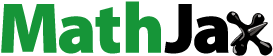
ABSTRACT
The present work was aimed at developing and characterizing biodegradable citrus pectin films incorporated with young apple polyphenols (YAPs). Fourier-transform infrared spectroscopy (FTIR), scanning electron microscopy analysis (SEM) and X-ray diffraction (XRD) analysis were performed to get acquaintance with the structure of the pectin/YAP films. An increase of tensile strength (TS) and elastic modulus (EM) accompanied with a decrease in elongation at break (% EB) was observed with YAP. FTIR spectrometry exhibited the emergence of new absorbance peak at 1520 cm−1, suggesting some interactions between both constituents of films. An increase in thermal stability of films was also observed by the inclusion of YAP into the film. Moreover, SEM analysis revealed that YAPs were homogenously distributed across pectin matrix. The antioxidant potential of the films was amplified by incorporating YAP. The microbiological assessment of the films confirmed the antimicrobial efficiency of newly developed films against three major food-borne pathogens.
RESUMEN
El presente trabajo tuvo como objetivo desarrollar y caracterizar películas biodegradables de pectina de cítricos a las que se incorporaron polifenoles de manzana joven (YAP). Para familiarizarse con la estructura de las películas de pectina/YAP se emplearon espectroscopía infrarroja por Transformadas de Fourier (FTIR), análisis de microscopía electrónica de barrido (SEM) y análisis de difracción de rayos X (XRD). Se observó que la adición de YAP provocó un aumento de la resistencia a la tracción (TS) y del módulo elástico (EM), acompañado de una disminución del alargamiento a la rotura (% EB). La espectrometría FTIR exhibió la aparición de un nuevo pico de absorbancia a 1520 cm−1, lo que sugiere que se produjeron algunas interacciones entre ambos componentes de las películas. Asimismo, la inclusión de YAP en las películas produjo un aumento de la estabilidad térmica de las mismas. Además, el análisis SEM reveló que los YAP se distribuyeron homogéneamente en la matriz de pectina. Al incorporar YAP se amplió el potencial antioxidante de las películas. La evaluación microbiológica de las mismas confirmó la eficacia antimicrobiana de las películas recientemente desarrolladas principalmente contra tres patógenos transmitidos por los alimentos.
Abbreviations: ATR-FTIR: attenuated total reflectance-Fourier transform infrared spectroscopy; DSC: differential scanning calorimetry; EB: elongation at break; EM: elastic modulus; SEM: scanning electron microscopy; SI: swelling index; TS: tensile strength; WVP: water vapor permeability; XRD: X-ray diffraction; YAP: young apple polyphenols
1. Introduction
The modern global market is experiencing a high demand of consumers for fulfilling the criteria of safety and quality. The total world production of plastic has been increased about 20-fold; however, only 10% or less are recycled, leading to excessive accumulation of plastic based materials in the environment (Sutherland et al., Citation2010). Consequently, research approaches associated with the usage of biopolymers as packaging material has been appeared as a substitute of synthetic packaging (Azeredo et al., Citation2009). Bioactive packaging is a smart system that involves the interaction between packaging material and the packaged food; either by migration to the headspace or onto the food-contact surface to maintain freshness of the food product. This bio-based packaging system is supposed to maintain the integrity of food during storage primarily by imparting functional properties, preventing lipid oxidation and acting as a barrier to external influences such as oxygen, light and water vapors (Bao, Xu, & Wang, Citation2009). Nutritionally fortified biodegradable films are mainly influenced by the nature of carriers (film forming matrix) and the type of functional agent incorporated into them. So, a clear understanding of the physical and chemical interactions between polymeric material and functional substance is vital for developing active films (Park & Zhao, Citation2004).
Pectin is a biodegradable polysaccharide and partially crystalline in the structure which makes it excellent for film formation and it has inherently low cost as compared to other packaging materials. Biodegradable films based on pectin presented good gas and lipid barrier properties but their mechanical strength is consider moderate and they exhibited poor water barrier properties (Giosafatto et al., Citation2014; Petersen et al., Citation1999). To overcome this problem their mechanical strength and water barrier properties can be enhanced by integrating some strengthening or crosslinking agents into the film matrix (Cabello, Takara, Marchese, & Ochoa, Citation2015). Pectin also has the potential to carry functional substances, as it is suitable for integration of phytochemical compounds for developing nutritionally fortified edible films (Eca, Machado, Hubinger, & Menegalli, Citation2015).
Apple polyphenols have been found to possess high antimicrobial and antioxidant potential by delaying the onset of lipid oxidation (Alberto, Canavosio, & Manca de Nadra, Citation2006), inhibiting the growth of cancerous cells in human body and also provide protection against viral infections (He et al., Citation2011). Sun, et al. (Citation2017) have also verified the strong antioxidant activities of apple polyphenols on grass carp surimi during cold storage. Fruit thinning is important in order to enhance the yield and quality of apples. In China, about 1.6 million tons of young apples are discarded every year in the process of fruit thinning. These discarded thinned young apples contain approximately 10 times higher content of total phenolic acids as compared to fully ripened apples. So it is necessary to make worthy use of thinned young apples by extracting exhibited bioactive compounds from them instead of discarding in apple grooves. In this context it is needed to seek higher value products from thinned young apples which could be economically valuable for commercial exploitation as well as for extraction of bioactive compounds (Sun, Guo, Fu, Li, & Li, Citation2013).
The incorporation of active substances into pectin-based films is an interesting way of extending films’ functional properties. Previous studies have that antioxidant activity of pectin, can be improved through integration of natural substances, such as clove essential oil (Nisar et al., Citation2018), papaya puree and cinnamaldehyde essential oil (Otoni et al., Citation2014), pomegranate juice (Azeredo et al., Citation2016), Mexican lime essential oil (Sánchez Aldana, Andrade-Ochoa, Aguilar, Contreras-Esquivel, & Nevárez-Moorillón, Citation2015) and cranberry extract (Park & Zhao, Citation2006). In a struggle to develop bio-based, novel edible films to be used as active packaging, the current study was intended at evaluating the influence of young apple polyphenols (YAPs) incorporation on physico-mechanical, antioxidant and antimicrobial properties of pectin films. Characterization involved the understanding of potential changes in transport, structural properties and thermal properties of films when YAPs were incorporated.
2. Material and methods
2.1. Materials
Pectin from citrus fruits (galacturonic acid ≥74%, methoxyl groups ≥6.7%, MW = 30–100 kg mol−1) was purchased from Sigma Aldrich (St. Louis, MO, USA). Anhydrous glycerol, calcium chloride, and magnesium nitrate hexahydrate and all other chemicals were purchased from Jingbo biological. Ltd. Xian, China. Young apples were handpicked after 30 days of blossoming from Liquan province, China.
2.2. Preparation of inoculum
Three different bacterial strains (Escherichia coli, Staphylococcus aureus and Listeria monocytogenes) were cultured in 10 mL of Nutrient broth, Luria-Bertani broth and Trypticase Soy Broth with yeast extract, respectively, and kept in oscillating incubator at 37°C for 24 h. Each bacterial suspension was re-cultured in respective broth medium and again incubated at 37°C for 24 h.
2.3. Preparation of YAP
The YAP were extracted and separated from thinned young apples according to the method reported by Sun et al. (Citation2013). Briefly, 500 g of young apple were grounded by a grinder and extracted with 5000 mL of 60% ethanol aqueous solution at 65°C in an immersion oscillator for 3 h. Then the extracts were concentrated using rotary evaporator at 65°C. The concentrated solution was then centrifuged at 1370× g for 20 min. Ultimately, the clear supernatant was loaded onto a glass column (45 mm × 600 mm) filled with X-5 resin at a flow rate of 1.0 BV/h and then lyophilization was done by using freeze drier (GAMMA 1–16 LSC, UK) working at −55°C and the inner pressure of the chamber was set at 60 Pa.
2.4. Determination of phenolic composition in YAP
The content of total polyphenols in YAP were determined using Dionex®® HPLC system (P680, California, U.S.A.) equipped with Thermo Ultimate 3000 UPLC system (Thermo Electron Co. USA) and Thermo syncronis C18 column (250 × 4.6 mm I.D., 5 µm, USA). The column temperature was maintained at 30°C. Solvent A was consist of acetonitrile and methanol in 30:70 ratio, respectively, and solvent B was consist of 5% methanol solution along with 1% trifluoroacetic acid. Elution of Solvent A and B was conducted as follows: From 0 to 3 min = 100% B solvent at speed of 0.95 mL/min, from 3 to 19 min, 100–60% B at same speed, from 19 to 30 min, 60% B fluent with the flow speed of 1 mL per minute, for next 30.1–30.2 min, solvent B at 60-100% with speed of 1–0.95 mL/min and for last 30.2–40 min, 100% B solvent at speed of 0.95 mL/min. Injection volume of the sample was 4 µL and the wavelength was set at 280 nm. Total 16 standards of the polyphenols were taken i.e. 1. Gallic acid, 2. Protocatechuic acid, 3. Epigallocatechin 4. Catechin, 5. Procyanidin B2, 6. Chlorogenic acid, 7. 4-hydroxybenzoic acid, 8. Epicatechin, 9. Caffeic acid, 10. Epicatechin gallate, 11. Rutin, 12. Hyperin, 13. Ellagic acid, 14. Quercitin 15. Phloridzin, 16. Meletin. The total content of the polyphenols as well as the percentage of individual polyphenols were identified (qualitative analysis) and calculated (quantitative analysis) by comparing against the retention time and peak area of the standards.
2.5. Preparation of the films
Bio-based Pectin films were prepared with the method used by (Otoni et al., Citation2014) with some modifications. Film forming solutions were made by dissolving pectin powder (2.75%) into distilled water. Glycerol, as a plasticizer, was added into the suspension at level of at 30% (w/w of pectin) and thoroughly mixed with magnetic stirring. Subsequently, the solution was warmed up to 70°C and 30 mL of calcium chloride solution (1% w/w of pectin) was added slowly to the film solution at a flow rate of 1 mL/min. The YAP solutions with different concentrations were formed by dissolving YAP into distilled water at concentrations 0% (as control), 0.5%, 1% and 1.5% (v/v) of film forming solution. The resulting film dispersions were completely homogenized using Rotor-stator Homogenizer (Ultra Turrax IKA, T25, Werke, Germany) at 300 rpm for 10 min. After cooling, the film solutions were degassed under vacuum for 20 minutes through ultrasonication. To prepare films, 80 mL of film mixture was casted onto 15 cm ceramic petri plates and dried for 24 h at ambient temperature in circulating air oven. The acquired pectin films were conditioned for 48 h at 50% relative humidity (RH) using saturated Mg(NO3)2.6H2O in an environmental chamber prior to experimental use.
2.6. Physical properties of the films
2.6.1. Film thickness and water content
Film thicknesses were measured by using a digital microcaliper (Mitutoyo Absolute, Tester Sangyo Co. Ltd., Tokyo, Japan) up to the nearest 0.001 mm. Ten measurements were taken on various regimes of each film. The moisture contents of the films (2 cm×2 cm) were determined by measuring the weight loss of films after drying the pieces at 105°C in hot air oven for 24 h up to constant weight.
2.6.2. Water solubility and swelling index
The water solubility and Swelling degree of the film samples were measured according to the method proposed by (Azeredo et al., Citation2016; Zhong, Song, & Li, Citation2011). For solubility, previously dried film squares (M1) were immersed in 50 mL deionized water for 6 h at 25°C under constant agitation (150 rpm). Residual film pieces were dried superficially with filter paper, followed by weighing the wet films pieces (M2).The final weight (M3) of the film pieces was obtained after drying at 105°C in fan oven for 24 h and cooling them up to room temperature in a desiccator containing anhydrous calcium chloride. Film solubility and swelling index were calculated by using the following equations, respectively,
2.6.3. Water vapor permeability (WVP)
The permeability to water vapors was measured gravimetrically by using the method of (Otoni et al., Citation2014) with some alterations. Special flat bottomed aluminum cups (internal diameter: 6 cm, exposed area: 28.27 cm2 and depth: 1.3 cm) were used to measure WVP. The bottom of the rounded test cups were filled with granular anhydrous calcium chloride (0% RH) and sealed with circular film samples (previously equilibrated at 53% RH for 2 days). The film sealed cells were placed in an environmental chamber maintained at 25°C and 100% RH conferred by deionized water. Eight weights of the cups were taken periodically by using an analytical balance after 2 h interval with an accuracy of 0.0001 g. The water vapor permeation ratio (g m−2 s−1) and water vapor permeability (g m−1 s−1 Pa−1) of the films were measured as follows:
WVP was calculated as follows:
where W is the weight of water vapors permeated through the film (g), t is the time of permeation (s), A is effective film area (m2), d is the thickness of the film samples (m) and Δ p is the saturation vapor pressure of water at the assay temperature (pa). All the measurements were done in quadruplicate.
2.6.4. Color and opacity measurement
Film color was determined using an automatic color difference meter (Shanghai Precision & Scientific instrument Co., Ltd., Shanghai, China). The CIE Lab scale was used, L*(luminosity) and chromaticity parameters a* (positive: red – negative: green) and b* (positive: yellow – negative: blue) were measured. A standard white plate CX 2064 was used as a standard for color calibration. Four replications were conducted for each treatment. Total color difference (ΔE) was calculated by
where ΔL, Δa and Δb are the differentials between color parameters of sample and standard used as the film background.
The opacity of the films were determined according to the method of Yong Peng and Li (Citation2014) by measuring the film absorbance at 600 nm using UV spectrophotometer (UV 2100, Unico Instruments Co., Ltd., Shanghai, China). The pectin films were cut into rectangular strips and placed inside of the spectrophotometer cell. An empty cell was used as the reference. The opacity of the films was measured by the following equation:
where O is the opacity of films, Abs is the value of absorbance at 600 nm and x is the thickness of film specimen (mm).
2.7. Mechanical properties of the films
The mechanical properties of the pectin films were determined according to the method defined by Yong Peng and Li (Citation2014) including tensile strength (TS) and percentage of elongation at break (%E) using a Universal Testing Machine (Model 5655, Instron Corporation, Canton, MA, USA) with load cell of 50 kg. The films pieces were cut into rectangular dimensions of 10cm×1cm and mounted between the tensile grips of universal testing machine. Film samples were stretched at cross-head speed of 1 mm/s from initial gap of 50 mm until breakage. TS was calculated by dividing the maximum force required by the cross-sectional area of film sample whereas elongation at break was calculated by dividing the film’s length at the time of breakage by its original length of film strip and multiplying by 100.
2.8. Fourier-transform infrared spectroscopy (FTIR)
The infrared (IR) spectra of the pectin films were determined with Fourier-transform infrared spectrometer (FTIR) (EQUINX55, Brucher, Germany), using Attenuated Total Reflectance (ATR) mode to study the interactions of pectin and YAP in the film matrix. A spectral resolution of 4 cm−1 was employed and 16 scans were acquired for each sample in the spectral range of 4000 to 400 cm−1.
2.9. Differential scanning calorimetry (DSC)
The melting point (Tm) and the change in enthalpy values (ΔH) were examined by differential scanning calorimetry (DSC) using Q1000 DSC system (TA instruments, New Castle, USA). Film samples were preconditioned at 53% RH in an environmental chamber containing saturated solution of magnesium nitrate [Mg (NO3)2 · 6H2O] for at least 48 h prior to analysis. 10 mg of film specimen was hermetically sealed in aluminum pans and heated from −50 to 350°C at a rate of 5°C min−1. Empty aluminum pan was used as reference. Thermal parameters such as Peak temperature (Tm) and enthalpy change (ΔH) were computed from the DSC thermograms by the TA Universal Analysis Software (USA).
2.10. Scanning electron microscopy (SEM)
The surface and cross sectional microstructure of the pectin films, previously conditioned at 53% RH and 25°C, was analyzed with TM3030 scanning electron microscope (SEM; Hitachi Co. Ltd., Kyoto, Japan), working in High Vacuum mode, with 30 KV accelerating voltage and 10 mm working distance. Film pieces (2 × 2 mm) were mounted on aluminum stub with carbon tape and sputter coated with gold using DC sputter coater (AGAR B7340, Agar Scientific, Stansted, UK) for 30 s at 20 mA. All film samples were scanned using an accelerating beam of 15 kV.
2.11. X-ray diffraction (XRD)
XRD curves were obtained using X-ray Diffractometer (Rigaku, Tokyo, Japan) supplied with copper tube at 40 mA current. The radiation of the film specimens were obtained over a range of 2θ = 0-60° diffraction angel with step size of 2θ = 0.02°. Diffractograms were formed by ORIGIN PRO 8 software (USA).
2.12. DPPH radical scavenging assay
The radical scavenging potential of pectin membranes was checked by following the method suggested by (Jouki, Mortazavi, Yazdi, & Koocheki, Citation2014). The DDPH free radical scavenging activity was calculated as follows:
where Asample is representing the absorbance value of sample solution and Acontrol is indicating the absorbance of DPPH solution at wavelength of 517 nm.
2.13. Antimicrobial activity of the films
Antimicrobial activity was evaluated by using agar diffusion technique according to (Pranoto, Salokhe, & Rakshit, Citation2005). Three bacterial strains (E. coli, S. aureus and L. monocytogenes) were cultured in their respective mediums in incubator at 37°C for about 24–48 h. McFarland standard was used to adjust the density of bacterial suspensions to 108 CFU/mL. In order to determine Inhibition zones against each strain, films were cut into 1 cm circles and put on specific agar plates containing bacterial growth. The plates were examined for zones of inhibition after 24 h of incubation at 37°C.
2.14. Statistical analysis
All data was investigated with one-way ANOVA using SPSS (SPSS Inc., USA) software and presented as means ± SD. The differences between mean values were find out with Duncan’s multiple at a level of p < 0.05 significance.
3. Results and discussion
3.1. Phenolic composition in YAP
The individual phenolic contents in YAP were investigated by use of high-performance liquid chromatography (HPLC) and are summarized in . The detected polyphenolic compounds were enumerated in the order of their retention times. The content of total polyphenols in YAP was 68.64%, in which phlorizin accounted for the most (21.22%), followed by ellagic acid (10.11%) and procyanidin B2 (9.29%). Epicatechin, epigallocatechin, catechin, chlorogenic acid, catechin and rutin were also found in small concentration.
Figure 1. (a) HPLC chromatograms of 16 phenolic standards: 1. Gallic acid, 2. Protocatechuic acid, 3. Epigallocatechin 4. Catechin, 5. Procyanidin B2, 6. Chlorogenic acid, 7. 4-hydroxybenzoic acid, 8. Epicatechin, 9. Caffeic acid, 10. Epicatechin gallate, 11. Rutin, 12. Hyperin, 13. Ellagic acid, 14. Quercitin 15. Phloridzin, 16. Meletin. (b) HPLC chromatogram of Young apple polyphenols (YAP).
Figura 1. (a) Cromatogramas de HPLC de 16 patrones fenólicos: 1. Ácido gálico, 2. Ácido protocatechuico, 3. Epigalocatequina 4. Catequina, 5. Procianidina B2, 6. Ácido clorogénico, 7. Ácido 4-hidroxibenzoico, 8. Epicatequina, 9. Ácido cafeico, 10. Galato de epicatequina, 11. Rutina, 12. Hiperina, 13. Ácido elágico, 14. Quercetina 15. Florizina, 16. Meletin. (b) Cromatograma de HPLC de polifenoles de manzana joven (YAP).
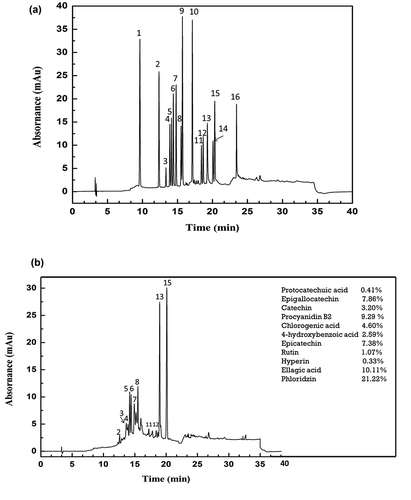
3.2. Film thickness and moisture content
The effects of YAP concentration on thickness and moisture content of the pectin/YAP films are shown in . The lowest thickness (0.060 mm) was observed in the films prepared without YAP, and by increasing the concentration of YAP in film forming solution. Thicker films were produced due to higher solid content introduced into the film matrix. The moisture content of the pectin films were significantly (p < 0.05) reduced after the addition of YAP. The control films showed the highest water content. However, after the addition of 0.5%, 1.0% and 1.5% YAP, the moisture content of pectin films decreased by 15.52%, 13.48% and 11.33%, respectively. Crosslinking of polysaccharides along with polyphenols has lowered the attraction of polymer particles towards moisture particles and proved helpful in creating films with low percentage of water content. Apple polyphenols may interact with polysaccharide molecules by potential hydrogen bonding, which limits the interactions with water molecules due to effect of competitive binding. Similar trend was observed for chitosan-based edible films after the addition of mineral and vitamin components (Park & Zhao, Citation2004).
Table 1. Physical properties of pectin films incorporated with YAP.
Tabla 1. Propiedades físicas de las películas de pectina a las que se incorporó YAP.
3.3. Solubility and swelling index
Solubility and swelling are both important characteristics of edible films because they can directly affect the water resistance properties of films, particularly in humid atmosphere. An increase (p < 0.05) in the solubility of films and the swelling degree was observed after increasing concentration of YAP in the film solutions (). For pure pectin films, the solubility in water and water uptake at equilibrium was the lowest at room temperature. The higher values of water solubility and swelling ratio of the YAP-pectin films may be attributed to the presence of hydrophilic groups of apple polyphenols that can interact with water molecules. The results of our study are in accordance with the previous study, in which incorporation of green tea and black tea extracts into chitosan films increased the solubility and swelling degree of chitosan films (Peng, Wu, & Li, Citation2013).
3.4. Water vapor permeability (WVP)
Water vapor permeability is an essential parameter to estimate the permeation of water vapors through the film matrix at any given temperature. The WVP value of pectin film was significantly (P < 0.05) decreased from 13.39 × 10−11 g·m−1s−1Pa−1 to 7.55 × 10−11 g·m−1s−1Pa−1 by increasing the amount of YAP from 0 to 1.5% in the film matrix, suggesting that the addition of YAP could improve the water barrier properties of the pectin films. One possible reason for enhancing the water resistance properties of the pectin/YAP films is the interactions between pectin and YAP indicated by the FTIR spectra, which could lessen the availability of the hydrophilic hydroxyl groups in pectin and diminish their interactions with water molecules. The mobility of pectin chains was affected negatively by interacting with polyphenols, so the diffusion or penetration of water molecules across the membranes was decreased. Polyphenols will not allow the water constituents to pass through polymeric substance easily, as they developed interconnecting bonds with film matrix. Wang et al. also observed a decrease in WVP values after the inclusion of tea polyphenols into chitosan based films (L. Wang, Dong, Men, Tong, & Zhou, Citation2013). According to them, the interaction between chitosan chain and tea polyphenols decreased the number of hydrophilic hydroxyl groups hence reduced water diffusion through the film surface. Similar finding were also observed by Du et al., that WVP of the apple films were significantly decreased after incorporation of apple skin polyphenol powder (Du, Olsen, Avena-Bustillos, Friedman, & McHugh, Citation2011).
3.5. Color and opacity of the films
Hunter Lab color values (L*, a* and b*) and total color difference (∆E) values were used to measure color properties of films. Pectin films without YAP were clear, colorless and showed a transparent appearance as expressed by highest ‘‘L” value, but the incorporation of polyphenols into the films led to the decrease in clarity and transparency of films. After the incorporation of YAP, a* and b* values of pectin films were significantly (p < 0.05) increased; however, the L* value was decreased, representing a tendency towards redness and darkness (). The increase in redness value of the resultant films could be attributed to the original color of YAP. The reduction in the edible film’s lightness may assist to avoid oxidative and enzymatic deterioration in wrapped foods triggered by the exposure to ultraviolet and visible light, leading to discoloration, nutrient losses and off-flavors (Rubilar et al., Citation2013). Siripatrawan and Harte (Citation2010) have also reported similar outcomes after the incorporation of green tea extract into chitosan based films.
Table 2. Color and opacity of pectin films incorporated with YAP.
Tabla 2. Color y opacidad de las películas de pectina a las que se incorporó YAP.
Control films were more transparent (indicated by lower opacity value) as compared to films incorporated with YAP (). This might be due to light-scattering effect of the emulsion that occurred by adding apple polyphenols into the pectin films. A decrease in film transparency has also been reported by (Qin et al., Citation2015) after incorporating pomegranate rind extract in to chitosan films.
3.6. Mechanical properties of the films
TS is recognized as the maximum stress supported by a film before breakage, elongation ratio (ER) is the maximum extension percentage of a film before rupture. Elastic modulus (EM) specifies the rigidity of a film (Srinivasa, Ramesh, & Tharanathan, Citation2007). The TS, EM and ER of the YAP-pectin films are shown in . The control film had the lowest TS (14.08 MPa) and highest ER (7.14%) values in all the film samples. With increasing YAP content up to 1.5%, the TS value was increased to 22.78 MPa, while the ER value was decreased significantly (3.41%).
Figure 2. Mechanical properties of pectin films incorporated with Young apple polyphenols (YAP). Results with different letters are significantly different (p < 0.05).
Figura 2. Propiedades mecánicas de las películas de pectina a las que se incorporaron polifenoles de manzana joven (YAP). Los resultados con letras diferentes son significativamente diferentes (p < 0.05).
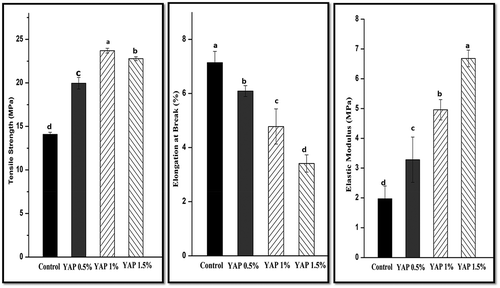
Mechanical properties of polysaccharide based film mainly depends on the interactions of particles, plasticizers and others compounds with the polymer network (Lorevice, Otoni, Moura, & Mattoso, Citation2016). Similar interactions between chitosan molecules and catechin have also been observed (Zhang & Kosaraju, Citation2007). It has been stated that the interaction mechanisms of various polyphenols with polysaccharide polymer are due to ester linkages, electrostatic interactions and hydrogen bonding (Silva-Weiss, Bifani, Ihl, Sobral, & Gómez-Guillén, Citation2013). An increase in TS value of edible polysaccharide film by phenolic extracts was also observed in previous studies; (Gómez-Estaca, Giménez, Montero, & Gómez-Guillén, Citation2009; Gómez-Guillén, Ihl, Bifani, Silva, & Montero, Citation2007; Siripatrawan & Harte, Citation2010).
3.7. Fourier transform infrared spectroscopy (FTIR)
FTIR spectroscopy was done to determine the intermolecular interactions within the film matrix. ATR-FTIR spectra of pectin/YAP films and YAP are shown in ,). The broad area of absorption between 3400 and 2500 cm−1 was allocated to O–H stretching vibrations due to both inter- and intra-molecular hydrogen bonding. The absorption band around 2933 cm−1 were credited to stretching of C-H bonds, these involved the CH, CH2 and CH3 stretching and bending vibrations (Lorevice et al., Citation2016). The strong absorption bands occurring between 1760–1730 cm−1 was attributed to the ester carbonyl groups and the absorption vibrations present at 1630–1600 cm−1 were allocated to stretching of free carboxylate groups. Carboxylate groups showed two absorption bands, an asymmetrical stretching of carboxylate ions near 1630–1600 cm−1 and a weaker symmetric stretching band near 1400 cm−1 (Gnanasambandam & Proctor, Citation2000). Besides, the bands at 1100–1040 cm−1 were assigned to C–O–C stretching vibrations of the polymer chain structure (Singthong, Cui, Ningsanond, & Goff, Citation2004).
Figure 3. (a) ATR-FTIR spectrum of pectin films incorporated with Young apple polyphenols (YAP). (b) ATR-FTIR spectrum of Young apple polyphenols (YAP) (C) Simplified representation of interaction between pectin chains and YAP molecules.
Figura 3. (A) Espectro ATR-FTIR de películas de pectina a las que se incorporaron polifenoles de manzana joven (YAP). (B) Espectro ATR-FTIR de polifenoles de manzana joven (YAP). (C) Representación simplificada de la interacción entre las cadenas de pectina y las moléculas de YAP.
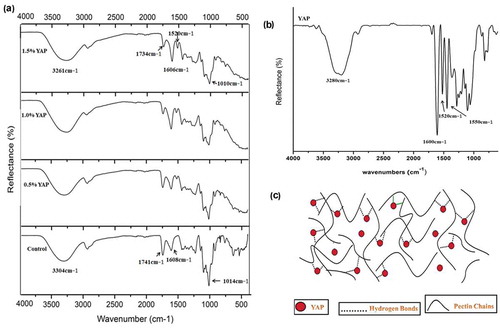
It was observed that the broad peak at 3304 cm−1 was moved to 3261 cm−1 by the addition of YAP ()), representing the reduced broadening of O-H bonds due to the interactions between polyphenols and pectin. Similar changes were observed by (Peng et al., Citation2013) and (Zhang & Kosaraju, Citation2007). Furthermore, it can be seen that the absorption peak located at 1741 cm−1 was shifted to 1734 cm−1 with increasing YAP concentration. Another obvious change was the emergence of a new peak at 1520 cm−1, indicating that functional group of phenolic compounds were detected and appeared to be more recognizable with increasing YAP concentration. Therefore, alterations in the control pectin film spectrum triggered by the inclusion of YAP suggest that polyphenols can develop hydrogen bonding with polymer molecules (), and consequently lower the number of free hydrogen groups which can form hydrogen bonds with water molecules. As an outcome, improved water barrier and mechanical properties and improved antioxidant activity were observed in the pectin films incorporated with YAP.
3.8. Differential scanning calorimetry (DSC)
Thermal stability of YAP incorporated pectin films was determined by DSC () analysis. According to (Einhorn-Stoll & Kunzek, Citation2009) thermal behavior of pectin depends primarily on the chemical composition and state transitions occurred during processing. In the current study the pectin‑YAP films presented two intense peaks in the DSC thermograms, including an endothermic transition peak (T1) at temperature around 100°C that is attributed to the loss of adsorbed and structural water, and an exothermic transition peak (T2) at temperature around 230°C that is responsible for the decomposition of pectin (Einhorn-Stoll, Kunzek, & Dongowski, Citation2007).
Figure 4. (a) DSC thermograms of Pectin films containing different percentage of Young apple polyphenols (YAP). (b) SEM micrographs of films’ surfaces: (a.1) control; (b.1) pectin films with 0.5% YAP; (c.1) pectin films with 1% YAP; (d.1) pectin films with 1.5% YAP and SEM micrographs of films’ cross-sections: (a.2) control film;(b.2) pectin films with 0.5%YAP; (c.2) pectin films with 1% YAP; (d.2) pectin films with 1.5% YAP.
Figura 4. (a) Termogramas DSC de películas de pectina que contienen diferentes porcentajes de polifenoles de manzana joven (YAP). (b) Micrografías SEM de las superficies de las películas: (a.1) control; (b.1) películas de pectina con 0.5% de YAP; (c.1) películas de pectina con 1% de YAP; (d.1) películas de pectina con 1.5% de YAP y micrografías SEM de secciones transversales de películas: (a.2) película de control; (b.2) películas de pectina con 0.5% de YAP; (c.2) películas de pectina con 1% de YAP; (d.2) películas de pectina con 1.5% de YAP.
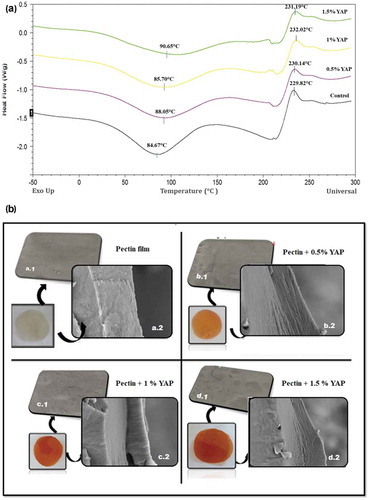
The first temperature transition (T1) for pectin/YAP films is associated with the evaporation of water. The Hydrogen bonding between water and phenolic compounds increased the endothermic temperature (T1) of the films from 84.67°C to 90.65°C by incorporating YAP. The endothermic peak (T1) of YAP integrated films was also found to be broader than control film. The DSC degradation peaks (T2) for 0.5%, 1% and 1.5% YAP/pectin films were appeared at 229.82°C, 230.14°C, 232.02°C and 231.19°C, respectively, showing that thermal stability of the films were increased slightly (). However, the enthalpy of the melting (ΔHm) in exothermic peak was decreased, representing that YAP has increased the thermal stability of films while reduced the heat generated by the decomposition of pectin. It has been stated that the thermal behavior of pectin molecule is largely defined by its internal and external bonding and on its configuration (Wang et al., Citation2016). The increase in degradation temperature for pectin/YAP films can be linked the H bonds formed between polyphenolic compounds (phlorizin, chlorogenic acid, Epicatechin, ellagic acid) of apple polyphenols and pectin. For instance, a larger amount of hydrogen bonding requires more heat to break down the structure of YAP-pectin complex. Similar results were also observed for chitosan films incorporated with tocopherol (Martins, Cerqueira, & Vicente, Citation2012) and by addition of apple skin polyphenols in acai-pectin edible films (Espitia et al., Citation2014).
Table 3. Thermal Characteristics of pectin films with YAP.
Tabla 3. Características térmicas de las películas de pectina a las que se incorporó YAP.
3.9. Scanning electron microscopy (SEM)
The microstructure of the edible films is influenced by the spatial organization of its components and by how they interact with each other during the drying process. SEM can give a better understanding of the structural properties of the film like water vapor transmission, mechanical and optical properties (Vargas, Albors, Chiralt, & González-Martínez, Citation2009). Surface and cross sectional SEM micrographs of pectin films () showed that YAP (in different concentrations) were uniformly incorporated in the pectin matrix. The reference film showed a smooth, compact and continuous microstructure without a porous and grainy structure. The images of pectin/YAP films proposed that polyphenols were completely assimilated and well distributed in the matrix and did not cause irregularities. However, when higher concentration of YAP was used, the film’s microstructure was altered slightly and caused a denser configuration.
3.10. X-ray diffraction analysis (XRD)
XRD analysis was used to investigate the crystalline structure and assess the compatibility of pectin and YAP. Well defined peaks at 12.70, 16.30°, 18.40°, 25.32° and 40.14° of 2θ related to pure pectin has been reported (Chaichi, Hashemi, Badii, & Mohammadi, Citation2017; Meneguin, Cury, & Evangelista, Citation2014). In the present study, pectin film exhibited a broad peak at 15.72° of 2θ confirming the presence of some crystalline structure (). Incorporation of YAP into pectin films did not change the peak position in the XRD pattern significantly. However, as the YAP content in pectin based films increased, the curve intensities diminished slightly (). The variation in diffraction peaks after the addition of YAP was possibly caused by substitution of Inter- and intra-molecular hydrogen bonds of pure pectin by YAP-pectin hydrogen bonds, thereby reducing the pectin intermolecular attraction. No new diffraction peaks were noticed in composite YAP/pectin films.
Figure 5. (a) XRD spectra of pectin films containing different percentage of Young apple polyphenols (YAP). (b) DPPH scavenging of pectin films incorporated with YAP (results with different letters are significantly different (p < 0.05).
Figura 5. (a) Espectros XRD de películas de pectina que contienen diferentes porcentajes de polifenoles de manzana joven (YAP). (b) Eliminación de DPPH de películas de pectina a las que se incorporó YAP (los resultados con letras diferentes son significativamente diferentes (p < 0.05).
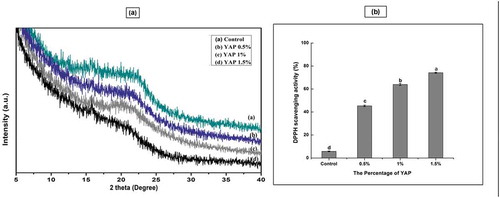
3.11. Antioxidant properties of the films
The DPPH assay was used to check the ability of pectin/YAP films to act as free radical scavengers and to determine their antioxidant potential. shows the antioxidant potential of the YAP-pectin films. The control films showed a low DPPH scavenging activity. However, the highest scavenging activity was exhibited by pectin films with 1.5% YAP. The present finding are in agreement with one previous study, in which the antioxidant property of chitosan films was significantly increased by the addition of tea polyphenols (Wang et al., Citation2013).
3.12. Antimicrobial activity of the films
Disk Diffusion assay was performed to determine the antimicrobial properties of YAP/pectin edible films against S. aureus, E. coli and L. monocytogenes. The control pectin film did not show any growth of inhibition against three pathogenic bacteria. The zones of inhibitions against all tested microorganisms were increased as the concentration YAP was increased ( and ). The antibacterial properties of polyphenol-polysaccharide edible films were also found in previous studies (Du et al., Citation2011; Espitia et al., Citation2014). The antibacterial effects of YAP films was may be due to the action of bioactive phenolic compounds that exerted the physiological changes of the microbial cell membrane, and eventually resulted in bacteria death (Kabir, Katayama, Tanji, & Nakamura, Citation2014). Alberto et al. (Citation2006) have also reported the bactericidal effect of polyphenols from apple skin against E. coli, S. aureus, Pseudomonas aeruginosa, and L. monocytogenes.
Table 4. Antimicrobial effect of pectin films incorporated with YAP against Staphylococcus aureus, Escherichia coli and Listeria monocytogenes.
Tabla 4. Efecto antimicrobiano de las películas de pectina a las que se incorporó YAP contra Staphylococcus aureus, Escherichia coli y Listeria monocytogenes.
Figure 6. Inhibitory effects of YAP-pectin films on the growth of (a) Staphylococcus aureus, (b), Escherichia coli, (c), Listeria monocytogenes.
Figura 6. Efectos inhibitorios de las películas de pectina YAP sobre el crecimiento de (a) Staphylococcus aureus; (b) Escherichia coli; y (c) Listeria monocytogenes.
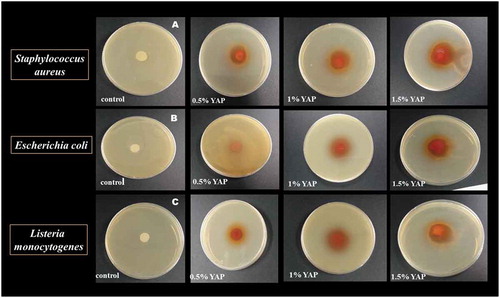
4. Conclusion
The inclusion of YAP contributed to decrease the moisture content and WVP of the films, suggesting improved water barrier properties of the resulting films. The composite films were more rigid, less flexible and less transparent as compared to control films. The alterations, as verified by FTIR analysis, could be credited to the interaction between functional groups of pectin and YAP. SEM analysis revealed that YAP was homogenously distributed across the film matrix. DSC exposed that the thermal stability of the pectin films increased slightly by the addition of YAP. Additionally, the films containing YAP exhibited a large inhibitory effect against foodborne bacteria. The developed packaging material can have applications in packaging of wide range of food products, particularly those that are highly oxidative and microbial sensitive. The can be used as packaging wraps to inactivate pathogenic bacteria on fruits, meat, and minimally processed ready to eat products. Moreover, these films can also be applied inside the heterogeneous foodstuffs at the interfaces between different layers of the components.
Disclosure statement
The author claims no conflict of interest in this work.
Additional information
Funding
References
- Alberto, M. R., Canavosio, M. A. R., & Manca de Nadra, M. C. (2006). Antimicrobial effect of polyphenols from apple skins on human bacterial pathogens. Electronic Journal of Biotechnology, 9(3), 205–209. doi:10.2225/vol9-issue3-fulltext-1
- Azeredo, H. M., Mattoso, L. H., Wood, D., Williams, T. G., Avena-Bustillos, R. J., & McHugh, T. H. (2009). Nanocomposite edible films from mango puree reinforced with cellulose nanofibers. Journal of Food Science, 74(5), N31–35. doi:10.1111/j.1750-3841.2009.01186.x
- Azeredo, H. M. C., Morrugares-Carmona, R., Wellner, N., Cross, K., Bajka, B., & Waldron, K. W. (2016). Development of pectin films with pomegranate juice and citric acid. Food Chemistry, 198, 101–106. doi:10.1016/j.foodchem.2015.10.117
- Bao, S., Xu, S., & Wang, Z. (2009). Antioxidant activity and properties of gelatin films incorporated with tea polyphenol-loaded chitosan nanoparticles. Journal of Scencei and Food Agriculture, 89(15), 2692–2700. doi:10.1002/jsfa.3775
- Cabello, S. D. P., Takara, E. A., Marchese, J., & Ochoa, N. A. (2015). Influence of plasticizers in pectin films: Microstructural changes. Materials Chemistry and Physics, 162, 491–497. doi:10.1016/j.matchemphys.2015.06.019
- Chaichi, M., Hashemi, M., Badii, F., & Mohammadi, A. (2017). Preparation and characterization of a novel bionanocomposite edible film based on pectin and crystalline nanocellulose. Carbohydrate Polymers, 157, 167–175. doi:10.1016/j.carbpol.2016.09.062
- Du, W. X., Olsen, C. W., Avena-Bustillos, R. J., Friedman, M., & McHugh, T. H. (2011). Physical and antibacterial properties of edible films formulated with apple skin polyphenols. Journal of Food Science, 76(2), M149–155. doi:10.1111/j.1750-3841.2010.02012.x
- Eca, K. S., Machado, M. T., Hubinger, M. D., & Menegalli, F. C. (2015). Development of active films from pectin and fruit extracts: Light protection, antioxidant capacity, and compounds stability. Journal of Food Science, 80(11), C2389–2396. doi:10.1111/1750-3841.13074
- Einhorn-Stoll, U., & Kunzek, H. (2009). The influence of the storage conditions heat and humidity on conformation, state transitions and degradation behaviour of dried pectins. Food Hydrocolloids, 23(3), 856–866. doi:10.1016/j.foodhyd.2008.05.001
- Einhorn-Stoll, U., Kunzek, H., & Dongowski, G. (2007). Thermal analysis of chemically and mechanically modified pectins. Food Hydrocolloids, 21(7), 1101–1112. doi:10.1016/j.foodhyd.2006.08.004
- Espitia, P. J., Avena-Bustillos, R. J., Du, W. X., Chiou, B. S., Williams, T. G., Wood, D., … Soares, N. F. (2014). Physical and antibacterial properties of acai edible films formulated with thyme essential oil and apple skin polyphenols. Journal of Food Science, 79(5), M903–910. doi:10.1111/1750-3841.12432
- Giosafatto, C. V., Di Pierro, P., Gunning, P., Mackie, A., Porta, R., & Mariniello, L. (2014). Characterization of Citrus pectin edible films containing transglutaminase-modified phaseolin. Carbohydrate Polymers, 106, 200–208. doi:10.1016/j.carbpol.2014.02.015
- Gnanasambandam, R., & Proctor, A. (2000). Determination of pectin degree of esterification by diffuse reflectance fourier transform infrared spectroscopy. Food Chemistry, 68, 327–332. doi:10.1016/S0308-8146(99)00191-0
- Gómez-Estaca, J., Giménez, B., Montero, P., & Gómez-Guillén, M. C. (2009). Incorporation of antioxidant borage extract into edible films based on sole skin gelatin or a commercial fish gelatin. Journal of Food Engineering, 92(1), 78–85. doi:10.1016/j.jfoodeng.2008.10.024
- Gómez-Guillén, M. C., Ihl, M., Bifani, V., Silva, A., & Montero, P. (2007). Edible films made from tuna-fish gelatin with antioxidant extracts of two different murta ecotypes leaves (Ugni molinae Turcz). Food Hydrocolloids, 21(7), 1133–1143. doi:10.1016/j.foodhyd.2006.08.006
- He, R. R., Wang, M., Wang, C. Z., Chen, B. T., Lu, C. N., Yao, X. S., … Kurihara, H. (2011). Protective effect of apple polyphenols against stress-provoked influenza viral infection in restraint mice. Journal of Agriculture and Food Chemistry, 59(8), 3730–3737. doi:10.1021/jf104982y
- Jouki, M., Mortazavi, S. A., Yazdi, F. T., & Koocheki, A. (2014). Characterization of antioxidant-antibacterial quince seed mucilage films containing thyme essential oil. Carbohydrate Polymers, 99, 537–546. doi:10.1016/j.carbpol.2013.08.077
- Kabir, F., Katayama, S., Tanji, N., & Nakamura, S. (2014). Antimicrobial effects of chlorogenic acid and related compounds. Journal of the Korean Society for Applied Biological Chemistry, 57(3), 359–365. doi:10.1007/s13765-014-4056-6
- Lorevice, M. V., Otoni, C. G., Moura, M. R. D., & Mattoso, L. H. C. (2016). Chitosan nanoparticles on the improvement of thermal, barrier, and mechanical properties of high- and low-methyl pectin films. Food Hydrocolloids, 52, 732–740. doi:10.1016/j.foodhyd.2015.08.003
- Martins, J. T., Cerqueira, M. A., & Vicente, A. A. (2012). Influence of α-tocopherol on physicochemical properties of chitosan-based films. Food Hydrocolloids, 27(1), 220–227. doi:10.1016/j.foodhyd.2011.06.011
- Meneguin, A. B., Cury, B. S., & Evangelista, R. C. (2014). Films from resistant starch-pectin dispersions intended for colonic drug delivery. Carbohydrate Polymers, 99, 140–149. doi:10.1016/j.carbpol.2013.07.077
- Nisar, T., Wang, Z. C., Yang, X., Tian, Y., Iqbal, M., & Guo, Y. (2018). Characterization of citrus pectin films integrated with clove bud essential oil: Physical, thermal, barrier, antioxidant and antibacterial properties. International Journal of Biological Macromolecules, 106, 670–680. doi:10.1016/j.ijbiomac.2017.08.068
- Otoni, C. G., Moura, M. R. D., Aouada, F. A., Camilloto, G. P., Cruz, R. S., Lorevice, M. V., & Mattoso, L. H. C. (2014). Antimicrobial and physical-mechanical properties of pectin/papaya puree/cinnamaldehyde nanoemulsion edible composite films. Food Hydrocolloids, 41, 188–194. doi:10.1016/j.foodhyd.2014.04.013
- Park, S., & Zhao, Y. (2004). Incorporation of a high concentration of mineral or vitamin into chitosan-based films. Journal of Agriculture and Food Chemistry, 52, 1933−1939. doi:10.1021/jf034612p
- Park, S., & Zhao, Y. (2006). Development and characterization of edible films from cranberry pomace extracts. Journal of Food Science, 71(2), 95–101. doi:10.1111/j.1365-2621.2006.tb08902.x
- Peng, Y., & Li, Y. (2014). Combined effects of two kinds of essential oils on physical, mechanical and structural properties of chitosan films. Food Hydrocolloids, 36, 287–293. doi:10.1016/j.foodhyd.2013.10.013
- Peng, Y., Wu, Y., & Li, Y. (2013). Development of tea extracts and chitosan composite films for active packaging materials. International Journal of Biological Macromolecules, 59, 282–289. doi:10.1016/j.ijbiomac.2013.04.019
- Petersen, K., Væggemose Nielsen, P., Bertelsen, G., Lawther, M., Olsen, M. B., Nilsson, N. H., & Mortensen, G. (1999). Potential of biobased materials for food packaging. Trends in Food Science & Technology, 10(2), 52–68. doi:10.1016/S0924-2244(99)00019-9
- Pranoto, Y., Salokhe, V. M., & Rakshit, S. K. (2005). Physical and antibacterialproperties of alginate-based edible film incorporated with garlic oil. Food Research International, 38(3), 267–272. doi:10.1016/j.foodres.2004.04.009
- Qin, Y. Y., Zhang, Z. H., Li, L., Yuan, M. L., Fan, J., & Zhao, T. R. (2015). Physio-mechanical properties of an active chitosan film incorporated with montmorillonite and natural antioxidants extracted from pomegranate rind. Journal of Food Science and Technology, 52(3), 1471–1479. doi:10.1007/s13197-013-1137-1
- Rubilar, J. F., Cruz, R. M. S., Silva, H. D., Vicente, A. A., Khmelinskii, I., & Vieira, M. C. (2013). Physico-mechanical properties of chitosan films with carvacrol and grape seed extract. Journal of Food Engineering, 115(4), 466–474. doi:10.1016/j.jfoodeng.2012.07.009
- Sánchez Aldana, D., Andrade-Ochoa, S., Aguilar, C. N., Contreras-Esquivel, J. C., & Nevárez-Moorillón, G. V. (2015). Antibacterial activity of pectic-based edible films incorporated with Mexican lime essential oil. Food Control, 50, 907–912. doi:10.1016/j.foodcont.2014.10.044
- Silva-Weiss, A., Bifani, V., Ihl, M., Sobral, P. J. A., & Gómez-Guillén, M. C. (2013). Structural properties of films and rheology of film-forming solutions based on chitosan and chitosan-starch blend enriched with murta leaf extract. Food Hydrocolloids, 31(2), 458–466. doi:10.1016/j.foodhyd.2012.11.028
- Singthong, J., Cui, S. W., Ningsanond, S., & Goff, H. D. (2004). Structural characterization, degree of esterification and some gelling properties of krueo ma noy (Cissampelos pareira) pectin. Carbohydrate Polymers., 58(4), 391–400. doi:10.1016/j.carbpol.2004.07.018
- Siripatrawan, U., & Harte, B. R. (2010). Physical properties and antioxidant activity of an active film from chitosan incorporated with green tea extract. Food Hydrocolloids, 24(8), 770–775. doi:10.1016/j.foodhyd.2010.04.003
- Srinivasa, P. C., Ramesh, M. N., & Tharanathan, R. N. (2007). Effect of plasticizers and fatty acids on mechanical and permeability characteristics of chitosan films. Food Hydrocolloids, 21(7), 1113–1122. doi:10.1016/j.foodhyd.2006.08.005
- Sun, L., Guo, Y., Fu, C., Li, J., & Li, Z. (2013). Simultaneous separation and purification of total polyphenols, chlorogenic acid and phlorizin from thinned young apples. Food Chemistry, 136(2), 1022–1029. doi:10.1016/j.foodchem.2012.09.036
- Sun, L., Sun, J., Thavaraj, P., Yang, X., & Guo, Y. (2017). Effects of thinned young apple polyphenols on the quality of grass carp (Ctenopharyngodon idellus) surimi during cold storage. Food Chemistry, 224, 372–381. doi:10.1016/j.foodchem.2016.12.097
- Sutherland, W. J., Clout, M., Cote, I. M., Daszak, P., Depledge, M. H., Fellman, L., … Watkinson, A. R. (2010). A horizon scan of global conservation issues for 2010. Trends in Ecology & Evolution, 25(1), 1–7. doi:10.1016/j.tree.2009.10.003
- Vargas, M., Albors, A., Chiralt, A., & González-Martínez, C. (2009). Characterization of chitosan–Oleic acid composite films. Food Hydrocolloids, 23(2), 536–547. doi:10.1016/j.foodhyd.2008.02.009
- Wang, L., Dong, Y., Men, H., Tong, J., & Zhou, J. (2013). Preparation and characterization of active films based on chitosan incorporated tea polyphenols. Food Hydrocolloids, 32(1), 35–41. doi:10.1016/j.foodhyd.2012.11.034
- Wang, W., Ma, X., Jiang, P., Hu, L., Zhi, Z., Chen, J., & Liu, D. (2016). Characterization of pectin from grapefruit peel: A comparison of ultrasound-assisted and conventional heating extractions. Food Hydrocolloids, 61, 730–739. doi:10.1016/j.foodhyd.2016.06.019
- Zhang, L., & Kosaraju, S. L. (2007). Biopolymeric delivery system for controlled release of polyphenolic antioxidants. European Polymer Journal, 43(7), 2956–2966. doi:10.1016/j.eurpolymj.2007.04.033
- Zhong, Y., Song, X., & Li, Y. (2011). Antimicrobial, physical and mechanical properties of kudzu starch–Chitosan composite films as a function of acid solvent types. Carbohydrate Polymers, 84(1), 335–342. doi:10.1016/j.carbpol.2010.11.041