citation2003).
This property has been used for the identification and quantification of the three phycobiliproteins. A specific problem for phycobilins detection is that their excitation and emission maxima are typically very close to each other but in order to reject stray light, a reasonable separation between excitation and detection wavelengths is required. Shifting the wavelengths away from the actual peak reduces the stray light effect, but decreases the signal intensity as well (Seppälä et al., Citation2007). This problem will solve with the synchronous spectrofluorimetry. This technique offers several advantages over the others methods: analysis is rapid and so allows a high throughput of samples; the solvent consumption is limited to that required for the preparation of the sample; the sensitivity is much better than that of UV–visible absorption methods. The selectivity of the technique is very high not only by the virtual absence of non-fluorescent interferences in the sample, but also by the simultaneous scanning of both the excitation and emission monochromators, which affords narrower bands than in conventional spectrofluorimetry and virtually eliminates interference from Rayleigh scattering.
The optimum excitation–emission wavelength for each phycobiliprotein was obtained by excitation and emission scanning in buffer phosphate pH 6.8 (). The optimum excitation–emission wavelength difference for each phycobiliprotein was obtained by excitation and emission of 40 scans of the solutions between 200 and 700 nm, with the difference set at 10 nm (scan 1) and increasing by 5 nm this difference for each subsequent scan. Optimum excitation–emission wavelength intervals (Δλ) for synchronous spectrofluorimetric analysis of the three phycobiliproteins were 10 nm for B-PE and APC and 40 nm for C-PC ().
Figure 1. Constant-wavelength synchronous fluorescence spectra (Δλ = 10 nm), of (A) 1 – buffer, 2 – standard solution of PE, PC, and APC in buffer and constant-wavelength synchronous fluorescence spectra (Δλ = 40 nm), 3 – buffer, 4 – standard solution of PE, PC, and APC in buffer and (B) their respective zoom.
Figura 1. Espectrofluorimetría sincrónica con diferencia constante de longitud de onda (Δλ = 10 nm), de A: 1 – tampón 2 – solución estándar de PE, PC y APC en tampón y espectrofluorimetría sincrónica con diferencia constante de longitud de onda (Δλ = 40 nm), de, 3 – tampón, 4 – solución estándar de PE, PC y APC en tampón y B: su zoom [acercamiento] respectivo.
![Figure 1. Constant-wavelength synchronous fluorescence spectra (Δλ = 10 nm), of (A) 1 – buffer, 2 – standard solution of PE, PC, and APC in buffer and constant-wavelength synchronous fluorescence spectra (Δλ = 40 nm), 3 – buffer, 4 – standard solution of PE, PC, and APC in buffer and (B) their respective zoom. Figura 1. Espectrofluorimetría sincrónica con diferencia constante de longitud de onda (Δλ = 10 nm), de A: 1 – tampón 2 – solución estándar de PE, PC y APC en tampón y espectrofluorimetría sincrónica con diferencia constante de longitud de onda (Δλ = 40 nm), de, 3 – tampón, 4 – solución estándar de PE, PC y APC en tampón y B: su zoom [acercamiento] respectivo.](/cms/asset/0114a309-1560-49d0-bcd2-977d1584736f/tcyt_a_728629_o_f0001g.jpg)
Table 1. Excitation and emission wavelength optimum.
Tabla 1. Longitudes de onda de excitación y de emisión óptimas.
For quantification, the calibration lines were constructed by regressing the peak obtained against the concentrations of the working solutions. Following the recommendations of the American Chemical Society's Subcommittee on Environmental Analytical Chemistry, limits of detection were calculated as the concentrations corresponding to the mean blank signal + three standard deviations (). There were no signs of interference from phosphate buffer used as solvent of standard working solutions and as extraction solvent ().
Table 2. Regression and correlation coefficients for the calibration lines obtained by synchronous spectrofluorimetry at the indicated values of Δλ, limits of detection (LD), precision (RSD), and recoveries (%R) for phycobiliproteins.
Rectas de calibrado, λexc, límite de detección (LD), precisión (RSD) y recuperación (%R) de las ficobiliproteínas analizadas por espectrofluorimetría sincrónica.
Precision and recovery
Method precision was determined by applying the full procedure to six replicate samples of algae Nori (Porphyra umbilicalis) from the same lot. Recoveries, estimated on the basis of determinations after spiking samples of brown algae (Undaria pinnatifida, without phycobiliproteins) with amounts of standards containing 0.2, 2, and 2 μg/ml of PE, PC, and APC, respectively. lists the precisions (%RSD) and recoveries (%R) obtained.
Levels found in dry algae
The moisture content of samples was 11.28 ± 0.67 and 13.71 ± 0.14 for algae Nori and Dulse, respectively.
The results (; ) have shown that the phycobiliprotein PC is the most abundant in the algae studied, followed by PE. Allophycocyanin has been not detectable.
Figure 2. Constant-wavelength synchronous fluorescence spectra (Δλ = 10 nm) of (A) 1 – buffer, 2 – Nori 2 in buffer and constant-wavelength synchronous fluorescence spectra (Δλ = 40 nm), 3 – buffer, 4 – Nori 2 in buffer and (B) zoom of Nori spectra.
Figura 2. Espectrofluorimetría sincrónica con diferencia constante de longitud de onda (Δλ = 10 nm), de A: 1 – tampón, 2 – Nori 2 en tampón y espectrofluorimetría sincrónica con diferencia constante de longitud de onda (Δλ = 40 nm), de 3 – tampón, 4 – Nori 2 en tampón y B: zoom de espectro Nori.
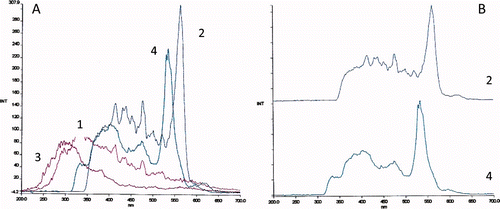
Table 3. Results of phycoerythrin (PE) and phycocyanin (PC) obtained in algae analyzed (mg/100g dry weight).
Tabla 3. Resultados de ficoeritrina (PE) y ficocianina (PC) en las algas analizadas (mg/100g peso seco).
Phycobilins found in these samples are also mainly found by other authors in other red algae, and generally extracted in phosphate buffer solutions (Benedetti et al., Citation2006; Sampath-Wiley & Neefus, Citation2007; Sun & Wang, Citation2000; Sun et al., Citation2003; Xu & Gao, Citation2008).
The results are variable in seaweed Dulse and Nori. It may be due to proteins are subject to large variations during the year. The samples analyzed are commercial and we do not know the date of collection. The photosynthetic membrane and its supramolecular components in red algae vary with light intensity. The phycobiliproteins in some red macroalgae were reported to show complementary chromatic adaption to different light. However, the effect of sun exposure on PE and PC was determined to be reliant on time of year the samples were collected. From May through October, sun exposed plants contained less phycobilins compared to all other collection dates. R-PE and R-PC contents during the late fall to spring months (November–April) did not vary among sun exposures (Denis et al., Citation2010; Martinez & Rico, Citation2008; Sampath-Wiley et al., Citation2008; Su, Xie, Zang, Zhou, & Zhang, Citation2010; Xu & Gao, Citation2008; Zhang et al., Citation2011).
On the other hand, in this work, we have analyzed dry algae. Another time, we analyzed fresh algae and the results were much higher than in dry samples – more than 100 times – and there is a very large decrease of its content over time.
Algae can be used in normally food use and special regimes with a unique place in the nutrition compared to other vegetables and other sources of nutrients (Denis et al., Citation2010; Marfaing & Lerat, Citation2007). Phycobiliproteins have antioxidant properties (Holdt & Kraan, Citation2011) and could be used in the prevention or treatment of degenerative diseases: cardiovascular, certain cancers, and eye linked to oxidative stress. Phycobiliproteins are extensively commercialized for fluorescent applications in clinical and immunological analysis. They are also used as a colorant, and their therapeutic value has also been categorically demonstrated (Sekar & Chandramohan, Citation2008). In literature, known methods of extraction and purification of phycobiliproteins (Básaca-Loya et al., Citation2009; Benedetti et al., Citation2006; Viskari & Colyer, Citation2003), however, a comprehensive knowledge and technological base for augmenting their commercial utilities is lacking. The future depends on the expansion of the range of products to be manufactured as well as improvements in bioprocess engineering. Phycobiliproteins have special spectroscopic properties, superior to many organic fluorescent dyes, which make phycobiliproteins a promising fluorescent probe for medical tagging such as photodynamic therapy on tumor. Their spectral characters and high antioxidant activity appeared to be a promising candidate for antioxidant nutritional supplements, a therapeutic agent in oxidative stress-induced diseases and potential probes (Guan et al., Citation2009; Sun et al., Citation2003).
Conclusions
This paper utilizes the special spectroscopic properties of aqueous extracts of phycobiliproteins to estimate the pigment contents and describes a constant-wavelength synchronous spectrofluorimetry method for the individual determination of the three phycobiliproteins, very abundant in red algae. The method is quick, easy, and reliable for determining PE, PC, and APC content in red algae. The developed method is simple, fast, inexpensive, and sensitive and has a good reproducibility and mainly free of organic solvent. The identification and quantification of these pigments is very interesting for the large number of applications, in the field of nutrition, food technology, clinical and immunological analysis, and pharmaceutical agent.
Acknowledgements
This work was supported by the Project 09TAL023203PR funded by the Xunta de Galicia. The technical assistance of P. Ferraces Casais is gratefully acknowledged.
References
- Básaca-Loya , G.A. , Valdez , M.A. , Enríquez-Guevara , E.A. , Gutierrez-Millán , L.E. and Burboa , M.G. 2009 . Extraction and purification of B-phycoerythrin from the red microalga Rhodosorus marinus, Ciencias Marinas . International Journal of Marine Science , 35 : 359 – 368 .
- Benedetti , S. , Rinadulcci , S. , Benvenuti , F. , Francogli , S. , Pagliarani , S. , Giorgi , L. … and Canestrari , F. 2006 . Purification and characterization of phycocyanin from the blue-green alga, Aphanizomenon flos-aquae . Journal of Chromatography B , 833 : 12 – 18 .
- Denis , C. , Morançais , M. , Li , M. , Deniaud , E. , Gaudin , P. , Wielgosz-Collin , G. … and Fleurence , J. 2010 . Study of the chemical composition of edible red macroalgae Grateloupia turuturu from Brittany (France) . Food Chemistry , 119 : 913 – 917 .
- Guan , X. , Zhang , W. , Zhang , X. , Li , Y. , Wang , J. and Lin , H. 2009 . A potent antioxidant property fluorescent recombinant alpha-phycocyanin of Spirulina . Journal of Applied Phycology , 106 : 1093 – 1100 .
- Holdt , S.L. and Kraan , S. 2011 . Bioactive compounds in seaweed: Functional food applications and legislation . Journal of Applied Phycology , 23 : 543 – 597 .
- Isailovic , D. , Sultana , I. , Phillips , G.J. and Yeung , E.S. 2006 . Formation of fluorescent proteins by the attachment of phycoerythrobilin to R-phycoerythrin alpha and beta apo-subunits . Analytical Biochemistry , 358 : 38 – 50 .
- Lawrentz , E. and Fedewa , E.J. 2011 . Extraction protocols for the quantification of phycobilins in aqueous phytoplankton extracts . Journal of Applied Phycology , 23 : 865 – 871 .
- Liu , L.N. , Chen , X.L. , Zhang , X.Y. , Zhang , Y.Z. and Zhou , B.C. 2005 . One-step chromatography method for efficient separation and purification of R-phycoerythrin from Polysiphonia urceolata . Journal of Biotechnology , 116 : 91 – 100 .
- Liu , L.N. , Su , H.N. , Yan , S.G. , Shao , S.M. , Xie , B.B. , Chen , X.L. … and Zhang , Y.Z. 2009 . Probing the pH sensitivity of R-Phycoerythrin: Investigation of active-conformational and functional variation . Biochimica and Biophysica Acta , 1787 : 939 – 946 .
- MacColl , R. 2004 . Allophycocyanin and energy transfer . Biochimica and Biophysica Acta , 1657 : 73 – 81 .
- MacColl , R. , Eisele , L.E. , Malak , H. , Endres , R.L. , Williams , E.C. and Bowser , S.S. 1999 . Studies on R-phycoerytrins from two Antartic marine red algae and a mesophilic red alga . Polar Biology , 22 : 384 – 388 .
- Marfaing , H. and Lerat , Y. 2007 . Les algues ont ells une place en nutrition? . Phytoterapie , HS : HS2 – HS5 .
- Martinez , B. and Rico , J.M. 2008 . Changes in nutrient content of Palmaria palmate in response to variable light and upwelling in northern Spain . Journal of Phycology , 44 ( 1 ) : 50 – 59 .
- Mishra , S. , Shrivastav , A. , Pancha , I. , Jain , D. and Mishra , S. 2010 . Effect of preservatives for food grade C-Phycoerythrin, isolated from marine Cyanobacteria Pseudanabaena sp . International Journal of Biological Macromolecules , 47 : 597 – 602 .
- Niu , J.F. , Wang , G.C. and Tseng , C.K. 2006 . Method for large-scale isolation and purification of R-phycoerythrin from red alga Polysiphonia urceolata Grev . Protein Expression and Purification , 49 : 23 – 31 .
- Sampath-Wiley , P. and Neefus , C.D. 2007 . An improved method for estimating R-Phycoerythrin and R-Phycocyanin contents from crude aqueous extracts of Porphyra (Bangiales, Rhodophyta) . Journal Applied Phycology , 19 : 123 – 129 .
- Sampath-Wiley , P. , Neefus , C.D. and Jahnke , L.S. 2008 . Seasonal effects of sun exposure and emersion on intertidal seaweed physiology: Fluctuation in antioxidant contents, photosynthetic pigments and photosynthetic efficiency in the red algae Porphyra umbilicalis Kützing (Rodophyta, Bangiales) . Journal of Experimental Marine Biology and Ecology , 361 : 83 – 91 .
- Sekar , S. and Chandramohan , M. 2008 . Phycobiliproteins as a commodity: Trends in applied research, patents and commercialization . Journal Applied Phycology , 20 : 113 – 136 .
- Seppälä , J. , Ylöstalo , P.Y. , Kaitala , S. , Hällfors , S. , Raateoja , M. and Maunula , P. 2007 . Ship-of-opportunity based phycocyanin fluorescence monitoring of the filamentous cyanobacteria bloom dynamics in the Baltic sea . Estuarine Coastal and Shelf Science , 73 : 489 – 500 .
- Su , H. , Xie , B. , Zang , X. , Zhou , B. and Zhang , Y. 2010 . The supermolecular architecture function and regulation of thylacoid membranes in red algae: An overview . Photosynthesis Research , 106 : 73 – 87 .
- Sun , L. and Wang , S. 2000 . A phycoerythrin-allophycocyanin complex from the intact phycobilisomes of the marine red alga Polysiphonia urceolata . Photosyntetica , 38 : 601 – 605 .
- Sun , L. , Wang , S. , Chen , L. and Gong , X. 2003 . Promising fluorescent probes from phycobiliproteins . IEEE Journal of Selected Topics in Quantum Electronics , 9 : 177 – 188 .
- Sun , L. , Wang , S. , Gong , X. , Zhao , M. , Fu , X. and Wang , L. 2009 . Isolation, purification and characteristics of R-phycoerythrin from a marine macroalga Heterosiphonia japonica . Protein Expression and Purification , 64 : 146 – 154 .
- Xu , . and Gao , K. 2008 . Growth, pigments, UV-absorbing compounds and agar yield of the economic red seaweed Gracilaria lemaneiformis (Rodophyta) grown at different depths in the coastal waters of South China sea . Journal Applied Phycology , 20 : 681 – 686 .
- Viskari , P.J. and Colyer , C.L. 2003 . Rapid extraction of phycobiliproteins from cultured cyanobacteria samples . Analytical Biochemistry , 319 : 263 – 271 .
- Zhang , T. , Shen , Z. , Xu , P. , Zhu , J. , Lu , Q. , Shen , Y. … and Jiang , H. 2011 . Analysis of photosynthetic pigments and chlorophyll fluorescence characteristics of different strains of Porphyra yezoensis . Journal Applied Phycology , doi: 10.1007/s10811-011-9708-x
- Zhao , F. and Qin , S. 2006 . Evolutionary analysis of phycobiliproteins: Implications for their structural and functional relationships . Journal of Molecular Evolution , 63 : 330 – 340 .