ABSTRACT
Starch-based foams (SBFs) were prepared with corn, potato, tapioca, and chayotextle starches. A compression baking process was used to develop the SBFs. Biodegradation studies showed that different sources of starch have a particular behavior. The starches showed values of degradation of ~85%, while the degradation of SBFs was ~70%. The SBFs were conditioned at relative humidities (RHs) of 0 and 75% and temperatures of 4 and 65°C. The starch source used to prepare the SBFs did not have an effect on the mechanical properties, but the storage conditions showed a significant effect on those properties; an increase of RH causes an increase in the mechanical properties. A similar behavior was observed in the conditioned SBFs at different temperatures. The increase of the storage temperature showed a slight reduction in the values of elongation at break. Microscopy revealed that the conditions of RH and storage temperature affect the internal structure of the SBFs.
RESUMEN
El material tipo espuma (MTE) fue preparado con almidón de maíz, patata, tapioca y chayotextle. Se utilizó un proceso de termo-compresión para elaborar el MTF. Los estudios de biodegradación mostraron que las diferentes fuentes de almidón tienen un particular comportamiento. Los almidones mostraron valores de degradación de ~ 85%, mientras que en el MTF fue de ~ 70%. Los MTF fueron acondicionados a humedades relativas (HR) de 0 y 75% y a temperaturas de 4 y 65°C. Las fuentes de almidones utilizados para preparar el MTF no afectaron a las propiedades mecánicas, pero las condiciones mostraron un significante efecto sobre estas propiedades; un incremento de la HR causa un incremento en las propiedades mecánicas. Similar comportamiento fue observado en el MTF acondicionado a diferentes temperaturas. El incremento en la temperatura de acondicionamiento mostro una ligera reducción en los valores de elongación a la ruptura. La microscopía reveló que las condiciones de HR y temperatura de almacenamiento afectan a la estructura interna de los MTF.
1. Introduction
Food packaging made of petroleum-based polymers has an impact on the environment because they are derived from a non-renewable resource and have poor biodegradability. One of the most common examples is polystyrene, which is used in foam containers for packaging food because of its high strength, low density, and low cost (Glenn & Orts, Citation2001). Currently, consumers are increasingly interested in environmentally friendly packaging (Shogren, Lawton, Doane, & Tiefenbacher, Citation1998). These biodegradable materials include polymers made by microbial systems, plants and higher organisms, and polymers produced by chemical polymerization of biological starting materials (US Congress, Citation1993). However, their high cost and limited quantity make their use in single-use food containers prohibitive. Polysaccharides are friendly to the environment, because they can biodegrade without leaving behind harmful residues associated with synthetic polymers (Šimkovic, Citation2013). Polysaccharides are considered to be good alternatives for biodegradable plastics because they are widely distributed in nature and are regarded as structural materials (Doane, Citation1992). Starch is considered to be one of the most popular polysaccharides for foam packaging applications because it is a renewable resource, abundant, inexpensive, non-toxic, and easily biodegradation material (Xu, Hanna, & Nag, Citation2005; Stevens, Klamcznski, & Glenn, Citation2010). Unfortunately, starch foam is sensitive to moisture, changes under temperature conditions, and has poor mechanical properties (Cinelli, Chiellini, Lawton, & Imam, Citation2006; Lee & Hanna, Citation2008). Several strategies are investigated to overcome or even minimize these drawbacks. Glenn and Irving (Citation1995) reported excellent thermal properties, using a solvent exchange process. The mechanical properties and a reduction in moisture sensitivity were improved by the latex, fiber, and chitosan addition (Kaisangsri, Kerdchoechuen, & Laohakunjit, Citation2012; Shey, Imam, Glenn, & Orts, Citation2006); an increase in flexibility was improved by incorporating chemical or genetically modified starches (Shogren, Lawton, & Tiefenbacher, Citation2002). Nevertheless, a more efficient way of making starch foams is by an extrusion process (Harper & Tribelhorn, Citation1992), which nowadays is used to make loose-fill as a commercial packaging product. These types of foams have useful mechanical properties, they are difficult to mold into shaped products. There are reports that the compression/explosion process may be used to development SBF materials with commercial potential (Glenn & Orts, Citation2001).
However, it is not clear if foams made of starch composites have the functional properties needed for commercial food containers. A fundamental understanding of the physical and mechanical properties, that are important in the functionality of hinged food containers, is important in evaluating baked foam composites and optimizing formulations. Studies report that the moisture content (MC), starch content, and source of starch used in dough formulations affected the density, flexibility, and strength of baked foams (Lawton, Shogren, & Tiefenbacher, Citation1999; Shogren et al., Citation1998). The use of unconventional source of starch and different storage conditions can contribute to greater understanding of the materials produced by thermo-compression.
Chayote plants produce an edible, starch-rich tuberous root (in Mexico they are called “chayotextle,” “cueza,” “camochayote”, and “chinchayote”). There is literature describing chayotextle for use in baby food and for medicinal purposes (Flores, Citation1989; Yang & Walters, Citation1992). However, in Mexico, there is no official information about the harvesting of chayotextle. The farmers of chayote in Tulancingo, Hidalgo, Mexico, reported tuber (chayotextle) production of up to four tubers per plant, weighing an average of 6.5 kg (Jiménez-Hernández, Salazar-Montoya, & Ramos-Ramírez, Citation2007). Therefore, ‘‘chayotextle’’ can be an attractive agronomic alternative feedstock.
There is limited information on chayotextle starch and its processability. Hernandez-Uribe, Agama-Acevedo, Gonzalez-Soto, Bello-Pérez, and Vargas-Torres (Citation2011) reported a starch yield of 0.55 kg/kg of chayotextle. The same authors described the differences in peak viscosity, gelatinization temperature, and molecular weight when chayotextle starch was compared with potato starch. Aila-Suárez et al. (Citation2013) showed that solution cast films containing either chayotextle or potato starch exhibited a similar behavior. Chayotextle starch can be a good substitute for potato starch in cast films. Similar studies on cast films with chayotextle starch are reported by Cotonieto-Morales et al. (Citation2015) and Terrazas-Hernández et al. (Citation2015). However, little information is available regarding the use of a new source of starch and storage conditions on the physical and mechanical properties of SBF products. The objective of the present study was to determine whether the sources of starch or storage conditions affected the mechanical and structural properties, and biodegradability of starch-based foams.
2. Materials and methods
2.1. Materials
Chayote (Sechium edule Sw.) tubers were collected in Tulancingo, Hidalgo, Mexico. Unmodified and pregelatinized potato starches were purchased from Avebe (Veendam, Holland). Unmodified dent corn and tapioca starches (PenCook 50) were provided by Penford Products (Englewood, CO). Bleached softwood fiber pulp (Leaf River 90) was provided by Georgia Pacific (Atlanta, GA). Magnesium stearate (MgStr) was purchased from Aldrich (Milwaukee, WI). Calcium carbonate (CaCO3) was provided by English China Clays International (Sylacauga, AL).
2.2. Isolation of chayotextle starch
The starch was isolated following the methodology by Flores-Gorosquera et al. (Citation2004). In brief, tubers were cut into 2 × 2 cm cubes and immediately macerated at low speed in a blender (500 g root: 500 g tap water) for 2 min. The homogenate was consecutively sieved, using 50, 100, 200, 270 and 325 US mesh, and washed until the washing water was clean. The starchy solution was pelleted overnight and then decanted. This material was dried in a convection oven at 35°C overnight. Dry starch was ground to a powder in a blender and then sieved through a standard 100 mesh. The starch powder was stored in a sealed container until used.
2.3. Starch-based dough preparation
A mixture of softwood fiber (8.8 g), CaCO3 (30.2 g), and 75 g of water were placed in the metal mixing bowl of a Hobart laboratory mixer (Model N-50, Hobart Mfb., Troy, OH). The materials were mixed at the highest setting for 15 min until the mixture was homogenous. Water (37.6 g) and pregelatinized potato starch (7.6 g) were then added to the blend while mixing at medium speed until the water component was dispersed. The materials were then mixed at the highest setting for 15 min to adequately disperse the fiber and produce homogenous dough. Finally, starch (37.8 g) from different sources (potato, corn, tapioca, or chayotextle) and MgStr (3 g) were added and mixed as described earlier to obtain homogeneous dough.
2.4. Baking process (thermo-pressing)
A laboratory-baking machine (ZQe Mini Hebenstreit GmbH, Germany) was used to bake rectangular starch-based foam (SBF) samples 0.2 cm in thickness, 16.5 cm in length, and 11.1 cm in width. The mold was preheated and maintained at 190°C. Foam panels were made by placing a dough sample (30 g) in the center of the mold, quickly closing the mold and baking for 1 min. Afterwards, the SBFs were removed from the baking machine and stored in a sealed plastic bag at 25 ± 2°C.
2.5. Density
The density (g/cm3) was calculated from the mass (g) and volume (cm3) of each sample (Shogren et al., Citation1998). Density tests were performed with rectangular strips measuring 100 by 25 mm. Each sample was weighed, and the volume was calculated by multiplying the length, width, and thickness together. The reported density values were the averages of five samples per formulation.
2.6. Storage condition of SBF samples
The SBF samples were prepared as per American Society for Testing Materials (ASTM) D 638 M–93 (Citation1993a). These conditions were used to study the effect of the starch source on mechanical properties. The effect of RH was studied by conditioning SBF samples for 1 week at 0 and 75% RH using silica-gel and a saturated NaCl solution, respectively. Meanwhile, the effect of temperature was studied by conditioning the samples at 4 and 65°C for a week.
2.7. Mechanical properties
The mechanical properties of load tensile strength (Ts), percent elongation at break (Eb), and elastic module (Em) were studied. The maximum breaking force, the deformation at break (extension at the moment of rupture, mm), and the elastic modulus were obtained from the force versus deformation curves using a universal testing machine (Instron, Model 4500, Canton, MA), equipped with 50-kg load cell. The panels were cut by a band saw into standard type I tensile bars (ASTM, Citation1993b). Tensile tests were performed at a deformation rate of 1 mm/min. Each SBF formulation was tested 15 times, and the reported value is the average.
2.8. Scanning electron microscopy (SEM)
SBF samples were mounted onto aluminum specimen stubs using double-sided carbon tabs (Ted Pella, Redding, CA). The surface and internal structure of the samples were observed. All specimens were coated with gold–palladium for 45 s in a Denton Desk II sputter coating unit (Denton Vacuum U.S.A., Moorestown, NJ). Specimens were viewed in a Hitachi S4700 field emission scanning electron microscope (Hitachi HTA, Japan) at 2 kV.
2.9. Biodegradability test
Samples were milled to an average particle size of 1 µm using a hammer mill. Approximately 0.2 g of each milled sample was mixed with 20 g of compost (60% moisture content, Garden Basics Topsoil, Swiss Farm Products, Las Vegas, NV) in a 250 mL reaction chamber. Sample chambers were connected to an automated and fully computerized closed-circuit Micro-Oxymax Respirometer System (Columbus Instruments, Columbus, OH) equipped with an expansion interface and a condenser. Experiments were carried out at room temperature (23 ± 0.2°C) over a period of 42 days. The respirometer was programmed to measure CO2 evolution from every 5 h. Each sample was run in triplicate and averaged (~2% variability). Two control samples, consisting of compost alone, provided a measurement of the background CO2 evolution (Imam & Gordon, Citation2002). Native starch (corn, potato, tapioca, and chayotextle) were subjected to the same biodegradation process.
3. Statistical analysis
A completely randomized design was used with a minimum of fifteen replicates for each type of starch and storage condition. An analysis of variance (ANOVA) was used to determine whether treatment effects were significant. All statistical analyses were performed using statistical software (Sigma-Stat version 11.0) (Fox, Shotton, & Ulrich, Citation1995). Mean comparisons were performed using Tukey’s multiple test (α = 0.05).
4. Results and discussion
4.1. Density
The SBF densities ranged from 0.17 to 0.19 g/cm3 (). Different starch sources did not affect the final densities of the foam samples (Tukey’s test, p ≤ 0.05). Densities determined in this study were higher than that reported for expanded polystyrene (0.06 g/cm3) (Glenn, Orts, & Nobes, Citation2001). The same authors reported density values from 0.12 to 0.22 g/cm3 in baked foam samples with starch content, similar to those reported in this study. Density values are affected by the contents of water and starch in the baked foam samples (Glenn et al., Citation2001). Shogren et al. (Citation1998) and Lawton, Shogren, and Tiefenbacher (Citation2004) reported that density values are influenced by the amylose content and the starch batter solids level. For the present study, the densities were comparable for all of the starch samples tested, which would suggest that the moisture content was similar among the different foam samples.
Table 1. Physical and mechanical properties of SBF made with four different sources of starch*.
Tabla 1. Propiedades físicas y mecánicas de películas tipo espuma elaboradas a partir de 4 fuentes diferentes de almidón*.
4.2. Mechanical properties
The effects of the starch source and its impact on mechanical properties are shown in . The chayotextle panel foam showed a significant reduction (α = 0.005) in tensile strength (Ts) relative to values observed for panels from tapioca, potato, and corn. The highest elastic modulus (Em) was observed in samples made of potato starch (47.98 MPa), and the lowest was observed in samples made of chayotextle starch (40.3 MPa). Glenn et al. (Citation2001) studied the effect of wheat, corn, and potato starches on Ts of baked foam panels and reported values of 70, 40, and 45 MPa, respectively. The authors mentioned that mechanical strength is related to the internal microstructure and moisture content of the foam matrix. Micrographs of the internal microstructure of SBF revealed an irregular cell shape and size. The largest cells were localized within the center region of the foam matrix while the smallest cells were localized near the top or bottom surfaces of the sample (). The smaller, dense cells at the upper and lower surfaces likely increased Ts and Em values.
Figure 1. Scanning electron micrographs of cross-section of SBFs made from corn starch (A), potato starch (B), tapioca starch (C), and chayotextle starch (D).
Figura 1. Micrografías electrónicas de barrido de la sección transversal de MTE elaboradas de almidón de maíz (A), papa (B), Tapioca (C), y chayotextle (D).
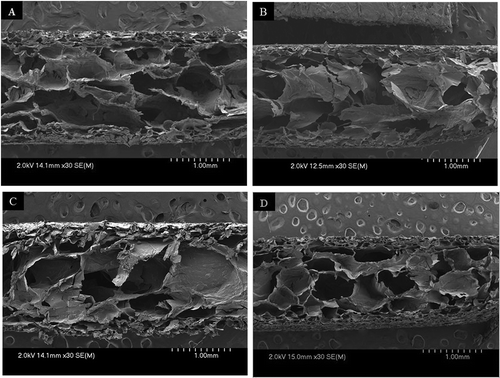
The elongation Eb properties of the four SBF samples used in this study were not significantly different (α = 0.005). Statistical analyses revealed that the source of starch does not generally influence the mechanical properties.
The effects of the RH at 0 and 75% on SBF mechanical properties were similar for all starch samples tested (). The Ts and Em values were reduced by approximately 3% and 5%, respectively, when compared with the 0% RH treatment. A similar trend was presented by Terrazas-Hernández et al. (Citation2015) for cast films made from chayotextle starch mixed with cellulose and cellulose nanoparticles stored at 33, 70, and 90% RH. Cova, Sandoval, Laredo, and Muller (Citation2009) attributed the influence of RH to a plasticizing effect of the water, where a reduction in the attractive forces between polymer chains occurs within the film matrix and reduces Ts and Em. SEM cross-sections revealed a distinct difference in the internal structures of chayotextle and tapioca foam panels stored at 0 and at 75% RH (). An increase in the cell size was observed for both types of foam panels maintained at 75% RH ( A–D). The same trend was observed in cross-sections of foam samples made with potato and corn starches (figures not shown). Absorption of water by the foam panels can lead to swelling, and an expansion in cell size can weaken the internal structure (plasticizer effect) and decrease Ts and Em ().
Table 2. Effect of relative humidity relative (HR) on mechanical properties in SBFs made with four different sources of starch+Δ.
Tabla 2. Efecto de la humedad relativa (HR) sobre las propiedades mecánicas de las películas tipo espuma elaboradas con cuatro diferentes fuentes de almidón+Δ.
Figure 2. Scanning electron micrographs of cross-sections of SBFs conditioned at 75% relative humidity: (A) chayotextle starch (0% RH); (B) chayotextle starch (75% RH); (C) tapioca starch (0% RH); and (D) tapioca starch (75% RH).
Figura 2. Micrografías electrónicas de barrido de la sección transversal de MTE acondicionadas a 75 % de humedad relativa: (A) almidón de chayotextle (0% HR): (B) almidón de chayotextle (75% HR); (C) almidón de tapioca (0% HR); (D) almidón de tapioca (75% HR).
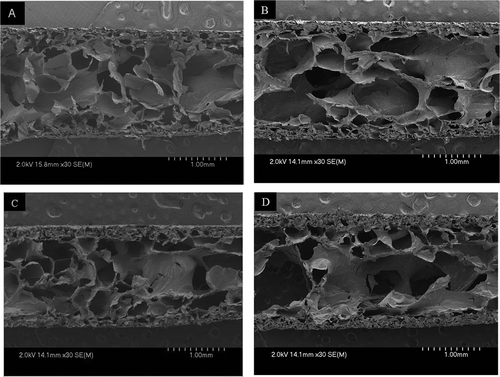
In general, the Eb values at 75% RH increased relative to the 0% RH treatment. A similar trend was reported by Chang, Cheah, and Seow (Citation2000) and Cova et al. (Citation2009) observing films made using tapioca and yucca starches. The increase in Eb at higher RH was most likely because of water acting as a plasticizer, increasing the volume of the free space, weakening the bonding forces and polymer support matrix, reducing Ts and Em values, and increasing the elongation (Cova et al., Citation2009). Glenn and Orts (Citation2001) also found that Ts and Em decreased when the water content of the foam increased. A higher concentration of water in the foam matrix is likely to promote increased mobility of the starch molecules and restrict the reorganization of the polymer chains. A higher relative humidity causes the composite material to soften with an increase in density and in elongation at break (Vargas-Torres et al., Citation2012).
In general, the sources of starch do not influence the mechanical properties of the foams (). However, the RH test showed that moisture has a significant effect on their mechanical properties (α = 0.05).
Storage temperatures of 4 and 65°C had a significant effect on the mechanical properties of SBFs. The Ts values for all SBFs decreased when the foam panels were stored at 65°C. The internal microstructure for corn and potato foams at 4 and 65°C revealed subtle differences, as shown in (A–D). Similar results were observed in cross-sections of chayotextle and tapioca foam samples (Figures not shown). The internal microstructure of samples stored at 65°C showed greater cracking than samples stored at 4°C. These micro-fractures could weaken the foam structure and decrease the force required to break the foam panels. For SBFs stored at 65°C, Ts and Eb values decreased, while Em increased. Vargas-Torres et al. (Citation2012) and Terrazas-Hernandez et al. (Citation2015) reported a similar effect in starch films where high temperatures produced a loss of plasticizer, which reduced polymer mobility and increased the interactions among polymer chains. It has been suggested that under such conditions starch molecules can undergo reorganization, causing the polymer matrix to harden (Chang et al., Citation2000; Ramkumar, Bhattacharya, & Vaidya, Citation1997). In general, Em showed an inverse pattern compared with that of Ts, where a slight increase in the values accompanied the temperature increase. Water loss at higher temperatures reduces the plasticizing effect and increases the stiffness and Em values of the samples. Statistical analyses showed that the starch source did not influence the mechanical properties of the foams, but temperature had a statistically significant effect (α = 0.05).
Figure 3. Scanning electron micrographs of cross-sections of SBFs conditioned at 4°C or 65°C. (A) Potato starch (4°C); (B) potato starch (65°C); (C) corn starch (4°C); and (D) corn starch (65°C).
Figura 3. Micrografías electrónicas de barrido de la sección transversal de MTE acondicionadas a 4°C o 65°C. (A) almidón de papa (4°C): (B) almidón de papa (65°C); (C) almidón de maíz (4°C); (D) almidón de maíz (65°C).
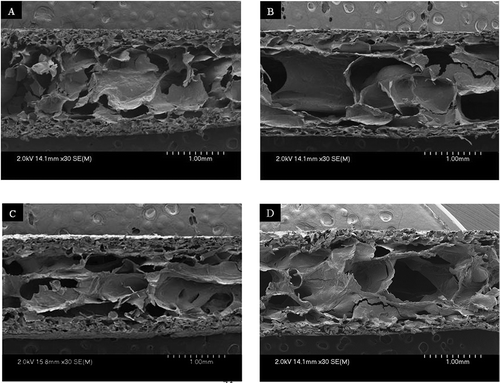
4.3. Microstructural properties
Scanning electron micrographs of corn, potato, tapioca, and chayotextle starch foams are shown in (control). The effects of temperature and relative humidity are shown in and . Cross-sections in show that cell size and shape varied. This variability could be due to the particular characteristics imparted by each type of starch. Hoseney, Zeleznak, and Yost (Citation1986) and Tang, Watanabe, and Mitsunaga (Citation2002) reported that the morphology, chemical composition, and molecular structure of the foam matrix will determine suitable applications for different foam products. Shogren et al. (Citation1998) reported that for foam samples made with the baking process, the surface is denser because of the direct contact between the starch dough and the hot mold, which resulted in rapid gelation and drying that limited foam expansion. Furthermore, such foams exhibited a number of cavities or holes on its surface, which were probably caused by bubbles of steam that contract and break during the drying step. They also reported, that the temperature of drying, starch type and water content play a crucial role in forming the internal structure of baked foam panels. The cross-section of chayotextle SBF ( D) showed a more homogeneous structure than that observed in the other foam panels. Salgado, Schmidt, Molina Ortiz, Mauri, and Laurindo (Citation2008) reported that the internal structure in foam panels is related to the density of the material. An additional contribution may originate with moisture from the raw material (Glenn & Orts, Citation2001) as reported by Hoseney et al. (Citation1986) and Tang et al. (Citation2002).
All foam panels stored at 75% RH showed an increase in the cell size compared with the corresponding 0% RH samples ( A–D). This phenomenon may be due to water absorption by the foam inducing an expansion of the cells (Glenn & Orts, Citation2001) or particular characteristics imparted by each type of starch reported by Hoseney et al. (Citation1986) and Tang et al. (Citation2002). This was similar to observations of foams stored at an increased temperature, and corresponded to a reduction in Ts and Eb values (). Vargas-Torres, Zamudio‐Flores, Salgado‐Delgado, and Bello‐Pérez (Citation2007) reported that 80°C was sufficient to cause depolymerization in extruded films made with plantain flour and poly(vinyl alcohol), accompanied a reduction in certain mechanical properties. A similar pattern was observed in this research. In this regard, the 65°C storage condition may be a sufficient temperature to begin the breakdown of the polymer chains in the foam panels.
Table 3. Effect of the temperature (T°C) on mechanical properties of SBF made of four different sources of starch+Δ.
Tabla 3. Efecto de la temperatura (T°C) sobre las propiedades mecánicas de las películas tipo espuma elaboradas a partir de cuatro fuentes de almidón+Δ.
4.4. Biodegradability tests
The biodegradation test was performed in starch alone and in SBF. The effects of the starch source and the addition of cellulose on the degradation rate of the SBFs were evaluated ( A–D). The test was conducted over 985 h (42 days). Degradation of the corn starch sample was nearly 50% in 7 days; but tapioca, chayotextle, and potato starch samples showed degradation values of 19%, 4%, and 0.5%, respectively, for the same time period. The biodegradation of starch is related to the amount of amorphous regions. These regions are associated with water uptake, which leaves the starch more susceptible to degradation by microorganisms (Fiedorowicz & Para, Citation2006; Myllärinen, Partanen, Seppälä, & Forssell, Citation2002; Wang & Wang, Citation2003). Rodríguez-Hernández, Tecante, and Doublier (Citation2001) reported that tuber starches show a low biodegradation rate because of a high amount of crystallinity (type B). Starch granule size or amylopectin content may affect the rate of biodegradation (Tester & Karkalas, Citation2002; Van Soest & Vliegenthart, Citation1997). Rao and Tattiyakul (Citation1999) reported that the granule size in tapioca starch is between 0.1 and 34 µm. The granule size of chayotextle and potato is 10–25 µm and 10–50 µm, respectively (Hernandez-Uribe et al., Citation2011). The results of this study agree with results reported by Van Soest and Vliegenthart (Citation1997), where the granule size plays an important role in the rate of biodegradation.
Figure 4. (A–D). Effect of the source of the starch on the degradation % of native starches or starch-based foams.
Figura 4. (A–D). Efecto de la fuente de almidón en la porciento de degradación de los almidones nativos o en el material tipo espuma.
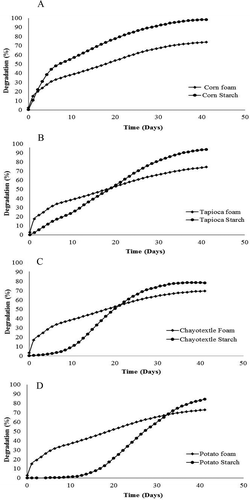
The biodegradation of foam panels reached 70% in 45 days for all SBFs. The percent degradation of SBFs was less than that for the native starches. This behavior may be due to wood fiber incorporated in the SBF. The cellulose present in wood fiber is highly crystalline (Hanna & Xu, Citation2009), which decreased the rate of biodegradation in the SBF samples (Rodríguez-Hernández et al., Citation2001; Terrazas-Hernández et al., Citation2015; Tsoumis, Citation1991). Some research studies mention that the ability of cellulolytic microorganism to degrade cellulose varies greatly with the physico-chemical characteristic of the substrate, such as the degree of crystallinity and polymerization of cellulose, of which the crystallinity degree of cellulose is the most important structural parameters (Amano et al. Citation2001; Beltrame, Carnitti, & Focher, Citation1984; Fan, Lee, & Beardmore, Citation1980). The SBF made with corn starch (less crystalline) showed a degradation of ~50% at day 10 of storage. Meanwhile, the SBFs made with tapioca, potato and chayotextle starches (more crystalline) showed values of degradation of ~40%. The endemic characteristics of starch (size, source, type of crystallinity) in blends with crystalline fiber affect the degradation rate in composites.
5. Conclusion
The type of starch did not have a significant influence over any of the measured mechanical properties. However, storage conditions (temperature and RH) did affect the mechanical properties and internal structure of the SBFs. Biodegradation rate was dependent upon the composition of SBF. In particular, the addition of cellulose fiber to SBF reduced the percent biodegradation. The chayotextle starch can be used in composite materials, the results shown in this study provide information that the common starches can be substituted without affecting the material properties. Besides, these studies revealed the impact of the storage conditions, which could be used to predict the behavior of SBFs in the packaging of dry and wet foods, thereby reducing the use of petroleum-based materials.
Disclosure statement
No potential conflict of interest was reported by the authors.
Additional information
Funding
References
- Aila-Suárez, S., Palma-Rodríguez, H. M., Rodríguez-Hernández, A. I., Hernández-Uribe, J. P., Bello-Pérez, L. A., & Vargas-Torres, A. (2013). Characterization of films made with chayote tuber and potato starches blending with cellulose nanoparticles. Carbohydrate Polymers, 98, 102–107. doi:10.1016/j.carbpol.2013.05.022
- Amano, Y., Nozaki, K., Araki, T., Shibasaki, H., Kuga, S., & Kanda, T. (2001). Reactivities of cellulases from fungi towards ribbon-type bacterial cellulose and band-shaped bacterial cellulose. Cellulose, 8, 267–274. doi:10.1023/A:1015167304364
- American Society for Testing Materials. (1993a). ASTM D 638 M–93. Standard test method for tensile properties of plastic (Metric). In Annual book of ASTM standards. Philadelphia, PA: Author.
- American Society for Testing Materials. (1993b). ASTM D638M-91a. Standard test method for tensile properties of plastics. In Annual book of ASTM standards. Philadelphia, PA: Author.
- Beltrame, P. L., Carnitti, P., & Focher, B. (1984). Enzymatic hydrolysis of cellulosic materials: A kinetic study. Biotechnology and Bioengineering, 31, 160–167.
- Chang, Y. P., Cheah, P. B., & Seow, C. C. (2000). Plasticizing—antiplasticizing effects of water on physical properties of tapioca starch films in the glassy state. Journal of Food Science, 65, 445–451. doi:10.1111/jfds.2000.65.issue-3
- Cinelli, P., Chiellini, E., Lawton, J. W., & Imam, S. H. (2006). Foamed articles based on potato starch, corn fibers and poly(vinyl alcohol). Polymer Degradation and Stability, 91, 1147–1155. doi:10.1016/j.polymdegradstab.2005.07.001
- Cotonieto-Morales, M. B., Palma-Rodríguez, H. M., Chavarría-Hernández, N., Hernández- Uribe, J. P., Zamudio-Flores, P. B., Bello-Pérez, L. A., & Vargas-Torres, A. (2015). Partial characterization of chayotextle starch composites with added polyvinyl alcohol. Starch/Stärke, 66, 1–10.
- Cova, A., Sandoval, J., Laredo, E., & Muller, A. J. (2009). Efecto plastificante y antiplastificante del agua en sistemas a base de almidón. Suplemento de la revista Latinoamericana de Metalurgia y Materiales, S2(1), 45–46.
- Doane, W. M. (1992). USDA research on starch-based biodegradable plastics. Starch – Stärke, 44, 293–295. doi:10.1002/star.19920440805
- Fan, L. T., Lee, Y.-H., & Beardmore, D. H. (1980). Mechanism of the enzymatic hydrolysis of cellulose: Effects of major structural features of cellulose on enzymatic hydrolysis. Biotechnology and Bioengineering, 22, 177–199. doi:10.1002/(ISSN)1097-0290
- Fiedorowicz, M., & Para, A. (2006). Structural and molecular properties of dialdehyde starch. Carbohydrate Polymers, 63, 360–366. doi:10.1016/j.carbpol.2005.08.054
- Flores, E. (1989). El chayote, Sechium edule swartz (Cucurbitaceae). Revista de Biología Tropical, 37, 1–54.
- Flores-Gorosquera, E., García-Suárez, F. J., Flores-Huicochea, E., Nuñez-Santiago, M. C., González-Soto, R. A., & Bello-Pérez, L. A. (2004). Rendimiento del proceso de extracción de almidón de frutos de plátano (Musa paradisiaca). Estudio en Plantapiloto. Acta Científica Venezolana, 55, 86–90.
- Fox, E., Shotton, K., & Ulrich, C. (1995). Sigma-stat user manual. San Rafael, CA: Jandel Scientific.
- Glenn, G., & Orts, W. (2001). Properties of starch- based foam formed by compression/explosion processing. Industrial Crops and Products, 13, 135–143. doi:10.1016/S0926-6690(00)00060-1
- Glenn, G., Orts, W. J., & Nobes, G. A. R. (2001). Starch, fiber and CaCO3 effects on the physical properties of foams made by a baking process. Industrial Crops and Products, 14, 201–212. doi:10.1016/S0926-6690(01)00085-1
- Glenn, G. M., & Irving, D. W. (1995). Starch-based microcellular foams. Cereal Chemistry, 72, 155–161.
- Hanna, M. A., & Xu, Y. (2009). Starch–Fiber Composites. In L. Yu (Ed.), Biodegradable Polymer Blends and Composites from Renewable Resources (pp. 340–363). Lincoln, USA: John Wiley & Sons, University of Nebraska.
- Harper, J. M., & Tribelhorn, R. E. (1992). Expansion of native cereal starch extrudates. In J. L. Kokini, C. Ho, & M. V. Karwe (Eds.), Food extrusion science and technology (pp. 653–667). New York, NY: Marcel Dekker.
- Hernandez-Uribe, J. P., Agama-Acevedo, E., Gonzalez-Soto, R. A., Bello-Pérez, L. A., & Vargas-Torres, A. (2011). Isolation and characterization of Mexican chayote tuber (Sechiumedule Sw.) starch. Starch/Stärke, 63, 32–41. doi:10.1002/star.v63.1
- Hoseney, R. C., Zeleznak, K. J., & Yost, D. A. (1986). A note on the gelatinization of starch. Starch/Stärke, 38, 407–409. doi:10.1002/(ISSN)1521-379X
- Imam, H., & Gordon, S. H. G. (2002). Biodegradation of coproducts from industrially processed corn in a compost environment. Journal of Polymers and the Environment, 10, 147–154. doi:10.1023/A:1021144104458
- Jiménez-Hernández, J., Salazar-Montoya, J. A., & Ramos-Ramírez, E. G. (2007). Physical, chemical and microscopic characterization of a new starch from chayote (Sechium edule) tuber and its comparison with potato and maize starches. Carbohydrate Polymers, 68, 679–686. doi:10.1016/j.carbpol.2006.07.035
- Kaisangsri, N., Kerdchoechuen, O., & Laohakunjit, N. (2012). Biodegradable foam tray from cassava starch blended with natural fiber and chitosan. Industrial Crops and Products, 37, 542–546. doi:10.1016/j.indcrop.2011.07.034
- Lawton, J. W., Shogren, R. L., & Tiefenbacher, K. F. (1999). Effect of batter solids and starch type on the structure of baked starch foams. Cereal Chemistry, 76, 682–687. doi:10.1094/CCHEM.1999.76.5.682
- Lawton, J. W., Shogren, R. L., & Tiefenbacher, K. F. (2004). Aspen fiber addition improves the mechanical properties of baked cornstarch foams. Industrial Crops and Products, 19, 41–48. doi:10.1016/S0926-6690(03)00079-7
- Lee, S.-Y., & Hanna, M. A. (2008). Preparation and characterization of tapioca starch-poly(lactic acid)-Cloisite NA+ nanocomposite foams. Journal of Applied Polymer Science, 110, 2337–2344. doi:10.1002/app.v110:4
- Myllärinen, P., Partanen, R., Seppälä, J., & Forssell, P. (2002). Effect of glycerol on behaviour of amylose and amylopectin films. Carbohydrate Polymers, 50, 355–361. doi:10.1016/S0144-8617(02)00042-5
- Ramkumar, D. H. S., Bhattacharya, M., & Vaidya, U. R. (1997). Properties of injection moulded starch/synthetic polymer blends-II. Evaluation of Mechanical Properties. European Polymer, 33, 729–742.
- Rao, M. A., & Tattiyakul, J. (1999). Granule size and rheological behavior of heated tapioca starch dispersions. Carbohydrate Polymers, 38, 123–132. doi:10.1016/S0144-8617(98)00112-X
- Rodríguez-Hernández, A. I., Tecante, A., & Doublier, J. L. (2001). Viscoelastic behavior of waxy maize starch-gellan mixtures under small amplitude oscillatory shear. In J. Welty-Chanes, G. V. Barbosa-Cánovas, & J. M. Aguilera (Eds.), Proceedings of the eighth international congress on engineering and food (Vol. 1, pp. pp. 509–513). Lancaster: Technomic Publishing.
- Salgado, P. R., Schmidt, V. C., Molina Ortiz, S. E., Mauri, A. N., & Laurindo, J. B. (2008). Biodegradable foams based on cassava starch, sunflower proteins and cellulose fibers obtained by a baking process. Journal of Food Engineering, 85, 435–443. doi:10.1016/j.jfoodeng.2007.08.005
- Shey, J., Imam, H., Glenn, G., & Orts, W. J. (2006). Properties of baked starch foam with natural rubber latex. Industrial Crops and Products, 24, 34–40. doi:10.1016/j.indcrop.2005.12.001
- Shogren, R. L., Lawton, J. W., Doane, W. M., & Tiefenbacher, K. F. (1998). Structure and morphology of baked starch foams. Polymer, 39, 6649–6655. doi:10.1016/S0032-3861(97)10303-2
- Shogren, R. L., Lawton, J. W., & Tiefenbacher, K. F. (2002). Baked starch foams: Starch modifications and additives improve process parameters, structure and properties. Industrial Crops and Products, 16, 69–79. doi:10.1016/S0926-6690(02)00010-9
- Šimkovic, I. (2013). Unexplored possibilities of all-polysaccharide composites. Carbohydrate Polymers, 95, 697–715. doi:10.1016/j.carbpol.2013.03.040
- Stevens, E. S., Klamcznski, A., & Glenn, G. (2010). Starch-lignin foams. Express Polymer Letters, 4, 311–320. doi:10.3144/expresspolymlett.2010.39
- Tang, H., Watanabe, K., & Mitsunaga, T. (2002). Characterization of storage starches from quinoa, barley and adzuki seeds. Carbohydrate Polymers, 49, 13–22. doi:10.1016/S0144-8617(01)00292-2
- Terrazas-Hernández, A. J., Berrios, J. De J., Glenn, G., Syed, H. I., Delilah, W., Bello-Pérez, L. A., & Vargas-Torres, A. (2015). Properties of cast made of chayote (Sechiumedule Sw.) tuber starch reinforced with cellulose nanocrystals. Journal of Polymer Environment, 23, 30–37. doi:10.1007/s10924-014-0652-0
- Tester, R. F., & Karkalas, J. (2002). Starch. In A. Steinbüchel, (Series Ed.) E. J. Vandamme, S. De Baets, & A. Steinbüchel (vol. Eds), Biopolymers, vol. 6. Polysaccharides.ll. Polysaccharides from eukaryotes (381–438). Weinheim: Wiley-VCH.
- Tsoumis, G. (1991). Science and technology of wood structure, properties, utilization (pp. 340–380). New York, NY: Van Nostrand Reinhold.
- US Congress, Office of Technology Assessment. (1993). Biopolymers (pp. 19–50). Washington, DC: OTA-BP-E-102, US Government Printing Office.
- Van Soest, J. J., & Vliegenthart, J. F. (1997). Crystallinity in starch plastics: Consequences for material properties. Trends in Biotechnology, 15, 208–213. doi:10.1016/S0167-7799(97)01021-4
- Vargas-Torres, A., Berrios, J. De J., Chiou, B., Wood, D., Bello-Pérez, L. A., Glenn, G., & Imam, H. (2012). Extruded/ injection-molded composites containing unripe plantain flour, ethylene-vinyl alcohol, and glycerol: Evaluation of mechanical property, storage conditions, biodegradability, and color. Journal of Applied Polymer Science, 124, 2632–2639. doi:10.1002/app.35271
- Vargas-Torres, A., Zamudio‐Flores, P. B., Salgado‐Delgado, R., & Bello‐Pérez, L. A. (2007). Morphological, thermal, and mechanical studies of film elaborated with the blend low‐density polyethylene and chemical‐modified banana starch. Journal of Applied Polymer Science, 106, 3994–3999. doi:10.1002/app.v106:6
- Wang, Y. J., & Wang, L. (2003). Physicochemical properties of common and waxy corn starches oxidized by different levels of sodium hypochlorite. Carbohydrate Polymers, 52, 207–217. doi:10.1016/S0144-8617(02)00304-1
- Xu, Y. X., Hanna, K. M., & Nag, D. (2005). Chitosan-starch composites film: Preparation and characterization. Industrial Crops and Products, 21, 185–192. doi:10.1016/j.indcrop.2004.03.002
- Yang, S.-L., & Walters, T. (1992). Ethnobotany and the economic role of the Cucurbitaceae of China. Economic Botany, 46, 349–367. doi:10.1007/BF02866506