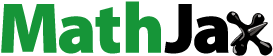
ABSTRACT
Herein, a QuEChERS (quick, easy, cheap, effective, rugged, and safe) procedure together with liquid chromatography and tandem mass spectrometry was applied to the multi-residue analysis of 203 pesticides in strawberries. To reduce the amount of co-eluents, we compared several dispersive solid-phase extraction cleanup procedures and found that cleanup using octadecylsilane provided the best recoveries. Spiked-sample recoveries of 70–120% with relative standard deviations of <20% were obtained for all tested pesticides except for quinmerac. Matrix-matched calibration curves exhibited good linearities (R2 > 0.99), and the limits of quantification (LOQs) were lower than the maximum residue limits recommended by the European Commission and Korea Food and Drug Administration. The developed method was applied to real samples, revealing that their pesticide contents exceeded the corresponding LOQs. The obtained results demonstrate that octadecylsilane-based dispersive solid-phase extraction is a rapid and high-throughput cleanup procedure for the multi-residue analysis of pesticides in strawberries.
RESUMEN
El presente estudio aplicó un procedimiento QuEChERS [acrónimo en inglés de rápido, fácil, barato, efectivo, resistente y seguro] junto con cromatografía líquida y espectrometría de masas en tándem para analizar residuos múltiples de 203 pesticidas detectados en fresas. Con el propósito de reducir la cantidad de coeluyentes, se compararon varios procedimientos de limpieza de extracción en fase sólida dispersiva, constatándose que la limpieza con octadecilsilano proporcionó las mejores recuperaciones. Así, se obtuvieron recuperaciones de muestras con picos de 70-120% y desviaciones estándar relativas <20% para todos los pesticidas analizados, excepto quinmerac. Las curvas de calibración de matriz coincidente exhibieron buenas linealidades (R2 > 0.99), mientras que los límites de cuantificación (LOQ) fueron inferiores a los límites máximos de residuos (LMR) recomendados por la Comisión Europea y la Administración de Alimentos y Medicamentos de Corea. El método desarrollado se aplicó a muestras reales, revelando que los contenidos de pesticidas de éstas excedían los LOQ correspondientes. Los resultados obtenidos demuestran que la extracción dispersiva en fase sólida basada en octadecilsilano es un procedimiento de limpieza rápido y de alto rendimiento para analizar residuos múltiples de pesticidas en fresas.
1. Introduction
The high nutritional value of fruits makes them important food components (Ruiz-Torralba, Guerra-Hernández, & García-Villanova, Citation2018); however, fruits can also be dietary sources of toxic substances such as pesticides. For example, pesticide residues are frequently detected in strawberries, which are very popular agricultural raw materials (Sharma, Nagpal, Pakade, & Katnoria, Citation2010). The increasing public concern over the potential health risks posed by pesticide residues in food has strengthened the management of agricultural products for improved food quality and safety. To ensure food-supply safety, maximum residue limits (MRLs, expressed in mg kg−1) have been established to regulate the use of pesticides (European Commission, Citation2019; KFDA, Citation2019). In Korea, MRLs have been set for most pesticides in a variety of animal-origin and agricultural products, and a uniform value of <0.01 mg kg−1 has been set for those for which no specific MRLs exist. To monitor MRL compliance in food commodities, multi-residue analysis methods have been developed and validated (Yang, Luo, Duan, Li, & Liu, Citation2018). However, the optimization of sample preparation and chromatographic conditions for multi-residue analysis remains challenging due to the large variation of analyte chemical and physical properties.
Various instrumental techniques, including gas chromatography (GC) with flame ionization or electron capture detection, liquid chromatography (LC) with UV, evaporative light scattering, or fluorescence detection, and GC-MS or MS/MS, have been used to sensitively detect multi-residues (Farha et al., Citation2018; Guo et al., Citation2018; Lee et al., Citation2016). Above all, LC coupled with tandem mass spectrometry (LC-MS/MS) and electrospray ionization (ESI) operating in multiple reaction monitoring (MRM) mode has proven to be the most powerful analytical technique for the quantification of pesticides and has been widely used for their multi-residue analysis owing to its high sensitivity and selectivity (Lee et al., Citation2016). High-resolution mass spectrometry techniques such as quadrupole time-of-flight (QTOF) MS are advantageous, allowing quantification and identification to be accomplished in a single run without the need for compound-specific optimization (Muehlwald, Buchner, & Kroh, Citation2018). Although the main drawback of the MRM technique is blinded non-targeted contaminants in food commodities, LC-MS/MS with MRM is quantitatively more sensitive and selective than full-scan TOF/MS.
Even though LC-MS/MS with MRM provides high sensitivity and selectivity, sample preparation is crucial for the accurate detection of multiple pesticide residues (Hamdy Abdelwahed, Khorshid, El-Marsafy, & Souaya, Citation2019). The multi-residue analysis of pesticides in real samples is considered to be a difficult task because of the high complexity and diversity of sample matrices. Strawberries contain some troublesome pesticide-analysis co-eluents such as pigments, polyphenols, and sugars (Forbes-Hernandez et al., Citation2016). Sample preparation using the QuEChERS (quick, easy, cheap, effective, rugged, and safe) method involves simple extraction and cleanup procedures. The original QuEChERS method introduced by Anastassiades, Lehotay, Štajnbaher, and Schenck (Citation2003) has been modified to be more suitable for the analysis of some pesticides, including those with planar structures, different polarities, and pH-dependent characteristics. The dispersive solid-phase extraction (d-SPE) technique was developed simultaneously with the QuEChERS method to minimize interference (Song et al., Citation2019). In d-SPE, a primary secondary amine (PSA) sorbent removes polar interferents (organic acids and sugars), while octadecylsilane (C18) is used to remove non-polar co-eluents such as lipids, and graphitized carbon black (GCB) is used to remove pigmented matrices such as chlorophyll and carotenoids. Various sorbent types have been evaluated to improve cleanup and recovery in the QuEChERS method. Oshita and Jardim (Citation2014) reported that PSA minimizes interference and presents the best cleanup during the determination of some pesticide residues in strawberries. Koesukwiwat, Lehotay, Miao, and Leepipatpiboon (Citation2010) determined 126 pesticides in strawberries employing QuEChERS (acetate buffered version) extraction combined with PSA, C18, and GCB cleanup, and achieving satisfactory recoveries. Among the ~500 pesticides for which MRLs are set in Korea for animal-origin and agricultural products, more than 150 are LC-amenable. Hence, sensitive detection methods and effective cleanup procedures are required to monitor a wide range of pesticide residues.
Herein, we introduce a method based on LC-MS/MS and QuEChERS with acetate-buffered acetonitrile for the simultaneous determination of 203 LC-amenable pesticides in strawberries, comparing different d-SPE cleanup techniques to improve cleanup efficiency for the tested pesticides. LC-MS/MS combined with the selected QuEChERS method was validated and used to determine the levels of pesticides in real strawberry samples.
2. Materials and methods
2.1. Chemicals and reagents
Standard pesticides (>95%) listed in were purchased from AccuStandard (New Haven, CT, USA). A QuEChERS AOAC extraction kit (P/N 5982–7755), QuEChERS d-SPE kits for general fruits and vegetables (P/N 5982–5022), fatty samples (P/N 5982–5122), pigments (P/N 5982–5222), pigments and fats (P/N 5982–5421), and other foods (P/N 5982–4956), as well as the end-capped C18 adsorbent were purchased from Agilent Technologies (Santa Clara, CA, USA). Ammonium acetate (>99.0%), triphenylphosphate, formic acid, and MgSO4 were acquired from Sigma-Aldrich (St. Louis, MO, USA). All solvents were of analytical or HPLC grade. Standard stock solutions (100 g mL−1 each) were prepared in acetonitrile and diluted with the same to afford standard mixed solutions. Stock and working solutions were stored at −20°C until required for analysis.
Table 1. Pesticides with low recoveries when using cleanup 1–4 at 0.1 mg kg−1 fortification level.
Tabla 1. Pesticidas que mostraron bajas recuperaciones al utilizarse las limpiezas 1–4 a un nivel de fortificación de 0.1 mg kg−1.
2.2. Sample preparation
The AOAC Official Method 2007.01 (AOAC, Citation2007) was followed for extraction. Extraction was performed using an AOAC extraction kit containing 6 g MgSO4 and 1.5 g sodium acetate. Each sample (15 g) was placed in a 50-mL centrifuge tube to which 1% acetic acid in acetonitrile (1%, 15 mL) was added as the extraction solvent. Triphenylphosphate was spiked directly into the centrifuge tube as the internal standard to a concentration of 1 µg mL−1. The centrifuge tube was shaken for 1 min, charged with the QuEChERS AOAC extraction kit, shaken vigorously for 10 min, and centrifuged at 4,000 g for 10 min at 4°C. Various d-SPE cleanup methods were then applied to the extracted analytes.
Five d-SPE cleanup methods were used: Cleanup 1 (50 mg PSA and 150 mg MgSO4), Cleanup 2 (50 mg C18, 50 mg PSA, and 150 mg MgSO4), Cleanup 3 (50 mg GCB, 50 mg PSA, and 150 mg MgSO4), Cleanup 4 (50 mg C18, 50 mg GCB, 50 mg PSA, and 150 mg MgSO4), and Cleanup 5 (50 mg C18 and 150 mg MgSO4). Each tube was strongly vortexed for 1 min and then centrifuged at 12,000 rpm for 5 min at 4°C. The extract was filtered through a syringe with a 0.22-μm nylon membrane filter and transferred into a vial for analysis by LC-MS/MS.
2.3. LC-MS/MS
An Agilent LC 1200 HPLC system (Agilent Technologies, Santa Clara, CA, USA) coupled to a 4000 QTRAP mass spectrometer equipped with a turbo ion-spray ionization source (AB SCIEX, Foster City, CA, USA) was used to analyze pesticides. An Agilent Zorbax Eclipse Plus C18 column (2.1 × 100 mm, 1.8 μm; Santa Clara, CA, USA) was used for chromatographic separation. The above separation was carried out with a binary mobile phase containing (A) 5 mM ammonium acetate and 0.1 vol% formic acid in water, and (B) 5 mM ammonium acetate and 0.1 vol% formic acid in methanol. A linear binary mobile phase gradient was used: 95% A at 0 min, 60% A at 1.5 min, 40% A at 2 min, 30% A at 3.5 min, 20% A at 15 min, 0% A from 16 to 22.5 min, and 95% A from 23 to 27 min. The flow rate, column temperature, and injection volume equaled 0.2 mL min−1, 40°C, and 2 μL, respectively. The pesticides were ionized in positive ESI mode and determined using scheduled MRM with the following general settings: curtain gas pressure = 30 psi, ion-source gas (1) pressure = 50 psi, ion-source gas (2) pressure = 55 psi, source temperature = 400°C, ion spray voltage = 5500 V. The MRM transitions, retention times, collision energies, and declustering potentials of analytes are summarized in the Appendix.
2.4. Validation study and matrix effects
The acceptability of the developed method for the analysis of target pesticides was validated following the European Commission SANTE/11813/2017 (European Commission, Citation2017) and ICH/2005/Q2/R1 (ICH, Citation2005) protocols. Linearities were determined using matrix-matched calibration curves with spiked blank samples at five concentrations (0.005, 0.01, 0.02, 0.05, and 0.1 mg kg−1). All coefficients of determination (R2 > 0.99) were acceptable.
Recoveries (%) and precisions, in terms of repeatability and reproducibility, were determined by analysis of blank samples spiked with standard solutions at three concentrations (0.01, 0.05, and 0.1 mg kg−1), with recoveries calculated using Equation (1). Repeatabilities and reproducibilities were determined based on the results of at least six replicate analyses performed on the same day and on different days, respectively. Precision was expressed as the relative standard deviation (RSD%) of replicate analyses.
Limits of detection (LODs) and limits of quantification (LOQs) were determined using five independently spiked pesticide concentrations (0.005, 0.01, 0.02, 0.05, and 0.1 mg kg−1) and were calculated based on the standard deviations of response and slope as LOD = 3.3 σ/s, LOQ = 10 σ/s, where σ is the standard deviation of the response, and s is the slope of the matrix-matched calibration curve.
To compensate for matrix effects (%MEs), the slopes of pesticide standards in solvent and in extracts were compared, with Equation (2) used to calculate %ME:
3. Results and discussion
3.1. Optimization of LC-MS/MS conditions
Optimization of mobile phase composition is important for the analysis of trace amounts of analytes by LC-MS, as it leads to satisfactory sensitivity and good ionization. Herein, ammonium acetate and formic acid were used as mobile phase additives to improve ionization efficiency, peak intensities, and target analyte separation (Berlioz-Barbier et al., Citation2014). Ammonium ions suppress the formation of sodium adducts under acidic conditions, increasing sensitivities and response consistency for some pesticides by facilitating the formation of [M + H]+ and [M+ NH4]+ ions (Hiemstra & de Kok, Citation2007). When water (containing 0.1 vol% formic acid and 5 mM ammonium acetate) and methanol (containing 0.1 vol% formic acid) were used as the mobile phases, analyte peaks eluted separately and featured good shapes (). A slightly longer run time (a total of 27 min) was needed when the Eclipse Plus C18 RRHD (2.1 × 100 mm, 1.8 µm) column was used; however, this column provided satisfactory separation and resolution (), and all analytes were eluted between 5 and 21 min ().
Figure 1. MRM chromatograms of 203 pesticides under the optimized LC-MS/MS conditions.
Figura 1. Cromatogramas MRM de 203 pesticidas en condiciones optimizadas de LC-MS/MS.
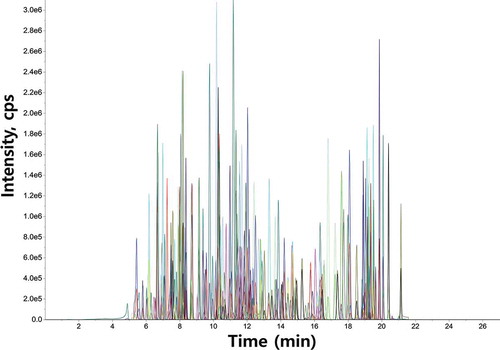
The MS operating conditions were optimized for maximum sensitivity to identify and quantify the target analytes. As shown in , the MS/MS parameters, including declustering potential and collision energy, were optimized through the direct infusion of each analyte with a syringe pump at a flow rate of 10 µL min−1. Two transitions were chosen for each pesticide to avoid false negatives in the MRM mode, and the most intense product ion was chosen as the quantifier ion.
3.2. Cleanup procedure
When Cleanup 3 (PSA and GCB) and Cleanup 4 (PSA, GCB, and C18) were used, 84 and 103 out of 203 pesticides, respectively, showed recoveries lower than those observed for Cleanup 1 (PSA alone) (). Although GCB is commonly applied to remove compounds with planar structures, such as chlorophyll and carotenoids, it absorbs and retains numerous planar pesticides (Lehotay et al., Citation2010; Nguyen et al., Citation2008). The low recoveries (>70%) observed for planar pesticides such as pyrimethanil, terbufos, and thiabendazole () were ascribed to the use of GCB (Kaczyński & Łozowicka, Citation2017; Zhang, Wang, Li, & Wang, Citation2017) ().
Figure 2. LC-MS/MS recoveries for various clean-up procedures at a spiking level of 0.1 mg kg−1. Cleanup 1 (50 mg PSA, and 150 mg MgSO4), Cleanup 2 (50 mg C18, 50 mg PSA, and 150 mg MgSO4), Cleanup 3 (50 mg GCB, 50 mg PSA, and 150 mg MgSO4), Cleanup 4 (50 mg C18, 50 mg GCB, 50 mg PSA, and 150 mg MgSO4), and Cleanup 5 (50 mg C18 and 150 mg MgSO4).
Figura 2. Recuperaciones de LC-MS/MS para diversos procedimientos de limpieza a un nivel de pico de 0.1 mg kg−1. Limpieza 1 (50 mg de PSA y 150 mg de MgSO4), limpieza 2 (50 mg de C18, 50 mg de PSA y 150 mg de MgSO4), limpieza 3 (50 mg de GCB, 50 mg de PSA y 150 mg de MgSO4), limpieza 4 (50 mg de C18, 50 mg de GCB, 50 mg de PSA y 150 mg de MgSO4), y limpieza 5 (50 mg de C18 y 150 mg de MgSO4).
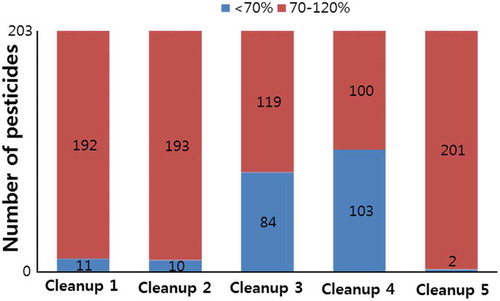
Eleven pesticides showed recoveries of less than 70% when fortified at 0.1 mg kg−1 using d-SPE with Cleanups 1–4 (). Nine sulfonylurea herbicides and one quinolinecarboxylic acid herbicide showed low recoveries in the 3.0–69.2% range with Cleanups 1–4 using GCB and/or PSA. The original QuEChERS method, employing PSA as the sorbent, showed weak recoveries (<70%) for sulfonylurea herbicides (Kaczyński & Łozowicka, Citation2017), which was ascribed to the known ability of PSA and GCB to absorb some weakly acidic herbicides such as sulfonylureas (Kaczyński & Łozowicka, Citation2017; Liu et al., Citation2015). A modified QuEChERS method employing C18 cleanup was introduced by Lee et al. (Citation2016) to overcome this issue; consistent with their results, the best recoveries for sulfonylurea herbicides in strawberries were observed for Cleanup 5 with C18 (). This outcome was rationalized by the fact that the C18 sorbent has a lower affinity for acidic herbicides than PSA or GCB and features excellent performance during extract purification (Liu et al., Citation2015). The combination of C18 and PSA (Cleanup 2) resulted in slightly higher recoveries of sulfonylurea herbicides, which, however, remained below 70%. Carbendazim exhibited poor recovery when PSA (Cleanup 1) or a combination of PSA and GCB (Cleanups 3 and 4) was used. Guan, Tang, Chen, Xu, and Li (Citation2013) ascribed the low recovery of carbendazim to the high amount of absorbent (i.e. GCB) strongly absorbing non-polar compounds. As a result, with the exception of quinmerac, 10 pesticides with low recoveries in showed better recoveries (74.6–95.5%) when Cleanup 5 (with C18 alone) was used. Consequently, cleanup with C18 was selected for the multi-residue analysis of pesticides in strawberries because of its high extraction efficiency. Quinmerac, which is a pH-dependent acidic compound (pKa = 4.31 at 25°C), was out of the recovery criterion range in the cases of all five cleanup methods.
3.3. Method validation and matrix effects
To evaluate the extraction and d-SPE cleanup procedure based on the use of C18, we validated our method in terms of linearity, accuracy, precision, LOD, and LOQ following the European Commission SANTE/11813/2017 guidelines (European Commission, Citation2017) and the ICH/2005/Q2/R1 (ICH, Citation2005) protocol.
Calibration curves for 203 pesticides were constructed by the matrix-matching method at concentrations of 0.005, 0.01, 0.02, 0.05, and 0.1 mg kg−1 in blank sample extracts. As shown in , all matrix-matched calibration curves were linear, with coefficients of determination (R2) exceeding 0.995 for all tested pesticides. According to the SANTE/11813/2017 guideline, average recoveries should be within 70–120%, with RSDs of ≤ 20% (European Commission, Citation2017). We found that 202 pesticides exhibited recoveries of 71–100% and 75–102% at fortification levels of 0.01 and 0.1 mg kg−1, respectively. Halosulfuron-methyl showed a recovery of 69.2% at the lowest fortification level; however, acceptable recoveries were observed at 0.05 and 0.1 mg kg−1. Although the recoveries of quinmerac were less than 70% at all fortification levels, repeatability and reproducibility were in the acceptable precision criterion ranges; hence, screening for this pesticide was not problematic. Although the lowest fortification level of carbofuran exceeded the EU-MRL level, the validated method provided satisfactory results at a lower level of the Korea-MRL. Precisions were acceptable in all cases, with %RSDs lower than 20%, and the repeatability and reproducibility RSDs were less than 14 and 16% for all pesticides, respectively. LODs ranged from 0.001 to 0.005 mg kg−1. LOQs of 0.002–0.01 mg kg−1 obtained for the target analytes were lower than the lowest points of each linear range. All pesticides exhibited LOQs lower than the lowest MRLs based on EU and Korean requirements.
Table 2. MRLs and method validation data of selected 203 pesticides in strawberry using C18 cleanup method with LC-MS/MS.
Tabla 2. LMR y datos de validación del método para 203 pesticidas detectados en fresa usando el método de limpieza C18 con LC-MS/MS.
Matrix effects (MEs) due to biological samples may result in quantification errors caused by the enhancement or suppression of ionization during LC-MS/MS (Guo et al., Citation2018). presents the retention times and MEs of each pesticide, revealing that the LC-MS/MS responses of 69 tested pesticides exhibited no ME (between −20 and 20%) in the 6–20 min retention time range. More than 70% of pesticides showed medium-level suppression effects (between −20 and −50%); however, several pesticides showed strongly suppressed signals, with MEs of less than −50%. Taylor (Citation2005) reported that LC-MS/MS signals are mainly suppressed by matrix co-eluents. To overcome this issue, alternative approaches such as the use of matrix-matched or standard addition calibration curves have been applied for accurate quantification (Hanafi, Dasenaki, Bletsou, & Thomaidis, Citation2018). We also used the matrix-matching method for pesticide quantitation. With the exception of quinmerac, the MEs of most pesticides did not affect their quantitation; and adequate recoveries and acceptable precisions were observed at all spiking levels ().
3.4. Application of the developed method to real-life sample analysis
The validated method was used to analyze pesticide residues in real strawberries. Ten commercial samples were collected from local markets in Jeonju and Wanju, Korea. Two fungicides (metalaxyl and tricyclazole) and two insecticides (carbofuran and dinotefuran) were detected in the real strawberry samples (). Metalaxyl is used in broadcast soil applications and is frequently detected in fruits and crops. Pous, Ruíz, Picó, and Font (Citation2001) reported that LC-MS is a powerful method for determining metalaxyl in strawberries at concentrations below the MRL. Tricyclazole, which is classified by the WHO as a moderately hazardous pesticide (WHO, Citation2005), was also concurrently found in these samples, albeit at concentrations below the EU and Korean MRLs of 0.01 mg kg−1. Dinotefuran accounts for more than 25% of globally used pesticides (van der Sluijs et al., Citation2013), and numerous studies on this pesticide in crops and vegetables have been reported (Morrissey et al., Citation2015). Some countries have banned the use of carbofuran on crops because of its high toxicity, suggesting less toxic carbosulfan as a substitute (Song et al., Citation2018). As shown in , all detected pesticides were present at levels below the MRLs recommended by Korea (KFDA, Citation2019) and the EU (European Commission, Citation2019); hence, no sample was found to be non-compliant. In conclusion, LC-MS/MS combined with the QuEChERS method and C18 purification was concluded to be suitable for pesticide detection and multi-residue analysis in strawberries.
Table 3. Application of C18 cleanup to 10 strawberry samples.
Tabla 3. Aplicación de limpieza C18 a 10 muestras de fresa.
4. Conclusion
Herein, we demonstrated that LC-MS/MS in combination with QuEChERS d-SPE/C18 extraction can be used to analyze multiple pesticide residues. The validated method provided satisfactory results (linearities, recoveries, and precisions), enabling the qualitative and quantitative analysis of 203 pesticides in strawberries. The introduced approach also provided adequate LOQs compliant with EU and Korean MRLs and was applied to monitor pesticide residues in commercial strawberries. Metalaxyl, tricyclazole, carbofuran, and dinotefuran were found in the tested samples at levels less than the corresponding LOQs. No MRLs were exceeded in all samples. Thus, AOAC extraction using acetate buffer and d-SPE using C18 in combination with LC-MS/MS was concluded to be a rapid, sensitive, and reliable method for the multi-residue analysis of pesticides in strawberries. However, further studies on pesticide residue monitoring in strawberries are required to assess the involved risks and determine cumulative exposure to pesticides through dietary intake.
Disclosure statement
No potential conflict of interest is reported by the authors.
Additional information
Funding
References
- Anastassiades, M., Lehotay, S. J., Štajnbaher, D., & Schenck, F. J. (2003). Fast and easy multiresidue method employing acetonitrile extraction/partitioning and “dispersive solid-phase extraction” for the determination of pesticide residues in produce. Journal of AOAC International, 86(2), 412–431.
- AOAC. (2007, 01). Pesticide residues in foods by acetonitrile extraction and partitioning with magnesium sulphate. AOAC official method (p. 9). Rockville, MD: AOAC International.
- Berlioz-Barbier, A., Vauchez, A., Wiest, L., Baudot, R., Vulliet, E., & Cren-Olivé, C. (2014). Multi-residue analysis of emerging pollutants in sediment using QuEChERS-based extraction followed by LC-MS/MS analysis. Analytical Bioanalytical Chemistry, 406, 1259–1266. doi:10.1007/s00216-013-7450-8
- European Commission. (2017). SANTE/11813/2017. Guidance document on analytical quality control and method validation procedures for pesticides residues analysis in food and feed. European Commission Directorate-General for Health and Food Safety. (rev.0). Retrieved from https://ec.europa.eu/food/sites/food/files/plant/docs/pesticides_mrl_guidelines_wrkdoc_2017-11813.pdf
- European Commission. (2019). Maximum residue levels. Retrieved from https://ec.europa.eu/food/plant/pesticides/max_residue_levels_en/
- Farha, W., Abd El‐Aty, A. M., Rahman, M. M., Jeong, J. H., Shin, H. C., Wang, J., … Shim, J. H. (2018). Analytical approach, dissipation pattern and risk assessment of pesticide residue in green leafy vegetables: A comprehensive review. Biomedical Chromatography, 32(1), e4134. doi:10.1002/bmc.4134
- Forbes-Hernandez, T. Y., Gasparrini, M., Afrin, S., Bompadre, S., Mezzetti, B., Quiles, J. L., … Battino, M. (2016). The healthy effects of strawberry polyphenols: Which strategy behind antioxidant capacity? Critical Reviews in Food Science and Nutrition, 56, S46–S59. doi:10.1080/10408398.2015.1051919
- Guan, Y., Tang, H., Chen, D., Xu, T., & Li, L. (2013). Modified QuEChERS method for the analysis of 11 pesticide residues in tea by liquid chromatography–Tandem mass spectrometry. Analytical Methods, 5(12), 3056–3067. doi:10.1039/c3ay40441b
- Guo, Q., Zhao, S., Zhang, J., Qi, K., Du, Z., & Shao, B. (2018). Determination of fipronil and its metabolites in chicken egg, muscle and cake by a modified QuEChERS method coupled with LC-MS/MS. Food Additives & Contaminants: Part A, 35, 1543–1552. doi:10.1080/19440049.2018.1472395
- Hamdy Abdelwahed, M., Khorshid, M. A., El-Marsafy, A. M., & Souaya, E. (2019). Validation of short run time LC-ESI (+) MS/MS method for determination of twenty recommended pesticide residues in food based on QuEChERS extraction technique. International Journal of Environmental Analytical Chemistry, 99(5), 409–427. doi:10.1080/03067319.2019.1597865
- Hanafi, A., Dasenaki, M., Bletsou, A., & Thomaidis, N. S. (2018). Dissipation rate study and pre-harvest intervals calculation of imidacloprid and oxamyl in exported Egyptian green beans and chili peppers after pestigation treatment. Food Chemistry, 240, 1047–1054. doi:10.1016/j.foodchem.2017.08.037
- Hiemstra, M., & de Kok, A. (2007). Comprehensive multi-residue method for the target analysis of pesticides in crops using liquid chromatography–Tandem mass spectrometry. Journal of Chromatography A, 1154, 3–25. doi:10.1016/j.chroma.2007.03.123
- ICH. (2005). Validation of analytical procedures: Text and methodology. Q2 (R1). International Conference on Harmonization, Geneva, Switzerland, p. 1.
- Kaczyński, P., & Łozowicka, B. (2017). One-step QuEChERS-based approach to extraction and cleanup in multiresidue analysis of sulfonylurea herbicides in cereals by liquid chromatography–Tandem mass spectrometry. Food Analytical Methods, 10(1), 147–160. doi:10.1007/s12161-016-0564-9
- KFDA. (2019). Food Code. Korea food and drug administration. Ministry of Food and Drug Safety. Retrieved from http://www.foodsafetykorea.go.kr/foodcode/02_01.jsp
- Koesukwiwat, U., Lehotay, S. J., Miao, S., & Leepipatpiboon, N. (2010). High throughput analysis of 150 pesticides in fruits and vegetables using QuEChERS and low-pressure gas chromatography–Time-of-flight mass spectrometry. Journal of Chromatography A, 1217(43), 6692–6703. doi:10.1016/j.chroma.2010.05.012
- Lee, Y. J., Rahman, M. M., El-Aty, A. A., Choi, J. H., Chung, H. S., Kim, S. W., … Shim, J. H. (2016). Detection of three herbicide, and one metabolite, residues in brown rice and rice straw using various versions of the QuEChERS method and liquid chromatography-tandem mass spectrometry. Food Chemistry, 210, 442–450. doi:10.1016/j.foodchem.2016.05.005
- Lehotay, S. J., Son, K. A., Kwon, H., Koesukwiwat, U., Fu, W., Mastovska, K., … Leepipatpiboon, N. (2010). Comparison of QuEChERS sample preparation methods for the analysis of pesticide residues in fruits and vegetables. Journal of Chromatography A, 1217, 2548–2560. doi:10.1016/j.chroma.2010.01.044
- Liu, S., Bian, Z., Yang, F., Li, Z., Fan, Z., Zhang, H., … Tang, G. (2015). Determination of multiresidues of three acid herbicides in tobacco by liquid chromatography/tandem mass spectrometry. Journal of AOAC International, 98, 472–476. doi:10.5740/jaoacint.14-089
- Morrissey, C. A., Mineau, P., Devries, J. H., Sanchez-Bayo, F. S., Liess, M., Cavallaro, M. C., & Liber, K. (2015). Neonicotinoid contamination of global surface waters and associated risk to aquatic invertebrates: A review. Environment International, 74, 291–303. doi:10.1016/j.envint.2014.10.024
- Muehlwald, S., Buchner, N., & Kroh, L. W. (2018). Investigating the causes of low detectability of pesticides in fruits and vegetables analysed by high-performance liquid chromatography–Time-of-flight. Journal of Chromatography A, 1542, 37–49. doi:10.1016/j.chroma.2018.02.011
- Nguyen, T. D., Han, E. M., Seo, M. S., Kim, S. R., Yun, M. Y., Lee, D. M., & Lee, G. H. (2008). A multi-residue method for the determination of 203 pesticides in rice paddies using gas chromatography/mass spectrometry. Analytica Chimica Acta, 619, 67–74. doi:10.1016/j.aca.2008.03.031
- Oshita, D., & Jardim, I. C. (2014). Comparison of different sorbents in the QuEChERS method for the determination of pesticide residues in strawberries by LC–MS/MS. Chromatographia, 77(19–20), 1291–1298. doi:10.1007/s10337-014-2726-5
- Pous, X., Ruíz, M., Picó, Y., & Font, G. (2001). Determination of imidacloprid, metalaxyl, myclobutanil, propham, and thiabendazole in fruits and vegetables by liquid chromatography–Atmospheric pressure chemical ionization–Mass spectrometry. Fresenius’ Journal of Analytical Chemistry, 371(2), 182–189. doi:10.1007/s002160100946
- Ruiz-Torralba, A., Guerra-Hernández, E. J., & García-Villanova, B. (2018). Antioxidant capacity, polyphenol content and contribution to dietary intake of 52 fruits sold in Spain. CyTA-Journal of Food, 16(1), 1131–1138. doi:10.1080/19476337.2018.1517828
- Sharma, D., Nagpal, A., Pakade, Y. B., & Katnoria, J. K. (2010). Analytical methods for estimation of organophosphorus pesticide residues in fruits and vegetables: A review. Talanta, 82(4), 1077–1089. doi:10.1016/j.talanta.2010.06.043
- Song, N. E., Lee, J. Y., Mansur, A. R., Jang, H. W., Lim, M. C., Lee, Y., … Nam, T. G. (2019). Determination of 60 pesticides in hen eggs using the QuEChERS procedure followed by LC-MS/MS and GC-MS/MS. Food Chemistry, 298, 125050. doi:10.1016/j.foodchem.2019.125050
- Song, W., Jia, C., Jing, J., Zhao, E., He, M., Chen, L., & Yu, P. (2018). Residue behavior and dietary intake risk assessment of carbosulfan and its metabolites in cucumber. Regulatory Toxicology and Pharmacology, 95, 250–253. doi:10.1016/j.yrtph.2018.03.023
- Taylor, P. J. (2005). Matrix effects: The Achilles heel of quantitative high-performance liquid chromatography–Electrospray–Tandem mass spectrometry. Clinical Biochemistry, 38(4), 328–334. doi:10.1016/j.clinbiochem.2004.11.007
- van der Sluijs, J. P., Simon-Delso, N., Goulson, D., Maxim, L., Bonmatin, J. M., & Belzunces, L. P. (2013). Neonicotinoids, bee disorders and the sustainability of pollinator services. Current Opinion in Environmental Sustainability, 5(3–4), 293–305. doi:10.1016/j.cosust.2013.05.007
- WHO. (2005). The WHO recommended classification of pesticides by hazards and guideline to classification: 2004. Geneva: Author.
- Yang, X., Luo, J., Duan, Y., Li, S., & Liu, C. (2018). Simultaneous analysis of multiple pesticide residues in minor fruits by ultrahigh-performance liquid chromatography/hybrid quadrupole time-of-fight mass spectrometry. Food Chemistry, 241, 188–198. doi:10.1016/j.foodchem.2017.08.102
- Zhang, H., Wang, J., Li, L., & Wang, Y. (2017). Determination of 103 pesticides and their main metabolites in animal origin food by QuEChERS and liquid chromatography–Tandem mass spectrometry. Food Analytical Methods, 10(6), 1826–zh1843. doi:10.1007/s12161-016-0736-7
AppendixApéndice
Table A1. LC-MS/MS parameter values used during the detection of 203 pesticides.
Tabla A1. Valores de parámetros LC-MS/MS utilizados durante la detección de 203 pesticidas.