ABSTRACT
Glycoprotein VI (GPVI) is the major collagen receptor on the platelet surface and is important in mediating granule secretion and atherothrombosis. Sulforaphane (SFN), a dietary isothiocyanate enriched in cruciferous vegetables, exerts multiple biological activities. This study aimed to investigate the efficacy of SFN on GPVI-mediated signaling and platelet granule secretion in vitro. We herein demonstrated that SFN greatly attenuated granule secretion in human gel-filtered platelets in response to collagen, including inhibiting CD62P and CD63 expression and PF4, CCL5, and ATP release. Mechanistically, these inhibitory effects of SFN were mainly mediated by its attenuation of cytosolic phospholipase A2 (cPLA2) phosphorylation and thromboxane A2 (TxA2) generation. Moreover, SFN did not further decrease the inhibitory effects of p38 MAPK inhibitor SB203580 on cPLA2 phosphorylation, TxA2 generation and granule secretion. Thus, SFN attenuates platelet granule secretion via down-regulating GPVI-mediated p38 MAPK/cPLA2/TxA2 signaling, which may play important preventive roles in cardiovascular diseases.
Introduction
Cardiovascular diseases are the leading causes of mortality and morbidity worldwide (Leong et al., Citation2017). Platelets are anucleate blood cells that play crucial roles in both the early (atherosclerosis) and late (thrombosis) stages of atherothrombosis and thus important in the progression of cardiovascular diseases (CVDs) (Gawaz et al., Citation2005; Violi & Pignatelli, Citation2012; Xu et al., Citation2016). Upon vascular damage, platelets are activated and rapidly adhere to the exposed extracellular matrix (ECM) (Wang et al., Citation2016). Collagen is the most abundant constituent of the ECM and plays a vital role in the initial atherothrombosis mainly through GPVI transmembrane receptor, leading to initiating a series of events including platelet activation, granule secretion, spreading, and aggregation (Perrella et al., Citation2021). Upon these events, GPVI-mediated platelet granule secretion plays both initial and amplifying roles in platelet activation and aggregation and thus facilitating the progression of atherothrombosis and CVDs (Joshi et al., Citation2018). These granules predominantly include α-granules, dense granules, and lysosomes (Bakogiannis et al., Citation2019 ; Ghasemzadeh & Hosseini, Citation2017). Platelet α-granules are the most abundant and contain both membrane-bound proteins (e.g. CD62P) and soluble proteins (e.g. PF4 and CCL5). Platelet dense granules contain ATP, adenosine diphosphate (ADP), calcium, and pyrophosphate. These granules can facilitate platelet activation and aggregation and enhance activation of leukocytes and endothelial cells (Blair & Flaumenhaft, Citation2009; Palabrica et al., Citation1992). Lysosomes containing some membrane proteins (e.g. CD63) and hydrolases are important in atherothrombosis (Azorsa et al., Citation1991). Therefore, GPVI-mediated granule secretion is one of the important targets for the development of more effective antithrombotic treatments in the early stages of CVDs (Perrella et al., Citation2021; Rayes et al., Citation2019).
It has been well documented that diet or dietary composition intervention is one of the key approaches to prevent CVDs (Martinez-Gonzalez et al., Citation2019; Mozaffarian, Citation2016; Ya et al., Citation2019). As a dietary isothiocyanate, sulforaphane (SFN) is enriched in many commonly consumed cruciferous vegetables, such as broccolis, cauliflowers, and cabbages (Conaway et al., Citation2000; Vanduchova et al., Citation2019). Studies have demonstrated that SFN exerts multiple biological activities, including anti-oxidant, anti-inflammation, and anti-cancer (Elkashty & Tran, Citation2021; Liu & Talalay, Citation2013). In recent years, increasing evidences have shown that SFN possesses cardiovascular protection, involving the disorder of glycolipid metabolism improvement, atherogenesis inhibition, metabolic detoxication, and immunological regulation (Bai et al., Citation2015; Briones-Herrera et al., Citation2018). Although previous studies have indicated that SFN could attenuate platelet aggregation stimulated by various agonists including thrombin, collagen, and ADP in vitro (Chuang et al., Citation2013; Gillespie et al., Citation2018; Jayakumar et al., Citation2013), whether and how SFN acts on platelet granule secretion are poorly understood. Our current study sought to investigate the efficacy of SFN on platelet granule secretion and to elucidate the underlying mechanisms in vitro.
Materials and methods
Chemicals and reagents
L-sulforaphane, thrombin, Fluo-4 AM, Pluronic F-127, U46619, aspirin (ASA) were purchased from Sigma-Aldrich (St. Louis, MO, U.S.A). Collagen was obtained from Chrono-Log Corp. (Havertown, PA, U.S.A). Convulxin and pyrrophenone were obtained from Cayman (Hamburg, Germany). Fluorescein isothiocyanate (FITC)-conjugated anti-human CD62P antibody, FITC-conjugated anti-human CD63 antibody, and CCL5 commercial enzyme linked immunosorbent assay (ELISA) kit were purchased from eBioscience (San Diego, CA, U.S.A). PF4 commercial ELISA kit was purchased from R&D Systems (Minneapolis, MN, U.S.A). ATP detection assay kit was obtained from Beyotime Institute of Biotechnology (Shanghai, China). TxB2 commercial ELISA kit was purchased from Enzo Life Sciences (Farmingdale, NY, U.S.A). Antibodies for phospho-cPLA2 (Ser505), phospho-Syk (Tyr323), phospho-LAT (Tyr171), phospho-PLCγ2 (Tyr759), β-actin, and GAPDH were purchased from Cell Signaling Technology (Danvers, MA, U.S.A). 740 Y-P, SP600125, U0126, and SB203580 were obtained from Selleck Chemical (Houston, Texas, U.S.A).
Human subject recruitment
Twenty healthy adults (6 males and 14 females; 25–40 years old) who had not taken any antiplatelet medications within the previous 2 weeks were enrolled in this study. Subjects were excluded if they had a history of serious diseases, including hemostatic disorders, diabetes mellitus, hypertension, CVDs, or other diseases that had been identified to significantly influence platelet function (Ya et al., Citation2018, Citation2019). This study was approved in China by the Ethics Committee of Dali University and all the subjects provided written informed consent, according to institutional guidelines and the Helsinki Declaration from all the subjects prior to enrollment.
Human blood collection and platelet preparation
Human blood collection and gel-filtered platelet preparation were according to our previously described methods (W. Li et al., Citation2022; Ya et al., Citation2019, Citation2021). In brief, whole human blood from independent healthy subjects was drawn into a vacutainer containing 3.2% sodium citrate (1/9, v/v) via venipuncture. Platelet-rich plasma was further obtained from anticoagulated blood by centrifugation at 300×g for 7 min. Plasma-free gel-filtered platelets were then isolated from the platelet-rich plasma using a Sepharose 2B column (Sigma-Aldrich, MO, U.S.A). Gel-filtered platelets were stored at 37°C until further use within 2 h.
Measurement of platelet surface CD62P and CD63 expression
Human gel-filtered platelets (5 × 106/mL) were pre-treated with various concentrations of SFN (1 μM, 5 μM, 10 μM, 50 μM, or 100 μM) or solvent control (0.05% DMSO) for indicated time. The platelets were then labeled with FTIC-conjugated anti-human CD62P antibody or CD63 antibody for 20 min, followed by the activation of collagen (2 μg/mL or 3 μg/mL) or thrombin (0.1 U/mL) for 5 min in the presence of 1 mM CaCl2. Platelets were then fixed with 1% paraformaldehyde, and the expression of CD62P and CD63 were further measured by using a CytoFLEX flow cytometer (Beckman Coulter Inc., CA, U.S.A), as we previously described (W. Li et al., Citation2022; Ya et al., Citation2019).
Measurement of platelet aggregation
Human gel-filtered platelets (2.5 × 108/mL) were pre-incubated with various concentrations of SFN (1 μM, 5 μM, or 10 μM) or solvent control for 40 min. Platelet aggregation induced by collagen (2 μg/mL) was carried out by using a Techlink 400 aggregometer (Techlink Biomedical Technology Corp., Beijing, China), as we described previously (W. Li et al., Citation2022; Ya et al., Citation2019, Citation2021).
Measurement of platelet secretion of PF4, CCL5, ATP and TxA2
Human gel-filtered platelets (1 × 108/mL) were pre-incubated with various concentrations of SFN or solvent control for 40 min and then stimulated by 2 μg/mL collagen, 0.1 U/mL thrombin, 50 ng/mL convulxin, or 5 µM U46619 for 5 min in the presence of 1 mM CaCl2. The reaction was stopped by adding equal volume of ice-cold EDTA (16 mM) for 5 min. The cell-free supernatant was collected by centrifugation at 12,000×g for 5 min at 4°C. The levels of PF4, CCL5, ATP, and TxB2 in the supernatant were measured by using commercial enzyme-linked immunosorbent assay (ELISA) kits, according to the manufacturer’s instructions. Since TxA2 is rapidly converted into the inactive TxB2 after its release, the levels of TxB2 generation were generally used to reflect the generation of TxA2 (Naik et al., Citation2017; W. Li et al., Citation2022).
Measurement of intracellular calcium concentration ([Ca2+]i)
[Ca2+]i was measured as we previously described (W. Li et al., Citation2022; Ya et al., Citation2021). Briefly, in the presence of 0.2 μg/mL Pluronic F-127, platelets were incubated with Fluo-4 AM (5 μM) for 30 min in the dark, followed by centrifugation at 3000×g for 5 min. Platelets were then suspended by calcium-free PIPES buffer and adjusted to the concentration of 1 × 108/mL. Platelets were treated with SFN for 40 min and then activated by 2 μg/mL collagen for 5 min. Fluorescence intensity (FI) was recorded by using a multimode reader at excitation/emission wavelength of 488 nm/520 nm. Data are presented as the fold change in the FI comparing maximum FI relative to FI prior to platelet activation.
Western blotting analysis
Human gel-filtered platelets (2.5 × 108/mL) were pre-incubated with various concentrations of SFN, followed by the stimulation with 2 μg/mL collagen in the presence of 1 mM CaCl2 for 5 min. Platelet pellets were lysed with lysis buffer (1% nonidet P 40 (NP40), 0.1% SDS, 50 mM Tris, 0.5% sodium deoxycholate, and 150 mM NaCl) supplemented with protease and phosphatase inhibitors for 30 min. The supernatant was collected and further separated by SDS-PAGE to perform Western blotting assays, as we previously described (Ya et al., Citation2018, Citation2019, Citation2020).
Statistical analysis
Statistical analyses were performed by using GraphPad Prism version 7.00 software (GraphPad Inc., San Diego, CA, U.S.A). Data were expressed as the means ± standard errors of the means (SEM) of at least three independent experiments. Statistical significance was assessed by using a one-way analysis of variance (ANOVA) followed by Dunnett’s t-test or followed by the Newman – Keuls test for multiple comparisons. Differences were considered statistically significant at P < .05.
Results
SFN attenuates platelet granule secretion and aggregation
As shown in , we demonstrated that SFN (1 ~ 10 μM) significantly attenuated collagen-induced platelet surface CD62P expression () and soluble protein PF4 () and CCL5 release () in response to 2 μg/mL collagen. Moreover, SFN greatly inhibited collagen-induced ATP secretion (), intracellular calcium concentration ([Ca2+]i) () and surface CD63 expression (). Although a trend toward an inhibitory effect was observed, SFN (1 ~ 10 μM) did not achieve a significant inhibition in platelet surface expression of CD62P and CD63 induced by 3 μg/mL collagen (Supplementary Appendix Figure S1). Moreover, we found that collagen-induced platelet aggregation was also greatly attenuated by SFN (5 and 10 μM) (). In addition, the inhibitory effects of SFN on thrombin-induced platelet granule secretion were only observed in concentrations of 50 and 100 μM (Supplementary Appendix Figure S2). The data indicate that SFN exhibits more potent inhibitory effects on platelet granule secretion induced by collagen than those induced by thrombin. We thus used collagen and other GPVI agonist (e.g. convulxin) to further clarify the underlying mechanism of SFN in the following experiment.
Figure 1. SFN attenuates platelet granule secretion and aggregation. Human gel-filtered platelets were pre-incubated with SFN (1, 5, or 10 µm) or the vehicle control (0.05% DMSO) for 40 min. (a-b) Platelet surface expression of CD62P was analyzed by flow cytometry after stimulation with 2 μg/mL collagen for 5 min. (c-d) the secretion of platelet PF4 (c), CCL5 (d), ATP (e) and levels of intracellular calcium concentration ([Ca2+]i) (f) were determined after stimulation with 2 μg/mL collagen for 5 min. (g-h) Same as (a-b), except platelet surface expression of CD63 was analyzed. (i-j) Platelet aggregation stimulated by 2 μg/mL collagen was performed. *P < .05, **P < .01 and ***P < .001 vs. the vehicle control as assessed by a one-way ANOVA followed by Dunnett’s t-test (n = 3).
![Figure 1. SFN attenuates platelet granule secretion and aggregation. Human gel-filtered platelets were pre-incubated with SFN (1, 5, or 10 µm) or the vehicle control (0.05% DMSO) for 40 min. (a-b) Platelet surface expression of CD62P was analyzed by flow cytometry after stimulation with 2 μg/mL collagen for 5 min. (c-d) the secretion of platelet PF4 (c), CCL5 (d), ATP (e) and levels of intracellular calcium concentration ([Ca2+]i) (f) were determined after stimulation with 2 μg/mL collagen for 5 min. (g-h) Same as (a-b), except platelet surface expression of CD63 was analyzed. (i-j) Platelet aggregation stimulated by 2 μg/mL collagen was performed. *P < .05, **P < .01 and ***P < .001 vs. the vehicle control as assessed by a one-way ANOVA followed by Dunnett’s t-test (n = 3).](/cms/asset/f7147b32-5621-490e-b77f-6bd117dd85ed/tcyt_a_2173307_f0001_b.gif)
SFN attenuates platelet granule secretion involving in reducing TxA2 generation
It has been clarified that TxA2 generation plays important roles in regulation of granule secretion (Z. Li et al., Citation2003). As shown in , SFN significantly inhibited TxA2 generation in human platelets stimulated by GPVI agonists including collagen and convulxin. Aspirin (ASA) has been well demonstrated to block cyclooxygenase-1 (COX-1) activity that is a key enzyme required for TxA2 generation and plays important roles in granule secretion (Lucotti et al., Citation2019). To further investigate whether the observed inhibitory effects of SFN on granule secretion were resulted from its attenuation of TxA2 generation, we determined the collagen-stimulated release of PF4, CCL5, and ATP in the presence of ASA. We observed that ASA (100 μM) alone greatly attenuated PF4, CCL5, and ATP release induced by collagen (). Importantly, SFN did not further significantly decrease PF4, CCL5, and ATP secretion when combined with ASA. Moreover, the inhibitory effects of SFN on PF4 and CCL5 secretion were overcome in platelets stimulated by TxA2 mimetic U46619 (5 μM) (). Thus, the data indicate that SFN-attenuated platelet granule secretion is mainly mediated by its down-regulation of TxA2 generation.
Figure 2. SFN attenuates platelet granule secretion involving in reducing TxA2 generation. Human gel-filtered platelets were pre-incubated with various concentrations of SFN or the vehicle control for 40 min. The levels of TxB2 generation were measured in platelets stimulated with 2 μg/mL collagen (a) or 50 ng/mL convulxin (b) for 5 min (n = 5). (c-e) Human gel-filtered platelets were incubated with SFN (10 µm) or the vehicle control for 40 min in the absence or presence of 100 µm ASA. The levels of platelet PF4 (c), CCL5 (d) and ATP (e) secretion were determined after stimulation with 2 μg/mL collagen for 5 min (n = 3). (f-g) Human gel-filtered platelets were incubated with various concentrations of SFN or the vehicle control for 40 min. The levels of platelet PF4 (f) and CCL5 (g) secretion were determined after stimulation with 5 µm U46619 for 5 min (n = 3). Data were assessed by a one-way ANOVA followed by the Newman–Keuls test in (c-e) and by Dunnett’s t-test in a, b, f and g. *P < .05 and ***P < .001 vs. the vehicle control, and #P < .05, ##P < .01; NS, not significant difference.
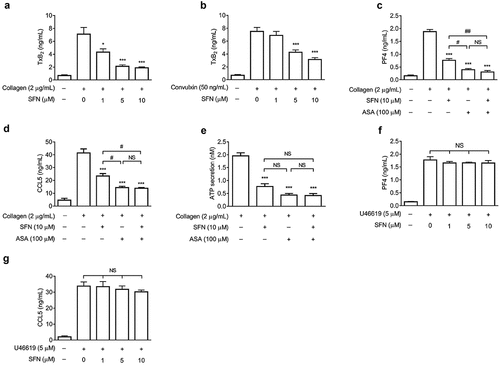
SFN down-regulates cPLA2 phosphorylation, resulting in decreased TxA2 generation and granule secretion
It has been evidenced that platelet TxA2 biogenesis is dependent on cPLA2 activation (Fontana et al., Citation2014). As shown in , collagen-increased phosphorylation of cPLA2 at Ser505 was favorably down-regulated by SFN treatment. To further investigate whether the inhibitory effects on TxA2 generation and granule secretion were dependent on its mediation of cPLA2 activation, a specific cPLA2 inhibitor pyrrophenone was used. We found that pyrrophenone (1 μM) alone significantly inhibited TxA2 generation as well as PF4 and CCL5 secretion, which were comparable to the inhibitory effects of SFN (10 μM) treatment (). Additionally, pyrrophenone did not show any additive inhibitory effects by the addition of SFN. These results indicate that the inhibitory effects of SFN on TxA2 generation and granule secretion are mainly mediated by its down-regulating cPLA2 activation.
Figure 3. SFN attenuates platelet TxA2 generation and granule secretion via down-regulating cPLA2 phosphorylation. (a) Human gel-filtered platelets were incubated with various concentrations of SFN or the vehicle control for 40 min, followed by the stimulation of 2 μg/mL collagen for 5 min. Western blotting was performed to assess total and phosphorylation of cPLA2 at Ser505. (b-d) Human gel-filtered platelets were incubated with SFN (10 µm) or the vehicle control for 40 min in the absence or presence of pyrrophenone (1 µm), then the levels of TxB2 generation (b), and PF4 (c) and CCL5 (d) release were measured after stimulation with 2 μg/mL collagen for 5 min. Data were assessed by a one-way ANOVA followed by Dunnett’s t-test in a and by the Newman–Keuls test in b-d (n = 3). *P < .05, **P < .01 and ***P < .001 vs. the vehicle control; NS, not significant difference.
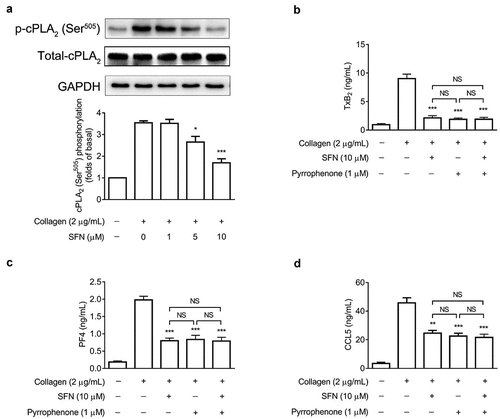
SFN regulates platelet TxA2 generation and granule secretion by down-regulating p38 MAPK/cPLA2 signaling pathway
It has been well documented that the MAPK signaling pathways are important in mediating platelet activation, granule secretion, and thrombosis (Patel & Naik, Citation2020). We sought to further evaluate whether the inhibitory effect of SFN on platelet granule secretion was attributed to its down-regulation of MAPK signaling pathways by using specific kinase inhibitors. As shown in , the combination of SFN with either Erk1/2 inhibitor U0126 or JNK1/2 inhibitor SP600125 resulted in additive inhibitory effects on TxA2 generation (). However, SFN failed to further inhibit TxA2 generation () and cPLA2 phosphorylation () when combined with p38 MAPK inhibitor SB203580 (20 μM). The concentration of SB203580 used in our current study has been reported to completely abolish agonists-induced p38 MAPK phosphorylation and platelet granule secretion (W. Li et al., Citation2022 ; Qi et al., Citation2021). Moreover, consistent with the results from the mediation of TxA2 generation, both U0126 and SP600125 but not SB203580 resulted in additive inhibitory effects on PF4 secretion when combined with SFN (). Previous reports have also demonstrated that PI3K/Akt signaling pathway is important in platelet activation and granule secretion (Moroi & Watson, Citation2015). As shown in , the inhibitory effects of SFN on platelet PF4 secretion was only partially reversed by the pan-PI3K agonist 740 Y-P (25 μg/mL); this concentration of 740 Y-P has been reported to completely reverse the agonist-induced platelet PI3K and Akt phosphorylation (W. Li et al., Citation2022). The cAMP/PKA signaling plays important roles in negatively regulating platelet hyper-reactivity (Fuentes et al., Citation2014). We found that the inhibitory effects of SFN (10 μM) on platelet surface CD62P expression, PF4 and CCL5 release was reversed by the PKA specific inhibitor H89 (10 μM) ().
Figure 4. SFN regulates platelet TxA2 generation and granule secretion by down-regulating p38 MAPK/cPLA2 signaling pathway. Human gel-filtered platelets were incubated with SFN (10 µm) or the vehicle control for 40 min in the absence or presence of 5 µm U0126 (a, e), 10 µm SP600125 (b, f), or 20 µm SB203580 (c, g) followed by the stimulation of 2 μg/mL collagen. The levels of TxB2 generation (a-c) and PF4 secretion (e-g) were measured. (d) same as (C), except cPLA2 (Ser505) phosphorylation was assessed by Western blotting. (h) same as (E), except platelets were incubated with SFN or the vehicle control in the absence or presence of 740 Y-P (25 µg/mL). (i-k) Human gel-filtered platelets were incubated with SFN (10 µm) or the vehicle control for 40 min in the absence or presence of H89 (10 µm), followed by the stimulation of 2 μg/mL collagen. The levels of platelet surface CD62P expression (i), PF4 (j) and CCL5 (k) release were measured. *P < .05, **P < .01 and ***P < .001 vs. the vehicle control using a one-way ANOVA followed by the Newman–Keuls test (n = 3). #P < .05 and ##P < .01; NS, not significant difference.
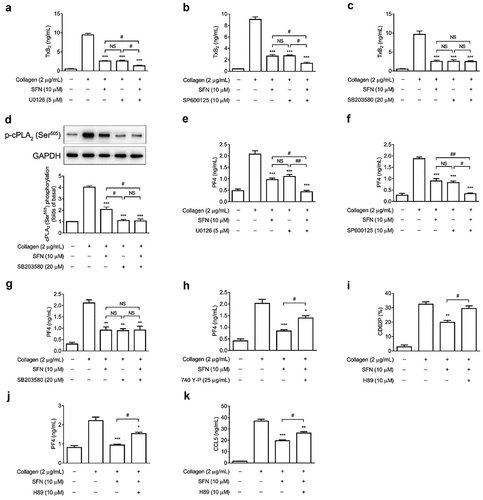
Discussion
High platelet reactivity and increased granule secretion are associated with a higher risk of thrombotic disorders, which are the leading causes of mortality and morbidity worldwide (Leong et al., Citation2017). Controlling platelet hyper-reactivity and granule secretion has been considered an effective strategy to dampen the progression of CVDs (Joshi et al., Citation2018; Xu et al., Citation2016). In this study, we identified that SFN attenuated platelet granule secretion mainly through a mechanism of down-regulating GPVI-mediated p38 MAPK/cPLA2/TxA2 signaling pathway. Thus, SFN may be a promising agent for the prevention and/or treatment of CVDs.
An increasing body of evidence suggests that platelet granule secretion plays vital roles in inflammatory responses upon the progression of atherosclerosis and thrombosis (Joshi et al., Citation2018). Under some pathological circumstances (e.g. hyperlipidaemia and diabetes mellitus), activated platelets can secrete many granules and thus facilitating atherothrombosis and CVDs. Platelets expressed CD62P on their surface play crucial roles in mediating monocyte rolling by interacting with monocyte P-selectin glycoprotein ligand-1 (Burger & Wagner, Citation2003). Consistent with the previous study (Gillespie et al., Citation2018), we herein demonstrated that SFN decreased platelet CD62P expression induced by collagen and thrombin. Moreover, our present study is a novel study to identify that SFN can also attenuate platelet surface CD63 expression and PF4, CCL5, and ATP release in human platelets in vitro. PF4 and CCL5-derived activated platelets can amplify platelet activation, spreading and aggregation, trigger monocyte recruitment and endotheliocyte activation (Blair & Flaumenhaft, Citation2009). Although currently unknown, our data indicate that the inhibitory effects of SFN on platelet proinflammatory granule molecule secretion may contribute to its attenuation of atherothrombosis, which, nevertheless, requires further clarification by using in vivo studies.
The mechanisms of platelet granule secretion are complicated and not fully illuminated. Collagen plays a vital role in the initial platelet activation and granule secretion mainly through GPVI receptor (Perrella et al., Citation2021). Our current results demonstrated that the inhibitory effect of SFN on collagen-induced granule secretion was observed in concentrations of 1 ~ 10 μM, but on thrombin-stimulated granule secretion was only observed in high concentrations (50 and 100 μM). These results were consistent with the previous study reporting the effect of SFN on platelet aggregation (Chuang et al., Citation2013). Our current data indicate that GPVI may play a predominant role in the efficacy of SFN on granule secretion. The interaction between collagen and GPVI initiates signaling cascades (e.g. Src family kinases, Syk tyrosine kinases, linker for activation of T cells (LAT), PLCγ2, and PKC) that contribute to platelet activation and granule secretion (Perrella et al., Citation2021). We found that SFN attenuated these signaling cascades including inhibiting Syk, LAT, and PLCγ2 phosphorylation in human platelets stimulated by collagen (Supplementary Appendix Figure S3). This was consistent with a previous report indicating that SFN-mediated inhibition of platelet aggregation was involved in down-regulating GPVI signaling (Jayakumar et al., Citation2013). Moreover, our current study demonstrated that the inhibitory effect of SFN on platelet surface CD62P expression induced by collagen was observed in 40 min and 50 min of SFN incubation but not in the 30-min (or less time) incubation (Supplementary Appendix Figure S4). This suggests that, just like any other cells, SFN seems to take time to go from extracellular to intracellular and further plays its roles in platelets. Although it is needed to further investigate, we thus speculate that SFN is unlikely to directly interact with the platelet surface GPVI receptor and affect its dimerization through a way of competitive inhibition. Although the health benefits of SFN have been well documented, the underlying mechanisms are not fully understood. Numerous studies have indicated that SFN acts on its function as a cytoprotective agent through targeting intracellular signaling pathways. There are still no reports about whether cell surface exists a receptor specific for SFN. Therefore, the underlying mechanisms of SFN remain to be deeply clarified in further studies.
TxA2 generation plays a key step downstream of GPVI-mediated Src/Syk/PLCγ2/PKC signaling in amplifying the initial platelet activation and granule secretion (Fontana et al., Citation2014; Naik et al., Citation2017; W. Li et al., Citation2022). We also observed that SFN attenuated platelet TxA2 generation in response to collagen and GPVI-specific agonist convulxin. Interestingly, SFN failed to further decrease granule secretion in the presence of aspirin that can inhibit TxA2 generation by blocking COX-1 activity, indicating an important role of COX-1/TxA2 axis in mediating SFN-inhibited granule secretion. Our results demonstrating that SFN-inhibited granule secretion was overcome by exogenous addition of the TxA2 mimetic U46619 further confirmed the predominant role of TxA2 generation in SFN-mediated granule secretion. Newly produced TxA2 binds to thromboxane prostanoid (TP) receptor, resulting in activation of integrin αIIbβ3 signaling that is required for platelet spreading, aggregation, and granule secretion (Estevez et al., Citation2015). Previous study has reported that SFN attenuated platelet aggregation and thrombosis possibly via decreasing αIIbβ3 activation (Gillespie et al., Citation2018). We speculate that SFN may down-regulate GPVI-mediated Src/Syk/LAT/PLCγ2 signaling, leading to decreased calcium release, PKC activation, and integrin αIIbβ3 inside-out and outside-in signaling, which may also contribute to decreased granule secretion and merits further investigation.
During platelet activation, TxA2 biogenesis is dependent on cPLA2 activation and phosphorylation at Ser505 (Fontana et al., Citation2014). Our present study found that SFN down-regulated collagen-induced cPLA2 phosphorylation, and that SFN did not further decrease TxA2 generation and granule secretion in the presence of the cPLA2 specific inhibitor pyrrophenone. The data indicate that TxA2 generation and granule secretion inhibited by SFN are dependent mainly on cPLA2 activation. Previous study has reported that MAPK (Erk1/2, JNK1/2, and p38 MAPK) signaling pathways were involved in the inhibitory effect of SFN on platelet aggregation (Jayakumar et al., Citation2013). We and others previously demonstrated that MAPK signaling pathways are important in inducing platelet cPLA2 activation and TxA2 generation, leading to increased granule secretion, platelet aggregation, and thrombus growth (Naik et al., Citation2017; W. Li et al., Citation2022). Consistent with previous reports, our current data also showed that collagen-induced TxA2 generation was inhibited by the MAPK inhibitors. Importantly, SFN did not further decrease cPLA2 phosphorylation, TxA2 generation, and PF4 secretion in the presence of the p38 MAPK inhibitor SB203580 but not the Erk1/2 inhibitor U0126 or the JNK1/2 inhibitor SP600125. The concentration of SB203580 (20 μM) used is sufficient to completely abolish agonists-induced p38 MAPK activation in human platelets (W. Li et al., Citation2022). These data indicate that p38 MAPK lied downstream of GPVI-mediated signaling cascades plays a predominant role in SFN-mediated cPLA2/TxA2 axis and granule secretion.
In addition, PI3K/Akt serving as a downstream of GPVI-mediated signaling is also important in mediating platelet activation, granule secretion, and thrombosis (Moroi & Watson, Citation2015). It has been indicated that down-regulation of PI3K/Akt pathway contributed to the inhibitory effect of SFN on platelet aggregation induced by collagen and thrombin in vitro (Chuang et al., Citation2013). Whether this signaling pathway is involved in SFN-mediated inhibition of granule secretion is poorly understood. Interestingly, although the used concentration of the PI3K agonist 740 Y-P (25 μg/mL) is sufficient to completely reverse the agonist-induced PI3K phosphorylation in human platelets (W. Li et al., Citation2022), we herein found that 740 Y-P (25 μg/mL) could only partially reverse the effect of SFN on platelet PF4 release. The data indicate that PI3K/Akt signaling pathway plays a minor role in the SFN-mediated inhibition of granule secretion in platelets activated by collagen. However, whether PI3K/Akt pathway is involved in the regulation of SFN in thrombin-induced granule secretion is unknown and needs to be further investigated. Moreover, previous report has indicated that SFN increased platelet cAMP levels, which was involved in its attenuation of platelet aggregation (Jayakumar et al., Citation2013). Interestingly, we found that the PKA specific inhibitor H89 could completely reverse the inhibitory effects of SFN on platelet surface CD62P expression, but partially reverse its effect on PF4 and CCL5 release. The data indicate that different mechanisms of how platelets regulate surface expression of membrane-bound proteins (e.g. CD62P) and secretion of soluble proteins (e.g. PF4 and CCL5) may affect the effects of SFN and H89. Our current data suggest that the cAMP/PKA signaling pathway also participates in the inhibitory effect of SFN on platelet granule secretion in vitro. Nevertheless, how cAMP/PKA signaling modulates GPVI-mediated platelet granule secretion and how SFN acts on cAMP metabolism require to be further investigated.
SFN is a dietary isothiocyanate enriched in many commonly consumed cruciferous vegetables and is well tolerated in humans (Russo et al., Citation2018). It has been reported that the administration of 200 μM per day of SFN-rich broccoli sprout extracts for 20 weeks is safe for prostate cancer (Alumkal et al., Citation2015). However, further studies are needed to assess whether SFN administration will serve as a safe antiplatelet and antithrombotic agent. Moreover, the plasma SFN concentration in healthy adults can be reached about 7.4 μM after supplementation of 100 g broccoli (Gasper et al., Citation2005). Previous study has shown that SFN at 0.1 ~ 100 μM exerted no significant cytotoxicity in human platelets in vitro (Gillespie et al., Citation2018). Our current study showed that the significant effects of SFN on platelet granule secretion were observed in the 5 μM and 10 μM doses in collagen-stimulated platelets, which are similar to other in vitro studies (Chuang et al., Citation2013; Gillespie et al., Citation2018). In addition, the inhibitory effect of SFN (10 μM) on platelet surface CD62P expression induced by collagen was observed in 40 min and 50 min of SFN incubation, but not in the 30-min incubation (Supplementary Figure S4). Scarlett et al. have demonstrated that the 30-min incubation of SFN (40 ~ 100 μM) exhibited a significant inhibitory effect on platelet aggregation (Scarlett et al., Citation2018). It seems that lower concentrations of SFN that achieve a significant inhibition are supposed to need a longer incubation period. Moreover, the incubation time (40 min) of SFN in our present study was closed to the elimination half-life (T1/2) of blood SFN reported by Eun et al., who showed that T1/2 of SFN was 41.9 min after an oral dose of 0.1 mg/kg SFN in rats (Eun et al., Citation2021).
Collectively, our present study demonstrates that SFN down-regulates GPVI-mediated p38 MAPK/cPLA2 signaling pathway in human platelets, leading to a decreased TxA2 generation and granule secretion. Our findings further indicate that dietary supplement of SFN may be a promising strategy for the protection against CVDs.
Supplemental Material
Download MS Word (1.1 MB)Disclosure statement
No potential conflict of interest was reported by the author(s).
Supplementary data
Supplemental data for this article can be accessed online at https://doi.org/10.1080/19476337.2023.2173307
Additional information
Funding
References
- Alumkal, J. J., Slottke, R., Schwartzman, J., Cherala, G., Munar, M., Graff, J. N., Beer, T. M., Ryan, C. W., Koop, D. R., Gibbs, A., Gao, L., Flamiatos, J. F., Tucker, E., Kleinschmidt, R., & Mori, M. (2015). A phase II study of sulforaphane-rich broccoli sprout extracts in men with recurrent prostate cancer. Investigational New Drugs, 33(2), 480–489. https://doi.org/10.1007/s10637-014-0189-z
- Azorsa, D. O., Hyman, J. A., & Hildreth, J. E. (1991). CD63/Pltgp40: A platelet activation antigen identical to the stage-specific, melanoma-associated antigen ME491. Blood, 78(2), 280–284. https://doi.org/10.1182/blood.V78.2.280.280
- Bai, Y., Wang, X., Zhao, S., Ma, C., Cui, J., & Zheng, Y. (2015). Sulforaphane protects against cardiovascular disease via Nrf2 Activation. Oxidative Medicine and Cellular Longevity, 2015, 407580. https://doi.org/10.1155/2015/407580
- Bakogiannis, C., Sachse, M., Stamatelopoulos, K., & Stellos, K. (2019). Platelet-derived chemokines in inflammation and atherosclerosis. Cytokine, 122, 154–157. https://doi.org/10.1016/j.cyto.2017.09.013
- Blair, P., & Flaumenhaft, R. (2009). Platelet α-granules: Basic biology and clinical correlates. Blood reviews, 23(4), 177–189. https://doi.org/10.1016/j.blre.2009.04.001
- Briones-Herrera, A., Eugenio-Perez, D., Reyes-Ocampo, J. G., Rivera-Mancia, S., & Pedraza-Chaverri, J. (2018). New highlights on the health-improving effects of sulforaphane. Food & Function, 9(5), 2589–2606. https://doi.org/10.1039/C8FO00018B
- Burger, P. C., & Wagner, D. D. (2003). Platelet P-selectin facilitates atherosclerotic lesion development. Blood, 101(7), 2661–2666. https://doi.org/10.1182/blood-2002-07-2209
- Chuang, W. Y., Kung, P. H., Kuo, C. Y., & Wu, C. C. (2013). Sulforaphane prevents human platelet aggregation through inhibiting the phosphatidylinositol 3-kinase/akt pathway. Thrombosis and Haemostasis, 109(6), 1120–1130. https://doi.org/10.1160/TH12-09-0636
- Conaway, C. C., Getahun, S. M., Liebes, L. L., Pusateri, D. J., Topham, D. K., Botero-Omary, M., & Chung, F. L. (2000). Disposition of glucosinolates and sulforaphane in humans after ingestion of steamed and fresh broccoli. Nutrition and Cancer, 38(2), 168–178. https://doi.org/10.1207/S15327914NC382_5
- Elkashty, O. A., & Tran, S. D. (2021). Sulforaphane as a promising natural molecule for cancer prevention and treatment. Current Medical Science, 41(2), 250–269. https://doi.org/10.1007/s11596-021-2341-2
- Estevez, B., Shen, B., & Du, X. (2015). Targeting integrin and integrin signaling in treating thrombosis. Arteriosclerosis, Thrombosis, and Vascular Biology, 35(1), 24–29. https://doi.org/10.1161/ATVBAHA.114.303411
- Eun, S. S., Fei, X., Yoon, J. H., Seo, S. Y., Maeng, H. J., Jeong, S. H., & Kim, Y. C. (2021). Comparison of pharmacokinetics and anti-pulmonary fibrosis-related effects of sulforaphane and sulforaphane N-acetylcysteine. Pharmaceutics, 13(7), 958. https://doi.org/10.3390/pharmaceutics13070958
- Fontana, P., Zufferey, A., Daali, Y., & Reny, J. L. (2014). Antiplatelet therapy: Targeting the TxA2 pathway. Journal of Cardiovascular Translational Research, 7(1), 29–38. https://doi.org/10.1007/s12265-013-9529-1
- Fuentes, E., Badimon, L., Caballero, J., Padro, T., Vilahur, G., Alarcon, M., Perez, P., & Palomo, I. (2014). Protective mechanisms of adenosine 5′-monophosphate in platelet activation and thrombus formation. Thrombosis and Haemostasis, 111(3), 491–507. https://doi.org/10.1160/TH13-05-0386
- Gasper, A. V., Al-Janobi, A., Smith, J. A., Bacon, J. R., Fortun, P., Atherton, C., Taylor, M. A., Hawkey, C. J., Barrett, D. A., & Mithen, R. F. (2005). Glutathione S-transferase M1 polymorphism and metabolism of sulforaphane from standard and high-glucosinolate broccoli. The American Journal of Clinical Nutrition, 82(6), 1283–1291. https://doi.org/10.1093/ajcn/82.6.1283
- Gawaz, M., Langer, H., & May, A. E. (2005). Platelets in inflammation and atherogenesis. The Journal of Clinical Investigation, 115(12), 3378–3384. https://doi.org/10.1172/JCI27196
- Ghasemzadeh, M., & Hosseini, E. (2017). Platelet granule release is associated with reactive oxygen species generation during platelet storage: A direct link between platelet pro-inflammatory and oxidation states. Thrombosis Research, 156, 101–104. https://doi.org/10.1016/j.thromres.2017.06.016
- Gillespie, S., Holloway, P. M., Becker, F., Rauzi, F., Vital, S. A., Taylor, K. A., Stokes, K. Y., Emerson, M., & Gavins, F. N. E. (2018). The isothiocyanate sulforaphane modulates platelet function and protects against cerebral thrombotic dysfunction. British Journal of Pharmacology, 175(16), 3333–3346. https://doi.org/10.1111/bph.14368
- Jayakumar, T., Chen, W. F., Lu, W. J., Chou, D. S., Hsiao, G., Hsu, C. Y., Sheu, J. R., & Hsieh, C. Y. (2013). A novel antithrombotic effect of sulforaphane via activation of platelet adenylate cyclase: Ex vivo and in vivo studies. The Journal of Nutritional Biochemistry, 24(6), 1086–1095. https://doi.org/10.1016/j.jnutbio.2012.08.007
- Joshi, S., Banerjee, M., Zhang, J., Kesaraju, A., Pokrovskaya, I. D., Storrie, B., & Whiteheart, S. W. (2018). Alterations in platelet secretion differentially affect thrombosis and hemostasis. Blood Advances, 2(17), 2187–2198. https://doi.org/10.1182/bloodadvances.2018019166
- Leong, D. P., Joseph, P. G., McKee, M., Anand, S. S., Teo, K. K., Schwalm, J. D., & Yusuf, S. (2017). Reducing the global burden of cardiovascular disease, part 2: Prevention and treatment of cardiovascular disease. Circulation Research, 121(6), 695–710. https://doi.org/10.1161/CIRCRESAHA.117.311849
- Li, W., Ma, Y., Zhang, C., Chen, B., Zhang, X., Yu, X., Shuai, H., He, Q., & Ya, F. (2022). Tetrahydrocurcumin downregulates MAPKs/cPLA2 signaling and attenuates platelet thromboxane A2 generation, granule secretion, and thrombus growth. Thrombosis and Haemostasis, 122(5), 739–754. https://doi.org/10.1055/s-0041-1735192
- Liu, H., & Talalay, P. (2013). Relevance of anti-inflammatory and antioxidant activities of exemestane and synergism with sulforaphane for disease prevention. Proceedings of the National Academy of Sciences, 110(47), 19065–19070. https://doi.org/10.1073/pnas.1318247110
- Li, Z., Zhang, G., Le Breton, G. C., Gao, X., Malik, A. B., & Du, X. (2003). Two waves of platelet secretion induced by thromboxane A2 receptor and a critical role for phosphoinositide 3-kinases. The Journal of Biological Chemistry, 278(33), 30725–30731. https://doi.org/10.1074/jbc.M301838200
- Lucotti, S., Cerutti, C., Soyer, M., Gil-Bernabe, A. M., Gomes, A. L., Allen, P. D., Smart, S., Markelc, B., Watson, K., Armstrong, P. C., Mitchell, J. A., Warner, T. D., Ridley, A. J., & Muschel, R. J. (2019). Aspirin blocks formation of metastatic intravascular niches by inhibiting platelet-derived COX-1/thromboxane A2. The Journal of Clinical Investigation, 129(5), 1845–1862. https://doi.org/10.1172/JCI121985
- Martinez-Gonzalez, M. A., Gea, A., & Ruiz-Canela, M. (2019). The Mediterranean diet and cardiovascular health. Circulation Research, 124(5), 779–798. https://doi.org/10.1161/CIRCRESAHA.118.313348
- Moroi, A. J., & Watson, S. P. (2015). Impact of the PI3-kinase/akt pathway on ITAM and hemITAM receptors: Haemostasis, platelet activation and antithrombotic therapy. Biochemical Pharmacology, 94(3), 186–194. https://doi.org/10.1016/j.bcp.2015.02.004
- Mozaffarian, D. (2016). Dietary and policy priorities for cardiovascular disease, diabetes, and obesity: A comprehensive review. Circulation, 133(2), 187–225. https://doi.org/10.1161/CIRCULATIONAHA.115.018585
- Naik, M. U., Patel, P., Derstine, R., Turaga, R., Chen, X., Golla, K., Neeves, K. B., Ichijo, H., & Naik, U. P. (2017). Ask1 regulates murine platelet granule secretion, thromboxane A2 generation, and thrombus formation. Blood, 129(9), 1197–1209. https://doi.org/10.1182/blood-2016-07-729780
- Palabrica, T., Lobb, R., Furie, B. C., Aronovitz, M., Benjamin, C., Hsu, Y. M., Sajer, S. A., & Furie, B. (1992). Leukocyte accumulation promoting fibrin deposition is mediated in vivo by P-selectin on adherent platelets. Nature, 359(6398), 848–851. https://doi.org/10.1038/359848a0
- Patel, P., & Naik, U. P. (2020). Platelet MAPKs—a 20+ year history: What do we really know? Journal of Thrombosis and Haemostasis, 18(9), 2087–2102. https://doi.org/10.1111/jth.14967
- Perrella, G., Nagy, M., Watson, S. P., & Heemskerk, J. W. M. (2021). Platelet GPVI (glycoprotein VI) and thrombotic complications in the venous system. Arteriosclerosis, Thrombosis, and Vascular Biology, 41(11), 2681–2692. https://doi.org/10.1161/ATVBAHA.121.316108
- Qi, Z., Hu, L., Zhang, J., Yang, W., Liu, X., Jia, D., Yao, Z., Chang, L., Pan, G., Zhong, H., Luo, X., Yao, K., Sun, A., Qian, J., Ding, Z., & Ge, J. (2021). PCSK9 (proprotein convertase subtilisin/kexin 9) enhances platelet activation, thrombosis, and myocardial infarct expansion by binding to platelet CD36. Circulation, 143(1), 45–61. https://doi.org/10.1161/CIRCULATIONAHA.120.046290
- Rayes, J., Watson, S. P., & Nieswandt, B. (2019). Functional significance of the platelet immune receptors GPVI and CLEC-2. The Journal of Clinical Investigation, 129(1), 12–23. https://doi.org/10.1172/JCI122955
- Russo, M., Spagnuolo, C., Russo, G. L., Skalicka-Wozniak, K., Daglia, M., Sobarzo-Sanchez, E., Nabavi, S. F., & Nabavi, S. M. (2018). Nrf2 targeting by sulforaphane: A potential therapy for cancer treatment. Critical Reviews in Food Science and Nutrition, 58(8), 1391–1405. https://doi.org/10.1080/10408398.2016.1259983
- Scarlett, G., Paul, M. H., Felix, B., Francesca, R., Shantel, A. V., Kirk, A. T., Karen, Y. S., Michael, E., & Felicity, N. G. (2018). The isothiocyanate sulforaphane modulates platelet function and protects against cerebral thrombotic dysfunction. British Journal of Pharmacology, 175(16), 3333–3346. https://doi.org/10.1111/bph.14368
- Vanduchova, A., Anzenbacher, P., & Anzenbacherova, E. (2019). Isothiocyanate from broccoli, sulforaphane, and its properties. Journal of Medicinal Food, 22(2), 121–126. https://doi.org/10.1089/jmf.2018.0024
- Violi, F., & Pignatelli, P. (2012). Platelet oxidative stress and thrombosis. Thrombosis Research, 129(3), 378–381. https://doi.org/10.1016/j.thromres.2011.12.002
- Wang, Y., Gallant, R. C., & Ni, H. (2016). Extracellular matrix proteins in the regulation of thrombus formation. Current Opinion in Hematology, 23(3), 280–287. https://doi.org/10.1097/MOH.0000000000000237
- Xu, X. R., Zhang, D., Oswald, B. E., Carrim, N., Wang, X., Hou, Y., Zhang, Q., Lavalle, C., McKeown, T., Marshall, A. H., & Ni, H. (2016). Platelets are versatile cells: New discoveries in hemostasis, thrombosis, immune responses, tumor metastasis and beyond. Critical Reviews in Clinical Laboratory Sciences, 53(6), 409–430. https://doi.org/10.1080/10408363.2016.1200008
- Ya, F., Li, K., Chen, H., Tian, Z., Fan, D., Shi, Y., Song, F., Xu, X., Ling, W., Adili, R., & Yang, Y. (2021). Protocatechuic acid protects platelets from apoptosis via inhibiting oxidative stress-mediated PI3K/Akt/GSK3β signaling. Thrombosis and Haemostasis, 121(7), 931–943. https://doi.org/10.1055/s-0040-1722621
- Ya, F., Tian, J., Li, Q., Chen, L., Ren, J., Zhao, Y., Wan, J., Ling, W., & Yang, Y. (2018). Cyanidin-3-O-β-glucoside, a natural polyphenol, exerts proapoptotic effects on activated platelets and enhances megakaryocytic proplatelet formation. Journal of Agricultural and Food Chemistry, 66(41), 10712–10720. https://doi.org/10.1021/acs.jafc.8b03266
- Ya, F., Xu, X. R., Shi, Y., Gallant, R. C., Song, F., Zuo, X., Zhao, Y., Tian, Z., Zhang, C., Xu, X., Ling, W., Ni, H., & Yang, Y. (2019). Coenzyme Q10 upregulates platelet cAMP/PKA pathway and attenuates integrin αiibβ3 signaling and thrombus growth. Molecular Nutrition & Food Research, 63(23), e1900662. https://doi.org/10.1002/mnfr.201900662
- Ya, F., Xu, X. R., Tian, Z., Gallant, R. C., Song, F., Shi, Y., Wu, Y., Wan, J., Zhao, Y., Adili, R., Ling, W., Ni, H., & Yang, Y. (2020). Coenzyme Q10 attenuates platelet integrin αIIbβ3 signaling and platelet hyper-reactivity in ApoE-deficient mice. Food & Function, 11(1), 139–152. https://doi.org/10.1039/C9FO01686D
Appendices
Supporting Information file includes the data regarding the effects of SFN on platelet surface expression of CD62P and CD63 induced by 3 μg/mL collagen (Supplementary Appendix Figure S1), the effect of SFN on platelet granule secretion induced by thrombin (Supplementary Appendix Figure S2), the effect of SFN on phosphorylation of Syk, LAT, and PLCγ2 in human platelets stimulated by collagen (Supplementary Appendix Figure S3), and the effect of SFN on platelet surface CD62P expression in different incubation time (Supplementary Appendix Figure S4).