ABSTRACT
In this study, an immunocompromised rat model was established by long-term diet control and excessive swimming and treated with extracts of raw licorice (RL) and honey-roasted licorice (HL) to explore the immunomodulatory efficacy and gut microbiota composition with their metabolites. Pharmacological experiment confirmed that honey-roasting enhanced the ability of RL to regulate inflammatory cytokines and gut mucosal-layer homeostasis. The analysis of 16S rRNA sequences showed that both RL and HL regulated the balance of gut microbiota, but HL exhibited better effects than RL in regulating microbiota closely related to inflammatory cytokines (Coriobacteriaceae_UCG-002) as well as short-chain fatty acids-producing bacteria (Dubosiella and Ruminococcus). Moreover, HL improved the contents of butyrate and isobutyrate in feces more significantly than RL. In conclusion, the balance of gut microbiota and short-chain fatty acids might be one of the pathways that honey-roasting improve the immunomodulatory efficacy of licorice.
Abbreviations: ANOVA: analysis of variance; BCA: bicinchoninic acid; ELISA: enzyme-linked immunosorbent assay; HRP: horseradish peroxidase; LDA: the linear discriminant analysis; LEfSe: the linear discriminant analysis effect size; MS: mass spectrometry; OTU: operational taxonomic units; PBS: phosphate buffered saline; PCoA: principal coordinate analysis; PVDF: polyvinylidene fluoride; RIPA: radio immunoprecipitation assay; SCFAs: short-chain fatty acids;
1. Introduction
Licorice, derived from the roots and rhizomes of Glycyrrhiza uralensis Fisch, is a used widely as a food and medicine. Generally, the product exists in two forms, raw licorice (RL) and honey-roasted licorice (HL) (Sun et al., Citation2010). Previous studies have investigated the changes in chemical composition after roasting with honey, such as the decomposition of glycosides into aglycones and sugars, the isomerization of flavonoids, and the increase in polysaccharide content (Kong et al., Citation2022; Ota et al., Citation2018; M. Wang et al., Citation2012). Pharmacological studies have shown that the immune activity of HL was enhanced compared to that of RL (Kong et al., Citation2022; Ota & Makino, Citation2022; Ota et al., Citation2018). Recent research has indicated that the biological activities of medicinal plants might be fortified by honey due to the synergism between licorice and honey (Kong et al., Citation2022).
In recent years, immunological research has evolved from a view of the immune system centered on lymphoid tissue to an understanding of the tissue microenvironment as a fundamental determinant of the immune response (Erttmann et al., Citation2022). Many modern studies have shown a strong correlation between the gut microbiota, the host’s immunity, and intestinal mucosal barrier permeability (Alemao et al., Citation2021). Gut microbiota not only directly influence the host immune function status, but also regulate intestinal epithelial cells and a variety of immune cell functions through the recognition of microbial metabolites and fermentation products, thus playing a key role in the homeostasis of the body’s immune function (Agus et al., Citation2018; Koh et al., Citation2016). Short-chain fatty acids (SCFAs), important intestinal metabolites, are produced from undigested carbohydrates (e. g. polysaccharides) and include acetate, propionate, butyrate, isobutyrate, 2-methyl-butyrate, valerate, and isovalerate. They can act on the gut epithelium, modulating downstream signaling pathways involved in the control of intestinal and systemic immune functions. (Koh et al., Citation2016; Nie et al., Citation2017; Topping & Clifton, Citation2001).
Recently, some studies have reported that both licorice water extraction (Shi et al., Citation2022) and licorice ethanol extract (Liu et al., Citation2022) can modulate the composition of gut microbiota. However, the underlying mechanism of the enhanced immunomodulatory efficacy after licorice honey-roasting is unclear whether it is linked to the regulation of gut microbiota and related metabolites such as SCFAs.
In this study, we evaluated inflammatory cytokines and intestinal barrier function following ingestion of the products and further investigated the gut microbiota and SCFAs using 16S rRNA and liquid chromatography-tandem mass spectrometry (LC-MS/MS), respectively. This study focused on comparing the the differences in the effects of raw licorice (RL) and honey-roasted licorice (HL) on the gut microbiota and their metabolites short-chain fatty acids.
2. Materials and methods
2.1. Sample preparation
2.1.1. Chemicals and reagents
RL were purchased from the Licorice Branch of the Inner Mongolia Yi Li Resources Group Co., Ltd. (Inner Mongolia, China) and identified by Associate Professor Hui Yan as the roots and rhizomes of Glycyrrhiza uralensis Fisch. Honey was purchased from Beijing Tongrentang Bee Products Co. Ltd. (Zhejiang, China). Sijunzi Keli (Lot: 202004002) was purchased from Lishizhen Pharmaceutical Co., Ltd. (Hubei, China). 3-nitrophenylhydrazine-HCl (3-NPH) (>98%) and N-(3-dimethylaminopropyl)-N-ethylcarbodiimide-HCl (EDC) (>98%) were purchased from Sigma-Aldrich (St. Louis, MO, U.S.A). SCFAs (acetate, propionate, butyrate, 2-methyl-butyrate, isobutyrate, valerate, and isovalerate) and the internal standard (IS, hexanoic acid-d3) were purchased from Sigma-Aldrich (St. Louis, MO, U.S.A).
2.1.2. Preparation of HL and extracts
The licorice samples comprised RL (unprocessed) and HL. The processing methods were as follows: RL was soaked in honey, with a mass fraction of one-fourth of the RL, and diluted with a small amount of water for 12 h at room temperature, then stir-fried on a stove at 110°C until the surfaces of the slices were dark yellow and not sticky. The slices were then removed, cooled, and weighed. The methods used to honey-roast licorice are described in the 2020 version of the “People’s Republic of China Pharmacopoeia” (Provision No. 0213).
Extract preparation: Each of the licorice products (equivalent to 120 g of RL) was refluxed with water three times (3 × 1,000 mL for 2 h each time). The filtrates were mixed and concentrated by rotary evaporation to produce extracts at a concentration of 0.9 g crude drug/mL. These were stored at −80°C before use. Extraction was carried out using a method similar to that for licorice extraction in the Chinese Pharmacopeia (China Pharmacopoeia Committee, Citation2020).
2.2. Immunological experiment
2.2.1. Animals and experimental design
Wistar rats (male, 180–220 g, 8 weeks old) were purchased from Shanghai Shilaike Laboratory Animal Co., Ltd., Shanghai, China and maintained in the animal house of Nanjing University of Chinese Medicine at room temperature (20–25°C) and constant relative humidity under a 12-h light/12-h dark cycle. Before the experiment, the rats were housed under laboratory conditions for 7 d to adapt to the new environment, during which they were allowed access to food and water ad libitum. All animal experiments were approved by the Animal Care and Use Committee of Nanjing University of Chinese Medicine. The rats were randomly divided into the control group, model group, positive group (4.73 g/kg Sijunzi Keli), high dose RL (1.8 g/kg) group, low dose RL (0.6 g/kg) group, high dose HL (1.8 g/kg RL equivalent) group, and low dose HL (0.6 g/kg RL equivalent) group. Rats in groups 2–7 were placed in a 31 cm diameter, 48 cm deep glass cylinder at 37 ± 1°C (the tank diameter and water depth were adjusted visually according to the size of the rat) and forced to swim until exhausted. They received 75 g/kg body weight of feed every other day to induce immunocompromise. Two weeks later, the rats in the control group were administered (i.g.) sterile physiological saline once daily for 2 weeks. The rats in the other group were orally administered decoctions at 10.0 mL/kg body weight once daily for 2 weeks.
Twenty-four hours after the final administration, rats were anesthetized using sodium pentobarbital by intraperitoneal injection. Blood samples were obtained from the abdominal aorta, and the rats were sacrificed. Then, ileum tissue and fecal material were collected.
2.2.2. Histopathological study
Ileum tissue was fixed in 10% neutral formalin and paraffin-embedded, and sections were HE-stained. Image acquisition was performed using microscopy under a 100× objective, and the length and villous interval of the intestinal villi were observed for histopathology analysis.
2.2.3. ELISA measurement
Blood samples were centrifuged at 4°C for 10 min at 3,500 × g to separate the sera, and the pro-inflammatory (TNF-α, IL-2, IL-6) and anti-inflammatory (IL-4) cytokines contained in the sera were determined by ELISA (Nanjing Lapuda Biotechnology Co., Ltd).
2.2.4. Western blotting
A tissue homogenate was made by homogenizing 100 mg of ileum with 1 mL of RIPA lysate on ice and then centrifuging at 12,000 × g for 20 min. The supernatant was collected and the total protein concentration was determined using a BCA Protein Assay Kit (Thermo Fisher Scientific, 23227). Protein samples (50 μg) and equal volume of 2× Laemmli buffer were heated at 95°C for 5 min. Samples were run on polyacrylamide gels and then transferred to a PVDF membrane under 90 V for 2 h using the wet transfer method. The membrane was covered in 5% skim milk for 2 h at room temperature and then incubated overnight at 4°C with primary antibodies. The following primary antibodies were used: anti-claudin-2 (Affbiotech, AF0128, 1: 2, 000), anti-occludin (Proteintech Group, Inc, Rosemont, IL, U.S.A; 27260–1-AP, 1: 5, 000), and anti-β-actin (Proteintech, 20536–1-AP, 1: 5, 000). Subsequently, the samples were incubated with horseradish peroxidase (HRP)-labeled anti-rabbit IgG (Proteintech, SA00001–2, 1: 20, 000) for 4 h. Finally, the protein bands were visualized using enhanced chemiluminescence (Shanghai Tianneng, 180–5001), and the grayscale values of the bands were calculated using ImageJ software. The grayscale values of each group of proteins of interest were divided by the grayscale values of the internal references (β-actin) to obtain the corresponding relative expression amounts.
2.3. The gut microbial community diversity
Based on the results of immune experiments, we conducted follow-up studies on a control group, mode group, high dose RL group and high dose HL group. Total microbial genomic DNA was extracted from rat fecal samples using the E.Z.N.A.® soil DNA Kit (Omega Bio-tek, Norcross, GA, U.S.A) according to the manufacturer’s instructions. The quality and concentration of DNA were determined by 1.0% agarose gel electrophoresis and a Nano Drop® ND-2000 spectrophotometer (Thermo Scientific Inc., U.S.A), and the DNA samples were kept at −80°C prior to further use. The hypervariable region V3-V4 of the bacterial 16S rRNA genes were amplified with primer pairs 338F (5”-ACTCCTACGGGAGGCAGCAG-3‘) and 806 R (5’-GGACTACHVGGGTWTCTAAT-3”) using an ABI Gene Amp® 9700 PCR thermocycler (Applied Biotechnology, San Louis, Obispo, CA, U.S.A). PCR amplification conditions were as follows: initial denaturation at 95°C for 3 min, followed by 27 cycles of denaturing at 95°C for 30 s, annealing at 55°C for 30 s, extension at 72°C extension at a single extension at 72°C for 10 min, and end at 4°C for 10 min, and an amplified in triplicate.
2.4. Targeted metabolite analysis
Fresh feces were collected and stored at −80°C. SCFAs (acetate, propionate, butyrate, 2-methyl-butyrate, isobutyrate, valerate, and isovalerate) were extracted and quantified using a previously reported method with minor adjustments (Han et al., Citation2015). Briefly, 3-nitrophenylhydrazine (3-NPH) was employed for pre-analytical derivatization to convert SCFAs to their 3-nitrophenylhydrazones and deuterated analogs (hexanoic acid-d3) were used as internal standards. The fecal SCFAs concentration was determined by LC-MS/MS. The representative chromatogram is presented in Figure S1.
2.5. Statistical analysis
Statistical differences between groups were evaluated by one-way analysis of variance (ANOVA) in GraphPad Prism v. 8.0.2.
Bioinformatic analysis of the gut microbiota was carried out using the Majorbio Cloud platform (https://cloud.majorbio.com). The similarity among the microbial communities in different samples was determined by principal coordinate analysis (PCoA) based on Bray-Curtis dissimilarity using Vegan v2.5–3. The PERMANOVA test was used to assess the percentage of variation explained by the treatment along with its statistical significance using Vegan v2.5–3. The linear discriminant analysis (LDA) effect size (LEfSe) (http://huttenhower.sph.harvard.edu/LEfSe) was performed to identify the significantly abundant taxa (phylum to genera) of bacteria among the different groups. To better show differences in the gut microbiota, the relative abundance of the differential communities was normalized by min-max normalization [x*=(x-min)/(max-min)] to generate a heatmap, where max is the maximum value of different samples in the same community and min is the minimum value of different samples of the same community.
3. Results
3.1 Effects of RL and HL on intestinal barrier function
To assess the potential effects of the two forms of licorice on intestinal barrier integrity, the gut morphological structure and barrier-associated protein expressions in the ileum were measured.
The results of intestinal histological hematoxylin & eosin (H&E) staining are shown in . H&E staining in the model group revealed a serious intestinal mucosal injury, short and thin villi, wider villi spacing, necrosis and collapse of intestinal mucosal epithelial cells, infiltration by inflammatory cells, gap formation beneath the epithelium, lamina propria edema, and capillary hemorrhage. However, a comparison between the model and the high dose RL/HL-treated rats revealed a healthier formation of the intestinal mucosa. Neutrophil infiltration in the intestinal mucosa was negligible, the expansion of the central lacteals was mild, and the intestinal mucosa was relatively complete. In addition, there were a few collapsed intestinal epithelial cells and mild hyperemia as well as edema under the epithelial cells after high-dose HL treatment.
Figure 1. The improvement of intestinal mucosal permeability. (a) Effects on small intestinal villi; (b) Effects on intestinal tight junction proteins in the intestinal epithelium. C, control group; M, model group; P, positive group; R-l, raw licorice low dose group; R-h, raw licorice high dose group; H-l, honey-roasted licorice low-dose group; H-h, honey-roasted licorice high-dose group. *p < .05, **p < .01 ***p < .001 vs. M; #p < .05, ##p < .01, ###p < .001 R-l vs. H-l, R-h vs. H-h (n = 3).
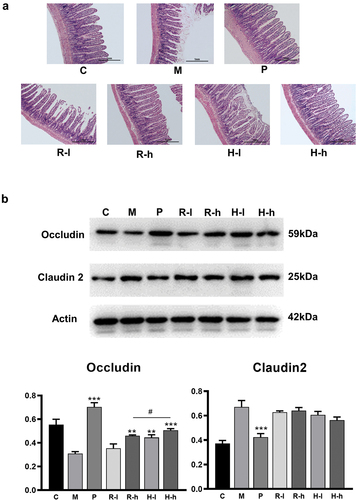
Western blot assays for intercellular tight junction-related proteins () exhibited that the levels of occludin and claudin 2 were dramatically altered in the ileum of the model rats (p < .001). The application of the positive drug, low and high doses of HL, and a high dose of RL, significantly increased the occludin levels (p < .01, p < .001) compared to the model group. Of note, the high dose of HL showed a stronger effect than the same dose of RL, and the difference between the two groups was significant (p < .05). However, as shown in , both RL and HL showed no effect on claudin 2.
3.2 Effects of RL/HL on inflammatory cytokine expression
shows that serum IL-2, IL-6, and TNF-α secretion levels were significantly higher and the serum IL-4 secretion level was significantly lower in the model (M) group, compared with the control (C) group. Licorice administration significantly restored these alterations, except for the IL-2 secretion level in the low-dose RL (R-l) group. Among them, the low dose HL (H-l) group showed improved levels of IL-2, IL-6, and TNF-α, exhibiting significant differences compared to the R-l group (p < .05, p < .01). In addition, the high-dose of HL (H-h) treatment resulted in a stronger recovery of IL-2, IL-6, IL-4, and TNF-α than the treatments using the RL extracts (p < .05, p < .01).
Figure 2. The improvement of immune function. C, control group; M, model group; P, positive group; R-l, raw licorice low-dose group; R-h, raw licorice high dose group; H-l, honey-roasted licorice low dose group; H-h, honey-roasted licorice high dose group. *p < .05, **p < .01, ***p < .001 vs. M; #p < .05, ##p < .01, ###p < .001 R-l vs. H-l, R-h vs. H-h (n = 6).
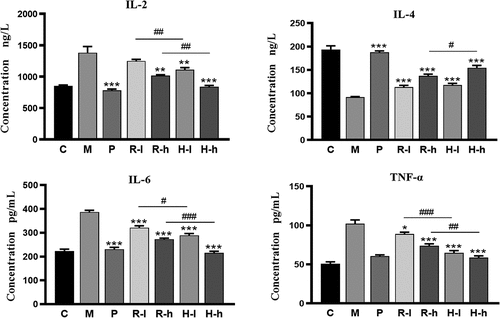
3.3 Effects of RL/HL extracts on gut microbiota composition
To further explore the effects of RL and HL extracts on gut microbiota composition, 16S rRNA pyrosequencing based on the V3-V4 region was performed. Based on the aforementioned study results, four representative groups, including the C, M, high-dose of RL (R-h), and H-h groups were compared as follows. A principal coordinates analysis (PCoA) based on a phylogenetic assay indicated a relative clustering of gut microbiota within each group () and showed clear separation between the groups. The microbial community structure at the phylum level was dominated by Firmicutes and Actinobacteriota. Compared with the C group, Actinobacteriota elevation remained evident in the experimental M group, whereas rats treated with R-h and H-h exhibited significantly reduced Actinobacteriota elevation compared with the M group. On the contrary, Firmicutes was significantly increased after RL and HL administration. Interestingly, recovery was more obvious in the H-h group than the R-h group ().
Figure 3. The overall structure of the gut microbiota. (a) PCoA on OUT level of different groups (n = 6). (b) the relative abundance at the phylum level. C, control group; M, model group; R-h, raw licorice high dose group; H-h, honey-roasted licorice high dose group.
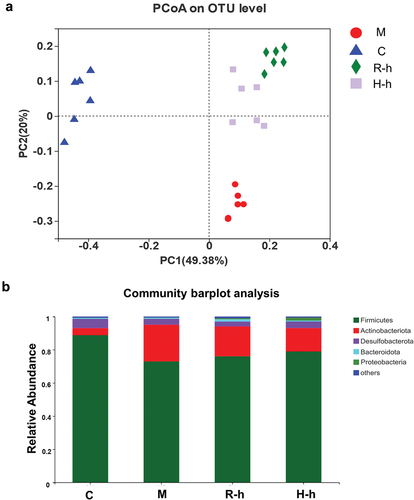
LEfSe analysis (LDA> 3.5, p < .05) found that 59 taxa with different taxonomic levels showed significant differences in the C group, M group, R-h group, and H-h group (). To better visualize gut microbiota differences, the relative abundance of 24 communities at the genus level were normalized to generate a heat map (). It showed a reduced relative abundance of seven communities and an increased relative abundance of 12 communities, which were dominated by Clostridia and Bacilli, after the controlled diet and excessive swimming. After administration of RL and HL, the imbalance of gut microbiota effectively improved, especially following consumption of HL, which clearly reversed the relative abundance of 12 of these communities.
Figure 4. The composition of gut microbiota (n = 6). (a) Cladogram generated from LEfSe analysis for C, M, R-h, and H-h groups; nonparametric factorial Kruskal-Wallis (KW) sum-rank test, LDA> 3.5, p < .05. (b) Heatmap of gut microbiota at the genus level based on LEfSe analysis results. (c) Group difference analysis of representative intestinal bacteria. (Compared to the M group, *p < .05, **p < .01. Comparison of R-h group and H-h group, #p < .05, ##p < .01). C, control group; M, model group; R-h, raw licorice high-dose group; H-h, honey-roasted licorice high-dose group.
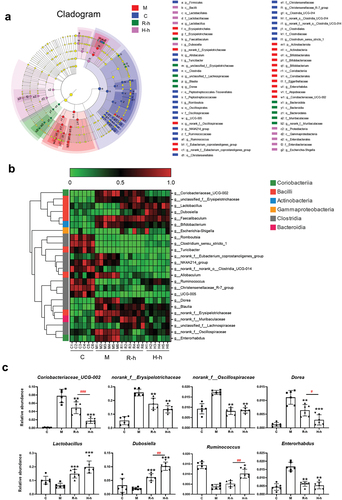
Among them, the regulatory effects of RL and HL on some bacteria were of great concern as shown in . The findings exhibited that the relative abundance of norank_f__Erysipelotrichaceae, Coriobacteriaceae_UCG-002, Dore, norank_f__Oscillospiraceae, Enterorhabdus, and Ruminococcus were reversed in the R-h and H-h groups. Although there was no significant difference between groups C and M, the relative abundance of Lactobacillus and Dubosiella was significantly increased after RL/HL intervention. Of these, the regulatory effect of the HL intervention on Dubosiella, Dorea, and Coriobacteriaceae_UCG-002 was more obvious than that of the RL intervention. At the same time, we also noticed a significant increase in the relative abundance of SCFA-producing bacteria such as Lactobacillus, Dubosiella, and Ruminococcus after RL/HL intervention ().
The above results showed that the RL/HL intervention had the effect of regulating the gut microbiota, and the regulatory effects of HL on some communities were better than those of RL.
3.4 Effects of RL/HL extracts on SCFAs metabolism
SCFAs are recognized as the key microbial metabolites in the gut, which exert multiple beneficial effects on the host. Considering the function of SCFAs on the immune response, the acetate, propionate, butyrate, isobutyrate, 2-methyl-butyrate, valerate, and isovalerate levels were measured in this study. As illustrated in , the SCFAs concentrations were markedly decreased in the immunocompromise model group compared with the control group (p < .001), indicating that the metabolic activity of the gut microbiota was unbalanced and impaired. After treatment with high-dose RL/HL extracts, acetate and propionate were significantly increased. However, treatment with RL or HL did not exhibit obvious effects in valerate, isovalerate, or 2-methyl-butyrate. Of note, it is apparent that the two types of licorice were distinct with regard to the regulation of butyrate and isobutyrate. The effect of H-h on improving butyrate and isobutyrate levels was stronger than that of R-h (p < .01).
4. Discussion
The intestine is the largest organ associated with the immune system and plays an extremely important role in maintaining the host′s normal immune defense functions. In this study, we investigated the mechanism of the immuno-enhancing effects of HL from the perspective of gut microbiota and their SCFAs metabolites. HL demonstrated better improvement of gut microbiota disorders at the phylum level, suggesting that HL could enhance host immune function by regulating gut microbiota. Further LefSe analysis found that treatment with RL/HL could reverse the increase in the relative abundance of the communities closely related to inflammatory cytokines such as norank_f__Erysipelotrichaceae and Coriobacteriaceae_ UCG-002 (Figure S2) and some harmful gut bacteria such as Dore (Jinato et al., Citation2022), norank_f__Oscillospiraceae (Yan et al., Citation2022) and Enterorhabdus (Xu et al., Citation2022). Of these, norank_f__Oscillospiraceae belongs to the Oscillospiraceae family, an opportunistic pathogenic gut bacterium associated with inflammation (K. Wang et al., Citation2022). Oscillospiraceae can promote intestinal barrier disruption and induce systemic inflammatory responses (Raimondi et al., Citation2021). Meanwhile, HL intervention modulated some important probiotics such as Lactobacillus, Dubosiella, and Ruminococcus, resulting in a significant increase in their abundance. Interestingly, six communities containing members of the Erysipelotrichaceae family exhibited significant differences, including Faecalibaculum, Dubosiella, norank_f__Erysipelotrichaceae, Allobaculum, Turicibacter, and unclassified_f__Erysipelotrichaceae (), which deserve more attention in future studies.
SCFAs are thought to be key bacterial metabolites in the intestinal immune response and in regulating the permeability of the intestinal mucosal barrier (Tan et al., Citation2014). Modern research has proven that SCFAs regulate intestinal pH to inhibit the growth of harmful bacteria (Gui & Shen, Citation2016; Lu et al., Citation2015) and participate in a variety of pathways such as those involved with barrier function (Kelly et al., Citation2015) and the immune response (Brown et al., Citation2003; Zimmerman et al., Citation2012) to reduce intestinal inflammation. In this study, both RL and HL significantly increased the levels of acetate and propionate, while HL exhibited a more striking effect than RL in modulating the levels of butyrate and isobutyrate. This may be due to the actions of RL/HL in regulating SCFAs-producing bacteria in the intestinal tract. Among them, Lactobacillus was found to be the dominant genus of the gut microbiota, which is involved in adsorption defense, promoting repair, and regulating SCFAs including lactate, acetate, and propionate, etc. (Ai et al., Citation2021). Further analysis showed that the relative abundance of Lactobacillus in groups treated with RL or HL was obviously higher than that of the model group. In addition, the relative abundance of Dubosiella increased dramatically after treatment with RL or HL. Of note, Dubosiella in the H-h group was significantly higher than that in the R-h group. The Dubosiella genus is a commensal saccharolytic bacteria that produces butyrate and has been proposed in human medicine as probiotic for the restoration of beneficial bacteria (Qiu et al., Citation2021; Rodríguez-Daza et al., Citation2020; Wan et al., Citation2021). This suggested that Dubosiella may be another important community increased by HL to improve the immunomodulatory ability through the SCFAs pathway. By regulating the balance of gut microbiota and metabolic disorders associated with SCFAs, the host′s immune factors and intestinal mucosal permeability may be improved significantly, which might be an important mechanism by which has an immunomodulatory efficacy.
Physical and chemical variations during herb processing are the basis for the potency of their effects. Previous studies have indicated that isoliquiritin may increase the abundance of lymphocytes, macrophages, and the CD4+/CD8+ ratio, improving human immunity (Martínez et al., Citation2019); glabridin, an isoflavonoid, has an anti-inflammatory and analgesic action (Parlar et al., Citation2020, Citation2022). And isoliquiritin has also the capacity to regulate the gut microbiota (Wu et al., Citation2016). These researches implied that the isomerization of flavonoid components after honey roasting may be one of the important mechanisms by which honey-roasting enhances the immunomodulatory ability of licorice. Moreover, polysaccharides are potential prebiotics that can provide a beneficial growth environment for probiotics in the intestinal tract. Additionally, indigestible polysaccharides are the raw material for microbial fermentation to produce SCFAs (Goldsmith & Sartor, Citation2014). Recent studies have confirmed that TCM polysaccharide intervention can modulated the gut microbiota community close to that of normal rat and increase the beneficial SCFAs-producing bacteria such as Lactobacillus and Dubosiella (Lai et al., Citation2022). Considering the structural features and function characteristics of polysaccharides, it can be speculated that these compounds are the most predominant components of HL that improve immune regulation through the gut microbiota and SCFAs pathway.
4. Conclusions
In conclusion, this study is the first to explore the enhancement of immunomodulatory effect of licorice after honey-roasting from the perspective of gut microbiota and fecal metabolomics and results demonstrated that the improved immune efficacy was mainly due to the regulation of gut microbiota balance, the promotion of the abundance of probiotics (Dubosiella) and the related metabolites of SCFAs such as acetic acid, propionic acid, and butyric acid. These findings provided a theoretical and empirical basis for processing of licorice. A follow-up studies would be needed to explore which component could achieve this efficacy.
Author contributions
Conceptualization, X.L. and J.S.; methodology, J.S.; software, X.L.; validation, X.L., J.S. and Y.W.; data curation, C.L.; writing – original draft preparation, X.L.; writing – review and editing, Y.Z.; visualization, Y.W.; supervision, C.L. and G.P.; project administration, Y.Z.; funding acquisition, Y.Z.
All authors have read and agreed to the published version of the manuscript.
Animal ethics
The study was approved by the Animal Ethics Committee of Nanjing University of Chinese Medicine (202104A054).
Supplemental Material
Download MS Word (146.6 KB)Acknowledgements
We thank Shanghai Majorbio Biopharm Technology Co., Ltd. for sequencing and help with bioinformatic analysis. Thank to International Science Editing (http://www.internationalscienceediting.com) for editing this manuscript.
Disclosure statement
No potential conflict of interest was reported by the author(s).
Data availability statement
The datasets used and/or analyzed during the current study are available from the corresponding author on reasonable request.
Supplementary material
Supplemental data for this article can be accessed online at https://doi.org/10.1080/19476337.2023.2193605
Additional information
Funding
References
- Agus, A., Planchais, J., & Sokol, H. (2018). Gut microbiota regulation of tryptophan metabolism in health and disease. Cell Host & Microbe, 23(6), 716–724. Cell Press. https://doi.org/10.1016/j.chom.2018.05.003
- Ai, X., Wu, C., Yin, T., Zhur, O., Liu, C., Yan, X., Yi, C. P., Liu, D., Xiao, L., Li, W., Xie, B., & He, H. (2021). Antidiabetic function of lactobacillus fermentum MF423-fermented rice bran and its effect on gut microbiota structure in type 2 diabetic mice. Frontiers in Microbiology, 12. https://doi.org/10.3389/fmicb.2021.682290
- Alemao, C. A., Budden, K. F., Gomez, H. M., Rehman, S. F., Marshall, J. E., Shukla, S. D., Donovan, C., Forster, S. C., Yang, I. A., Keely, S., Mann, E. R., el Omar, E. M., Belz, G. T., & Hansbro, P. M. (2021). Impact of diet and the bacterial microbiome on the mucous barrier and immune disorders. Allergy: European Journal of Allergy and Clinical Immunology, 76(3), 714–734. Blackwell Publishing Ltd. https://doi.org/10.1111/all.14548
- Brown, A. J., Goldsworthy, S. M., Barnes, A. A., Eilert, M. M., Tcheang, L., Daniels, D., Muir, A. I., Wigglesworth, M. J., Kinghorn, I., Fraser, N. J., Pike, N. B., Strum, J. C., Steplewski, K. M., Murdock, P. R., Holder, J. C., Marshall, F. H., Szekeres, P. G., Wilson, S., Ignar, D. M., & Dowell, S. J. (2003). The orphan G protein-coupled receptors GPR41 and GPR43 are activated by propionate and other short chain carboxylic acids. The Journal of Biological Chemistry, 278(13), 11312–11319. https://doi.org/10.1074/jbc.M211609200
- China Pharmacopoeia Committee. (2020). Pharmacopoeia of people’s republic of China, IV. China Medical Science Press.
- Erttmann, S. F., Swacha, P., Aung, K. M., Brindefalk, B., Jiang, H., Härtlova, A., Uhlin, B. E., Wai, S. N., & Gekara, N. O. (2022). The gut microbiota prime systemic antiviral immunity via the cGAS-STING-IFN-I axis. Immunity, 55(5), 847–861.e10. https://doi.org/10.1016/j.immuni.2022.04.006
- Goldsmith, J. R., & Sartor, R. B. (2014). The role of diet on intestinal microbiota metabolism: Downstream impacts on host immune function and health, and therapeutic implications. Journal of Gastroenterology, 49(5), 785–798. Springer-Verlag Tokyo. https://doi.org/10.1007/s00535-014-0953-z
- Gui, H., & Shen, Z. (2016). Concentrate diet modulation of ruminal genes involved in cell proliferation and apoptosis is related to combined effects of short-chain fatty acid and pH in rumen of goats. Journal of Dairy Science, 99(8), 6627–6638. https://doi.org/10.3168/jds.2015-10446
- Han, J., Lin, K., Sequeira, C., & Borchers, C. H. (2015). An isotope-labeled chemical derivatization method for the quantitation of short-chain fatty acids in human feces by liquid chromatography-tandem mass spectrometry. Analytica chimica acta, 854, 86–94. https://doi.org/10.1016/j.aca.2014.11.015
- Jinato, T., Chayanupatkul, M., Dissayabutra, T., Chutaputti, A., Tangkijvanich, P., & Chuaypen, N. (2022). Litchi-derived polyphenol alleviates liver steatosis and gut dysbiosis in patients with non-alcoholic fatty liver disease: A randomized double-blinded, placebo-controlled study. Nutrients, 14(14), 2921. https://doi.org/10.3390/nu14142921
- Kelly, C. J., Zheng, L., Campbell, E. L., Saeedi, B., Scholz, C. C., Bayless, A. J., Wilson, K. E., Glover, L. E., Kominsky, D. J., Magnuson, A., Weir, T. L., Ehrentraut, S. F., Pickel, C., Kuhn, K. A., Lanis, J. M., Nguyen, V., Taylor, C. T., & Colgan, S. P. (2015). Crosstalk between microbiota-derived short-chain fatty acids and intestinal epithelial HIF augments tissue barrier function. Cell Host & Microbe, 17(5), 662–671. https://doi.org/10.1016/j.chom.2015.03.005
- Koh, A., de Vadder, F., Kovatcheva Datchary, P., & Bäckhed, F. (2016). From dietary fiber to host physiology: Short-chain fatty acids as key bacterial metabolites. Cell, 165(6), 1332–1345. Cell Press. https://doi.org/10.1016/j.cell.2016.05.041
- Kong, S., Li, P., Verpoorte, R., Wang, J., Zhu, C., Dai, Y., & Chen, S. (2022). Synergistic mechanism for the bioactivity fortification of licorice by honey. Journal of Ethnopharmacology, 289, 115048. https://doi.org/10.1016/j.jep.2022.115048
- Lai, W., Wang, C., Lai, R., Peng, X., & Luo, J. (2022). Lycium barbarum polysaccharide modulates gut microbiota to alleviate rheumatoid arthritis in a rat model. Npj Science of Food, 6(1), 34. https://doi.org/10.1038/s41538-022-00149-z
- Liu, F., Tang, X., Mao, B., Zhang, Q., Zhao, J., Cui, S., & Chen, W. (2022). Ethanol extract of licorice alleviates HFD-Induced liver fat accumulation in association with modulation of gut microbiota and intestinal metabolites in obesity mice. Nutrients, 14(19), 4180. https://doi.org/10.3390/nu14194180
- Lu, Z., Gui, H., Yao, L., Yan, L., Martens, H., Aschenbach, J. R., & Shen, Z. (2015). Short-chain fatty acids and acidic pH upregulate UT-B, GPR41, and GPR4 in rumen epithelial cells of goats. American Journal of Physiology-Regulatory, Integrative and Comparative Physiology, 308(4), 283–293. https://doi.org/10.1152/ajpregu.00323.2014.-Currently
- Martínez, G., Mijares, M. R., & de Sanctis, J. B. (2019). Effects of flavonoids and its derivatives on immune cell responses. Recent Patents on Inflammation & Allergy Drug Discovery, 13(2), 84–104. https://doi.org/10.2174/1872213x13666190426164124
- Nie, Y., Lin, Q., & Luo, F. (2017). Effects of non-starch polysaccharides on inflammatory bowel disease. International Journal of Molecular Sciences, 18(7), 1372. MDPI AG. https://doi.org/10.3390/ijms18071372
- Ota, M., & Makino, T. (2022). History and the immunostimulatory effects of heat-processed licorice root products with or without honey. Journal of Ethnopharmacology, 292, 115108. https://doi.org/10.1016/j.jep.2022.115108
- Ota, M., Nagachi, Y., Ishiuchi, K., Tabuchi, Y., Xu, F., Shang, M. Y., Cai, S. Q., & Makino, T. (2018). Comparison of the inducible effects of licorice products with or without heat-processing and pre-treatment with honey on granulocyte colony-stimulating factor secretion in cultured enterocytes. Journal of Ethnopharmacology, 214, 1–7. https://doi.org/10.1016/j.jep.2017.11.040
- Parlar, A., Arslan, S. O., & Çam, S. A. (2020). Glabridin alleviates inflammation and nociception in rodents by activating BKCa channels and reducing NO levels. Biological & Pharmaceutical Bulletin, 43(5), 884–897. https://doi.org/10.1248/BPB.B20-00038
- Parlar, A., Köprülü, R. E. P., Arslan, S. O., Çam, S. A., Özdoğan, F. P., Yumrutaş, Ö., Üçkardeş, F., Ríos, M., & Martinez, J. L. (2022). Extract of Glycyrrhiza glabra root attenuates nociception in experimental pain models: The role of BKCa channels. Boletin Latinoamericano y Del Caribe de Plantas Medicinales y Aromaticas, 21(4), 464–484. https://doi.org/10.37360/blacpma.22.21.4.29
- Qiu, X., Macchietto, M. G., Liu, X., Lu, Y., Ma, Y., Guo, H., Saqui-Salces, M., Bernlohr, D. A., Chen, C., Shen, S., & Chen, X. (2021). Identification of gut microbiota and microbial metabolites regulated by an antimicrobial peptide lipocalin 2 in high fat diet-induced obesity. International Journal of Obesity, 45(1), 143–154. https://doi.org/10.1038/s41366-020-00712-2
- Raimondi, S., Musmeci, E., Candeliere, F., Amaretti, A., & Rossi, M. (2021). Identification of mucin degraders of the human gut microbiota. Scientific Reports, 11(1). https://doi.org/10.1038/s41598-021-90553-4
- Rodríguez-Daza, M. C., Roquim, M., Dudonné, S., Pilon, G., Levy, E., Marette, A., Roy, D., & Desjardins, Y. (2020). Berry polyphenols and fibers modulate distinct microbial metabolic functions and gut microbiota enterotype-like clustering in obese mice. Frontiers in Microbiology, 11. https://doi.org/10.3389/fmicb.2020.02032
- Shi, G., Kong, J., Wang, Y., Xuan, Z., & Xu, F. (2022). Glycyrrhiza uralensis Fisch. Alleviates dextran sulfate sodium-induced colitis in mice through inhibiting of NF-κB signaling pathways and modulating intestinal microbiota. Journal of Ethnopharmacology, 298, 115640. https://doi.org/10.1016/j.jep.2022.115640
- Sun, L. L., Zhan, T., Zhou, Q., Shi, D. H., Zhang, L. L., & Dai, Y. P. (2010). Overview of licorice processing methods in past dynasties. Chinese Traditional Patent Medicine, 7, 3. https://doi.org/10.3969/j.issn.1001-1528.2010.07.028
- Tan, J., McKenzie, C., Potamitis, M., Thorburn, A. N., Mackay, C. R., & Macia, L. (2014). The role of short-chain fatty acids in health and disease. Advances in Immunology, 121, 91–119. Academic Press Inc. https://doi.org/10.1016/B978-0-12-800100-4.00003-9
- Topping, D. L., & Clifton, P. M. (2001). Short-chain fatty acids and human colonic function: Roles of resistant starch and nonstarch polysaccharides. http://physrev.physiology.org
- Wan, F., Han, H., Zhong, R., Wang, M., Tang, S., Zhang, S., Hou, F., Yi, B., & Zhang, H. (2021). Dihydroquercetin supplement alleviates colonic inflammation potentially through improved gut microbiota community in mice. Food & Function, 12(22), 11420–11434. https://doi.org/10.1039/d1fo01422f
- Wang, K., Guo, J., Chang, X., & Gui, S. (2022). Painong-San extract alleviates dextran sulfate sodium-induced colitis in mice by modulating gut microbiota, restoring intestinal barrier function and attenuating TLR4/NF-κB signaling cascades. Journal of Pharmaceutical and Biomedical Analysis, 209, 114529. https://doi.org/10.1016/j.jpba.2021.114529
- Wang, M., Zhang, M., Tang, Q., & Li, X. (2012). Influence of honey-roasting on the main pharmacological activities and the water-soluble active glycosides of licorice. African Journal of Traditional, Complementary and Alternative Medicines, 9(2). https://doi.org/10.4314/ajtcam.v9i2.2
- Wu, M., Wu, Y., Deng, B., Li, J., Cao, H., Qu, Y., Qian, X., & Zhong, G. (2016). Isoliquiritigenin decreases the incidence of colitis-associated colorectal cancer by modulating the intestinal microbiota. Oncotarget, 7(51), 85318–85331. https://doi.org/10.18632/oncotarget.13347
- Xu, X., Liu, S., Zhao, Y., Wang, M., Hu, L., Li, W., & Xu, H. (2022). Combination of houttuynia cordata polysaccharide and lactiplantibacillus plantarum P101 alleviates acute liver injury by regulating gut microbiota in mice. Journal of the Science of Food and Agriculture, 102(15), 6848–6857. https://doi.org/10.1002/jsfa.12046
- Yan, S., Chen, J., Zhu, L., Guo, T., Qin, D., Hu, Z., Han, S., Zhou, Y., Akan, O. D., Wang, J., Luo, F., & Lin, Q. (2022). Oryzanol attenuates high fat and cholesterol diet-induced hyperlipidemia by regulating the gut microbiome and amino acid metabolism. Journal of Agricultural and Food Chemistry, 70(21), 6429–6443. https://doi.org/10.1021/acs.jafc.2c00885
- Zimmerman, M. A., Singh, N., Martin, P. M., Thangaraju, M., Ganapathy, V., Waller, J. L., Shi, H., Robertson, K. D., Munn, D. H., & Liu, K. (2012). Butyrate suppresses colonic inflammation through HDAC1-dependent fas upregulation and fas-mediated apoptosis of T cells. American Journal of Physiology - Gastrointestinal and Liver Physiology, 302(12), G1405–1415. https://doi.org/10.1152/ajpgi.00543.2011