ABSTRACT
To evaluate the effects of simvastatin on the degeneration of cartilage and subchondral bone in a mice model of osteoarthritis (OA) induced by obesity. Thirty male 3-month-old C57BL/6J mice were randomized into three groups: mice with normal diet + vehicle (distilled water) (Control), mice with High-fat diet + vehicle (HFD), mice with HFD + Simvastatin (HFD+S). HFD+S group were treated with simvastatin (10 mg/kg/day) for 12 weeks. The pathology of OA was assessed by histomorphology analyses, immunohistochemistry, micro-computed tomography, and enzyme-linked immunosorbent assay. Histomorphological analysis revealed that OA was significantly exacerbated by the HFD-induced obesity and markedly alleviated by the simvastatin intervention. In details, simvastatin ameliorated the abnormal metabolic status and cartilage lesions, significantly increased aggrecan and collagen-II expression and decreased the expression of MMP-13 and leptin in cartilage. Furthermore, the results of micro-CT analysis revealed that the HFD+S group exhibited higher BMD, BV/TV, and Tb.N values but a lower Tb.Sp value than that of the HFD group. Serum glucose, leptin, and IL-1β concentrations were significantly correlated with the OARSI score. Histomorphological analysis revealed that OA was significantly exacerbated by the HFD-induced obesity and markedly alleviated by the simvastatin intervention. In details, simvastatin ameliorated the abnormal metabolic status and cartilage lesions, significantly increased aggrecan and collagen-II expression and decreased the expression of MMP-13 and leptin in cartilage. Furthermore, the results of micro-CT analysis revealed that the HFD+S group exhibited higher BMD, BV/TV, and Tb.N values but a lower Tb.Sp value than that of the HFD group. Serum glucose, leptin, and IL-1β concentrations were significantly correlated with the OARSI score. The HFD-induced obesity aggravates articular degeneration and deterioration in subchondral bone, which could be improved by the intervention of simvastatin, suggesting that simvastatin may be a potential candidate for amelioration of the progression of obesity induced-OA.
Highlights
Obesity has certain influence on OA due to its induction effect of chronic inflammation and abnormal metabolic syndrome. In this study, we further studied the pathology of obesity induced OA in mice.
Simvastatin has potential therapeutic effects on OA. However, it is currently unknown whether the simvastatin intervention can amplify the remission of the pathological state of OA induced by obesity.
Our study confirmed for the first time that simvastatin intervention has a strong effect in protecting obese mice from joint damage.
Introduction
Osteoarthritis (OA) is a whole-joint disorder involving the cartilage, subchondral bone, synovium, ligament, and joint capsule. Aetiologies of this disease have not been fully elucidated, but epidemiological studies have shown that the incidence of OA in obese individuals is increased and is 33% more likely to require joint replacement surgery than individuals with a normal body mass (Bourne et al., Citation2007). The potential mechanism of obesity-related OA is complex and controversial (Wluka et al., Citation2013). One contributing factor appears to be overweight-associated mechanical overload, which has long been pointed out to explain the link between obesity and osteoarthritis. Related clinical studies also showed positive correlations between body mass index (BMI) and the onset or progression of knee OA (Reijman et al., Citation2007). In addition to abnormal loading, obesity-related systemic metabolic factors may play an important role in the pathological process of OA. Previous studies have shown that abnormal lipid metabolism has been associated with OA in non-weight bearing joints (such as the hands), which indicates that metabolic factors may also contribute to OA pathogenesis (Pottie et al., Citation2006). Besides, according to other studies, the elevation of leptin in mouse chondrocytes induced by high-fat diet also seems to be an important pathogenic factor (Datta et al., Citation2017). As far as we know, there is currently no targeted therapy for OA induced by obesity.
Simvastatin, a 3‐hydroxy‐3‐methylglutaryl coenzyme‐A reductase inhibitor, has been widely used in the initial treatment of hyperlipidemia and prevention of cardiovascular disease. Furthermore, simvastatin has been reported to have a protective effect on the cartilage in the knee joint, such as promoting the proliferation and inhibiting the apoptosis of chondrocytes (Zhou et al., Citation2017), stimulating bone formation (Fukui et al., Citation2012), and enhancing anti‐inflammatory effects (Sverdrup et al., Citation2010). Previous in vivo studies have shown that the application of statins reduces cartilage degradation in rabbits with experimental osteoarthritis and inhibits the expression of matrix metalloproteinase‐3 (MMP-3) in a mechanically induced knee osteoarthritis (Aktas et al., Citation2011; Goto et al., Citation2017). Moreover, in vitro experiments have shown that statins up-regulate the mRNA levels of bone morphogenetic protein-2 (BMP-2), aggrecan, collagen-II and increase the synthesis of proteoglycan in rat chondrocytes (Hatano et al., Citation2003). Simvastatin has also been found to inhibit the expression of leptin, but whether it can reduce the level of leptin in articular cartilage has not been studied (Datta et al., Citation2017). These findings suggest a possible effect of simvastatin for treating obesity-induced OA involving cartilage degeneration, subchondral bone loss, and metabolic disorders. The purpose of this study is to further explore the pathogenesis of OA induced by obesity in high-fat diet (HFD) mice and to investigate the therapeutic effects of simvastatin on knee OA.
Materials and methods
Animals and treatments
All experimental procedures were approved by the Institutional Animal Care and Use Committee. Thirty male 3-month-old C57BL/6J mice (Vital River Experimental Animal Technical Co., Ltd., China) were used in this research. All mice were randomized into three groups (n = 10/group): mice with normal diet + vehicle (distilled water) (Control), mice with High-fat diet + vehicle (HFD), mice with HFD + Simvastatin (HFD+S). Drug administration was initiated on the same day the HFD was given. Mice in the HFD+S group received oral gavage of Simvastatin (SL Pharmaceutical Co., Ltd., Beijing, China) at 10 mg/kg/day, other groups were given distilled water. After 12 weeks of intervention, all mice were euthanized to collect blood and samples. For details see Supplementary Materials.
Macroscopic observation
After all mice were euthanized, the abdominal subcutaneous fat was photographed with a Canon 550D digital camera (Canon, Tokyo, Japan).
Body weight, waist circumference, and body mass index (BMI)
Body weight was measured weekly, and waist circumference was measured before the macroscopic observation. The body mass index (BMI) was calculated using the formula: (Weight in kg/Body length in m2) (Jeyakumar et al., Citation2009). Body length was defined as the distance from the tip of the mouse’s nose to the anus.
Histological assessments
In the mice, perirenal adipose tissue and knee joints were harvested and sequentially fixed in 10% neutral-buffered formalin, dehydrated in a graded series of ethanols and embedded in paraffin wax (bone tissue needs decalcification), and finally cut into 5-μm sections. Adipose tissues were stained with hematoxylin and eosin (H&E) to observe the cross-sectional area of fat cells. Bone tissues sections were stained for light microscopy with H&E to evaluate the pathological changes in subchondral bone marrow fat cells, cartilage degradation was assessed using Safranin-O and fast green staining and scored according to the Osteoarthritis Research Society International (OARSI) scoring system (Table S1). We also performed tartrate-resistant acid phosphatase (TRAP) staining to evaluate TRAP+ osteoclasts within the subchondral bone following a standard protocol (CK20203, MultiSciences Co., Ltd., Zhejiang, China).
Immunohistochemistry
The expression levels of aggrecan (Boster, China) (1:100), collagen-II (Boster, China) (1:200), ADAMTS-4 (Boster, China) (1:200), MMP-13 (Boster, China) (1:100), leptin (Boster, China) (1:100) in cartilage and the expression of Osteocalcin (OCN) (Boster, China) (1:100) in subchondral bone were analyzed. For details see Supplementary Methods.
Micro-computed tomography (micro-CT) analysis
To investigate the changes in the subchondral bone micro-architecture, the knee joint was imaged using a Micro-CT (SkyScan1176 Software: Version 1.1 (build 6), Bruker, Kontich, Belgium). Bone mineral density (BMD, g/cm3), bone volume/tissue volume (BV/TV, %), trabecular number (Tb.N, 1/mm), trabecular thickness (Tb.Th, μm), trabecular separation (Tb.Sp, mm), and structure model index (SMI) were calculated.
Biomarker assays
Serum concentrations of fasted glucose (Yaji, China), insulin (Abbexa, UK), total cholesterol (TC) (Abcam, UK), high-density lipoprotein (HDL) (Boster, China), leptin (Boster, China), OCN (Abbexa, UK), C-telopeptide of type I collagen (CTX-I) (Abbexa, UK), IL-1β (Boster, China), cartilage oligomer protein (COMP) (Cusabio, China) were determined using enzyme-linked immunosorbent assay (ELISA) kit according to the manufacturer’s instructions. The data were measured using an iMARK Reader (BioRad Laboratories Inc., U.S.A.).
Data analysis and statistics
All experimental evaluations were performed by two independent researchers in a blinded manner. The data are presented as mean ± standard deviation or mean with 95% confidence interval and were analyzed using SPSS version 22.0 (IBM Corp., Armonk, NY, U.S.A.). Based on the results of homogeneity of variance analysis with Levene’s test, groups were compared using one-way ANOVA followed by LSD post-hoc tests or Kruskal-Wallis followed by Dunn’s post-hoc tests. Spearman’s rank correlation analyses were used to investigate correlations between the concentration of serum glucose, leptin, IL-1β, and OARSI score. The level of statistical significance was established at P < .05.
Results
Macroscopic findings of abdominal subcutaneous fat
In HFD-treated mice, macroscopic images showed evident abnormal accumulation of subcutaneous fat in the abdomen, while simvastatin treatment improved the abnormality in the HFD+S group ().
Changes in body weight, waist circumference, and BMI index
The body weight of HFD mice was significantly higher than Control mice (P < .05). The intervention of simvastatin significantly reduced the weight of mice fed a HFD (P < .05) (). The waist circumference and BMI index of mice in the HFD group substantially increased compared with the Control group at 12 weeks (P < .001 and P < .01). Compared with HFD mice, the waist circumference and BMI index of HFD+S mice were significantly reduced after intervention (P < .001, and P < .05) (). There was no statistical difference in the body length of mice in each group ().
Histological evaluation of adipose tissue
The cross-sectional area of adipocytes observed in the HFD group was significantly increased compared to the control group (P < .001), whereas after simvastatin intervention, mice in the HFD+S group showed a significantly lower area than that in the HFD group (P < .05) (). The high-fat diet greatly promoted the differentiation of adipocytes in the subchondral bone marrow of mice (). The number of adipocytes in subchondral bone marrow was significantly higher in the HFD group than in the Control group (P < .001). The effects were inhibited by gavage of simvastatin (P < .001) ().
Figure 2. (a, c, e) Representative histological sections indicated that the accumulation of neutral lipid and the progression of OA was attenuated by simvastatin. (B) the quantified cross-sectional area of adipocytes among the groups. (d) the quantified number of adipose cells among the groups. (f) OARSI scores of each group. Data are expressed as mean with 95% confidence interval. Bar = 100 μm. *P < .05; **P < .01; ***P < .001.
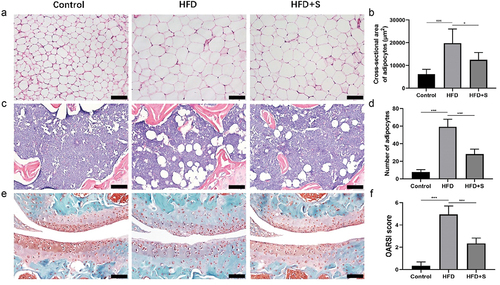
Histological changes in articular cartilage
In the Control group, the cartilage surface and the structures of chondrocytes were normal, Safranin-O staining of extracellular matrix (ECM) was evenly distributed. With the induction of high-fat diet, the articular cartilage of HFD group exhibited extensive cartilage lesions, and Safranin-O staining area was significantly reduced. The administration of simvastatin alleviated the severity of cartilage degeneration (). The HFD group presented a significantly higher OARSI score than the Control group (P < .001), and the score was significantly decreased after simvastatin intervention (P < .001) ().
Simvastatin improve cartilage degeneration
Aggrecan and collagen-II expression was significantly lower in the HFD group than in the Control group (P < .001), whereas these two protein expressions were significantly higher in the HFD+S group than in the HFD group (P < .001) (). Significantly higher ADAMTS-4 and MMP-13 expression levels were observed in the HFD group than in the Control group (P < .05 and P < .01). After simvastatin intervention, the expression level of MMP-13 but ADAMTS-4 in the HFD+S group was significantly reduced (P < .001) (). In addition, with the induction of high-fat diet, the leptin-positive chondrocytes in mouse cartilage increased significantly, but the intervention of simvastatin inhibited this pathological change (P < .001) ().
Figure 3. Immunohistochemical staining for aggrecan, collagen-II, ADAMTS-4, MMP-13 and leptin in cartilage. (a, c, e, g, i) the intervention of simvastatin promotes aggrecan, collagen-II expression and inhibits MMP-13, leptin expression in the cartilage of mice with a HFD. (b, d, f, h, j) the quantified protein levels of aggrecan, collagen-II, ADAMTS-4, MMP-13 and leptin in cartilage. Data are expressed as mean ± standard deviation. Bar = 20 μm. *P < .05; **P < .01; ***P < .001.
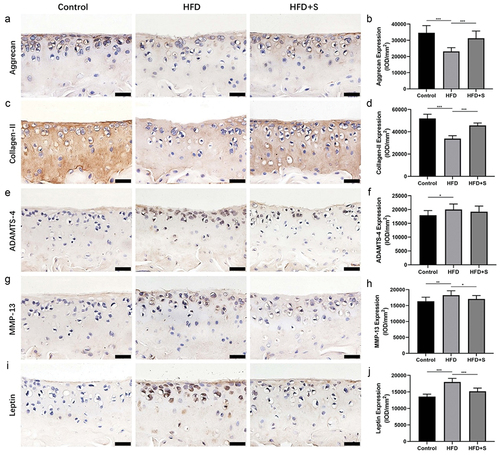
Simvastatin improve subchondral bone microstructure
OCN immunohistochemical staining and TRAP staining showed a significantly decreased number of osteoblasts and increased number of osteoclasts on trabecular bone surface in the HFD mice compared with Control mice (P < .001). However, the intervention of simvastatin significantly decreased the number of osteoclasts in HFD+S group (P < .001) ()
Figure 4. The intervention of simvastatin improves the imbalanced subchondral bone metabolism in HFD mice. (a) Representative OCN-stained sections with quantification of the (b) number of osteoblasts (N. OBs) on trabecular bone surface (BS) in tibia subchondral bone. (c) Representative TRAP-stained sections with quantitation of the (d) number of osteoclasts (N. OCs). Data are expressed as mean with 95% confidence interval. Bar = 100 μm. *P < .05; **P < .01; ***P < .001.
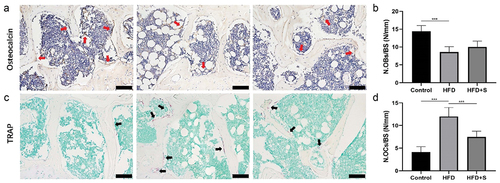
Micro-CT parameters of subchondral bone
The Micro-CT image of the femur and tibial subchondral bone of mice in each group are shown in . Quantitative analysis of the subchondral bone of the tibia showed that HFD mice exhibited significantly lower BMD, BV/TV, Tb.N, Tb.Th, and significantly higher Tb.Sp than that in Control mice (P < .001, P < .001, P < .001, P < .01, and P < .001, respectively). Compared with those of the HFD group, the BMD, BV/TV, and Tb.N values of the HFD+S group were significantly higher, but the Tb.Sp value was significantly lower (P < .001, P < .01, P < .05, and P < .001, respectively) ().
Figure 5. The simvastatin improves the decreased bone mass and impaired bone microstructures induced by HFD. (a) the representative micro-CT images of the femur and tibial subchondral bone. (b-g) Morphological parameters of the trabecular bone mineral density (BMD), Bone Volume/Total Volume (BV/TV), Trabecular Number (Tb.N), Trabecular Thickness (Tb.Th), Trabecular Separation Distance (Tb.Sp), Structure model index (SMI). Data are expressed as mean ± standard deviation. *P < .05; **P < .01; ***P < .001.
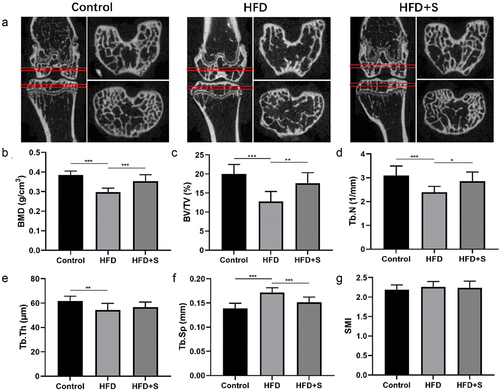
Biomarker analysis
The concentration of serum glucose, insulin, total cholesterol, leptin, CTX-I, and IL-1β were significantly higher in the HFD group than in the Control group (P < .001, P < .05, P < .001, P < .01, P < .01 and P < .01, respectively), after the intervention of simvastatin, the glucose, total cholesterol, leptin, and CTX-I concentrations of mice in the HFD+S group were significantly decreased (P < .01, P < .001, P < .001, and P < .05, respectively). The serum high-density lipoprotein, OCN, and COMP concentration were not significantly different among groups ().
Table 1. Differences between serum metabolite parameters measured in all groups.
n = 10 for each group. aP < .05 vs Control group, bP < .05 vs HFD group.
Correlation analysis
Significant positive correlation was observed between Serum concentrations of glucose, leptin, IL-1β, and OARSI score (r = 0.651, P < .001, r = 0.876, P < .001, and r = 0.435, P = .016, respectively) ().
Discussion
Osteoarthritis and obesity are two common health problems worldwide, which are usually related to age-related joint wear and obesity determined by diet and lifestyle (Tukker et al., Citation2009). In this study we subjected mice to normal and high-fat diet to identify their contributions to OA pathogenesis. The results of the present study demonstrate that HFD increased body weight, abdominal subcutaneous fat, BMI index, serum glucose, insulin, and leptin concentration at 12 weeks of diet compared to control mice. With these metabolic disorders, the degradation of the cartilage matrix and the destruction of subchondral bone in mice are aggravated, and a significant increase in leptin-positive cells in articular cartilage was observed.
Studies have suggested that the extracellular matrix (ECM) homeostasis imbalanced in cartilage, high-magnitude mechanical stress and high levels of inflammatory cytokines all exacerbate the inflammatory responses of OA (Maldonado & Nam, Citation2013; Xia et al., Citation2014). In our study, the HFD group displayed distinct cartilage degeneration and a higher histological score than in the control group. Immunohistochemical results indicated that the expression levels of ADAMTS-4 and MMP-13 were significantly increased, whereas the expression of aggrecan and collagen-II was significantly decreased in HFD mice. Aggrecan and collagen-II are components of chondrocyte ECM, which plays an important role in maintaining the proper function of cartilage (Han et al., Citation2019; Sivan et al., Citation2014). And MMP-13 and ADAMTS-4, two matrix-degrading enzymes, involved in the process of degrading aggrecan and collagen-II and usually highly expressed in OA cartilage (Slovacek et al., Citation2020; Wan et al., Citation2020). In addition, previous researches have pointed out that in OA, subchondral bone undergoes an abnormal remodeling process with the regulation of both mechanical and biological signals (Hu et al., Citation2021). Our study indicated a significantly lower number of osteoblasts on trabecular bone surface in the HFD mice compared with control mice. One possible mechanism is that high-fat diet induces bone marrow mesenchymal stem cells to differentiate into adipocytes instead of osteoblast (Tencerova et al., Citation2018). Besides, TRAP staining indicates a significant increase the osteoclast number in obesity-induced OA. This elevated number and distribution of osteoclasts adjacent to the trabecular bone is also considered a representative phenomenon in subchondral bone marrow lesions (Wang et al., Citation2019). The BMD, BV/TV, Tb.N, and Tb.Th values of the subchondral bone in HFD mice were significantly reduced, but the Tb.Sp value was significantly increased, indicating that the subchondral bone of obesity-induced OA mice was damaged. Deleterious alterations of subchondral bone may transmit abnormal stress to articular cartilage and cause the deterioration of cartilage (G. Li et al., Citation2013). The accelerated OA progression and increased cartilage catabolism observed in this study may be partly attributable to overweight induced by a high-fat diet and may also involve other biological mediators.
Indeed, we found that after 12 weeks of HFD, many indicators of the metabolic state of mice were significantly abnormal. Compared with the control group, serum levels of glucose, insulin, total cholesterol, leptin, CTX-I, and IL-1β of HFD mice were significantly increased, indicating that HFD mice are undergoing a pathological process of insulin resistance, systemic inflammation, and high bone turnover, which have previously been identified to have a significant correlation with the development of OA (Tchetina et al., Citation2020; Zhuo et al., Citation2012). Similar to previous studies, the results of spearman correlation analysis show a strong and significant correlation between serum glucose, IL-1β, leptin, and OARSI score. It has been proved that leptin, whether it is the signal in the blood or expression in tissues, is closely related to the progress of OA (Jiang et al., Citation2020; Scotece & Mobasheri, Citation2015). In addition to the significantly elevated leptin levels in the serum of HFD mice, significantly increased leptin expression was also observed in the articular cartilage. A study on mouse OA has shown that the leptin mRNA expression in cartilage was up-regulated by HFD, suggesting that leptin may be a secondary mediator of osteoarthritis pathogenesis (Jiang et al., Citation2020).
Statins are clinically used for the treatment of dyslipidemia currently. With the deepening of research, it has been discovered that statins, in addition to reducing blood lipids, also have potential therapeutic effect on osteoarthritis (Yu et al., Citation2019). Notably, the doses of simvastatin used in the present study were converted from the clinical doses according to the guidelines issued by the FDA (U.S. Food and Drug Administration Citation2018) and had been used in previous animal studies focusing on musculoskeletal disorders (X. Li et al., Citation2011; Long et al., Citation2016). From the data, the intervention of simvastatin significantly reduced the body weight, the accumulation of neutral lipid in visceral adipose tissue and the subchondral bone marrow adipocytes of HFD mice. The weight control effect of simvastatin has been observed (Taylor et al., Citation2017), although it is not a weight loss drug in essence, simvastatin has a beneficial effect on the regulation of abnormal lipid metabolism to retard the progression of OA induced by obesity.
There have been studies discussing the direct therapeutic effects of osteoarthritis with statins, which is found to be able to promote the proliferation of chondrocytes in the knee joint and inhibit their apoptosis, as well as delay the degradation of cartilage matrix (Bayyurt et al., Citation2015; Lazzerini et al., Citation2004). In this study, we confirmed that simvastatin had a protective effect on the impaired OA cartilage. Simvastatin intervention significantly reduces the OARSI score and the expression level of MMP-13 in cartilage of OA mice and significantly increased the expression of aggrecan and collagen-II. It has been demonstrated that the effect of statin on matrix metalloproteinases (MMPs) is dependent on the inhibition of mevalonate synthesis (Tanaka et al., Citation2019; Wilson et al., Citation2005). Another report also suggested that simvastatin, by blocking HMG CoA-reductase and interfering in the prenylation processes, is able to inhibit MMP-3 production from cultured human chondrocytes, suggesting a possible mechanism for statins in counteracting chronic joint disease-related cartilage damage (Lazzerini et al., Citation2004). Furthermore, we found that simvastatin can significantly reduce the expression of leptin in articular cartilage. Studies have shown that simvastatin inhibits the expression of leptin in preadipocytes, but there is no research to explore the effect of simvastatin on the expression of leptin in articular cartilage (Maeda & Horiuchi, Citation2009). Therefore, the inhibitory effect of simvastatin on leptin in articular cartilage may be another contributor to slowdown the progression of OA. Not only cartilage, the subchondral bone is also closely associated with the development and progression of OA (Goldring & Goldring, Citation2016). In the present study, the deterioration of subchondral bone micro-architecture was remarkably ameliorated by intervention with simvastatin. Compared with the HFD group, the number of subchondral bone osteoclasts in the HFD+S group was significantly reduced, and the Micro-CT parameters were also significantly improved. The bone-improving effect of simvastatin has been proven by previous studies. Simvastatin has been found to increase bone formation by promoting osteoblast differentiation and mineralization (Jadhav & Jain, Citation2006). Simvastatin may also inhibit the RANKL-induced NF-κB activation pathway that leads to suppression of osteoclastogenesis which is related to bone resorption (Ahn et al., Citation2008). Therefore, the improvement of subchondral bone may be another effect of simvastatin in alleviating the progression of OA.
Simvastatin also improves obesity-induced glycolipid and bone metabolism disorders. Significantly reduced serum glucose, total cholesterol, leptin, and CTX-I concentrations were observed in the HFD+S group, and its decreased serum IL-1β levels, although the difference was not statistically significant. Recent studies indicated that metabolic conditions with high cholesterol levels may be related to an increased risk of OA (Farnaghi et al., Citation2017). Simvastatin blocks IL‐1β‐induced reactive oxygen species (ROS) generation by blocking the NF‐κB pathway and also inhibits the expression of matrix degrading enzymes MMP-1 and MMP-13 suggesting that simvastatin can modulate the catabolic factors that contribute to cartilage destruction (Yu et al., Citation2019, Citation2020). Furthermore, since leptin is a known metabolism-regulating hormone induced by HFD and linked to OA pathogenesis, the inhibitory effect of simvastatin on leptin may be another potential factor to protect the knee joint (Griffin et al., Citation2010).
Conclusions
In summary, obesity aggravates articular degeneration, characterized by enhanced cartilage catabolism, increased expression of leptin, and the deterioration of subchondral bone. Simvastatin has a potential therapeutic effect on obesity-induced OA and can simultaneously reduce the level of leptin in serum and cartilage. For OA patient with obesity, simvastatin may be used to treat hyperlipidemia or weight control and also have a protective effect on the knee joint. But for OA patient with no obesity, the side effects of simvastatin should also be noted. Further research is needed before it can be put into clinical practice. In addition, this study also had several limitations. We only selected a single time-point (12 weeks), which provided no insight into the rate of disease progression and only one concentration of simvastatin was assessed. Thus, a better experimental regimen may exist. These issues should be resolved in future studies. Other OA models could be used to observe whether simvastatin improves subchondral bone microstructure through osteogenic effects, indirectly preventing cartilage degeneration.
Supplemental Material
Download MS Word (18.2 KB)Disclosure statement
No potential conflict of interest was reported by the author(s).
Supplementary data
Supplemental data for this article can be accessed online at https://doi.org/10.1080/19476337.2023.2245008.
References
- Ahn, K. S., Sethi, G., Chaturvedi, M. M., & Aggarwal, B. B. (2008). Simvastatin, 3-hydroxy-3-methylglutaryl coenzyme a reductase inhibitor, suppresses osteoclastogenesis induced by receptor activator of nuclear factor-kappaB ligand through modulation of NF-kappaB pathway. International Journal of Cancer, 123(8), 1733–1740. https://doi.org/10.1002/ijc.23745
- Aktas, E., Sener, E., & Gocun, P. U. (2011). Mechanically induced experimental knee osteoarthritis benefits from anti-inflammatory and immunomodulatory properties of simvastatin via inhibition of matrix metalloproteinase-3. Journal of Orthopaedics and Traumatology: Official Journal of the Italian Society of Orthopaedics and Traumatology, 12(3), 145–151. https://doi.org/10.1007/s10195-011-0154-y
- Bayyurt, S., Küçükalp, A., Bilgen, M. S., Bilgen, Ö. F., Çavuşoğlu, İ., & Yalçınkaya, U. (2015). The chondroprotective effects of intraarticular application of statin in osteoarthritis: An experimental study. Indian Journal of Orthopaedics, 49(6), 665–671. https://doi.org/10.4103/0019-5413.168751
- Bourne, R., Mukhi, S., Zhu, N., Keresteci, M., & Marin, M. (2007). Role of obesity on the risk for total hip or knee arthroplasty. Clinical Orthopaedics and Related Research, 465, 185–188. https://doi.org/10.1097/BLO.0b013e3181576035
- Datta, P., Zhang, Y., Parousis, A., Sharma, A., Rossomacha, E., Endisha, H., Wu, B., Kacprzak, I., Mahomed, N. N., Gandhi, R., Rockel, J. S., & Kapoor, M. (2017). High-fat diet-induced acceleration of osteoarthritis is associated with a distinct and sustained plasma metabolite signature. Scientific Reports, 7(1), 8205. https://doi.org/10.1038/s41598-017-07963-6
- Farnaghi, S., Crawford, R., Xiao, Y., & Prasadam, I. (2017). Cholesterol metabolism in pathogenesis of osteoarthritis disease. International Journal of Rheumatic Diseases, 20(2), 131–140. https://doi.org/10.1111/1756-185X.13061
- Fukui, T., Ii, M., Shoji, T., Matsumoto, T., Mifune, Y., Kawakami, Y., Akimaru, H., Kawamoto, A., Kuroda, T., Saito, T., Tabata, Y., Kuroda, R., Kurosaka, M., & Asahara, T. (2012). Therapeutic effect of local administration of low-dose simvastatin-conjugated gelatin hydrogel for fracture healing. Journal of Bone and Mineral Research: The Official Journal of the American Society for Bone and Mineral Research, 27(5), 1118–1131. https://doi.org/10.1002/jbmr.1558
- Goldring, S. R., & Goldring, M. B. (2016). Changes in the osteochondral unit during osteoarthritis: Structure, function and cartilage-bone crosstalk. Nature Reviews Rheumatology, 12(11), 632–644. https://doi.org/10.1038/nrrheum.2016.148
- Goto, N., Okazaki, K., Akasaki, Y., Ishihara, K., Murakami, K., Koyano, K., Ayukawa, Y., Yasunami, N., Masuzaki, T., & Nakashima, Y. (2017). Single intra-articular injection of fluvastatin-PLGA microspheres reduces cartilage degradation in rabbits with experimental osteoarthritis. Journal of Orthopaedic Research: Official Publication of the Orthopaedic Research Society, 35(11), 2465–2475. https://doi.org/10.1002/jor.23562
- Griffin, T. M., Fermor, B., Huebner, J. L., Kraus, V. B., Rodriguiz, R. M., Wetsel, W. C., Cao, L., Setton, L. A., & Guilak, F. (2010). Diet-induced obesity differentially regulates behavioral, biomechanical, and molecular risk factors for osteoarthritis in mice. Arthritis Research & Therapy, 12(4), R130. https://doi.org/10.1186/ar3068
- Han, B., Li, Q., Wang, C., Patel, P., Adams, S. M., Doyran, B., Nia, H. T., Oftadeh, R., Zhou, S., Li, C. Y., Liu, X. S., Lu, X. L., Enomoto-Iwamoto, M., Qin, L., Mauck, R. L., Iozzo, R. V., Birk, D. E., & Han, L. (2019). Decorin regulates the aggrecan network integrity and biomechanical functions of cartilage extracellular matrix. ACS nano, 13(10), 11320–11333. https://doi.org/10.1021/acsnano.9b04477
- Hatano, H., Maruo, A., Bolander, M. E., & Sarkar, G. (2003). Statin stimulates bone morphogenetic protein-2, aggrecan, and type 2 collagen gene expression and proteoglycan synthesis in rat chondrocytes. Journal of Orthopaedic Science: Official Journal of the Japanese Orthopaedic Association, 8(6), 842–848. https://doi.org/10.1007/s00776-003-0724-9
- Hu, Y., Chen, X., Wang, S., Jing, Y., & Su, J. (2021). Subchondral bone microenvironment in osteoarthritis and pain. Bone Research, 9(1), 20. https://doi.org/10.1038/s41413-021-00147-z
- Jadhav, S. B., & Jain, G. K. (2006). Statins and osteoporosis: New role for old drugs. The Journal of Pharmacy and Pharmacology, 58(1), 3–18. https://doi.org/10.1211/jpp.58.1.0002
- Jeyakumar, S. M., Lopamudra, P., Padmini, S., Balakrishna, N., Giridharan, N. V., & Vajreswari, A. (2009). Fatty acid desaturation index correlates with body mass and adiposity indices of obesity in Wistar NIN obese mutant rat strains WNIN/Ob and WNIN/GR-Ob. Nutrition & Metabolism, 6(1), 27. https://doi.org/10.1186/1743-7075-6-27
- Jiang, M., He, J., Gu, H., Yang, Y., Huang, Y., Xu, X., & Liu, L. (2020). Protective effect of resveratrol on obesity-related osteoarthritis via alleviating JAK2/STAT3 signaling pathway is independent of SOCS3. Toxicology and Applied Pharmacology, 388, 114871. https://doi.org/10.1016/j.taap.2019.114871
- Lazzerini, P. E., Capecchi, P. L., Nerucci, F., Fioravanti, A., Chellini, F., Piccini, M., Bisogno, S., Marcolongo, R., & Laghi Pasini, F. (2004). Simvastatin reduces MMP-3 level in interleukin 1beta stimulated human chondrocyte culture. Annals of the Rheumatic Diseases, 63(7), 867–869. https://doi.org/10.1136/ard.2003.009746
- Li, X., Song, Q. S., Wang, J. Y., Leng, H. J., Chen, Z. Q., Liu, Z. J., Dang, G. T., & Song, C. L. (2011). Simvastatin induces estrogen receptor-alpha expression in bone, restores bone loss, and decreases ERα expression and uterine wet weight in ovariectomized rats. Journal of Bone and Mineral Metabolism, 29(4), 396–403. https://doi.org/10.1007/s00774-010-0231-y
- Li, G., Yin, J., Gao, J., Cheng, T. S., Pavlos, N. J., Zhang, C., & Zheng, M. H. (2013). Subchondral bone in osteoarthritis: Insight into risk factors and microstructural changes. Arthritis Research & Therapy, 15(6), 223. https://doi.org/10.1186/ar4405
- Long, T., Tang, T., Hao, Y., Zhu, Z., Shao, L., & Liu, M. (2016). Effect of simvastatin on osteogenesis of the lumbar vertebrae in ovariectomized rats. Experimental and Therapeutic Medicine, 12(6), 3951–3957. https://doi.org/10.3892/etm.2016.3904
- Maeda, T., & Horiuchi, N. (2009). Simvastatin suppresses leptin expression in 3T3-L1 adipocytes via activation of the cyclic AMP-PKA pathway induced by inhibition of protein prenylation. Journal of Biochemistry, 145(6), 771–781. https://doi.org/10.1093/jb/mvp035
- Maldonado, M., & Nam, J. (2013). The role of changes in extracellular matrix of cartilage in the presence of inflammation on the pathology of osteoarthritis. BioMed Research International, 2013, 284873. https://doi.org/10.1155/2013/284873
- Pottie, P., Presle, N., Terlain, B., Netter, P., Mainard, D., & Berenbaum, F. (2006). Obesity and osteoarthritis: More complex than predicted! Annals of the Rheumatic Diseases, 65(11), 1403–1405. https://doi.org/10.1136/ard.2006.061994
- Reijman, M., Pols, H. A., Bergink, A. P., Hazes, J. M., Belo, J. N., Lievense, A. M., & Bierma-Zeinstra, S. M. (2007). Body mass index associated with onset and progression of osteoarthritis of the knee but not of the hip: The Rotterdam study. Annals of the Rheumatic Diseases, 66(2), 158–162. https://doi.org/10.1136/ard.2006.053538
- Scotece, M., & Mobasheri, A. (2015). Leptin in osteoarthritis: Focus on articular cartilage and chondrocytes. Life Sciences, 140, 75–78. https://doi.org/10.1016/j.lfs.2015.05.025
- Sivan, S. S., Wachtel, E., & Roughley, P. (2014). Structure, function, aging and turnover of aggrecan in the intervertebral disc. Biochimica et Biophysica Acta (BBA) - General Subjects, 1840(10), 3181–3189. https://doi.org/10.1016/j.bbagen.2014.07.013
- Slovacek, H., Khanna, R., Poredos, P., Jezovnik, M., Hoppensteadt, D., Fareed, J., & Hopkinson, W. (2020). Interrelationship of osteopontin, MMP-9 and ADAMTS4 in patients with osteoarthritis undergoing total joint arthroplasty. Clinical and Applied Thrombosis/Hemostasis: Official Journal of the International Academy of Clinical and Applied Thrombosis/Hemostasis, 26, 1076029620964864. https://doi.org/10.1177/1076029620964864
- Sverdrup, F. M., Yates, M. P., Vickery, L. E., Klover, J. A., Song, L. R., Anglin, C. P., & Misko, T. P. (2010). Protein geranylgeranylation controls collagenase expression in osteoarthritic cartilage. Osteoarthritis and Cartilage, 18(7), 948–955. https://doi.org/10.1016/j.joca.2010.03.015
- Tanaka, T., Matsushita, T., Nishida, K., Takayama, K., Nagai, K., Araki, D., Matsumoto, T., Tabata, Y., & Kuroda, R. (2019). Attenuation of osteoarthritis progression in mice following intra-articular administration of simvastatin-conjugated gelatin hydrogel. Journal of Tissue Engineering and Regenerative Medicine, 13(3), 423–432. https://doi.org/10.1002/term.2804
- Taylor, B. A., Ng, J., Stone, A., Thompson, P. D., Papasavas, P. K., & Tishler, D. S. (2017). Effects of statin therapy on weight loss and diabetes in bariatric patients. Surgery for obesity and related diseases. Official Journal of the American Society for Bariatric Surgery, 13(4), 674–680. https://doi.org/10.1016/j.soard.2016.11.018
- Tchetina, E. V., Markova, G. A., & Sharapova, E. P. (2020). Insulin resistance in osteoarthritis: Similar mechanisms to type 2 diabetes mellitus. Journal of Nutrition and Metabolism, 2020, 4143802. https://doi.org/10.1155/2020/4143802
- Tencerova, M., Figeac, F., Ditzel, N., Taipaleenmäki, H., Nielsen, T. K., & Kassem, M. (2018). High-fat diet-induced obesity promotes expansion of bone marrow adipose tissue and impairs skeletal stem cell functions in mice. Journal of Bone and Mineral Research: The Official Journal of the American Society for Bone and Mineral Research, 33(6), 1154–1165. https://doi.org/10.1002/jbmr.3408
- Tukker, A., Visscher, T. L., & Picavet, H. S. (2009). Overweight and health problems of the lower extremities: Osteoarthritis, pain and disability. Public Health Nutrition, 12(3), 359–368. https://doi.org/10.1017/S1368980008002103
- U.S. Food and Drug Administration. (2018). Estimating the maximum safe starting dose in initial clinical trials for therapeutics in adult healthy volunteers. https://www.fda.gov/media/72309/download
- Wang, F., Luo, A., Xuan, W., Qi, L., Wu, Q., Gan, K., Zhang, Q., Zhang, M., & Tan, W. (2019). The bone marrow edema links to an osteoclastic environment and precedes synovitis during the development of collagen induced arthritis. Frontiers in Immunology, 10, 884. https://doi.org/10.3389/fimmu.2019.00884
- Wan, Y., Li, W., Liao, Z., Yan, M., Chen, X., & Tang, Z. (2020). Selective MMP-13 inhibitors: Promising agents for the therapy of osteoarthritis. Current Medicinal Chemistry, 27(22), 3753–3769. https://doi.org/10.2174/0929867326666181217153118
- Wilson, W. R., Evans, J., Bell, P. R., & Thompson, M. M. (2005). HMG-Coa reductase inhibitors (statins) decrease MMP-3 and MMP-9 concentrations in abdominal aortic aneurysms. European Journal of Vascular and Endovascular Surgery: The Official Journal of the European Society for Vascular Surgery, 30(3), 259–262. https://doi.org/10.1016/j.ejvs.2005.02.044
- Wluka, A. E., Lombard, C. B., & Cicuttini, F. M. (2013). Tackling obesity in knee osteoarthritis. Nature Reviews Rheumatology, 9(4), 225–235. https://doi.org/10.1038/nrrheum.2012.224
- Xia, B., Chen, D., Zhang, J., Hu, S., Jin, H., & Tong, P. (2014). Osteoarthritis pathogenesis: A review of molecular mechanisms. Calcified Tissue International, 95(6), 495–505. https://doi.org/10.1007/s00223-014-9917-9
- Yu, S. M., Han, Y., & Kim, S. J. (2019). Simvastatin induces differentiation in rabbit articular chondrocytes via Wnt/β-catenin pathway. European Journal of Pharmacology, 863, 172672. https://doi.org/10.1016/j.ejphar.2019.172672
- Yu, S. M., Han, Y., & Kim, S. J. (2020). Simvastatin abolishes nitric oxide- and reactive oxygen species-induced cyclooxygenase-2 expression by blocking the nuclear factor κB pathway in rabbit articular chondrocytes. Cell Biology International, 44(10), 2153–2162. https://doi.org/10.1002/cbin.11424
- Zhou, B., Chen, D., Xu, H., & Zhang, X. (2017). Proliferation of rabbit chondrocyte and inhibition of IL-1β-induced apoptosis through MEK/ERK signaling by statins. In vitro cellular & developmental biology. In Vitro Cellular & Developmental Biology - Animal, 53(2), 124–131. https://doi.org/10.1007/s11626-016-0086-1
- Zhuo, Q., Yang, W., Chen, J., & Wang, Y. (2012). Metabolic syndrome meets osteoarthritis. Nature Reviews Rheumatology, 8(12), 729–737. https://doi.org/10.1038/nrrheum.2012.135