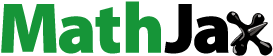
ABSTRACT
The addition of red cactus pear peel and its mucilage on color, bioactive compounds and antioxidant capacity (AC) of yogurt was investigated. Peel and its mucilage were dried and used for the formulation of different yogurt blends following a constrained mixture design. Color, total phenolic compounds, total flavonoids, total betalains and AC were evaluated in formulated yogurts. An optimization process was conducted thereafter, and the bioactive compounds and AC of this yogurt were evaluated before and after gastrointestinal simulation. Results indicated that cactus pear peel and its mucilage give a nice magenta color to the yogurts, and also increase the bioactive compounds and AC of them. Optimization procedure indicates that yogurt formulated with 5.5% of cactus pear peel and 7.5% of mucilage displays the highest bioactive compounds and AC; moreover, they were significantly increased (p < 0.05) by the simulated gastrointestinal process.
RESUMEN
En el presente estudio se investigaron los efectos provocados por la adición de cáscara de tuna roja y su mucílago en el color, los compuestos bioactivos y la capacidad antioxidante (CA) del yogurt. Para ello se secaron la cáscara y su mucílago, y se los usó para formular diferentes mezclas de yogurt siguiendo un diseño de mezclas restringido. En los yogures formulados se evaluaron el color, los compuestos fenólicos totales, los flavonoides totales, las betalaínas totales y la CA. Posteriormente se aplicó un proceso de optimización, y se evaluaron los compuestos bioactivos y la CA de este yogur antes y después de la simulación gastrointestinal. Los resultados indican que la cáscara de tuna y su mucílago otorgan un color magenta agradable a los yogures y aumentan los compuestos bioactivos y la CA presentes en ellos. El procedimiento de optimización indica que el yogurt formulado con 5.5% de cáscara de tuna y 7.5% de mucílago muestra los mayores valores de compuestos bioactivos y CA; además, éstos se incrementaron significativamente (p < 0.05) después de la aplicación del proceso gastrointestinal simulado.
1. Introduction
Tendencies and demands of consumers have been focusing on food products with high sensory and nutritional quality, as fresh as possible and free of synthetic additives or chemical preservatives (Asioli et al., Citation2017). However, the knowledge in nutrition and its relation on human health is constantly increasing and nowadays has focused on studying the effect of non-nutrient compounds and their relation to maintain good health and reduce the appearance of different diseases (Tapsell, Neale, Satija, & Hu, Citation2016). On the other hand, the increase of chronical degenerative diseases such as cancer, diabetes, obesity, chronic heart disease, among others has driven consumers to demand foods with high content of bioactive compounds such as fiber, vitamins, pigments, minerals, terpenoids and phenolic compounds (Sharma et al., Citation2016; Tresserra-Rimbau et al., Citation2014). Thus, a frequent consumption of natural and formulated plant-based foods is necessary for an adequate intake of these compounds (Luna-Guevara, Luna-Guevara, Hernández-Carranza, Ruíz-Espinosa, & Ochoa-Velasco, Citation2019; Stagos et al., Citation2012).
Fruits and vegetables are a well-known source of bioactive compounds; however, during their processing several by-products such as peel, seed, core, and pomace are obtained, reaching between 25% and 30% of wastes of a whole commodity group (Ayala-Zavala et al., Citation2011; Sagar, Pareek, Sharma, Yahia, & Lobo, Citation2018). These by-products possess in many cases different and/or higher content of bioactive compounds that the traditionally exploited portion of the vegetable; therefore, they may be used for the fortification and/or formulation of food products (Berto, Braga, Evelázio, Fernandes, & Campos, Citation2015; Elleuch et al., Citation2011; Helkar, Sahoo, & Patil, Citation2016).
Cactus pear is the fruit of Opuntia ficus-indica L., is a well-accepted fruit in Mexico due to its juicy and sweet flavor (Ochoa-Velasco & Guerrero-Beltrán, Citation2012). Different studies have indicated that cactus pear contains bioactive compounds such as polyphenols and betalains, which provide it high antioxidant capacity and attractive color (Ochoa-Velasco & Guerrero-Beltrán, Citation2012; Otálora, Carriazo, Iturriaga, Nazareno, & Osorio, Citation2015). In this sense, betalains are natural plant pigments which can be divided into red-violet betacyanins or yellow betaxanthins; these pigments are gaining popularity for using as a natural colorant in the food industry (Gengatharan, Dykes, & Choo, Citation2015). However, one of the main problems of cactus pear is its high amount of peel, representing about 40–45% of the whole fruit (Ochoa-Velasco & Guerrero-Beltrán, Citation2014, Citation2016). As many other fruits, the peel of cactus pear has a considerable amount of fiber and bioactive compounds, which are generally discarded. However, cactus pear peel is a good source of mucilage, which is a heteropolysaccharide constituted of L-arabinose, D-galactose, D-xylose, L-rhamnose, D-galacturonic acid, etc. It has been associated with different health benefits (Otálora et al., Citation2015) and with a great capacity to absorb water and act as a hydrocolloid (Hernández-Carranza et al., Citation2019). However, there is evidence which indicates that these complex carbohydrates directly interact with the bioactive compounds of food, affecting their bioaccessibility and bioavailability in the gastrointestinal process (Palafox-Carlos, Ayala-Zavala, & González-Aguilar, Citation2011).
Yogurt is a dairy product obtained by the fermentation process carried out by Streptococcus thermophilus and Lactobacillus delbruckii spp. bulgaricus (German, Citation2014). It is an excellent source of proteins, medium-chain fatty acids, vitamins, and minerals, and its continuous consumption causes a slight reduction in stomach pH reducing the risk of illnesses caused by pathogenic microorganisms (Hashemi, Hadi, Mesbahi, & Amin, Citation2015). Moreover, probiotic microflora of yogurt has shown to induce measurable health benefits, as it improves lactose digestion, reduces the acute diarrheal disorders, enhances the immune response in immunocompromised population, induces anti-tumorigenic activities and reduces risk of colon cancers (Fazilah, Ariff, Khayat, Rios-Solis, & Halim, Citation2018; Guarner et al., Citation2005). Although yogurt is one of the most complete foods, it is not considered a good source of bioactive compounds such as fiber, phenolic compounds, and pigments; therefore, its supplementing with plant-based products has been evaluated (Hashemi et al., Citation2015; Iriondo-DeHond, Miguel, & Del Castillo, Citation2018). For example, passion fruit, orange and date by-products were added as a source of fiber (García-Pérez et al., Citation2005; Hashim, Khalil, & Afifi, Citation2009; Viva de Toledo et al., Citation2018), pomegranate peel extract and seed as sources of phenolic compounds and antioxidant capacity (El-Said, Haggag, Fakhr, Gad, & Farahat, Citation2014; Ersöz, Kınık, Yerlikaya, & Açu, Citation2011), pineapple waste powder as antioxidant and antimutagenic (Sah, Vasiljevic, McKechnie, & Donkor, Citation2016) and herbs extracts to enhance the antioxidant activity (Dabija, Codină, Ropciuc, Gâtlan, & Rusu, Citation2018). However, the use of fruit, seed or peel from cactus pear has not been evaluated yet. Therefore, the aim of this study was to evaluate the effect of cactus pear peel and its mucilage addition on the antioxidant compounds content and color of yogurt and to evaluate the gastric simulation effect in a selected yogurt.
2. Material and methods
2.1. Chemical and reagents
All chemical reagents and solvents used in this study were obtained from Sigma-Aldrich, Inc. (Toluca, Mexico) and J.T. Baker (Mexico City, Mexico), respectively.
2.2. Yogurt and plant material
Fresh natural yogurt was purchased from a local producer of Puebla, Puebla, Mexico. Red cactus pear (Opuntia ficus-indica L.) was obtained from San Sebastian Villanueva, Puebla, Mexico. Fruits were selected according to their homogeneous red color, free from physical and microbiological appearance damage. Cactus pears were washed and disinfected using a sodium hypochlorite solution (100 mg L−1) and dried with absorbent paper. The peel was manually obtained using a stainless-steel knife, then cut in squares (1 cm2) and dried at 60°C until constant weight (24 h approximately). Dried peel was grinded and sieved to obtain a fine powder (180 µm). To obtain the mucilage, peel powder and water were mixed in a 1:20 mass ratio (Hernández-Carranza et al., Citation2019). The mixture was stirred at 40°C, 300 rpm for 10 min. Then, it was centrifuged (Premiere XC-2450, USA) at 4000 rpm for 20 min, the supernatant was recovered and dried for 36 h at 60°C. Dried sample was grinded and sieved to obtain a fine powder (75 µm). Both peel powder and mucilage were stored at room temperature in a hermetic bottle covered from the light.
2.3. Formulation of natural yogurt added with cactus pear peel powder and its mucilage
The effect of the addition of red cactus pear peel (i = 1) and its mucilage (i = 2) to enhance the bioactive compounds and antioxidant capacity of natural yogurt (i = 3) was evaluated using a constrained mixture design with 9 formulations (, ), including the vertices (A, B, C, and D), edges (E, F, G, and H) and centroid (I) of the rhombus-shaped factor space. Cactus pear peel and mucilage were explored with a maximum substitution of 10% (preliminary experiments) each in yogurt blend (0 ≤ x1, x2 ≤ 0.1, 0.8 ≤ x3 ≤ 1). Experimental responses were analyzed according to the following second-order modified L-pseudocomponent model (Cornell, Citation2002):
Table 1. Mixture design for the formulation of yogurt added with peel and mucilage powder of red cactus pear.
Tabla 1. Diseño de mezclas para la formulación de yogurt adicionado con polvo de cáscara y mucílago de tuna roja.
Figure 1. Constrained mixture design for the formulation of yogurt (x3) added with peel (x1) and mucilage (x2) powder of red cactus pear.
Figura 1. Diseño de mezcla restringido para la formulación de yogurt (x3) adicionado con polvo de cáscara (x1) y mucílago (x2) de tuna roja.

where y is the fitted response; b1, …, b23 are the model parameters and zi (i = 1,2,3) represents the pseudocomponents calculated according to:
In above equations k is the component with the largest range (Ri = Ui - Li, i = 1,2,3), where U and L are the upper and lower bounds of x (k = 3 in this study). Transformation of regular compositions (x) to pseudocomponents (z) is necessary to avoid unstable coefficient estimates due to collinearity arising in highly constrained regions such as that given in (Cornell, Citation2002).
2.4. Color
The color parameters of fresh and formulated yogurt were evaluated at the surface of each one using a precise colorimeter reader (TCR 200, Beijing). The L* (lightness), a* (red to green), and b* (yellow to blue) color parameter of the CIELAB color space were used to calculate the Hue and Chroma values following the next equations, respectively.
2.5. Bioactive compounds and antioxidant capacity determination
For determining the bioactive compounds and antioxidant capacity from red cactus pear peel powder, mucilage, fresh yogurt, and blends; extracts were obtained (Hernández-Carranza et al., Citation2019). The extract was obtained by placing 1 g of sample with 19 mL of distilled water, the mixture was stirred for 1 min using an electric stirrer (IKA Vortex 3, USA) and then centrifuged at 4000 rpm for 10 min. The supernatant was taken immediately to analyze the bioactive compounds and antioxidant capacity.
2.6. Total phenolic compounds
Total phenolic compounds (TPC) were evaluated according to the methodology proposed by Hernández-Carranza et al. (Citation2016). One milliliter of an adequate dilution of extract was mixed with 1 mL of Folin-Ciocalteau reagent (0.1 M) and 1 mL of Na2CO3 (0.5% w/v) solution (3 min later). The mixture was incubated for 30 min in a dark environment at room temperature. Total phenolic compounds were read at 765 nm using a Jenway UV-Vis spectrophotometer (model 6405, Staffordshire, UK). To quantify the total phenolic compounds a standard curve of gallic acid was done. The result was expressed as mg of gallic acid equivalents (GAE) per 100 g of sample.
2.7. Total flavonoids
Total flavonoids (TF) were analyzed following the methodology proposed by Hernández-Carranza et al. (Citation2016). 0.5 mL of an adequate dilution of extract was mixed with 0.5 mL of NaNO2 (1.5% w/v) in an amber glass tube, the mixture was vortexed and let stand for 5 min. Later, 1 mL of AlCl3 (3% w/v) was added and mixed for 1 min; then, 1 mL of NaOH (1 N) was added. The mixture was let to stand for 1 min and the absorbance was read at 490 nm using an UV-Vis spectrophotometer. To quantify the total flavonoids a standard curve of quercetin was done. The result was expressed as mg of quercetin per 100 g of sample.
2.8. Total betalains
Total betalains (TB) were reported as the sum of the betacyanins and betaxanthins following the methodology proposed by Stintzing, Herbach, & Mosshammer (Citation2005). Briefly, 1 mL of extract was diluted with Mcllvaine buffer (pH 6.5, citrate-phosphate) to obtain absorption values between 0.9 and 1.0. The concentration of betacyanins and betaxanthins were determined spectrophotometrically at 535 and 483 nm, respectively. For betacyanins a molecular weight of 550 g/mol, and molar extinction coefficient of 60,000 L/mol cm were used; while for indicaxanthin a molecular weight 308 g/mol, and molar extinction coefficient 48,000 L/mol cm were employed.
where B is the betacyanins or betaxanthins content (mg/100 g), A is the absorbance, DF is the dilution factor, MW is molecular weight (g/mol), l is the cell path (1 cm), ε is the extinction molar coefficient.
2.9. Antioxidant capacity
The antioxidant capacity (AC) was evaluated using both the iron (III) to iron (II) reduction (FRAP) assay and the inhibition of the DPPH (2,2-diphenyl-1-picrylhydrazyl) radical. For the first one, the methodology proposed by Dorman, Kosar, Kahlos, Holm, & Hiltunen (Citation2003) was used. One milliliter of extract was mixed with 2.5 mL of phosphate buffer (0.2 M, pH 7.0) and 2.5 mL of potassium hexacyanoferrate solution (1% w/v) and let to stand for 30 min at 50°C. Then, 2.5 mL of trichloroacetic acid solution (10% w/v) was added and the mixture was centrifuged for 10 min (1000 rpm). At least, 2.5 mL of the supernatant was mixed with 2.5 mL of water and 0.5 mL of FeCl3 solution (0.1% w/v) and the absorbance was read at 700 nm using an UV-Vis spectrophotometer. To quantify the reducing capacity a standard curve of ascorbic acid was done. The reducing capacity was expressed as mg of ascorbic acid (AA) per 100 g of sample. The inhibition of DPPH radical was conducted following the methodology proposed by Hernández-Carranza et al. (Citation2016). One milliliter of an adequate dilution of extract was added in an amber glass tube and mixed with 1 mL of DPPH (0.004% w/v) solution. The mixture was stirred and let stand in dark environment at room temperature for 30 min. Absorbance was recorded at 517 nm using an UV-Vis spectrophotometer. A standard curve of Trolox was used for quantifying the antioxidant capacity. Results were expressed as mg of Trolox equivalent per 100 g of sample.
2.10. Effect of cactus pear peel and mucilage on bioactive compounds and antioxidant capacity of yogurt formulation
The change in a given response resulting from a change in the proportions of component i (i = 1,2,3) while keeping constant the relative proportions of the remaining components is the effect of component i, and was investigated using the Cox’s direction, that is, the line or ray projected from a reference mixture (the centroid) to the vertex xi = 1 (i = 1,2,3; Cornell, Citation2002). If the coordinates of reference mixture are denoted by {r1, r2, r3} and the proportion of component i is changed in Δi, the formulations along Cox’s directions are obtained as:
Coordinates defined in Equations (7) and (8) are further used in Equation (1) to obtain the responses (TPC, TF, TB, AC FRAP, AC DPPH) along the xi ray.
2.11. Product optimization
The use of peel powder and mucilage added to natural yogurt was optimized to obtain a formulation with the highest level of bioactive compounds and antioxidant capacity. Yogurt blend was optimized with the desirability function approach (Cornell, Citation2002), involving the transformation of estimated responses yi (i = 1, 2, …, q) to a desirability value di, where 0 ≤ di ≤ 1. The desirability values are combined in the index:
The closer the value of D to 1, the higher overall desirability of the resulting blend. If Li is the minimum acceptable limit of yi and Ui is the limit above which a perfect desirability is achieved, then
On the other hand, if response is unacceptable when yi < Li or yi > Ui, and desirability of yi increases for Li ≤ yi ≤ Mi and decreases for Mi < yi ≤ Ui, then di is given by
In Equations (7) and (8), both s and t define the shape of the interpolation function, a straight-line in this study (s = 1, t = 1). Desirability of TC (y1), TF (y2), TB (y3), AC DPPH (y4) and AC FRAP (y5) was evaluated with Equation (7), where Li and Ui were set as the minimum and maximum responses in the experimental region predicted with Equation (1). The amount of peel powder and mucilage added to formulation was also controlled to achieve the highest level of bioactive compounds and antioxidant capacity without affecting other characteristics of product. This information was added to optimization problem by setting y6 = x1 and y7 = x2 and mapping these values into desirability functions with Equation (8). In this case, L6,7 = 0.1, M6,7 = 0.5 and U6,7 = 0.9. Finally, all responses (yi, i = 1, …, q, q = 7) were combined in overall desirability index defined in Equation (6) and formulation was optimized to maximize its value.
2.12. Gastrointestinal simulation
Gastrointestinal simulation was conducted following the methodology proposed by Saura-Calixto, García-Alonso, Goñi, & Bravo (Citation2000) with some modifications. Gastrointestinal simulation was divided into two steps, gastric and intestinal phase. For the phase one, 1 g of optimum yogurt blend was placed with 19 mL of HCl-KCl (0.5 M, pH = 1.5) buffer and 0.2 mL of pepsin (Merck, 7190) solution (30% w/v). Sample was incubated at 40°C for 1 h under constant agitation (INO650 M, Mexico). For intestinal simulation, 1 mL of the gastric digestion was taken and added with 19 mL of phosphates buffer (0.1 M, pH = 7.5) and 1 mL of pancreatin (Sigma P-1750) solution (0.05% w/v). Sample was then incubated at 37°C for 6 h under constant agitation. At the end of the gastrointestinal process, samples were analyzed for their antioxidant capacity and bioactive compounds.
2.13. Statistical analysis
Linear regression and optimization procedures were performed with the Matlab R2010a program (MathWorks Inc., Natick, MA, USA). Mean comparisons (Tukey’s test) and correlation analysis were conducted using with the Minitab 15 software (Minitab Inc., PA, USA, 2008).
3. Results and discussion
3.1. Bioactive compounds and antioxidant capacity of yogurt, cactus pear peel powder, and its mucilage
displays the bioactive compounds and antioxidant capacity of yogurt, peel and mucilage powders. As expected, yogurt showed a low amount of bioactive compounds and consequently a low antioxidant capacity; however, it had a higher content of total phenolic compounds and antioxidant capacity than those reported by Karaaslan, Ozden, Vardin, & Turkoglu (Citation2011) and Najgebauer-Lejko, Sady, Grega, & Walczycka (Citation2011), respectively; which may be attributed to the differences in the raw materials used in its preparing. On the other hand, mucilage powder presented both a higher amount of bioactive compounds and higher antioxidant capacity than the peel from which it was obtained. In this aspect, as mucilage is an aqueous extract obtained from peel powder, it could be that polarity of water increases the extraction of polar compounds with antioxidant capacity such as betalains, flavonoids and phenolic acids (Wybraniec et al., Citation2009; Yeddes, Chérif, Guyot, Sotin, & Ayadi, Citation2013). To date, no studies about the bioactive compounds and antioxidant capacity of mucilage obtained from cactus pear peel are reported, but cactus pear peel has been studied and the values of total betalains and antioxidant capacity obtained in this study are slightly higher than those reported by Aparicio-Fernández et al. (Citation2018), who reported values of 112.6 mg betanin/100 g, 420.9 mg GAE/100 g and 879.1 mg AA/100 g for total betalains, inhibition capacity and reducing power, respectively.
Table 2. Bioactive compounds of natural yogurt, red cactus pear peel powder, and its mucilagea.
Tabla 2. Compuestos bioactivos de yogurt natural, polvo de cáscara de tuna roja y mucílagoa.
3.2. Bioactive compounds, antioxidant capacity, and color of formulated yogurts
shows the results of bioactive compounds, antioxidant capacity, and color obtained from the nine yogurts formulations. At least, an increase of 81.2%, 100.1%, 825.0%, 263.0%, and 635.0% for total phenolic compounds, total flavonoids, total betalains, inhibition capacity, and reducing power, respectively was observed by the addition of cactus pear and/or mucilage to the formulation A (yogurt alone). The highest value of bioactive compounds and antioxidant capacity was obtained with the higher concentration of peel and mucilage powder (Formulation D). Until now, no information has been found in the literature about the use of cactus pear or its by-product added to yogurt; however, the results obtained are higher than those reported by the addition of extract or waste from other plant-based foods. In this regard, Marchiani et al. (Citation2016) informed an increase in 37.3–68.7% and 15–52% in the total phenolic compounds and antioxidant capacity, respectively, in yogurt added with grape pomace obtained from different cultivars. Similarly, Bertolino et al. (Citation2015) reported an increase in 74.9–90.8% and 161–202% for the same responses in yogurt added with 6% of hazelnut skin. On the other hand, Chouchouli et al. (Citation2013) evaluated the same antioxidant compounds that those evaluated in his study in yogurt added with grape seed extracts. They informed similar values of reducing power (223–311 mg AA/100 g); although total phenolic compounds and radical scavenging capacity were lower and higher, respectively, than the obtained in this study. Color parameters of formulated yogurts are presented in . It can be observed that the addition of cactus pear peel and/or mucilage significantly decreased (p < 0.05) the luminosity and increased the red color (a* color parameter) of the formulated yogurts, while b* color parameter did not show any tendency by the addition of the by-products. As the color of cactus pear peel and mucilage powder presented a deep red color, yogurts added with them had a pleasant magenta color with different intensities, thus hue and chroma values were increased by the addition of cactus pear peel and/or mucilage, while yogurt without powders shows a whitish color ().
Table 3. Bioactive compounds, antioxidant capacity and color parameters of yogurt added with cactus pear peel powder and its mucilagea.
Tabla 3. Compuestos bioactivos, capacidad antioxidante y parámetros de color de yogurt adicionado con polvo de cáscara de tuna y mucílagoa.
Figure 2. Hue angle and Chroma color parameters of yogurt added with red cactus pear peel and mucilage.
Figura 2. Parámetros de color de ángulo de tono y croma de yogurt adicionado con cáscara y mucílago de tuna roja.

Pearson´s correlation coefficients between bioactive compounds and antioxidant capacity are given in . The antioxidant capacity of yogurts evaluated by the two methodologies showed a higher correlation with total phenolic compounds (0.984–0.988) and total flavonoids (0.748–0.808); therefore, the antioxidant capacity of yogurts could associate to those compounds (p < 0.05), and less to total betalains, that shows a lower correlation, thus the antioxidant capacity may be associated to another group of phenolic compounds such as phenolic acids, tannins or coumarins (Luna-Guevara et al., Citation2019). On the other hand, a negative and positive high correlation (p < 0.05) were observed for total betalains and L* (−0.840) and a* (0.862) color parameters, respectively. This behavior may be attributed to the intense red color presented by the red cactus pear peel and mucilage, which increases the red color, but reduces the luminosity of the yogurts.
Table 4. Pearson´s correlation coefficient for bioactive compounds, antioxidant capacity and color parameters.
Tabla 4. Coeficiente de correlación de Pearson para compuestos bioactivos, capacidad antioxidante y parámetros de color.
3.3. Optimization process using constrained mixture design and its validation under experimental condition
shows the regression parameters and Pearson´s correlation coefficients of the second-order L-pseudocomponent model used in this study. As observed, proposed models achieved a satisfactory reproduction of experimental data (R2 > 0.76, ); therefore, they can be used for the optimization of yogurt formulation. The statistical analysis of model parameters revealed that all blend components contributed to the observed response (p < 0.05). Moreover, a significant binary synergism was observed between peel-mucilage, peel-yogurt and mucilage-yogurt for TPC and between mucilage-yogurt for TB (p < 0.05), that is, the observed response in those mixtures is higher than the expected from averaging contribution from single components. On the other hand, components antagonism was observed for peel-mucilage and mucilage-yogurt in the case of TF (p < 0.05). A visual representation of response behavior can be observed in -). In addition, Cox’s plots can be used to examine the effect of components on studied responses (). Cox’s plots show the effect of both adding and removing a certain component to a reference mixture (the centroid in this case, blend I), while the others are kept unaffected. It can be observed that TPC and AC evaluated by FRAP and DPPH methods have a similar response behavior (,,)), a fact previously confirmed by its high correlation (>0.90), reaching a maximum response when peel and mucilage are in their highest levels. Moreover, Cox’s plots for these responses exhibit the same behavior, where TPC and AC (DPPH and FRAP methods) of the reference blend are increased with higher levels of peel and mucilage substitution and decrease when yogurt proportion is increased (,,)). According to ), the TF increases by adding mucilage to the mixture, a fact also observed in Cox’s plot ()). Here, the addition of peel powder to reference blend also causes an increase of TF, but it is much lower than the caused by adding mucilage to the blend. Finally, the analysis of TB in Cox’s plot ()) revealed a complex behavior. Adding peel powder to reference formulation increases TB; however, addition of mucilage powder causes the increase of TB only up to a certain limit, beyond which response begins to decrease.
Table 5. Regression analysis of constrained mixture design for the formulation of yogurt added with peel and mucilage powder of red cactus peara.
Tabla 5. Análisis de regresión del diseño de mezclas restringido para la formulación de yogurt adicionado con polvo de cáscara y mucílago de tuna rojaa.
Figure 3. Fitness quality of second-order mixture models.
Figura 3. Calidad de ajuste de los modelos de mezcla de segundo orden.

Figure 4. Contour plots for studied responses in constrained factors space. Length of triangle edges corresponds to a composition change of 0.01. Arrows indicate the direction in which x1 and x2 are increased.
Figura 4. Gráficos de contorno para las respuestas estudiadas en el espacio de factores restringido. La longitud de los lados de los triángulos corresponde a un cambio de composición de 0.01. Las flechas indican la dirección en la que se incrementan x1 y x2.

Figure 5. Plots of the estimated responses along the xi (i = 1,2,3) rays (refer to to see Cox’s directions).
Figura 5. Gráficos de las respuestas estimadas a lo largo de los rayos de xi (i = 1,2,3). (consulte la para ver las direcciones de Cox).

The desirability function approach was applied to optimize the formulation. The lower and upper limits for desirability function of TPC, TF, TB, and AC (DPPH and FRAP methods), as evaluated with fitted models within the constrained factor space, are shown in . The mapping of studied response into an overall desirability produced the behavior shown in ). The maximum desirability was obtained for a yogurt formulated with 5.5% of cactus pear peel and 7.5% of mucilage (D = 0.663) was the best combination to get the desired responses. This formulation was experimentally evaluated and the results are shown in . As observed, a good agreement was found between predicted and experimental responses in optimized formulation. It is well known that bioactive compounds and antioxidant capacity are always evaluated by in vitro assays; however, further analysis should be done to elucidate the metabolism of bioactive compounds in human body. Therefore, optimal yogurt was submitted to in vitro digestion process, resulting in 1224.3%, 246.3%, 641.9%, 131.4%, and 429.4% increments of bioactive compounds and antioxidant capacity after the gastrointestinal simulation for total phenolic compounds, total flavonoids, total betalains, and inhibition capacity and reducing power, respectively. The increase in the bioactive compounds and antioxidant capacity after the gastrointestinal simulation may be due to the action of gastrointestinal enzymes (pepsin and pancreatin) and the acid conditions in the gastric phase. In this regard, phenolic compounds (including flavonoids) and betalains are usually linked to carbohydrates, proteins and cell wall structural components by covalent bonds, hydrogen bonding, and other interactions, this linkage may be disrupted due to the condition mentioned before releasing the bioactive compounds (Lingua, Wunderlin, & Baroni, Citation2018; Pacheco-Ordaz, Antunes-Ricardo, Gutiérrez-Uribe, & González-Aguilar, Citation2018). Then, the hydrolysis of bioactive compounds may produce aglycones which are more active than glycoside structures (Pacheco-Ordaz et al., Citation2018; Xiao, Citation2017).
Table 6. Predicted, experimental and gastrointestinal simulation responses of bioactive compounds and antioxidant capacity of optimum yogurt blenda.
Tabla 6. Respuesta predicha, experimental y de simulación gastrointestinal de los compuestos bioactivos y capacidad antioxidante de la mezcla óptima de yogurta.
4. Conclusions
Cactus pear peel and its mucilage were successfully incorporated into natural yogurt to give it a high amount of bioactive compound, antioxidant capacity and an attractive range of magenta color. Desirability function approach was successfully applied to formulate a yogurt with the highest bioactive compounds and antioxidant capacity, but with a reduced peel and mucilage substitution. Yogurt submitted to in vitro gastrointestinal process showed higher values of the bioactive compounds and antioxidant capacity than non-digested yogurt. Although further studies should be conducted, the results obtained in this study may be of great useful for the fortification of yogurt using cactus pear by-products.
Conflict of interest
The authors declare that they have no conflict of interest in the publication.
Acknowledgments
The author Paola Hernández-Carranza would like to thank to “Programa para el Desarrollo Profesional Docente” for the financial support of this work, project number (PRODEP 511-6/17-8017) and “Vicerrectoria de Investigación y Estudios de Posgrado” project number (100517615-VIEP 2019).
Additional information
Funding
References
- Aparicio-Fernández, X., Vega-Ahuatzin, A., Ochoa-Velasco, C. E., Cid-Pérez, S., Hernández-Carranza, P., & Ávila-Sosa, R. (2018). Physical and antioxidant characterization of edible films added with red prickly pear (Opuntia ficus-indica L.) cv. San Martín peel and/or its aqueous extracts. Food Bioprocess Technology, 11, 368–379. doi:10.1007/s11947-017-2017-x
- Asioli, D., Aschemann-Witzel, J., Caputo, V., Vecchio, R., Annunziata, A., Næs, T., & Varela, P. (2017). Making sense of the “clean label” trends: A review of consumer food choice behavior and discussion of industry implications. Food Research International, 99, 58–71. doi:10.1016/j.foodres.2017.07.022
- Ayala-Zavala, J. F., Vega-Vega, V., Rosas-Domínguez, C., Palafox-Carlos, H., Villa-Rodríguez, J. A., Siddiqui, W., Md., … González-Aguilar, G. A. (2011). Review Agro-industrial potential of exotic fruit byproducts as a source of food additives. Food Research International, 44, 1866–1874. doi:10.1016/j.foodres.2011.02.021
- Berto, A., Braga, R. A., Evelázio, D. N., Fernandes, E., & Campos, C. R. (2015). Bioactive compounds and scavenging capacity of pulp, peel and seed extracts of the Amazonian fruit Quararibea cordata against ROS and RNS. Food Research International, 77, 236–243. doi:10.1016/j.foodres.2015.06.018
- Bertolino, M., Belviso, S., Dal Bello, B., Ghirardello, D., Giordano, M., Rolle, L., … Zeppa, G. (2015). Influence of the addition of different hazelnut skins on the physicochemical, antioxidant, polyphenol and sensory properties of yogurt. LWT – Food Science and Technology, 63, 1145–1154. doi:10.1016/j.lwt.2015.03.113
- Chouchouli, V., Kalogeropoulos, N., Konteles, S. J., Karvela, E., Makris, D. P., & Karathanos, V. T. (2013). Fortification of yogurts with grape (Vitis vinifera) seed extracts. LWT – Food Science and Technology, 53, 522–529. doi:10.1016/j.lwt.2013.03.008
- Cornell, J. (2002). Experiments with mixtures. Designs, models, and the analysis of mixture data (3 rd ed.). New York, USA: Wiley-Interscience.
- Dabija, A., Codină, G. G., Ropciuc, S., Gâtlan, A. M., & Rusu, L. (2018). Assessment of the antioxidant activity and quality attributes of yogurt enhanced with wild herbs extracts. Journal of Food Quality, 2018, 1–12. doi:10.1155/2018/5329386
- Dorman, H. J., Kosar, M., Kahlos, K., Holm, Y., & Hiltunen, R. (2003). Antioxidant properties and composition of aqueous extracts from Mentha species, hybrids, varieties, and cultivars. Journal of Agricultural and Food Chemistry, 51(16), 4563–4569. doi:10.1021/jf034108k
- Elleuch, M., Bedigian, D., Roiseux, O., Besbes, S., Blecker, C., & Attia, H. (2011). Dietary fibre and fibre-rich by-products of food processing: Characterisation, technological functionality and commercial applications: A review. Food Chemistry, 124, 411–421. doi:10.1016/j.foodchem.2010.06.077
- El-Said, M. M., Haggag, H. F., Fakhr, E. H. M., Gad, A. S., & Farahat, A. Z. (2014). Antioxidant activities and physical properties of stirred yoghurt fortified with pomegranate peel extracts. Annals of Agricultural Science, 59(2), 207–212. doi:10.1016/j.aoas.2014.11.007
- Ersöz, E., Kınık, Ö., Yerlikaya, O., & Açu, M. (2011). Effect of phenolic compounds on characteristics of strained yoghurts produced from sheep milk. African Journal of Agricultural Research, 6(23), 5351–5359.
- Fazilah, N. F., Ariff, A. B., Khayat, M. E., Rios-Solis, L., & Halim, M. (2018). Influence of probiotics, prebiotics, symbiotic and bioactive phytochemicals on the formulation of functional yogurt. Journal of Functional Foods, 48, 387–399. doi:10.1016/j.jff.2018.07.039
- García-Pérez, F. J., Lario, Y., Fernández-López, J., Sayas, E., Pérez-Alvarez, J. A., & Sendra, E. (2005). Effect of orange fiber addition on yogurt color during fermentation and cold storage. Industrial Applications, 30(6), 457–463.
- Gengatharan, A., Dykes, G. A., & Choo, W. S. (2015). Betalains: Natural plant pigments with potential application in functional foods. LWT - Food Science and Technology, 64, 645–649. doi:10.1016/j.lwt.2015.06.052
- German, J. B. (2014). The future of yogurt: Scientific and regulatory needs. The American Journal of Clinical Nutrition, 99, 1271S–1278S. doi:10.3945/ajcn.113.076844
- Guarner, F., Perdigon, G., Corthier, G., Salminen, S., Koletzko, B., & Morelli, L. (2005). Should yoghurt cultures be considered probiotic? British Journal of Nutrition, 93, 783–786. doi:10.1079/bjn20051428
- Hashemi, G. H., Hadi, E. M., Mesbahi, G., & Amin, H. M. (2015). Scientific and technical aspects of yogurt fortification: A review. Food Science and Human Wellness, 4, 1–8. doi:10.1016/j.fshw.2015.03.002
- Hashim, I. B., Khalil, A. H., & Afifi, H. S. (2009). Quality characteristics and consumer acceptance of yogurt fortified with date fiber. Journal of Dairy Science, 92(11), 5403–5407. doi:10.3168/jds.2009-2234
- Helkar, P. B., Sahoo, A. K., & Patil, N. J. (2016). Review: Food industry by-products used as a functional food ingredients. International Journal of Waste Resources, 6, 248.
- Hernández-Carranza, P., Ávila-Sosa, R., Guerrero-Beltrán, J. A., Navarro-Cruz, A. R., Corona-Jiménez, E., & Ochoa-Velasco, C. E. (2016). Optimization of antioxidant compounds extraction from fruit by-products: Apple pomace, orange and banana peel. Journal of Food Processing and Preservation, 40, 103–115. doi:10.1111/jfpp.2016.40.issue-1
- Hernández-Carranza, P., Rivadeneyra-Mata, M., Ramos-Cassellis, M. E., Aparicio-Fernández, X., Navarro-Cruz, A. R., Ávila-Sosa, R., & Ochoa-Velasco, C. E. (2019). Characterization of red prickly pear peel (Opuntia ficus-indica L.) and its mucilage obtained by traditional and novel methodologies. Journal of Food Measurement and Characterization, 13, 1111–1119. doi:10.1007/s11694-018-00026-y
- Iriondo-DeHond, M., Miguel, E., & Del Castillo, M. D. (2018). Food byproducts as sustainable ingredients for innovative and healthy dairy foods. Nutrients, 10, 1–24. doi:10.3390/nu10101358
- Karaaslan, M., Ozden, M., Vardin, H., & Turkoglu, H. (2011). Phenolic fortification of yogurt using grape and callus extracts. LWT – Food Science and Technology, 44, 1065–1072. doi:10.1016/j.lwt.2010.12.009
- Lingua, M. S., Wunderlin, D. A., & Baroni, M. V. (2018). Effect of simulated digestion on the phenolic components of red grapes and their corresponding wines. Journal of Functional Foods, 44, 86–94. doi:10.1016/j.jff.2018.02.034
- Luna-Guevara, M. L., Luna-Guevara, J. J., Hernández-Carranza, P., Ruíz-Espinosa, E., & Ochoa-Velasco, C. E. (2019). Phenolic compounds: A good choice against chronic degenerative diseases. In F. R. S. Atta-ur-Rahman (Ed.), Studies in natural products chemistry (pp. 79–108). Karachi, Pakistan: ELSEVIER.
- Marchiani, R., Bertolino, M., Belviso, S., Giordano, M., Ghirardello, D., Torri, L., … Zeppa, G. (2016). Yogurt enrichment with grape pomace: Effect of grape cultivar on physicochemical, microbiological and sensory properties. Food Quality, 39, 77–89. doi:10.1111/jfq.12181
- Najgebauer-Lejko, D., Sady, M., Grega, T., & Walczycka, M. (2011). The impact of tea supplementation on microflora, pH and antioxidant capacity of yoghurt. International Dairy Journal, 21, 568–574. doi:10.1016/j.idairyj.2011.03.003
- Ochoa-Velasco, C. E., & Guerrero-Beltrán, J. A. (2012). Efecto del almacenamiento a diferentes temperaturas sobre la calidad de tuna roja (Opuntia ficus indica (L.) Miller). Información Tecnológica, 23(1), 117–128. doi:10.4067/S0718-07642012000100013
- Ochoa-Velasco, C. E., & Guerrero-Beltrán, J. A. (2014). Postharvest quality of peeled prickly pear fruit treated with acetic acid and chitosan. Postharvest Biology and Technology, 92, 139–145. doi:10.1016/j.postharvbio.2014.01.023
- Ochoa-Velasco, C. E., & Guerrero-Beltrán, J. A. (2016). The effects of modified atmospheres on prickly pear (Opuntia albicarpa) stored at different temperatures. Postharvest Biology and Technology, 111, 314–321. doi:10.1016/j.postharvbio.2015.09.028
- Otálora, M. C., Carriazo, J. G., Iturriaga, L., Nazareno, M. A., & Osorio, C. (2015). Microencapsulation of betalains obtained from cactus fruit (Opuntia ficus-indica) by spray drying using cactus cladode mucilage and maltodextrin as encapsulating agents. Food Chemistry, 187, 174–181. doi:10.1016/j.foodchem.2015.04.090
- Pacheco-Ordaz, R., Antunes-Ricardo, M., Gutiérrez-Uribe, J. A., & González-Aguilar, G. A. (2018). Intestinal permeability and cellular antioxidant activity of phenolic compounds from mango (Mangifera indica cv. Ataulfo) peels. International Journal of Molecular Sciences, 19, 514. doi:10.3390/ijms19020514
- Palafox-Carlos, H., Ayala-Zavala, J. F., & González-Aguilar, G. A. (2011). The role of dietary fiber in the bioaccessibility and bioavailability of fruit and vegetable antioxidants. Journal of Food Science, 76, R6–R15. doi:10.1111/j.1750-3841.2010.01957.x
- Sagar, N. A., Pareek, S., Sharma, S., Yahia, E. M., & Lobo, M. G. (2018). Fruit and vegetable waste: Bioactive compounds, their extraction, and possible utilization. Comprehensive Reviews in Food Science and Food Safety, 17, 512–531. doi:10.1111/crf3.2018.17.issue-3
- Sah, B. N. P., Vasiljevic, T., McKechnie, S., & Donkor, O. N. (2016). Effect of pineapple waste powder on probiotic growth, antioxidant and antimutagenic activities of yogurt. Journal of Food Science and Technology, 53(3), 1698–1708. doi:10.1007/s13197-015-2100-0
- Saura-Calixto, F., García-Alonso, A., Goñi, I., & Bravo, L. (2000). In vitro determination of the indigestible fraction in foods: An alternative to dietary fiber analysis. Journal of Agricultural and Food Chemistry, 48, 3342–3347. doi:10.1021/jf0000373
- Sharma, S. K., Bansal, S., Mangal, M., Kumar, D. A., Gupta, R. K., & Mangal, A. K. (2016). Utilization of food processing by-products as dietary, functional, and novel fiber: A review. Critical Reviews in Food Science and Nutrition, 56, 1647–1661. doi:10.1080/10408398.2013.794327
- Stagos, D., Amoutzias, G. D., Matakos, A., Spyrou, A., Tsatsakis, A. M., & Kouretas, D. (2012). Chemoprevention of liver cancer by plant polyphenols. Food and Chemical Toxicology, 50, 2155–2170. doi:10.1016/j.fct.2012.04.002
- Stintzing, F. C., Herbach, H. M., & Mosshammer, M. R. (2005). Color, betalain pattern, and antioxidant properties of cactus pear (Opuntia spp.) clones. Journal and Agricultural Food Chemistry, 26(2), 442–451. doi:10.1021/jf048751y
- Tapsell, L. C., Neale, E. P., Satija, A., & Hu, F. B. (2016). Foods, nutrients, and dietary patterns: Interconnections and implications for dietary guidelines. Advances in Nutrition, 7(3), 445–454. doi:10.3945/an.115.011718
- Tresserra-Rimbau, A., Rimm, E. B., Medina-Remón, A., Martínez-González, M. A., López-Sabater, M. C., Covas, M. I., … Lamuela-Raventós, R. M. (2014). Polyphenol intake and mortality risk: A re-analysis of the PREDIUM trial. BMC Medicine, 12(77), 1–11. doi:10.1186/1741-7015-12-77
- Viva de Toledo, N. M., Costa de Camargo, A., Bortolotto, M. R. P., Charles, B. D., Granato, D., & Canniatti-Brazaca, S. G. (2018). Potentials and pitfalls on the use of passion fruit by-products in drinkable yogurt: Physicochemical, technological, microbiological, and sensory aspects. Beverages, 4(47), 1–15.
- Wybraniec, S., Stalica, P., Jerz, G., Klose, B., Gebers, N., Winterhalter, P., … Mizrahi, Y. (2009). Separation of polar betalain pigments from cacti fruits of Hylocereus polyrhizus by ion-pair high-speed countercurrent chromatography. Journal of Chromatography A, 1216, 6890–6899. doi:10.1016/j.chroma.2009.08.035
- Xiao, J. (2017). Dietary flavonoid aglycones and their glycosides: Which show better biological significance? Critical Reviews in Food Science and Nutrition, 57(9), 1874–1905. doi:10.1080/10408398.2015.1032400
- Yeddes, N., Chérif, J. K., Guyot, S., Sotin, H., & Ayadi, M. T. (2013). Comparative study of antioxidant power, polyphenols, flavonoids and betacyanins of the peel and pulp of three tunisian Opuntia forms. Antioxidants, 2, 37–51. doi:10.3390/antiox2020037