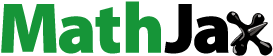
ABSTRACT
Soxhlet and accelerated solvent extraction, using propanol as a solvent, and supercritical CO2 extraction methods presented statistically insignificant differences in the crude oil yield obtained from spent coffee grounds. Supercritical CO2 extraction method was selected to recover the oil. The optimal extraction condition for a high crude oil yield, low acid value, percentage of free fatty acids, peroxide value and thiobarbituric acid reactive substances (TBARS) value, and high iodine and saponification values, was obtained simultaneously at 50°C, 200 bar and 2 h. Under this condition, the experimental data obtained, namely 12.14% oil yield, acid value 3.89 mg KOH/g oil, 2.11% free fatty acids, iodine value 90.70 g I2/g oil, saponification value 212.05 mg KOH/g oil, peroxide value 3.77 mEqO2/kg oil and TBARS value 0.76 mg malondialdehyde/kg oil, were reasonably close to the predicted values. Additionally, the oil from spent coffee grounds is a precious source of palmitic and linoleic acids.
RESUMEN
La extracción Soxhlet, la extracción acelerada de disolventes utilizando propanol como disolvente y los métodos de extracción supercrítica con CO2 mostraron diferencias estadísticamente no significativas en la cantidad de aceite crudo obtenida a partir de granos de café de desecho (ya utilizados). Para recuperar el aceite se seleccionó el método de extracción supercrítica con CO2. La condición de extracción óptima para un alto rendimiento de aceite crudo, con bajos índices de acidez, de porcentaje de ácidos grasos libres, de peróxido, de sustancias reactivas al ácido tiobarbitúrico (TBARS), y altos valores de yodo y saponificación, se obtuvo cuando simultáneamente se establecieron las siguientes condiciones: 50ºC, 200 bar y 2 h. Bajo estas condiciones, los datos experimentales obtenidos estuvieron razonablemente cerca de los valores predichos: rendimiento del aceite de 12.14%, índice de acidez de 3.89 mg KOH/g de aceite, porcentaje de ácidos grasos libres de 2.11%, índice de yodo de 90.70 g I2/g de aceite, valor de saponificación de 212.05 mg de KOH/g de aceite, valor de peróxido de 3.77 mEqO2/kg de aceite y valor de TBARS de 0.76 mg de malondialdehído/kg de aceite. Además, el aceite obtenido de granos de café usados es una valiosa fuente de ácidos palmíticos y linoleicos.
1. Introduction
Coffee is one of the most widely traded commodities and is the most popular beverage in the world. According to the International Coffee Organisation (ICO), approximately 9 million tons of coffee per year is produced from green coffee beans (ICO, Citation2018; Loyao, Villasica, Dela Peña, & Go, Citation2018). Moreover, world coffee exports rose from 9.89 million bags in February 2017 to 9.93 million bags in February 2018 (ICO, Citation2018). Most coffee is produced from Coffea arabica, as Arabica coffee has a high quality due to its relatively smoother, milder, and more flavourful taste enhanced during roasting process of Arabica beans (Bertrand, Guyot, Anthony, & Lashermes, Citation2003).
During coffee processing, roasting, and selling for human consumption, large amounts of solid residues called as spent coffee grounds are produced approximately 6 million tons per year by the global instant coffee industry (Tokimoto, Kawasaki, Nakamura, Akutagawa, & Tanada, Citation2005). Spent coffee grounds are generally discarded as organic fertilizer, soil amendment or biomass fuel, etc. Moreover, spent coffee grounds contain large amounts of natural antioxidant compounds, lipid, protein, carbohydrate, and other valuable components (Campos-Vega, Loarca-Piña, Vergara-Castañeda, & Oomah, Citation2015). Spent coffee grounds contain approximately 10–15% by weight of oil, depending on the coffee species (Jenkins, Stageman, Fortune, & Chuck, Citation2014), and the main fatty acid constituents are palmitic, linoleic, oleic, stearic and arachidic (Oliveira, Franca, Mendonça, & Barros-Júnior, Citation2006; Oliveira, Franca,; Campos-Vega et al., Citation2015; Cornelio-Santiago, Gonçalves, de Oliveira, & de Oliveira, Citation2017; Romano et al., Citation2014). Additionally, spent coffee grounds contain phenolic compounds including chlorogenic acid, caffeoylquinic acid, dicaffeoylquinic acid, feruloylquinic acid, and p-coumaroylquinic acid (Clifford, Johnston, Knight, & Kuhnert, Citation2003; Farah & Donangelo, Citation2006; Moeenfard, Rocha, & Alves, Citation2014). In recent years, scientific and medical communities have paid increasing attention to essential oils, polyphenols, and other natural compounds derived from plants and various biological sources, because of their antioxidant activity and promising influences on human health; for example, anti-tumour and anti-allergic activities, protective action against cancer, cardiovascular disease, and chronic degenerative diseases, including cataracts, macular degeneration, diabetes, and neurodegeneration diseases (Le, Vu, Nguyen, Tran, & Le, Citation2017; Raba et al., Citation2015; Ramalakshmi, Rao, Takano-Ishikawa, & Goto, Citation2009; Rice-Evans, Miller, & Paganga, Citation1997; Scalbert, Johnson, & Saltmarsh, Citation2005). Moreover, phenolic compounds have been used for several applications in the food, food supplement, cosmetic, and also pharmaceutical industries. Extraction of oil, phenolic compounds and other antioxidant compounds from spent coffee grounds is considered as an interesting alternative procedure to obtain these compounds from the low-cost solid residue while adding value to spent coffee grounds (Raba et al., Citation2015).
Extraction is an important separation process used to recover oil or antioxidant phenolic compounds from agricultural wastes, food industry residues and natural materials. Numerous extraction techniques have been utilized for recovery of essential oils and antioxidant phenolic compounds from valuable natural sources; for example, solid–liquid extraction with organic solvents, ultrasound- or microwave-assisted extraction, supercritical fluids extraction and high-pressure extraction processes (Cortazar et al., Citation2005; Markom, Hasan, Daud, Singh, & Jahim, Citation2007; Martins, Aguilar, de la Garza-Rodriguez, Mussatto, & Teixeira, Citation2010). Among these techniques, supercritical CO2 has been widely used as an excellent extraction solvent for the extraction of oil from agro-industrial residues and plant materials (Araújo & Sandi, Citation2007; Karmee et al., Citation2014). Moreover, extraction using supercritical CO2 is a green and environmentally friendly technology, with notable applications in the food, food supplement, pharmaceutical, and cosmetic industries, due to several advantages over conventional extraction techniques (Sahena et al., Citation2009). Besides the high-quality extracts obtained by supercritical fluid extraction (Brunner, Citation2005), the unique properties of CO2 make it the preferred solvent of choice for many substances when compressed into its liquid or supercritical state. CO2 is inert, non-hazardous, non-explosive, non-flammable, inexpensive, naturally abundant, recyclable, easily removed from extracted materials and allows extraction at a comparatively lower temperature and relatively low pressure, in addition to, being non-polar and Generally-Recognised-as-Safe (GRAS) solvent for use in food products (Rai, Mohanty, & Bhargava, Citation2015; Sahena et al., Citation2009; Wang, Wang, Wang, Xiao, & Liu, Citation2016). Since CO2 as a nonpolar lipophilic compound, CO2 is a weak solvent used to extract bioactive compounds with high polarity, for instance, phenolic compounds (Berna, Cháfer, Montón, & Subirats, Citation2001). Moreover, the addition of a polar co-solvent, such as ethanol or methanol, to the extraction system has been proposed to increase the solubility of polar bioactive compounds, resulting in an improved yield of the desired compounds (Gil-Chavez et al., Citation2013; Khaw, Parat, Shaw, & Falconer, Citation2017).
Several researcher groups have investigated the application of supercritical CO2 extraction to spent coffee ground by-products for polyphenols extraction (Ahangari & Sargolzaei, Citation2013; Andrade et al., Citation2012; de Azevedo et al., Citation2008). However, to date, there are no literature reports on the optimal process variables for the supercritical CO2 extraction of oil from spent coffee grounds and its physicochemical properties, using a Box–Behnken experimental design. This study aims to extract oil from spent coffee grounds, using supercritical CO2 extraction technology. All the obtained crude oil extracts are analyzed for oil yield and their fatty acid composition and physicochemical properties. Additionally, the response surface methodology (RSM) is employed to identify the optimal operating conditions of the supercritical CO2 extraction.
2. Materials and methods
2.1. Chemicals and reagents
Malondialdehyde bis(dimethyl acetal) (MDA), trichloroacetic acid (TCA), and potassium hydroxide were obtained from Merck, Darmstadt, Germany. Thiobarbituric acid (TBA), 1,1,3,3-tetraethoxypropane, and HPLC grade acetonitrile were purchased from Sigma–Aldrich (St. Louis, MO, USA). Phenolphthalein, 95% w/w ethanol, 37% w/w HCl and propanol were supplied by RCI Labscan Ltd. (Bangkok, Thailand), and Lanna Industrial Gases Co., Ltd. (Chiang Mai, Thailand) provided the CO2 (mass fraction purity of approximately 0.999 in the liquid phase). All the chemicals and reagents employed in the experiments were analytical grade.
2.2. Preparation of spent coffee grounds sample
The spent coffee grounds of Arabica blends were collected from the Wawee coffee shops, located in Chiang Mai Province, Thailand. The spent coffee grounds were oven-dried at 105°C for 24 h, until constant water content. The dried spent coffee grounds sample had 0.39 wt% moisture content, 0.09 water activity, and 14.11 wt% crude fat. The dehydrated spent coffee grounds were pulverized into a fine powder (50 mesh), using a Moulinex Super Junior S coffee grinder (Ecully, France), for 30 s. The powder sample was collected and kept in vacuum-sealed bags at 4°C until required.
2.3. Spent coffee grounds extraction methods
2.3.1. Soxhlet extraction
Propanol was employed for Soxhlet extraction of oil from spent coffee grounds. The spent coffee grounds (10 g) were packed in a thimble, and the oil was extracted with 180 mL of solvent, which was recycled over the sample for 6 h extraction at the boiling temperature of the selected solvent. After the extraction process, the mixture of solvent and oil was separated at 40°C using a vacuum rotary evaporator. The oil sample was further dried at 105°C for 3 h, to remove residual solvent. The oil yield extracted from the spent coffee grounds samples was calculated on the basis of the dry weight of the sample.
2.3.2. Accelerated solvent extraction (ASE)
Accelerated solvent extraction was carried out using a Dionex ASE 350 extractor (Thermo Fisher Scientific, Inc., Sunnyvale, CA, USA) as shown in ). Dried and powdered samples (0.5 g) were placed between two layers of diatomaceous earth, a dispersing inert agent that was used to reduce the solvent volume needed for the extraction process, in a 22 mL stainless-steel cell. The bottom of each extraction cell was fitted with a stainless-steel frit and a cellulose filter (Thermo Fisher Scientific, Inc., Sunnyvale, CA, USA) for preventing the suspended particle from getting in the collection vial. The extraction cell was placed in the cell tray, and the sample was extracted using an extraction temperature of 120°C at constant extraction pressure of 1500 psi for 5 min. To avoid the oxidation of the analytes during the operating conditions, nitrogen was used to previously degas the extraction solvent. The extraction cycle proceeded automatically, as follows: the extraction cell with the sample was filled with the selected extraction solvent (propanol), pressurized at a pressure of 1500 psi and then heated at the selected temperature for 5 min, followed by 5 min of a static extraction period. Then, the extraction cell was rinsed with a fresh extraction solvent and purged by nitrogen gas for 90 s at a pressure of approximately 150 psi. The extracts obtained were collected into 60 mL glass vials and the solvent removed by using a vacuum rotary evaporator at 40°C until the sample weight was constant. The oil yield was then calculated. The experiment was carried out in two batches including three replications for each sample.
Figure 1. Schematic representation of (a) accelerated solvent extraction (ASE) system and (b) Supercritical CO2 extraction system (showing front and back views).
Figura 1. Representación esquemática del (a) sistema de extracción acelerada de solventes (ASE) y (b) sistema de extracción supercrítica de CO2 (vistas frontal y posterior).
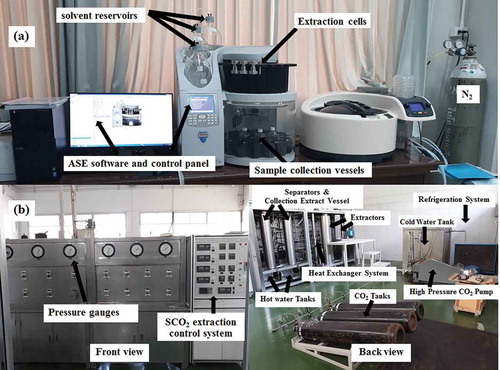
2.3.3. Supercritical CO2 extraction of spent coffee grounds samples
Supercritical CO2 extraction procedure was performed using a supercritical CO2 extractor (Guangzhou Heavensent Industrial Co., Ltd., China), equipped with a 5-liter volume extractor vessel and two gravimetric separators in series as shown in ). For each extraction, 1.5 kg of spent coffee grounds sample was placed inside the extractor vessel. The porous stainless-steel mesh filters were placed at both ends of the extractor vessel. Supercritical CO2 extractions of oil from spent coffee grounds samples were carried out at three different extraction temperatures (40, 50 and 60°C), pressures (175, 200, and 250 bar) and times (1, 3, and 5 h). The CO2 in liquid cylinders was delivered to the CO2 storage tank for recirculation and reuse in the extraction process. Then, the CO2 pre-cooled to 4°C using a cooling heat exchanger was pressurized by a high-pressure diaphragm pump and further delivered into the extractor vessel. The extractions were carried out by passing supercritical CO2 through a packed bed of spent coffee grounds sample. The temperatures of the extractor and the two separators were automatically controlled by using the recirculation system of thermostated water from two individually regulated water baths. During the extraction process, the CO2 flow rate could be regulated between 110 and 170 L/h. The liquid extract was precipitated in the separators and finally CO2 was released into the atmosphere. After completing each extraction, the obtained oil extract was collected in a sampling bottle, sealed and stored at 4°C until analysis. All experiments were performed in triplicate.
2.4. Experimental design
Optimal supercritical CO2 extraction conditions were investigated using RSM, which was carried out using the Minitab Version 16.0 software (Minitab, Inc., State College, PA, USA). A Box–Behnken design was used to determine the effects of three independent variables (extraction temperature, extraction pressure and extraction time) on the dependent variables (oil yield, acid value, FFAs, iodine value (IV), saponification value (SV), peroxide value (PV) and thiobarbituric acid reactive substances (TBARs)). Three levels were applied to each of the three factors, as introduced in .
Table 1. The coded and uncoded levels of independent variables used in the response surface methodology design.
Tabla 1. Niveles codificados y no codificados de variables independientes utilizadas en el diseño de la metodología de superficie de respuesta.
The experimental data obtained from the Box–Behnken design was analyzed by fitting to an empirical second-degree polynomial equation using regression analysis. The estimated second-degree polynomial regression equation is given by Equation (1):
where Y is the dependent variables; Xi and Xj are independent variables (i and j ranging from 1 to k); k is the number of independent variables; βo is the constant coefficient; and βi, βii and βij represent the regression coefficients of linear, quadratic and interaction term, respectively. The coefficient of regression (R2), the lack of fit and the Fisher test value (F-value) obtained from the analysis of variance (ANOVA) were used to measure the goodness of fit of the model equation. Statistical significance of the estimated regression equation and model variables was accepted at a probability level of 5% (p ≤ 0.05). The estimated second-degree polynomial regression equation (Equation (1)) was used to generate three-dimensional (3D) response surface plots by Minitab software. The Box–Behnken design has 15 experimental runs with three runs at the center point shown in .
Table 2. Box–Behnken design.
Tabla 2. Diseño de Box-Behnken.
2.5. Crude oil yield
The percentage of crude oil yield obtained from each experiment was determined by the following equation below:
where M1 is the weight of crude oil obtained after each extraction process and M2 is the dry weight of the spent coffee ground sample before extraction.
2.6. Physicochemical characteristics of oils
2.6.1. Oil quality parameters
Acid value, the percentage of free fatty acids (% FFAs), iodine value, peroxide value, and saponification value of the oil samples were measured by the official standard method (AOAC, Citation2000).
2.6.2. TBARS
The extent of lipid oxidation was measured as TBARS, as detailed by Buege and Aust (Citation1978). Thiobarbituric acid (TBA) was reacted with MDA, thus leading to a colored compound. Briefly, the oil sample (0.2 mL) was mixed thoroughly with a volume of 1 mL of TBA reagent solution which contains 0.375% TBA, 15% trichloroacetic acid (TCA), and 0.25 N HCl. The mixture was heated in a water bath (95 ± 5°C) for 20 min before cooling with running cold tap water. The mixture was further centrifuged at 3600 rpm for 20 min. After removing the obtained precipitate by the centrifugation, the supernatant layer was taken and its absorbance at 532 nm was measured against a corresponding blank solution. The MDA stock solution was transferred to a 25 mL volumetric flask and completely mixed with 0.1 M HCl. Final MDA concentrations for the calibration curve were 0, 2, 4, 6, 8, and 10 µg/mL, which generated the calibration equation y= 0.2686x–0.0449 (R2 = 0.9938). TBARS values were determined from a standard curve of MDA, which was prepared by the acidification of 1,1,3,3-tetraethoxypropane. The TBARS results were expressed in milligrams of malondialdehyde per kilogram of oil sample (mg MDA/kg oil).
2.6.3. Fatty acid compositions
The fatty acid compositions of the spent coffee ground oils were analyzed by a Shimadzu GC-14B gas chromatograph (Shimadzu Co., Kyoto, Japan), according to the official AOAC method (AOAC, Citation2005). An Rt-2560 biscyanopropyl polysiloxane capillary column (100 m length × 0.25 mm i.d., 0.20 μm thick film; Restek, Bellefonte, PA, USA) was employed. The initial oven temperature was 100°C and held at 100°C for 5 min before it was increased at 3°C/min, from 100°C to 230°C and held for 15 min. The injector temperature and the detector temperature were set at 225°C and 250°C, respectively. Additionally, nitrogen was used as the carrier gas at a constant flow rate of 1 mL/min. The fatty acids in the oil samples were identified using reference standards (fatty acid methyl esters) obtained from Sigma–Aldrich. The Shimadzu GC Solution software was used to detect and integrate the peaks. The fatty acid compositions of spent coffee ground oil samples obtained from supercritical carbon dioxide extraction method were compared with those of the commercial oils including rice bran oil (King brand, Thai Edible Oil Company Limited, Thailand), soybean oil (A-Ngoon brand, Thai Vegetable Oil Public Company Limited, Thailand); and sesame oil (Butterfly brand, Chaiseri Company Limited, Thailand).
2.7. Statistical analysis
The experimental data points were statistically analyzed and compared using one-way ANOVA, by Minitab Version 16.0 software. Duncan’s new multiple range test was used to further evaluate differences between means that were statistically significant at p ≤ 0.05.
3. Results and discussion
3.1. Comparison of soxhlet extraction, accelerated solvent extraction and supercritical CO2 extraction techniques
shows the amount of crude oil yielded from the spent coffee grounds was affected by the solvent used in the extraction technique. Comparing all three extraction techniques, accelerated solvent extraction using propanol (14.02%) had a higher average oil yield compared to the Soxhlet extraction with propanol (13.75%) and supercritical CO2 extraction (12.11%) but there were no statistically significant differences (p> 0.05). Soxhlet extraction has several disadvantages such as a long extraction time, a usage of a large volume of solvent, losses, and degradation of volatile organic compounds and operation at high extraction temperature leading to undesirable off-flavor compounds (Grigonis, Venskutonis, Sivik, Sandahl, & Eskilsson, Citation2005). Meanwhile, accelerated solvent extraction and supercritical CO2 extraction methods have several advantages over conventional Soxhlet extraction method; for instance, low operating temperature leading to reduce thermal degradation of volatile compounds, a shorter extraction time, and an increasing selectivity in oil and bioactive compound extraction. Supercritical CO2 extraction and accelerated solvent extraction seem to be an expensive extraction process at laboratory scale; however, an accurate economic evaluation requires additional extraction experiments for establishment of the large industrial scale. Given the great interest in utilizing CO2 as the solvent for oil extraction, this research selected supercritical CO2 extraction for oil extraction from spent coffee grounds. Additionally, our laboratory has a pilot-scale supercritical CO2 extractor with a large reactor volume (5 l). Thus, it has the potential to produce up to several times more crude oil content per experimental batch than an accelerated solvent extractor.
Table 3. Crude oil yield from Soxhlet extraction, accelerated solvent extraction, and supercritical CO2 extraction.
Tabla 3. Rendimiento del aceite crudo a partir de la extracción Soxhlet, la extracción acelerada de solventes y la extracción supercrítica con CO2.
3.2. Supercritical CO2 extraction
describes the experimental conditions under which the supercritical CO2 extraction experiments were conducted, as well as the acid value, %FFAs, IV, SV, PV, TBARS value, and oil yield (expressed as a percentage based on g oil/100 g dry spent coffee) obtained for each experiment. Supercritical CO2 extracted a maximum crude oil yield of 13.65% after 3 h of extraction at 50°C and 225 bar compared to 4.67% at 40°C and 175 bar for 2 h. The acid value, %FFAs, IV, SV, PV, and TBARS value of the crude oil extracts were in the ranges of 4.01–6.39 mg KOH/g oil, 2.02–3.21% (oleic), 80.55–91.80 g I2/g oil, 185.26–212.55 mg KOH/g oil, 3.13–12.52 mEqO2/kg oil and 0.69–2.54 mg MDA/kg oil. The acid value, FFA content, and SV was close to the result obtained from Al-Hamamre, Foerster, Hartmann, Kröger, and Kaltschmitt (Citation2012) who investigated the properties of the oil extracted from spent coffee grounds by Soxhlet extraction, using various non-polar and polar solvents. The maximum oil yield (approximately 15.3%) using hexane as the solvent was achieved at an extraction temperature of 69°C for 30 min. Under those conditions, the resultant oil had an acid value, FFA content and SV of 7.30 mg KOH/g oil sample, 3.65% and 173.9 mg KOH/g oil sample, respectively.
Table 4. Crude oil yield, acid value, percentage free fatty acids (%FFAs), iodine value (IV), saponification value (SV), peroxide value (PV), and thiobarbituric acid reactive substances (TBARS) of extracted oil from spent coffee grounds using supercritical CO2 extraction.
Tabla 4. Rendimiento del aceite crudo, índice de acidez, porcentaje de ácidos grasos libres (% FFA), índice de yodo (IV), valor de saponificación (SV), valor de peróxido (PV) y sustancias reactivas al ácido tiobarbitúrico (TBARS) del aceite extraído de café molido usado utilizando la extracción supercrítica con CO2.
3.2.1. Model fitting for % crude oil yield and physicochemical properties of oil samples
The percentage crude oil yield and physicochemical properties of oil from spent coffee grounds (acid value, %FFAs, IV, SV, PV, and TBARS value) obtained from all the experiments according to RSM design and ANOVA for the Box–Behnken design matrix is presented in . The coefficients of the quadratic models and the regression coefficients () were estimated by using multivariable linear regression analysis. In addition, a suitable response surface model, the significance of the model equation and the model terms were selected using ANOVA. The ANOVA results showed that all the proposed models had low p-values (≤0.05), high F- and adjusted-R2 (adj.-R2) values and a low lack-of-fit. The adj.-R2 as a corrected value for R2 was obtained after the elimination of the unnecessary model terms.
Table 5. Analysis of variance table of the quadratic response surface model for the responses of percentage crude oil yield and physicochemical properties of the oil.
Tabla 5. Tabla de análisis de varianza del modelo de superficie de respuesta cuadrática para las respuestas del porcentaje de rendimiento de aceite crudo y propiedades fisicoquímicas del aceite.
After the application of the response surface regression process (), the predicted polynomial equations obtained for the percentage crude oil yield and physicochemical properties of the oil, such as acid value, %FFAs, IV, SV, PV, and TBARS, as a function of the independent process variables (extraction temperature, extraction pressure and extraction time), their correspondent adj.-R2 coefficients and p-values were determined ().
Table 6. Quadratic response surface model of acid value, percentage free fatty acids (%FFAs), iodine value (IV), saponification value (SV), peroxide value (PV), and thiobarbituric acid reactive substances (TBARS) of crude oil obtained from spent coffee grounds by supercritical CO2 extraction.
Tabla 6. Modelo de superficie de respuesta cuadrática del índice de acidez, porcentaje de ácidos grasos libres (% FFA), índice de yodo (IV), valor de saponificación (SV), valor de peróxido (PV) y sustancias reactivas al ácido tiobarbitúrico (TBARS) del aceite crudo obtenido por extracción supercrítica con CO2 de café molido usado.
From , the adj.-R2 values of the quadratic models were 0.9313, 0.9581, 0.9763, 0.9707, 0.9137, 0.9478 and 0.9637 for percentage crude oil yield, acid value, %FFAs, IV, SV, PV, and TBARS value, respectively. The adj.-R2 of the quadratic models of all values was higher than 0.90, and also the p-values of the quadratic models were less than 0.05 (p ≤ 0.05), indicating the quadratic models were statistically significant. It can be interpreted that the quadratic models were adequate to represent the real relationship between the factors and responses. Additionally, the insignificant lack-of-fit indicated in , shows the generated quadratic models adequately predicted the responses.
The response surface (3D) plots demonstrate the influence of the various extraction parameters (extraction temperature, extraction pressure and extraction time) on the percentage crude oil yield ()), acid value ()), %FFAs ()), IV ()), SV ()), PV ()) and TBARS value ()) obtained, using supercritical CO2 extraction.
Figure 2. Response surface (3D) plots showing the effect of different extraction temperature, pressure and time on (a) crude oil yield, (b) acid value (AV), (c) percentage of free fatty acids (%FFAs), (d) iodine value (IV), (e) saponification value (SV), (f) peroxide value (PV), and (g) thiobarbituric acid reactive substances (TBARS) value of crude oil obtained using supercritical CO2 extraction.
Figura 2. Gráficos de superficie de respuesta (3D) que muestran el efecto de diferentes temperaturas de extracción, presión y tiempo en: (a) el rendimiento de aceite crudo, (b) el índice de acidez (AV), (c) el porcentaje de ácidos grasos libres (% FFA), (d) el valor de yodo (IV), (e) el valor de saponificación (SV), (f) el valor de peróxido (PV) y (g) el valor de sustancias reactivas al ácido tiobarbitúrico (TBARS) del aceite crudo obtenido mediante la extracción supercrítica con CO2.
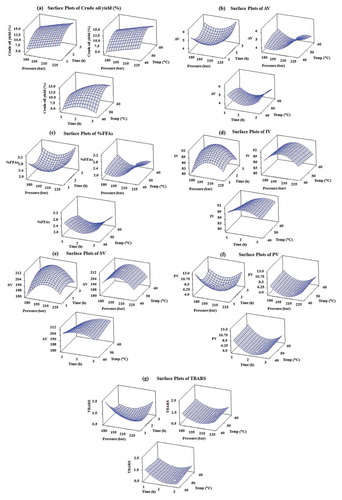
3.2.2. Effects of the process variables on the percentage of crude oil yield
presents the 3D response surface plots of estimated quadratic models for percentage crude oil yield. The data in and reveal that the positive linear effect of extraction pressure, time and temperature, and significant negative quadratic effect of time and temperature, increased the percentage of crude oil yield. The percentage of crude oil yield can be improved with higher extraction pressure, time, and temperature, as shown in . It could be seen in ) that an increase of temperature and time beyond the experimental range of 50°C and 2 h, respectively, caused a reduction in the percentage crude oil yield. As the extraction pressure has a significant effect compared to time and temperature for oil extraction from spent coffee grounds, extending the extraction time (>2 h) and raising the extraction temperature (>50°C) might be effective for the extraction process but would be detrimental to the crude oil yield, as it would undergo oxidation and polymerization (Zahir, Saeed, Hameed, & Yousuf, Citation2017). If the given extraction temperature increased above approximately 50°C while the extraction pressure was increasing, the crude oil yield increased with increasing pressure levels. This trend could be explained that the elevation of the extraction pressure increased the supercritical CO2 density leading to enhance oil solubility in supercritical CO2.
As presented in ), it was noticed that the crude oil yield increased with the increase of the extraction temperature in the initial extraction stage. This observation occurred because the change of solute vapor pressure is more effective than that of solvent density. However, the observed trend reversal occurred when the extraction temperature reached about 50°C under the range of extraction pressure investigated in this research. Similar results were also reported in supercritical CO2 extraction of other lipids including myristic acid and tripalmitin (Wang et al., Citation2008). However, the elevated temperature decreases the CO2 density, resulting in the reduction of the dissolving ability for the solvent. Additionally, the temperature elevation increases the vapor pressure of the solutes and, therefore, the solubility of the solute decreases with elevating extraction temperature at constant extraction pressure (Thana et al., Citation2008). In summary, the RSM analysis demonstrates that the maximum crude yield of spent coffee ground oil was predicted to be 15.08%, by applying 225 bar extraction pressure, 53.74°C extraction temperature and 2.62 h extraction time ()).
3.2.3. Effects of the process variables on acid value, %FFAs, IV, SV, PV, and TBARS value
Acid value, %FFAs, IV, SV, PV, and TBARS value are the most important physicochemical properties affecting the fatty acid composition of the extracted crude oil from spent coffee grounds. Therefore, the effect of the independent variables (extraction temperature, pressure and time) on the dependent variables including acid value, %FFAs, IV, SV, PV, and TBARS value could be visualized by creating surface response plots of the equation models. The effects of extraction pressure, extraction temperature and extraction time on the acid value, %FFAs, IV, SV, PV and TBARS value are illustrated in , respectively.
In ), the acid value increases when (i) increasing the pressure and time; (ii) lowering the temperature and raising the pressure (>220 bar) and (iii) reducing the pressure (<220 bar) but increasing the temperature. From ), it is, therefore, evident that at an extraction time of approximately 2.5 h, the acid value increased with the decrease of extraction temperature. The %FFAs displayed a similar behavior to the acid value, as seen in ). Low values of acid and %FFAs indicate that the degree of edibility and the storage life of the oil are high. The crude oil obtained from spent coffee grounds had a low acid value (4.01–6.39 mg KOH/g oil) and low content of FFAs (2.02–3.21%). It is also possible to further refine the crude oil samples for the production of edible oil or utilize the oil samples for the production of biodiesel.
In general, at a constant temperature, increasing the pressure increases the density of supercritical CO2, resulting in the increase of the solubility of solutes such as lipids, fatty acids, triglycerides, etc.. As the density of supercritical CO2 increases, the distance between molecules of solvent and solute decreases. Therefore, the interaction between oil and CO2 increases to improve the solubility of oil in CO2 resulting in the increase of mass transfer rate. Thus, a higher extraction pressure of supercritical CO2 would produce a stronger lipid extraction power. Free fatty acids can be recovered by using supercritical CO2 extraction method. At higher extraction pressure and time, the extraction of total fatty acids in supercritical CO2 is increased. It is indicated that a higher pressure at a given temperature resulted in an increase of the density of the supercritical CO2 and its solvating power leading to an increased solubility of the free fatty acids (Santana, Jesus, Larrayoz, & Filho, Citation2012). The extracted oil samples obtained showed higher peroxide and acid values, indicating that the extracted oils might be less stable leading to higher TBARS value.
In the current study, the RSM results confirm that the minimal acid value (3.58 mg KOH/g oil) and %FFAs response (1.81%) () and ) were achieved by applying 201.26 bar extraction pressure, 60°C extraction temperature and 1.85 h extraction time. The greater the acid value and the FFA content the lower the quality of the oil.
) shows the 3D plots of the response surface. It is demonstrated the effect of extraction pressure and extraction time, extraction pressure, and extraction temperature on the IV. It is noticed that the IV of crude oil significantly increased with increasing pressure from 175 bar to approximately 200 bar at fixed temperature and time. The extraction pressure and time exhibited an important effect on the IV while the extraction temperature was insignificant compared with pressure and time. Additionally, the IV increased when the extraction time increased from 1 h to 2 h. However, the IV decreased with the increase of pressure and time above 200 bar and 2 h, respectively.
The content of unsaturated and saturated fatty acids of extracted oil increased when the extraction pressure and time increased. A higher content of unsaturated fatty acids indicates a higher the iodine value because the iodine value (IV) is a measure of unsaturation fatty acid content (Igwenyi & Akubugwo, Citation2010; Olubi, Felix-Minnaar, & Jideani, Citation2019). However, at higher pressure (>200 bar) for longer extraction time (>2 h) at a certain extraction temperature, the iodine value slightly decreased. Although the yield of extracted oil at higher extraction pressure and longer extraction time increased the observed iodine value decreased. This suggested that the extracted oil might contain a higher fraction of saturated fatty acids than that of unsaturated fatty acids leading to lower iodine value (IV).
) shows the 3D plots of the response surface for the SV, respectively. ) demonstrates the effect of extraction pressure and extraction time, extraction pressure and extraction temperature on the SV at the given temperature of 50°C and time of 2 h, respectively. It was observed that the SV of crude oil significantly increased with increasing pressure from 175 bar to approximately 200 bar at fixed temperature and time. The extraction pressure and time exhibited an important effect on the SV while the extraction temperature was insignificant compared with pressure and time. Moreover, the SV increased when the extraction time increased from 1 h to 2 h. Nevertheless, a higher pressure (>200 bar) and longer time (>2 h) led to a decrease of the SV.
The SV is an indication of the molecular weights of triglycerides in oil, and a significantly high SV in the oil (approximately 260.22 mg KOH/g) indicates that the oil is a suitable raw material for soap manufacturing. A high SV indicates a high proportion of low molecular weight fatty acids because SV is inversely proportional to the mean molecular weight or the fatty acid chain length (Sadoudi & Ali Ahmed, Citation2017). Hence, the shorter the mean chain length (C4–C12) the greater the saponification value (Dimberu, Atinafu, & Bedemo, Citation2011). Based on the SV value obtained in this study (), the oils contain a high content of short-chain fatty acids (<C12) and low molecular weight fatty acids, at levels close to the permissible amount of 190–209 mg KOH/g for palm oil, set by the Codex Alimentarius (Citation1999) in CODEX-STAN 210–1999. The low SV value suggests that the average molecular weight of the fatty acids is low or that the quantities of ester bonds are few, possibly implying that there are no interactions among the fat molecules (Denniston, Topping, & Caret, Citation2004). However, the SV of the crude oil extracted at maximum levels of extraction pressure of 197.73 bar, extraction temperature of 53.33°C and extraction time of 2.43 h, was the least (211.87 mg KOH/g oil) ()).
The 3D plots for the PV of crude oil as a function of pressure, temperature, and time are shown in ). As it can be observed in ), the PV of crude oil significantly decreased with increasing pressure from 175 bar to approximately 200 bar at fixed temperature and time. Additionally, the PV decreased when the extraction time was increased from 1 h to 2 h. However, a higher pressure (>200 bar) and longer time (>2 h) led to an increase in the PV. From ), the minimum PV (~3.21 mgEqO2/kg oil) was achieved at the pressure 200.25 bar, time 2.19 h, and extraction temperature 46.26°C.
The development of secondary lipid oxidation products is commonly determined by the TBARS method. The TBARS values for the extracted oil samples were below 1, which is the limit of acceptance of a product as rancid. ) shows 3D plots of the response surface for the TBARS value, respectively. The TBARS values decreased (less than 1) with an increasing pressure and extraction time from 175 to 200 bar and 1 to 2.5 h, respectively. When the extraction pressure and time were higher than 200 bar and 2.5 h, respectively, the TBARS value increased. As the extraction pressure and the extraction time increased, the total fatty acids increased (Santana et al., Citation2012), indicating that the extracted oils obtained might be less stable causing higher TBARS value. At higher extraction temperature, a longer extraction pressure and extraction time, not exceeding 200 bar and 2.5 h, respectively, resulted in a correspondingly lower TBARS value. Overall, the RSM results show that the minimum TBARS value (0.62 mg MDA/kg oil) was achieved by 197.73 bar extraction pressure, 40°C extraction temperature and 2.47 h extraction time.
3.2.4. Optimization and verification of the models
Process optimization investigates the factor level combinations that simultaneously satisfy the requirements imposed on the responses and factors, using the desirability function in the RSM. It shows simultaneously the responses of percentage crude oil yield, acid value, %FFAs, IV, SV, PV and TBARS value being affected by the combination of the experimental factors, i.e., extraction temperature (40°C, 50°C, and 60°C), extraction pressure (175, 200, and 225 bar) and extraction time (1, 3 and 5 h). In the present study, the desirability was set to achieve the optimal condition that maximises the crude oil yield and obtain favorable acid value, %free fatty acid value, saponification value, and TBARS value ().
Figure 3. Optimum region overlaying the curves of the responses crude oil yield and its acid value (AV), percentage free fatty acids (%FFAs), iodine value (IV), saponification value (SV), peroxide value (PV) and thiobarbituric acid reactive substances (TBARS) value as a function of the extraction temperature, extraction time, and extraction pressure used during the extraction process.
Figura 3. Superposición de la región óptima a las curvas de respuesta del rendimiento del aceite crudo y su valor ácido (AV), porcentaje de ácidos grasos libres (% FFA), valor de yodo (IV), valor de saponificación (SV), valor de peróxido (PV) y valor de sustancias reactivas al ácido tiobarbitúrico (TBARS) en función de la temperatura, el tiempo y la presión utilizadas durante el proceso de extracción.
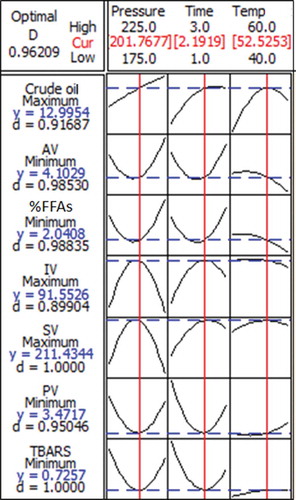
From , according to canonical analysis, the stationary points for percentage crude oil yield, acid value, %FFAs, IV, SV, PV, and TBARS value was the maximal point. The overlapped contour plot () was applied for the optimization of the crude oil yield. The optimal supercritical CO2 extraction condition was obtained at 52.52°C, 201.77 bar for 2.19 h, predicting the maximum crude oil yield of 12.99%, a low acid value of 4.10 mg KOH/g oil, low 2.04% FFAs, a high IV of 91.55 g I2/g oil, a high SV of 211.43 mg KOH/g oil, a low of PV 3.47 mgEqO2/kg oil, and a low TBARS value of 0.73 under this condition. Furthermore, the reliability of the experimental method was tested; the extraction experiment was performed by adopting the program of the optical analytical model. The extraction condition was adjusted by using the extraction temperature of 50°C, extraction pressure of 200 bar and extraction time of 2 h. The experimental results reported a crude oil yield of 12.14%, an acid value of 3.89 mg KOH/g oil, 2.11% FFAs, IV of 90.70 g I2/g oil, SV of 212.05 mg KOH/g oil, PV of 3.77 mgEqO2/kg oil, and TBARS of 0.76 mg MDA/kg oil.
The validation of the response surface models was determined by the comparison between the experimental results and the predicted results by the models. It was confirmed that the prediction model was adequate and reliable. The application of the RSM to crude oil extraction from spent coffee grounds using supercritical CO2 extraction was successful.
A high SV indicates a high proportion of low molecular weight and short-chain length of fatty acids. The SV obtained in this work (185.26–211.15 mg KOH/g oil, shown in ) was close to that of crude palm oil (190–209 mg KOH/g oil; Codex Alimentarius, Citation1999). It shows that the extracted oils may not only have a high potential for soap and cosmetics production but also could be a useful resource of essential fatty acids required in the human body (Akanni, Adekunle, & Oluyemi, Citation2005; Japir et al., Citation2017; Koushki, Nahidi, & Cheraghali, Citation2015).
3.3. Fatty acid composition of extracted oils
The crude oil sample obtained from the optimal supercritical CO2 extraction condition (50°C, 200 bar for 2 h) was determined its fatty acid composition by gas chromatography-mass spectroscopy. Additionally, its fatty acid composition was compared to the selected oil extracts obtained from two extraction conditions of 40°C, 175 bar for 2 h and 60°C, 200 bar for 3 h which gave the lower yield of crude oil (~4.67% and 11.75%, respectively, as shown in ). gives the fatty acid composition of the selected oil extracts obtained by supercritical CO2 extraction. The two main fatty acids presented in spent coffee ground oils were linoleic acid (C18:2) (approximately 34.99–42.45%) and palmitic acid (C16:0) (approximately 32.98–41.83%), followed by oleic acid (C18:1) (7.50–8.30%), stearic acid (C18:0) (7.08–7.78%), and arachidic acid (C20:0) (3.25–3.44%). As shown in , there was no noticeable variation in the composition of the main fatty acids of the oil recovered by the different supercritical CO2 extraction conditions. The fatty acid composition of all extracted oil samples was almost similar. These results are similar to those of Ixtaina et al. (Citation2011), who investigated different conditions of supercritical CO2 extraction of chia seed oils and reported that the fatty acid composition of oil samples was not affected by the extraction conditions.
Table 7. Fatty acid composition of crude oil from spent coffee grounds using supercritical CO2 extraction.
Tabla 7. Composición de ácidos grasos del aceite crudo obtenido de granos de café usados utilizando la extracción supercrítica con CO2.
These results agree with those reported by Somnuk, Eawlex, and Prateepchaikul (Citation2017) who demonstrated that the oil extracted from spent coffee grounds using hexane contained 43% linoleic acid, 34.44% palmitic acid, 7.74% oleic acid, 2.83% arachidic acid and 1.18% α-linoleic acid. Meanwhile, Efthymiopoulos, Hellier, Ladommatos, Kay, and Mills-Lamptey (Citation2017) reported that the oil obtained from spent coffee grounds by Soxhlet extraction method using hexane consisted of 40.7–43.1% linoleic acid, 32.4–34.6% palmitic acid, 7.5–8.1% stearic acid, 9.3–10.2% oleic acid, and 3.1–4.0% arachidic acid. Le et al. (Citation2017) showed that the oil compositions obtained from maceration and microwave-assisted extraction were similar and composed of approximately 35.39% and 33.68% linoleic acid (C18:2), 28.73% and 31.55% palmitic acid (C16:0), and 25.78% and 25.89% oleic acid (C18:1), respectively.
Additionally, the result shown in illustrated that a highly polyunsaturated oil due to its high linoleic acid content (34.99–42.45%), high saturated fatty acid content, such as palmitic acid (32.98–41.83%), and low stearic acid (7.08–7.78%) and unsaturated oleic acid contents (7.50–7.78%), on average (). The large amount of linoleic acid (C18:2) presented in spent coffee ground oils is beneficial considering this fatty acid cannot be synthesized by the body (Le et al., Citation2017).
As presented in , the fatty acid compositions of the crude oils highlight the potential for recovering oil from the spent coffee grounds. Comparing the crude oil extracted from spent coffee grounds with rice bran oil, soybean oil, and sesame oil (), the extracted oil has a higher palmitic acid content (32.98–41.83%) than rice bran oil (19.20%), soybean oil (10.78%), and sesame oil (8.87%), respectively, but lower oleic acid content. The linoleic acid content (34.99–42.45%) in the oil extracts recovered from the spent coffee ground was less than that found in soybean oil (50.61%) and sesame oil (44.96%) but higher than that found in rice bran oil (31.49%).
The relationship between the polyunsaturated and saturated fatty acid content can be expressed by the ratio of polyunsaturated to saturated fatty acids (or P/S index). This index can be used to determine the nutritional value of oils and fats. The oils and fats are considered to have nutritional value when the value of P/S index is greater than one. Vegetable oils cover a wide P/S range (approximately 0.24–3.80) (Yun & Surh, Citation2012). The P/S values of the crude oil samples recovered in this study were in the range of 0.66–0.91 (). Although high levels of saturated fatty acids help to provide a high stability of the oil, they are nutritionally undesirable (Bauer, Kostik, & Gjorgjeska, Citation2015). The current nutritional recommendation is that the P/S ratio in the human diet should be above 0.45 (Abedi et al., Citation2016; Poskitt, Citation2003). Considering the results, it is possible to extract relatively healthy oil from the spent coffee ground that represents a good source of not only saturated but also polyunsaturated fatty acids.
4. Conclusions
In this work, Soxhlet and accelerated solvent extraction methods for extraction of oil from spent coffee grounds were performed using propanol as the solvent, and the results compared with supercritical CO2 fluid extraction. There was no statistically significant difference in the crude oil yields extracted by the different methods. Supercritical CO2 extraction was selected for further analysis in the extraction of crude oil from spent coffee grounds and three operating parameters (extraction pressure, temperature, and time) were optimized by using RSM to obtain oil with a high yield and suitable physicochemical properties (acid value, %FFAs, IV, SV, PV, and TBARS value). The results showed that the estimated second-order polynomial equation model adequately fitted the experimental data. The linear, quadratic, and interaction terms of the independent variables of extraction pressure, temperature and time had a significant effect on the crude oil yield and its acid value, %FFAs, IV, SV, PV, and TBARS value. Based on a range of the studied independent variables used in the experimental design, the optimal extraction condition for high crude oil yield and favorable acid value, %FFAs, SV and TBARS value was at 50°C, 200 bar and 2 h. Under this optimal condition, the experimental responses obtained a crude oil yield of 12.14%, an acid value of 3.89 mg KOH/g oil, 2.11% FFAs, IV of 90.70 g I2/g oil, SV of 212.05 mg KOH/g oil, PV of 3.77 mgEqO2/kg oil, and TBARS of 0.76 mg MDA/kg oil, which were reasonably matched with the predicted responses. The fatty acid compositions of the crude oils extracted by supercritical CO2 were analyzed by gas chromatography. The fatty acids presented in spent coffee ground oils were linoleic acid (34.99–42.45%), palmitic acid (32.98–41.83%), oleic acid (7.50–8.30%), stearic acid (7.08–7.78%), and arachidic acid (3.25–3.44%). The results of the study demonstrate the potential of supercritical CO2 extraction as an attractive alternative green technology for the extraction of oil from spent coffee grounds as the residual solid remaining after the production of the coffee beverage. Additionally, spent coffee grounds could be a renewable natural resource of natural lipids for edible oil or biofuel production.
Disclosure statement
No potential conflict of interest was reported by the authors.
Additional information
Funding
References
- Abedi, A. S., Hosseini, H., Mohammadi, A., Abdollahi, Z., Hajifaraji, M., & Mousavi Khaneghah, A. (2016). Fatty acid (FA) compositions and trans content of frequently consumed edible oils and fats from Iran’ market. Current Nutrition & Food Science, 12, 56–64.
- Ahangari, B., & Sargolzaei, J. (2013). Extraction of lipids from spent coffee grounds using organic solvents and supercritical carbon dioxide. Journal of Food Processing and Preservation, 37, 1014–1021.
- Akanni, M. S., Adekunle, A. S., & Oluyemi, E. A. (2005). Physicochemical properties of some non-conventional oilseeds. Journal of Food Technology, 3, 177–181.
- Al-Hamamre, Z., Foerster, S., Hartmann, F., Kröger, M., & Kaltschmitt, M. (2012). Oil extracted from spent coffee grounds as a renewable source for fatty acid methyl ester manufacturing. Fuel, 96, 70–76.
- Andrade, K. S., Gonçalvez, R. T., Maraschin, M., Ribeiro-do-Valle, R. M., Martínez, J., & Ferreira, S. R. S. (2012). Supercritical fluid extraction from spent coffee grounds and coffee husks: Antioxidant activity and effect of operational variables on extract composition. Talanta, 88, 544–552.
- AOAC. (2000). Official methods of analysis (17th ed.). Washington DC, USA: Association of Official Analytical Chemists.
- AOAC. (2005). Official methods of analysis (18th ed.). Gaithersburg, MD, USA: Association of Official Analytical Chemists.
- Araújo, J. M. A., & Sandi, D. (2007). Extraction of coffee diterpenes and coffee oil using supercritical carbon dioxide. Food Chemistry, 101, 1087–1094.
- Bauer, B., Kostik, V., & Gjorgjeska, B. (2015). Fatty acid composition of seed oil obtained from different canola varieties. Farmaceutski Glasnik, 71, 1–7.
- Berna, A., Cháfer, A., Montón, J. B., & Subirats, S. (2001). High-pressure solubility data of system ethanol (1) + catechin (2) + CO2 (3). Journal of Supercritical Fluids, 20, 157–162.
- Bertrand, B., Guyot, B., Anthony, F., & Lashermes, P. (2003). Impact of the Coffea canephora gene introgression on beverage quality of C. arabica. Theoretical and Applied Genetics, 107, 387–394.
- Brunner, G. (2005). Supercritical fluids: Technology and application to food processing. Journal of Food Engineering, 67, 21–33.
- Buege, J. A., & Aust, S. D. (1978). Microsomal lipid peroxidation. Methods in Enzymology, 52, 302–310.
- Campos-Vega, R., Loarca-Piña, G., Vergara-Castañeda, H. A., & Oomah, B. D. (2015). Spent coffee grounds: A review on current research and future prospects. Trends in Food Science & Technology, 45, 24–36.
- Clifford, M. N., Johnston, K. L., Knight, S., & Kuhnert, N. (2003). Hierarchical scheme for LC-MSn identification of chlorogenic acids. Journal of Agricultural and Food Chemistry, 51, 2900–2911.
- Codex Alimentarius. (1999). Codex standard for named vegetable oils (CODEX-STAN 210-1999). Food and Agricultural Organisation of the United Nations and the World Health Organisation.
- Cornelio-Santiago, H. P., Gonçalves, C. B., de Oliveira, N. A., & de Oliveira, A. L. (2017). Supercritical CO2 extraction of oil from green coffee beans: Solubility, triacylglycerol composition, thermophysical properties and thermodynamic modelling. Journal of Supercritical Fluids, 128, 386–394.
- Cortazar, E., Bartolomé, L., Delgado, A., Etxebarria, N., Fernández, L. A., Usobiaga, A., & Zuloaga, O. (2005). Optimisation of microwave-assisted extraction for the determination of nonylphenols and phthalate esters in sediment samples and comparison with pressurised solvent extraction. Analytica Chimica Acta, 534, 247–254.
- de Azevedo, A. B. A., Kieckbusch, T. G., Tashima, A. K., Mohamed, R. S., Mazzafera, P., & Vieira de Melo, S. A. B. (2008). Supercritical CO2 recovery of caffeine from green coffee oil: New experimental solubility data and modelling. Química Nova, 31, 1319–1323.
- Denniston, K. J., Topping, J. J., & Caret, R. L. (2004). In: General, organic, and biochemistry (4th ed., pp. 432–433). New York, NY, USA: McGraw Hill Companies.
- Dimberu, G., Atinafu, D. G., & Bedemo, B. (2011). Estimation of total free fatty acid and cholesterol content in some commercial edible oils in Ethiopia, Bahir DAR. Journal of Cereals and Oil Seeds, 2, 71–76.
- Efthymiopoulos, I., Hellier, P., Ladommatos, N., Kay, A., & Mills-Lamptey, B. (2017). Effect of solvent extraction parameters on the recovery of oil from spent coffee grounds for biofuel production. Waste and Biomass Valorization. doi:10.1007/s12649-017-0061-4
- Farah, A., & Donangelo, C. M. (2006). Phenolic compounds in coffee. Brazilian Journal of Plant Physiology, 18(1), 23–36.
- Gil-Chavez, G. J., Villa, J. A., Ayala-Zavala, J. F., Heredia, J. B., Sepulveda, D., Yahia, E. M., & Gonzalez-Aguilar, G. A. (2013). Technologies for extraction and production of bioactive compounds to be used as nutraceuticals and food ingredients: An overview. Comprehensive Reviews in Food Science and Food Safety, 12(1), 5–23.
- Grigonis, D., Venskutonis, P. R., Sivik, B., Sandahl, M., & Eskilsson, C. S. (2005). Comparison of different extraction techniques for isolation of antioxidants from sweet grass (Hierochloë odorata). Journal of Supercritical Fluids, 33, 223–233.
- ICO. International Coffee Organisation. 2018. Coffee production by exporting countries and world coffee consumption. Retrieved from http://www.ico.org/
- Igwenyi, I. O., & Akubugwo, E. I. (2010). Analysis of four seeds used as soup thickeners in the south eastern part of Nigeria. In: Proceedings ICCCE 2010–2010 International Conference on Chemistry and Chemical Engineering, Kyoto, Japan, 426–430.
- Ixtania, V. Y., Martínez, M. L., Sportorno, V., Mateo, C. M., Maestri, D. M., Diehl, B. W. K., … Tomas, M. C. (2011). Characterization of chia seed oils by pressing and solvent extraction. Journal of Food Composition and Analysis, 24, 166–174.
- Japir, A. A. W., Salimon, J., Derawi, D., Bahadi, M., Al-Shuja‘a, S., & Yusop, M. R. (2017). Physicochemical characteristics of high free fatty acid crude palm oil. Oilseeds and Fats, Crops and Lipids, 24, D506.
- Jenkins, R. W., Stageman, N. E., Fortune, C. M., & Chuck, C. J. (2014). Effect of the type of bean, processing, and geographical location on the biodiesel produced from waste coffee grounds. Energy & Fuels, 28, 1166–1174.
- Karmee, S. K., Niemeijer, B., Casiraghi, L., Mlambo, B., Lapkin, A., & Greiner, L. (2014). Facile biocatalytic synthesis of a macrocyclic lactone in sub- and supercritical solvents. Biocatalysis and Biotransformation, 32, 125–131.
- Khaw, K. Y., Parat, M. O., Shaw, P. N., & Falconer, J. R. (2017). Solvent supercritical fluid technologies to extract bioactive compounds from natural sources: A review. Molecules, 22, 1186.
- Koushki, M., Nahidi, M., & Cheraghali, F. (2015). Fatemeh Cheraghali. Physico-chemical properties, fatty acid profile and nutrition in palm oil. Journal of Paramedical Sciences, 6, 117–134.
- Le, P. T. K., Vu, Q. T. H., Nguyen, Q. T. V., Tran, K. A., & Le, K. A. (2017). Extraction and evaluation the biological activities of oil from spent coffee grounds. Chemical Engineering Transactions, 56, 1729–1734.
- Loyao, A. S., Villasica, S. L. G., Dela Peña, P. L. L., & Go, A. W. (2018). Extraction of lipids from spent coffee grounds with non-polar renewable solvents as alternative. Industrial Crops and Products, 119, 152–161.
- Markom, M., Hasan, M., Daud, W. R. W., Singh, H., & Jahim, J. M. (2007). Extraction of hydrolysable tannins from Phyllanthus niruri Linn.: Effects of solvents and extraction methods. Separation and Purification Technology, 52, 487–496.
- Martins, S., Aguilar, C. N., de la Garza-Rodriguez, I., Mussatto, S. I., & Teixeira, J. A. (2010). Kinetic study of nordihydroguaiaretic acid recovery from Larrea tridentata by microwave-assisted extraction. Journal of Chemical Technology and Biotechnology, 85, 1142–1147.
- Moeenfard, M., Rocha, L., & Alves, A. (2014). Quantification of caffeoylquinic acids in coffee brews by HPLC-DAD. Journal of Analytical Methods in Chemistry, 2014, 1–10.
- Oliveira, L. S., Franca, A. S., Mendonça, J. C. F., & Barros-Júnior, M. C. (2006). Proximate composition and fatty acids profile of green and roasted defective coffee beans. LWT - Food Science and Technology, 39, 235–239.
- Olubi, O., Felix-Minnaar, J. V., & Jideani, V. A. (2019). Physicochemical and fatty acid profile of egusi oil from supercritical carbon dioxide extraction. Heliyon, 5, e01083.
- Poskitt, E. (2003). Nutrition in childhood. In J. B. Morgan & J. W. T. Dickerson (Eds.), Nutrition in early life: Concepts and practice (pp. 291–325). Chichester, UK: Wiley & Sons.
- Raba, D. N., Poiana, M. A., Borozan, A. B., Stef, M., Radu, F., & Popa, M. V. (2015). Investigation on crude and high-temperature heated coffee oil by ATR-FTIR spectroscopy along with antioxidant and antimicrobial properties. PloS one, 10, e0138080.
- Rai, A., Mohanty, B., & Bhargava, R. (2015). Modeling and response surface analysis of supercritical extraction of watermelon seed oil using carbon dioxide. Separation and Purification Technology, 141, 354–365.
- Ramalakshmi, K., Rao, L. J. M., Takano-Ishikawa, Y., & Goto, M. (2009). Bioactivities of low-grade green coffee and spent coffee in different in vitro model systems. Food Chemistry, 115, 79–85.
- Rice-Evans, C., Miller, N., & Paganga, G. (1997). Antioxidant properties of phenolic compounds. Trends in Plant Science, 2, 152–159.
- Romano, R., Santini, A., Le Grottaglie, L., Manzo, N., Visconti, A., & Ritieni, A. (2014). Identification markers based on fatty acid composition to differentiate between roasted Arabica and Canephora (Robusta) coffee varieties in mixtures. Journal of Food Composition and Analysis, 35, 1–9.
- Sadoudi, R., & Ali Ahmed, D. (2017). Studies on physico-chemical characteristics and fatty acid composition of commercially available Algerian frying edible oils. International Food Research Journal, 24(1), 60–67.
- Sahena, F., Zaidul, I. S. M., Jinap, S., Karim, A. A., Abbas, K. A., Norulaini, N. A. N., & Omar, A. K. M. (2009). Application of supercritical CO2 in lipid extraction—A review. Journal of Food Engineering, 95, 240–253.
- Santana, A., Jesus, S., Larrayoz, M. A., & Filho, R. M. (2012). Supercritical carbon dioxide extraction of algal lipids for the biodiesel production. Procedia Engineering, 42, 1755–1761.
- Scalbert, A., Johnson, I. T., & Saltmarsh, M. (2005). Polyphenols: Antioxidants and beyond. American Journal of Clinical Nutrition, 81, 215S–217S.
- Somnuk, K., Eawlex, P., & Prateepchaikul, G. (2017). Optimization of coffee oil extraction from spent coffee grounds using four solvents and prototype-scale extraction using circulation process. Agriculture and Natural Resources, 51, 181–189.
- Thana, P., Machmudah, S., Goto, M., Sasaki, M., Pavasant, P., & Shotipruk, A. (2008). Response surface methodology to supercritical carbon dioxide extraction of astaxanthin from Haematococcus pluvialis. Bioresource Technology, 99, 3110–3115.
- Tokimoto, T., Kawasaki, N., Nakamura, T., Akutagawa, J., & Tanada, S. (2005). Removal of lead ions in drinking water by coffee grounds as vegetable biomass. Journal of Colloid and Interface Science, 281, 56–61.
- Wang, L., Wang, X., Wang, P., Xiao, Y., & Liu, Q. (2016). Optimization of supercritical carbon dioxide extraction, physicochemical and cytotoxicity properties of Gynostemma pentaphyllum seed oil: A potential source of conjugated linolenic acids. Separation and Purification Technology, 159, 147–156.
- Wang, X., Chen, H., Guo, Y., Su, Y., Wang, H., & Li, J. (2008). Preparation of ibuprofen/lipid composite microparticles by supercritical fluid technique. Frontiers of Chemical Engineering in China, 2, 361–367.
- Yun, J. M., & Surh, J. (2012). Fatty acid composition as a predictor for the oxidation stability of Korean vegetable oils with or without induced oxidative stress. Preventive Nutrition and Food Science, 17, 158–165.
- Zahir, E., Saeed, R., Hameed, M. A., & Yousuf, A. (2017). Study of physicochemical properties of edible oil and evaluation of frying oil quality by Fourier transform-infrared (FT-IR) spectroscopy. Arabian Journal of Chemistry, 10, S3870–S3876.