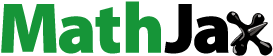
ABSTRACT
In this study, the methylcellulose edible coating additives, namely, salicylic acid (SA) (1–2.5 mM), canola oil (0.05–0.1%) and tween-80 (0.05–0.1%) were optimized using RSM to improve quality management of pomegranate arils. The central composite design was used, and the response variables included mass loss (ML), total soluble solids (TSS), total phenolic content (TPC) and 2,2-Diphenyl-1-picrylhydrazyl (DPPH scavenging activity). The results showed that ML was affected by the quadratic terms of canola oil, tween-80 and SA, whereas TSS was affected by the quadratic terms of canola oil and tween-80. The interaction of SA and canola oil showed a significant effect on TSS and TPC, whereas DPPH scavenging activity was affected by linear terms of SA and canola oil. The optimized conditions for the independent variables were 1.55 mM, 0.079% and 0.079% for SA, canola oil and tween-80, respectively. The predicted responses were consistent with experimental values, indicating the model accuracy and suitability.
1. Introduction
Pomegranate (Punica granatum L.) is a widely cultivated fruit with excellent nutritional properties (Fawole & Opara, Citation2013; Mditshwa et al., Citation2013). Recently, pomegranate has gained high consumer acceptability, which is linked to increasing consumer awareness of the fruit’s health benefits, including anti-cancer, anti-hypertension, anti-mutagenic and high antioxidant capacity (O’Grady et al., Citation2014; Viuda-Martos et al., Citation2010).
Despite the acclaimed health benefits, pomegranate fruit is prone to external rind disorders during storage, which largely impact only its cosmetic appeal (Arendse et al., Citation2018). Thus, to enhance fruit consumption, the industry has focused on developing fresh-cut/minimally processed products, e.g. ready-to-eat arils, which offer convenience and ensure quality (Rokalla et al., Citation2022). However, ready-to-eat pomegranate arils are characterized by a faster quality deterioration rate, resulting from induced stress due to tissue damage during processing (Mwelase & Fawole, Citation2022). Thus, quality management techniques are essential to improve the storability of ready-to-eat pomegranate arils. However, the approach should focus more on product quality, safety, and environmental sustainability.
Recently, edible coatings have gained acceptance as an effective and safe strategy for maintaining quality, improving storability and delaying the senescence of different minimally processed products, including pomegranate arils (Jokar et al., Citation2021; Kawhena et al., Citation2020; Özdemir & Gökmen, Citation2017; Singla et al., Citation2022; Yousuf & Srivastava, Citation2017). Methylcellulose (MC) is one of the most used edible coating materials owing to its excellent film properties, non-toxicity, biodegradability, and high availability (Cazón et al., Citation2017; Maftoonazad & Ramaswamy, Citation2005). MC has been reported to effectively maintain quality and delay the senescence of different whole fruits and fresh-cut products, including avocados (Maftoonazad & Ramaswamy, Citation2005), strawberries (Nadim et al., Citation2015), peaches (Neda Maftoonazad et al., Citation2008) and ready-to-eat pomegranate arils (Kawhena et al., Citation2020). This effect is attributable to the ability of MC to provide reduced permeability to water loss and modify the internal gaseous atmosphere, resulting in higher CO2 and lower O2, thereby reducing the rate of many physiological reactions (Maftoonazad & Ramaswamy, Citation2005; Neda; Maftoonazad et al., Citation2008).
Incorporating different bioactive compounds with enhanced antimicrobial and antioxidant properties helps improve edible coatings’ functional properties and performance. In line with this, Kawhena et al. (Citation2020) reported improved storability and enhanced antioxidant capacity of ready-to-eat pomegranate arils treated with MC 1% in combination with thyme oil (0.5%) compared with arils treated with MC alone during 16-day storage at 5°C; 85% RH. Similarly, Ayranci and Tunc (Citation2004) found significantly lower weight loss and enhanced retention of vitamin C in apricots and green peppers coated with MC-ascorbic acid composite coating during 10 and 12 days storage, respectively, at 25°C; 84% RH compared with fruit coated with MC alone. These suggest that MC is a promising coating material; however, additional bioactive and natural-based compounds are essential to enhance its functionality.
Salicylic acid (SA) is one of the natural and safe compounds widely incorporated in the formulation of edible coatings to improve the quality management of fresh horticultural produce. SA is a plant hormone known for its high free radical scavenging capacity, which is essential in preserving fresh produce quality. Hanif et al. (Citation2020) reported higher texture, ascorbic acid, total phenol content, total antioxidant, and enhanced activity of antioxidant enzymes of papaya fruit treated with SA (1.5 mM) during 28-day storage at 12°C; 90% RH. Similar results have been observed on cucumber (Zhang et al., Citation2015), pomegranate (Sayyari et al., Citation2016) and blackcurrant berries (Xing et al., Citation2021). Notably, Sayyari et al. (Citation2016) reported significantly lower weight loss, respiration rate and better retention of the antioxidant capacity of pomegranate fruit treated with the combination of chitosan and SA during 5 months of storage at 2°C and 85% RH. This is attributed to the overall synergistic effect of combining edible coating materials and bioactive compounds, thus highlighting the importance of incorporating this bioactive compound in the edible coating formulation. However, little or no information is available regarding the synergistic effect of MC and SA in maintaining the quality of minimally processed products.
Many base coating materials, including MC, possess poor emulsifying properties and are highly hydrophilic (Ayranci & Tunc, Citation2004; Cazón et al., Citation2017). Therefore, emulsifiers and lipid-based materials are incorporated into the coating formulations to improve the physical and other functional properties, including adhesion, flexibility and hygroscopicity (Ayranci & Tunc, Citation2004). However, it has been noted that the most used lipid-based additives, such as oleic acid and stearic acid, cannot provide a homogeneous and cohesive matrix, resulting in compromised quality of the coatings matrix (Chaple et al., Citation2017; Nindjin et al., Citation2015).
Thus, researchers have been interested in exploring natural and economically feasible hydrophobic substances with improved properties. Among different natural additives, plant-based oils such as canola oil are recently gaining attention to improve the hydrophobicity of the coating formulations (Mohaddese et al., Citation2022; Mwelase et al., Citation2022; Nxumalo & Fawole, Citation2022; Reinoso et al., Citation2007). Concurrently, emulsifiers like tween-80 are being introduced to stabilize these emulsions. While the integration of these substances has demonstrated efficacy, extreme concentrations, either too high or too low, may negatively affect the product’s organoleptic qualities. This includes attributes such as flavour and appearance, which are particularly significant in fresh-cut or minimally processed products. Therefore, striking an optimal balance in the use of these substances is crucial to maintaining the desired product characteristics. Therefore, studies exploring the optimization of these coatings’ additives for improved quality management and developing low-cost innovative technologies of fresh-cut/minimally processed products are still essential (Gunasekera et al., Citation2017).
Response surface methodology (RSM) is a practical approach used to study the effect of several variables, their interactive effect and the establishment of optimal experiment conditions and predictive mathematical models with high accuracy. The RSM technique has been highly adopted to overcome the limitations of the time-consuming processes requiring extensive experimental data to draw reliable conclusions (Weremfo et al., Citation2023). In line with this, RSM has been successfully employed to optimize postharvest coating formulations for pomegranate (Kawhena et al., Citation2021), strawberry (Hajji et al., Citation2018), mango (Qambrani et al., Citation2022), pear (Nandane et al., Citation2017), apple (Rashid et al., Citation2020) and fresh-cut orange (Mohaddese et al., Citation2022). Therefore, this study aimed to optimize the composition of additives, SA, tween-80 and canola oil, of MC-based edible coating for improved quality management of ready-to-eat pomegranate arils using RSM.
2. Materials and methods
2.1 Fruit procurement and processing
Mature pomegranate (cv. Kessari) fruit were procured from Johannesburg fresh produce market in Gauteng Province, South Africa (26°13’26.6“S, 28°04’47.8“E) and transported to the Postharvest and Agroprocessing Research Centre at the University of Johannesburg. Pomegranate fruit were assessed and sorted for uniformity of colour, size, shape and external defects before being disinfected with 0.01% sodium hypochlorite and left to dry at 23 ± 2°C; 65 ± 5% RH.
Fruit were carefully cut with a sterilized sharp knife, and arils were extracted manually to avoid damage or breakage. The extracted arils were disinfected with 0.01% of sodium hypochlorite. The arils were dried under standard room conditions (23 ± 2°C; 65 ± 5% RH). A sterilized paper towel was used to remove excess water from the arils and then stored at 4.5 ± 0.5°C, 85 ± 0.5% RH while waiting for treatment application (Martínez-Romero et al., Citation2013).
2.2 Chemicals and solvents
Sodium hypochlorite, methylcellulose (~M7140), tween-80, HPLC grade methanol, Folin – Ciocalteu reagent, sodium carbonate (Na2CO3), 2,2-diphenyl-1-picrylhydrazyl (DPPH) were procured from Sigma-Aldrich (Johannesburg, South Africa). The 100% natural canola oil was also procured from Sigma-Aldrich (Johannesburg, South Africa). Salicylic acid was purchased from Merck (Pty) Ltd (Johannesburg, South Africa). All chemicals used in this study were of analytical grade.
2.3 Experimental design
This study comprised three independent variables; canola oil, tween-80, and SA, which were evaluated at five coded levels, from low (−1), mid (0), high (1), -α and +α (). The concentration ranges were selected based on preliminary experimental results (data not shown). Based on the preliminary experimental results and previous studies (Kawhena et al., Citation2020), 1% MC showed optimal effectiveness in maintaining arils’ quality and kept constant in the formulation. Central composite design (CCD) was used to design the experiment, resulting in a total of 20 runs with 6 replicates at the centre point to calculate the repeatability and reliability of the method (). The effect of different experimental combinations was evaluated using response variables, including mass loss (ML), total soluble solids (TSS), total phenolic content (TPC) and 2,2-Diphenyl-1-picrylhydrazyl (DPPH) radical scavenging capacity. Based on our preliminary results (data not shown), these parameters have shown a better estimate of fruit quality, mainly taste, moisture loss, and antioxidant capacity/defence system.
Table 1. The levels of experimental independent variables used in the central composite design.
Table 2. The central composite design used to formulate edible coating formulations and experimental data obtained for canola oil, tween-80 and salicylic acid applied on “Kessari” ready-to-eat pomegranate arils during refrigerated storage at 4.5°C for 12 days.
2.4 Coating preparation and application
The coating solutions were prepared by first dissolving 1 g (1% w/v) of MC in 100 mL of water – ethyl alcohol mixture (3:1) under magnetic stirring for 30 min at a controlled temperature of 70°C (Maftoonazad & Ramaswamy, Citation2005). SA (1–2.5 mM) was then added to the solution with the subsequent addition of canola oil (0.05–0.1%) and tween-80 (0.05–0.1%) and further mixed under magnetic stirring for 15 min. The solution was homogenized at 300 g for 30 seconds and sonicated using an ultrasonic bath (705, Labotec, Johannesburg, Gauteng, South Africa) for 10 min to facilitate the removal of bubbles.
The extracted arils were randomly divided into 20 treatment runs, each containing 1.6 kg of arils. These runs were treated with distinct coating treatments through immersion in the specific coating formulations for 5 min. In order to ensure consistent and even application of the coatings, each of the 20 groups was subdivided into half, and the arils within each subdivision were immersed in 500 mL of the respective coating solution. Subsequently, the excess solution was drained off using a strainer, and arils were collected on a sterilized tray and left to dry at 23 ± 2°C; 65 ± 5% RH. The arils (100 g) were then packaged in polypropylene punnets and stored in a refrigerator at 4.5 ± 0.5°C, 85 ± 0.5% RH. Four punnets per treatment were sampled at 3-day intervals till day 12 to monitor the response variables. Eight punnets were sampled immediately after washing for baseline measurements.
2.5 Determination of mass loss (ML)
ML of the stored arils was evaluated by randomly weighing four punnets for each treatment at each sampling day using a high-precision laboratory balance (Labotec Precision Balance, XP20K1, China, 0.001 g accuracy). The ML percentage was then calculated based on the sample ML at day 0 and expressed as mean ± S.E. The measurements were conducted in four replicates at 3-day intervals till day 12.
2.6 Measurement of total soluble solids (TSS)
TSS was measured using a handheld calibrated refractometer (Refractometer PAL-1, Atago, Tokyo, Japan) at room 23 ± 2°C; 65 ± 5% RH and results were expressed as °Brix (Mwelase & Fawole, Citation2022). The measurements of TSS were conducted in four replicates at 3-day intervals till day 12.
2.7 Measurements of total phenolic content (TPC) and DPPH radical-scavenging activity
A method by Fawole and Opara (Citation2013) with slight modifications was followed to prepare PJ extracts for phytochemical analyses. This study used pomegranate juice (PJ) prepared using a jump start juicer (Milex, Johannesburg, South Africa). Subsequently, 0.5 mL PJ was mixed with 15 mL of 50% aqueous methanol. The mixture was vortexed and then sonicated in ice-cold water for 10 min using an ultrasonic bath (705, Labotec, Johannesburg, Gauteng, South Africa). Afterwards, the mixture was centrifuged for 10 min at 10,000 g using a centrifuge (Heraeus, Kendro Biofuge Stratos, German) and kept at 4°C until further analyses. The fruit were sampled in four replicates every 3 days until day 12 for the measurements of both TPC and DPPH.
TPC was determined using a method previously described by Mwelase and Fawole (Citation2022) with slight modifications. Briefly, 50 µL of PJ extract was mixed with 450 µL of 50% methanol, followed by the addition of 500 µL of Folin-C reagent. After 10 min of incubation, 2.5 mL of Na2CO3 (2% w/v) was added, and the mixture was further incubated for 40 min in the dark. Sample absorbance was measured at 725 nm using the Ultraviolet-visible (UV-Vis) spectrophotometer (United Scientific, SP-UV 300, Shanghai City, China). The results were expressed as milligrams of gallic acid equivalent per mL of PJ (mg GAE/mL PJ) as mean ± S.E.
The DPPH radical-scavenging capacity was determined following the method of Fawole and Opara (Citation2014) with slight modifications. Briefly, 60 µL of PJ extract was mixed with 2.94 mL of absolute methanol and 3 mL of DPPH solution (0.1 mM) in the dark. The solution was thoroughly mixed and incubated for 30 min. Sample absorbance was measured at 517 nm using the UV-Vis spectrophotometer (Spectrum instruments, SP-UV 300, Shanghai City, China). DPPH radical-scavenging capacity was calculated as ascorbic acid (mM) equivalent per mL of PJ and expressed as mean ± S.E.
2.8 Statistical analysis and optimization
The data were analysed using analysis of variance (ANOVA) to determine the experimental variables’ effect and regression coefficients by computing the F-value at 5% significance level. In addition, three-dimensional (3-D) plots were produced for each response variable to better visualize the influence of independent variables on the responses. A polynomial equation was used to predict the response variables using the linear, quadratic, and interaction terms using EquationEquation (1).(1)
(1)
Where b0 is a constant term, bi, bii, and bij are linear, quadratic, and interaction coefficients, respectively. The terms Xi and Xj represent the coded values of independent variables.
The optimal concentrations of independent variables were calculated using numerical and graphical optimization options of Design Expert software version 12 (Statease Inc., MN, U.S.A.). The optimization procedure followed was (i) minimum weight loss and TSS and (ii) maximum TPC and DPPH targeting minimum amounts of SA, tween-80, and canola oil. The best coating formulation with the highest desirability was selected.
3. Results and discussion
3.1 Effect of independent variables on ML
The experimental data for aril ML are presented in , and regression coefficients in . The independent variables showed different effects on ML. The linear terms of canola oil and tween-80 showed significant effects (p < .05) on ML with coefficients of −0.0093 and −0.0106, respectively. The linear term of SA had a coefficient of −0.0051, indicating its insignificant effect on ML. The quadratic terms of canola oil, tween-80 and SA all showed highly significant effects (p < .001) on ML with coefficients of 0.0481, −0.0453 and 0.195, respectively, which suggests that these variables are more effective in their quadratic terms than linear terms. Among the interaction terms, none of the interaction terms had a statistically significant effect on ML.
Table 3. The regression coefficients, mean, R2 and model F-values for the four experimental dependent variables.
The final equation for actual coded factors for ML is presented below.
The model F-value of 39.64 (p < .0001) and non-significant “Lack of Fit” p-value of 0.64 were obtained, indicating the significance (p < .05) and fit of the model for predicting ML under different treatment conditions. The signal-to-noise ratio of 16.22 indicated an adequate signal and suggested that the model can be used to navigate the design space. A typical signal-to-noise ratio greater than 4 is desirable. Furthermore, the high R2 value of 0.92 indicated that the current model explained most of the total variation, with a difference of <0.2 between the predicted R2 (0.74) and adjusted R2 (0.86), which suggests that the used model is highly significant. The high values of R2, predicted R2, and adjusted R2 suggested a strong correlation between the observed and predicted data (Abdelkarim et al., Citation2022; Almusallam et al., Citation2021; Fadimu et al., Citation2020).
ML values range from 1.43% to 1.61%, indicating that the ML is relatively small and varies slightly based on the levels of canola oil, tween-80 and SA (). Thus, the independent variables’ levels can be optimized to achieve the minimum ML of the ready-to-eat pomegranate arils. As shown in , aril ML decreased as the concentration of both canola oil and tween-80 increased. These observations support the negative coefficients of canola oil, SA and tween-80 (). However, a more detailed examination of the pattern () indicates that the concentration levels of these variables contribute to the important effect, with lower ML observed at their optimal levels of 0.078% and 0.075% for canola oil and tween-80, respectively. However, it is worth noting that the efficacy was reduced beyond the optimal region of 0.08% for both independent variables. Using higher concentrations of canola oil and tween-80 causes undesirable effects such as anaerobic respiration, resulting in fermentation and, thus, accelerated senescence, which increases ML (Gu et al., Citation2021).
Figure 1. Interactive effect of canola oil and tween-80 on mass loss of pomegranate arils (cv. ‘Kessari’) (a) 3D-surface plot and (b) contour plot.
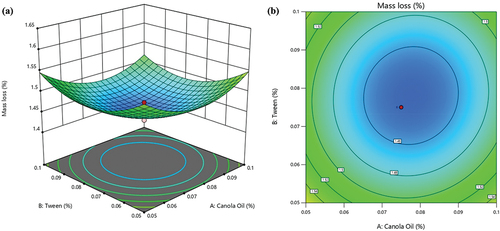
Consistent with our findings regarding the beneficial effects of canola oil and tween-80, Lee et al. (Citation2022) observed that incorporating tween-80 and oregano essential oil decreased oregano oil droplet size in the coating mixture, forming complex mechanisms such as increased surface area. As a result, the coating treatment with oregano oil showed enhanced effectiveness in reducing strawberry fruit weight loss and respiration rate during 6-day storage at 6°C; 25% RH. Further, our findings agree with those of Nandane et al. (Citation2017), who reported a decrease in weight loss of pear fruit with a relative increase in olive oil concentration in soy protein isolate-hydroxypropyl methylcellulose composite coating during 5-day storage at 28°C; 60% RH.
Similarly, Azarakhsh et al. (Citation2014) reported a significant reduction of weight loss in fresh-cut pineapple treated with alginate-lemongrass coating incorporated with sunflower oil (0.025%) during 16-day storage at 10°C; 75% RH. Hernández-López et al. (Citation2020) also reported reduced weight loss of bell peppers coated with nanostructured chitosan edible coating functionalized with canola oil (0.1%) during 21-day storage at 12°C; 89% RH. These results suggest that incorporating vegetable oils in the coating formulation while considering the concentration is a promising approach to reducing fresh produce weight loss.
3.2 Effect of independent variables on TSS
TSS content is one of the critical indicators of quality through its estimate of the total soluble sugars, which are respiratory substrates in pomegranate fruit (Mwelase & Fawole, Citation2022). The independent variables (canola oil, tween-80, and SA) had different impacts on TSS, as shown in . The coefficients for canola oil and tween-80 were −0.0343 and −0.0329, respectively, which indicate their influence on TSS; however, the effect was not statistically significant (p > .05). The quadratic terms of both canola oil and tween-80 significantly affected (p < .05) TSS (0.1470 and 0.0940, respectively), indicating that changes in these variables in quadratic terms can significantly affect TSS than their linear terms. Contrary to ML, the quadratic term for the SA was not significant (p > .05), suggesting that its effect on TSS does not change significantly at different concentrations (). Further, SA had a coefficient of −0.0343, indicating its effect on TSS was also not statistically significant (p > .05). Among the interaction terms, only the AC term showed a significant effect on TSS (coefficient of 0.0525), suggesting that the interaction between canola oil and SA significantly affects TSS.
The final equation of actual coded factors for TSS content is presented below.
This model accuracy is supported by the significant (p < .05) F-value of 14.42, non-significant “lack of fit” p-value of 0.53 and signal-to-noise ratio of 10.95, demonstrating that the model is adequate for predicting TSS under different treatment conditions. Furthermore, a signal-to-noise ratio of 10.95 suggested that the model can be used to navigate the design space. In addition, the high R2 value of 0.92 indicated that the current model explained most of the total variation, with a difference of <0.2 between the predicted R2 (0.74) and adjusted R2 (0.86), which shows a strong correlation between the observed and predicted data. This suggests that the model was accurate.
From , it can be observed that TSS values ranged from 14.20 to 14.73 °Brix under various conditions, signifying that the TSS of the ready-to-eat pomegranate arils can be influenced by varying the levels of canola oil, tween-80 and SA. However, the variation is minimal, so optimizing these independent variables can contribute to achieving a desirable level of TSS in the minimally processed pomegranate product. As shown in , a quadratic increment in TSS content was observed with the increase in the concentration of canola oil and tween-80. This observation supports our earlier findings, demonstrating that the quadratic terms of both canola oil and tween-80 have a significant positive impact (p < .05) on TSS. This suggests an overall increase in TSS levels with increased canola oil and tween-80 concentrations. These results highlight the vital role the additives play in regulating the quality and senescence of ready-to-eat pomegranate arils. Notably, canola oil showed a more pronounced effect on TSS than tween-80. This observation is supported by the highly significant effect (p < .0001) of canola ().
Figure 2. Interactive effect of tween-80 and canola oil on TSS of pomegranate arils (cv. ‘Kessari’) (a) 3D-surface plot and (b) contour plot.
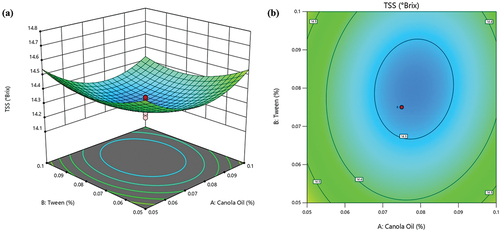
The optimal TSS content was obtained at a concentration of 0.076% (). Beyond this concentration, TSS decreased, which supports the obtained quadratic model ().
Mwelase et al. (Citation2022) and Kawhena et al. (Citation2020) observed an increase in the TSS content of minimally processed pomegranate fresh products with extended storage attributed to high moisture loss, leading to an increased concentration of sugars within the arils. Therefore, the low TSS content with increasing canola oil concentration signifies the effect of oil in the coating mixture to reduce the rate of physiological processes responsible for the senescence of fresh produce. Our results are consistent with those of Hernández-López et al. (Citation2020), who reported delayed TSS changes in bell peppers coated with nanostructured chitosan edible coating functionalized with canola oil (0.1%) during 21-day storage at 12°C; 89% RH. In contrast, Nandane et al. (Citation2017) reported no effect of olive oil concentration on the TSS content of pear fruit during 5-day storage at ambient temperature. However, as previously mentioned, it is worth noting that the efficacy of these additives is significantly diminished beyond the optimal regions.
3.3 Effect of independent variables on TPC
The linear terms of both canola oil and SA showed significant effects (p < .05) on TPC, as indicated by the coefficients 2.20 and −1.97, respectively (). However, tween-80 showed a relatively smaller and insignificant effect, as indicated by the coefficient of 0.7819, suggesting that the concentration of tween-80 has a limited impact on TPC. Considering the interaction terms, the AC term was statistically significant (p < .05) with a coefficient of 2.04, indicating a significant interactive effect of canola oil and SA on TPC, with the remaining interaction terms AB and BC showing an insignificant effect (p > .05) (). The significant interaction involving AC highlights the synergetic effect of canola oil and SA combined, resulting in considerable TPC enhancement.
Among the quadratic terms, canola oil and tween-80 showed highly significant effects (p < .001) with coefficients of −7.73 and −3.79, respectively, indicating that changes in these variables have a significant non-linear effect on TPC (). Further, the quadratic term for SA also exhibited a significant effect (p < .05) as indicated by the coefficient of −2.42, suggesting that its effect on TPC changed notably at different concentrations.
The final equation of actual coded factors for TSS content is presented below.
This model’s fit and accuracy were supported by the significant (p < .05) F-value of 32.82 and non-significant “lack of fit” p-value of 0.139, demonstrating that the model is adequate for predicting TPC under different treatment combinations. The results showed a signal-to-noise ratio of 17.85, suggesting that this model can navigate the design space. Further, the high R2 value of 0.97 was obtained, suggesting that the current model did not explain only 0.03% of the total variation, with the predicted R2 (0.78) in reasonable agreement with the adjusted R2 (0.94), which implies high accuracy of the developed model due to the strong correlation between the observed and predicted values for TPC.
As shown in , the TPC values under different treatment conditions ranged from 70.60 to 96.54 mg GAE/mL PJ. This suggests that altering the levels of canola oil, tween-80, and SA can impact the phenolic content in ready-to-eat pomegranate arils. Given the significant effect of these independent variables, especially canola oil and SA, optimizing their concentrations would be crucial for achieving the desired level of phenolic content in the investigated pomegranate arils. As shown in , a quadratic decrease of TPC was observed with increasing concentrations of canola oil and tween-80. The highest TPC was observed at optimum values of 0.078 and 0.076 for canola oil and tween-80, respectively. However, as previously discussed, it is essential to consider the concentration levels of canola oil, tween-80, and SA when assessing their impact on TPC. The optimal concentration levels for these variables lead to an enhanced TPC, whereas, at extreme concentrations, their effect tends to diminish, as shown by the negative coefficients obtained (). Further, this is in line with our earlier findings, which showed that the quadratic terms of canola oil (A = −7.73), tween-80 (B = −3.79), and SA (C = −2.42) have a significant and negative effect on TPC. This suggests an overall reduction in TPC as the concentrations of canola oil, tween-80, and SA increase. As previously discussed, this adverse effect can largely be attributed to the higher concentration of these compounds, leading to detrimental effects.
Figure 3. Interactive effect of canola oil and tween-80 on TPC of pomegranate arils (cv. ‘Kessari’) (a) 3D-surface plot and (b) contour plot.
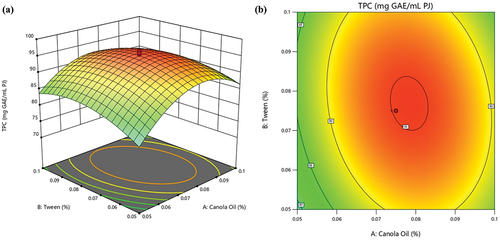
The increase in total phenols is related to the enhanced activity of phenylalanine ammonia-lyase (PAL) (Sayyari et al., Citation2016). In contrast, the decline in total phenols results from the oxidation of these compounds through the enzymatic action of polyphenol oxidase (PPO) using oxygen as a co-substrate (Wu et al., Citation2021). Therefore, the ability of coatings to reduce oxygen intake is understood to inhibit PPO activity, thus maintaining higher total phenols (Sayyari et al., Citation2016). In line with this, the significant interaction between canola oil and SA could be attributable to the synergetic effects of inhibiting PPO activity while enhancing PAL activity. Similarly, higher TPC has been observed in SA-treated papaya (Hanif et al., Citation2020), orange (Rasouli et al., Citation2019) and lime (Tavallali & Zareiyan, Citation2018).
3.4 Effect of independent variables on DPPH
As observed with TPC, the linear terms of both canola oil and SA exhibited significant (p < .05) effects on the DPPH radical scavenging capacity, as shown by the coefficients 0.0312 and −0.0191, respectively (). Tween-80 showed an insignificant effect on DPPH radical scavenging capacity, indicating its inconsiderable effect on the antioxidant system of ready-to-eat pomegranate arils. Notably, none of the interaction terms significantly affected DPPH radical scavenging capacity (). Among the quadratic terms, canola oil showed a highly significant effect (p < .001) on DPPH radical scavenging capacity as indicated by the coefficient of −0.0501, suggesting that changes in lipid composition in the coating formulation have a considerable effect on DPPH radical scavenging capacity ().
The final equation of actual coded factors for DPPH radical scavenging capacity is presented below.
The fit of this model was confirmed by a non-significant “Lack of fit” value of 0.23 and model F-value of 10.66, suggesting that the model is significant (p < .05); and thus, it is suitable for predicting DPPH radical scavenging capacity. The results further showed a high signal-to-noise ratio of 13.46, suggesting that the model can navigate the design space and optimize DPPH radical scavenging capacity. The high R2 value of 0.91 indicated that the current model explained most of the total variation. Moreover, the predicted R2 (0.74) reasonably agreed with the adjusted R2 (0.82), indicating the developed model’s high accuracy in predicting DPPH radical scavenging capacity.
As shown in , the DPPH radical scavenging capacity values under different treatment combinations ranged from 1.09 to 1.33 mM AAE/mL PJ. shows the 3D-surface plots for the interactive effects of canola oil and tween-80 on DPPH radical scavenging capacity. Notably, only canola oil showed a considerable impact on DPPH radical scavenging capacity. This is supported by this variable’s highly significant effect (p < .001) on DPPH radical scavenging capacity. Further, the quadratic term of canola oil was negative (A = −0.0501), suggesting an overall reduction in DPPH radical scavenging capacity with increased concentrations of canola oil. shows that the DPPH radical scavenging capacity increased with increasing canola oil concentrations until reaching a peak at 0.078%, subsequently showing a slight decline, resulting in the non-linearity of the data (). This suggests that extreme concentrations of canola oil are not conducive to improving DPPH radical scavenging capacity. This may be attributed to aerobic respiration as a result of complete inhibition of gaseous exchange. The physicochemical processes that occur during aerobic respiration result in the loss of important bioactive compounds, thereby lowering the antioxidant capacity of fresh produce (Gu et al., Citation2021).
Figure 4. Interactive effect of tween-80 and canola oil on DPPH radical scavenging capacity of pomegranate arils (cv. ‘Kessari’) (a) 3D-surface plot and (b) contour plot.
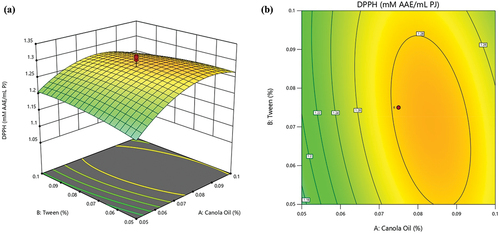
The effect of SA on the antioxidant capacity of fresh produce has also been observed in previous studies. Sinha et al. (Citation2021) reported higher DPPH radical scavenging capacity retention in fruit treated with chitosan incorporated with SA during storage at 1°C; 95% RH for 67 days. Similarly, Khalili et al. (Citation2022) reported that incorporating SA in the coating mixture significantly enhanced the DPPH radical scavenging capacity of grapes. A similar effect of SA has also been observed in mandarin (Haider et al., Citation2020), lime (Tavallali & Zareiyan, Citation2018) and pointed gourd (Yadav et al., Citation2022). SA is reported to enhance the activity of antioxidant enzymes, including catalase, superoxide dismutase and peroxidase, which are the key to improving the fruit’s antioxidant capacity. Furthermore, Sinha et al. (Citation2021) also highlighted the synergistic effect of SA and edible coating, which modify the internal gaseous composition of fresh produce resulting in a reduced rate of metabolomic processes and enhanced retention of polyphenol compounds which also contribute to the antioxidant capacity.
4. Optimization
The optimization process aims to identify the best conditions for the independent variables, in this case, the concentrations of tween-80, canola oil, and SA in the MC-based edible coating, that will optimize the response variables, which are ML, TSS, TPC, and DPPH.
presents the comparison between the experimental values and the predicted values for each of the response variables, which were calculated using the optimized models (EquationEquation (2)–(5)(2)
(2) ). The experimental value for ML was 1.53%, and the model predicted a ML value of 1.48%. Similarly, the experimental value for TSS was 14.46 °Brix, and the predictive value was 14.31 °Brix (). These results suggest that the optimization model can successfully reduce the actual ML and TSS of pomegranate arils, thus, improving quality maintenance during storage. On the other hand, the experimental value for TPC was 85.59 mg GAE/mL PJ, while the predictive value is much higher at 94.16 mg GAE/mL PJ (). The same results were observed for DPPH, with the predictive value (1.31 mM AAE/mL PJ) slightly higher than the experimental value (1.24 mM AAE/mL PJ), suggesting that the optimization model may improve both TPC and DPPH, thus, improving the antioxidant capacity of ready-to-eat pomegranate arils.
Table 4. The experimental and predictive values obtained for pomegranate (cv. “Kessari”) arils treated with MC-based edible coating with addictive of tween-80, canola oil and salicylic acid.
In general, these findings indicate that the optimization model provides relatively close estimates of the experimental values for each response variable, suggesting that the model’s assumptions are reliable and are a good fit for the data. This also indicates that the optimization process using this model can provide meaningful and reliable results for improving the quality management of ready-to-eat pomegranate arils in real-world scenarios. Further, according to RSM analysis, SA (1.55 mM), canola oil (0.079%) and tween-80 (0.079%) were the optimum coating concentrations to improve quality management of ready-to-eat pomegranate arils with a good model desirability of 0.89.
5. Conclusion
In this study, RSM successfully optimized MC edible coating additive’s (SA, tween-80, and canola oil) for improved quality management of ready-to-eat “Kessari” pomegranate arils. Response variables, including ML, TSS, TPC and DPPH radical scavenging capacity, facilitated the development of different mathematical models.
The results showed that the ML was mainly affected by the quadratic terms of canola oil, tween-80 and SA than their linear and interaction terms, which showed slight effects. Similarly, the quadratic terms of both canola oil and tween-80 showed a significant effect on TSS. Notably, TSS was also significantly influenced by the interaction between SA and canola oil. Similarly, TPC was influenced considerably by the linear terms of SA and canola and also the interaction between these terms. In addition, canola oil and SA also had a significant effect on DPPH radical scavenging capacity, where an enhancement in DPPH was observed with increasing canola oil and SA. The results revealed that the optimal concentrations of SA, canola oil and tween-80 were 1.55 mM, 0.079% and 0.079%, respectively. This was accompanied by close estimates of each variable’s experimental and predicted values, suggesting that the model results are reliable and accurate. The model had a good desirability of 0.89, implying that edible coating formulations can be successfully optimized using RSM. However, the optimized concentrations still need to be evaluated on a wide range of fruit responses and properties, including enzyme activities, metabolites, phytochemical contents, and physicochemical properties.
Disclosure statement
No potential conflict of interest was reported by the author(s).
Data availability statement
Data available on request from the authors.
Additional information
Funding
References
- Abdelkarim, D. O., Ahmed, K. A., Younis, M., Yehia, H. M., El-Abedein, A. I. Z., Alhamdan, A., & Ahmed, I. A. M. (2022). Optimization of infrared postharvest treatment of barhi dates using response surface methodology (RSM). Horticulturae, 8(4), 342. https://doi.org/10.3390/horticulturae8040342
- Almusallam, I. A., Mohamed Ahmed, I. A., Babiker, E. E., Al Juhaimi, F. Y., Fadimu, G. J., Osman, M. A., Al Maiman, S. A., Ghafoor, K., & Alqah, H. A. S. (2021). Optimization of ultrasound-assisted extraction of bioactive properties from date palm (Phoenix dactylifera L.) spikelets using response surface methodology. LWT, 140, 110816. https://doi.org/10.1016/j.lwt.2020.110816
- Arendse, E., Fawole, O. A., Magwaza, L. S., Nieuwoudt, H., & Opara, U. L. (2018). Evaluation of biochemical markers associated with the development of husk scald and the use of diffuse reflectance NIR spectroscopy to predict husk scald in pomegranate fruit. Scientia Horticulturae, 232, 240–249. https://doi.org/10.1016/j.scienta.2018.01.022
- Ayranci, E., & Tunc, S. (2004). The effect of edible coatings on water and vitamin C loss of apricots (Armeniaca vulgaris Lam.) and green peppers (Capsicum annuum L.). Food Chemistry, 87(3), 339–342. https://doi.org/10.1016/j.foodchem.2003.12.003
- Azarakhsh, N., Osman, A., Ghazali, H. M., Tan, C. P., & Mohd Adzahan, N. (2014). Lemongrass essential oil incorporated into alginate-based edible coating for shelf-life extension and quality retention of fresh-cut pineapple. Postharvest Biology and Technology, 88, 1–7. https://doi.org/10.1016/j.postharvbio.2013.09.004
- Cazón, P., Velazquez, G., Ramírez, J. A., & Vázquez, M. (2017). Polysaccharide-based films and coatings for food packaging: A review. Food Hydrocolloids, 68, 136–148. https://doi.org/10.1016/j.foodhyd.2016.09.009
- Chaple, S., Vishwasrao, C., & Ananthanarayan, L. (2017). Edible composite coating of methyl cellulose for post-harvest extension of shelf-life of finger hot Indian pepper (Pusa jwala). Journal of Food Processing and Preservation, 41(2), e12807. https://doi.org/10.1111/jfpp.12807
- Fadimu, G. J., Ghafoor, K., Babiker, E. E., Al-Juhaimi, F., Abdulraheem, R. A., & Adenekan, M. K. (2020). Ultrasound-assisted process for optimal recovery of phenolic compounds from watermelon (Citrullus lanatus) seed and peel. Journal of Food Measurement and Characterization, 14(3), 1784–1793. https://doi.org/10.1007/s11694-020-00426-z
- Fawole, O. A., & Opara, U. L. (2013). Seasonal variation in chemical composition, aroma volatiles and antioxidant capacity of pomegranate during fruit development. African Journal of Biotechnology, 12(25). https://doi.org/10.5897/AJB2013.12337
- Fawole, O. A., & Opara, U. L. (2014). Physicomechanical, phytochemical, volatile compounds and free radical scavenging properties of eight pomegranate cultivars and classification by principal component and cluster analyses. British Food Journal, 116(3), 544–567. https://doi.org/10.1108/BFJ-06-2012-0145
- Gu, X., Xue, L., Lu, L., Xiao, J., Song, G., Xie, M., & Zhang, H. (2021). Melatonin enhances the waterlogging tolerance of prunus persica by modulating antioxidant metabolism and anaerobic respiration. Journal of Plant Growth Regulation, 40(5), 2178–2190. https://doi.org/10.1007/s00344-020-10263-5
- Gunasekera, D., Parsons, H., & Smith, M. (2017). Post-harvest loss reduction in Asia-Pacific developing economies. Journal of Agribusiness in Developing and Emerging Economies, 7(3), 303–317. https://doi.org/10.1108/JADEE-12-2015-0058
- Haider, S.-A., Ahmad, S., Sattar Khan, A., Anjum, M. A., Nasir, M., & Naz, S. (2020). Effects of salicylic acid on postharvest fruit quality of “kinnow” mandarin under cold storage. Scientia Horticulturae, 259, 108843. https://doi.org/10.1016/j.scienta.2019.108843
- Hajji, S., Younes, I., Affes, S., Boufi, S., & Nasri, M. (2018). Optimization of the formulation of chitosan edible coatings supplemented with carotenoproteins and their use for extending strawberries postharvest life. Food Hydrocolloids, 83, 375–392. https://doi.org/10.1016/j.foodhyd.2018.05.013
- Hanif, A., Ahmad, S., Shahzad, S., Liaquat, M., & Anwar, R. (2020). Postharvest application of salicylic acid reduced decay and enhanced storage life of papaya fruit during cold storage. Journal of Food Measurement and Characterization, 14(6), 3078–3088. https://doi.org/10.1007/s11694-020-00555-5
- Hernández-López, G., Ventura-Aguilar, R. I., Correa-Pacheco, Z. N., Bautista-Baños, S., & Barrera-Necha, L. L. (2020). Nanostructured chitosan edible coating loaded with α-pinene for the preservation of the postharvest quality of Capsicum annuum L. and alternaria alternata control. International Journal of Biological Macromolecules, 165, 1881–1888. https://doi.org/10.1016/j.ijbiomac.2020.10.094
- Jokar, A., Barzegar, H., Maftoon Azad, N., & Shahamirian, M. (2021). Effects of cinnamon essential oil and persian gum on preservation of pomegranate arils. International Journal of Food Sciences and Nutrition, 9(5), 2585–2596. https://doi.org/10.1002/fsn3.2213
- Kawhena, T. G., Fawole, O. A., & Opara, L. A. (2021). Optimization of gum Arabic and starch-based edible coatings with lemongrass oil using response surface methodology for improving postharvest quality of whole “wonderful” pomegranate fruit. Coatings, 11(4), 442. https://doi.org/10.3390/coatings11040442
- Kawhena, T. G., Tsige, A. A., Opara, U. L., & Fawole, O. A. (2020). Application of gum arabic and methyl cellulose coatings enriched with thyme oil to maintain quality and extend shelf life of “acco” pomegranate arils. Plants (Basel), 9(12), 1690. https://doi.org/10.3390/plants9121690
- Khalili, N., Oraei, M., Gohari, G., Panahirad, S., Nourafcan, H., & Hano, C. (2022). Chitosan-enriched salicylic acid nanoparticles enhanced anthocyanin content in grape (Vitis vinifera L. cv. Red Sultana) berries. Polymers, 14(16), 3349. https://doi.org/10.3390/polym14163349
- Lee, D., Shayan, M., Gwon, J., Picha, D. H., & Wu, Q. (2022). Effectiveness of cellulose and chitosan nanomaterial coatings with essential oil on postharvest strawberry quality. Carbohydrate Polymers, 298, 120101. https://doi.org/10.1016/j.carbpol.2022.120101
- Maftoonazad, N., & Ramaswamy, H. S. (2005). Postharvest shelf-life extension of avocados using methyl cellulose-based coating. LWT-Food Science and Technology, 38(6), 617–624. https://doi.org/10.1016/j.lwt.2004.08.007
- Maftoonazad, N., Ramaswamy, H. S., & Marcotte, M. (2008). Shelf-life extension of peaches through sodium alginate and methyl cellulose edible coatings []. International Journal of Food Science & Technology, 43(6), 951–957. https://doi.org/10.1111/j.1365-2621.2006.01444.x
- Martínez-Romero, D., Castillo, S., Guillén, F., Díaz-Mula, H. M., Zapata, P. J., Valero, D., & Serrano, M. (2013). Aloe vera gel coating maintains quality and safety of ready-to-eat pomegranate arils. Postharvest Biology and Technology, 86, 107–112. https://doi.org/10.1016/j.postharvbio.2013.06.022
- Mditshwa, A., Fawole, O. A., Al-Said, F., Al-Yahyai, R., & Opara, U. L. (2013). Phytochemical content, antioxidant capacity and physicochemical properties of pomegranate grown in different microclimates in South Africa. South African Journal of Plant and Soil, 30(2), 81–90. https://doi.org/10.1080/02571862.2013.802033
- Mohaddese, A., Behnaz, B.-G., Narjes, A., & Amir Daraei, G. (2022). Response surface optimization of the effect of aloe vera gel coating enriched with golpar essential oil on the shelf life, postharvest quality, color change and sensory attributes of fresh-cut orange fruit. Journal of Food Processing and Preservation, 46(11), e17019. https://doi.org/10.1111/jfpp.17019
- Mwelase, S., & Fawole, O. A. (2022). Effect of chitosan-24-epibrassinolide composite coating on the quality attributes of late-harvested pomegranate fruit under simulated commercial storage conditions. Plants (Basel), 11(3), 351. https://doi.org/10.3390/plants11030351
- Mwelase, S., Opara, U. L., & Fawole, O. A. (2022). Relationship between quality attributes of backslopped fermented gari and the sensory and instrumental texture profile of the cooked dough (eba). Journal of Food Processing and Preservation, 46(1), e17096. https://doi.org/10.1111/jfpp.17096
- Nadim, Z., Ahmadi, E., Sarikhani, H., & Amiri Chayjan, R. (2015). Effect of methylcellulose-based edible coating on strawberry fruit’s quality maintenance during storage. Journal of Food Processing and Preservation, 39(1), 80–90. https://doi.org/10.1111/jfpp.12227
- Nandane, A. S., Dave, R. K., & Rao, T. V. R. (2017). Optimization of edible coating formulations for improving postharvest quality and shelf life of pear fruit using response surface methodology. Journal of Food Science and Technology, 54(1), 1–8. https://doi.org/10.1007/s13197-016-2359-9
- Nindjin, C., Beyrer, M., & Amani, G. (2015). Effects of sucrose and vegetable oil on properties of native cassava (Manihot esculenta Crantz) starch-based edible films. African Journal of Food Agriculture Nutrition & Development, 15(69), 9905–9921. https://doi.org/10.18697/ajfand.69.15015
- Nxumalo, K. A., & Fawole, O. A. (2022). Effects of chitosan coatings fused with medicinal plant extracts on postharvest quality and storage stability of purple passion fruit (Passiflora edulis var. Ester). Food Quality and Safety, 6. https://doi.org/10.1093/fqsafe/fyac016
- O’Grady, L., Sigge, G., Caleb, O. J., & Opara, U. L. (2014). Bioactive compounds and quality attributes of pomegranate arils (Punica granatum L.) processed after long-term storage. Food Packaging and Shelf Life, 2(1), 30–37. https://doi.org/10.1016/j.fpsl.2014.06.001
- Özdemir, K. S., & Gökmen, V. (2017). Extending the shelf-life of pomegranate arils with chitosan-ascorbic acid coating. Lwt - Food Science and Technology, 76, 172–180. https://doi.org/10.1016/j.lwt.2016.10.057
- Qambrani, S., Talpur, F. N., Panhwar, A. A., Afridi, H. I., Talpur, M. K., Khan, A., & Hab, S. A. (2022). Development of guar gum-based coating with castor oil for improved postharvest quality of fresh mangoes using response surface methodology. Applied Food Research, 2(2), 100220. https://doi.org/10.1016/j.afres.2022.100220
- Rashid, F., Ahmed, Z., Hussain, S., Kausar, T., Nadeem, M., Ainee, A., & Mehmood, T. (2020). Optimization of fenugreek and flax polysaccharides‐based edible coating formulation to preserve the quality and storability of apple after harvesting. Journal of Food Processing and Preservation, 44(10). https://doi.org/10.1111/jfpp.14812
- Rasouli, M., Saba, M., & Ramezanian, A. (2019). Inhibitory effect of salicylic acid and aloe vera gel edible coating on microbial load and chilling injury of orange fruit. Scientia Horticulturae, 247, 27–34. https://doi.org/10.1016/j.scienta.2018.12.004
- Reinoso, E., Mittal, G. S., & Lim, L.-T. (2007). Influence of whey protein composite coatings on plum (Prunus Domestica L.) fruit quality. Food and Bioprocess Technology, 1(4), 314–325. https://doi.org/10.1007/s11947-007-0014-1
- Rokalla, P., Inbaraj, B. S., Dikkala, P. K., Sridhar, K., Dasi, D. S., Koka, L., Munakala, R., Galipothula, R., Chelli, K. S., & Kalletlapally, N. K. (2022). Active-modified atmosphere packaging of ready-to-eat pomegranate (Punica granatum L.) arils at ambient temperature for extending shelf-life. Agriculture, 12(2), 155. https://doi.org/10.3390/agriculture12020155
- Sayyari, M., Aghdam, M. S., Salehi, F., & Ghanbari, F. (2016). Salicyloyl chitosan alleviates chilling injury and maintains antioxidant capacity of pomegranate fruits during cold storage. Scientia Horticulturae, 211, 110–117. https://doi.org/10.1016/j.scienta.2016.08.015
- Singla, M., Pareek, S., Kumar, N., Sagar, N. A., & Fawole, O. A. (2022). Chitosan-cinnamon oil coating maintains quality and extends shelf life of ready-to-use pomegranate arils under low-temperature storage. Journal of Food Quality, 2022, 3404691. https://doi.org/10.1155/2022/3404691
- Sinha, A., Gill, P. P. S., Jawandha, S. K., Kaur, P., & Grewal, S. K. (2021). Chitosan-enriched salicylic acid coatings preserves antioxidant properties and alleviates internal browning of pear fruit under cold storage and supermarket conditions. Postharvest Biology and Technology, 182, 111721. https://doi.org/10.1016/j.postharvbio.2021.111721
- Tavallali, V., & Zareiyan, F. (2018). Maintenance of physicochemical qualities of lime during cold storage using vacuum infiltration with salicylic acid. Journal of Food Measurement and Characterization, 12(4), 2955–2963. https://doi.org/10.1007/s11694-018-9911-4
- Viuda-Martos, M., Fernandez-Lopez, J., & Perez-Alvarez, J. A. (2010). Pomegranate and its many functional components as related to human health: A review. Comprehensive Reviews in Food Science and Food Safety, 9(6), 635–654. https://doi.org/10.1111/j.1541-4337.2010.00131.x
- Weremfo, A., Abassah-Oppong, S., Adulley, F., Dabie, K., & Seidu-Larry, S. (2023). Response surface methodology as a tool to optimize the extraction of bioactive compounds from plant sources. Journal of the Science of Food and Agriculture, 103(1), 26–36. https://doi.org/10.1002/jsfa.12121
- Wu, D., Zhang, M., Bhandari, B., & Guo, Z. (2021). Combined effects of microporous packaging and nano-chitosan coating on quality and shelf-life of fresh-cut eggplant [Article]. Food Bioscience 43, 101302. Article 101302. https://doi.org/10.1016/j.fbio.2021.101302
- Xing, Y., Yue, T., Wu, Y., Xu, Q., Guo, X., Wang, X., Yang, S., Xu, L., & Yang, P. (2021). Effect of chitosan composite coatings with salicylic acid and titanium dioxide nanoparticles on the storage quality of blackcurrant berries. Coatings, 11(6), 738. https://doi.org/10.3390/coatings11060738
- Yadav, N., Singh, A. K., Emran, T. B., Chaudhary, R. G., Sharma, R., Sharma, S., & Barman, K. (2022). Salicylic acid treatment reduces lipid peroxidation and chlorophyll degradation and preserves quality attributes of pointed gourd fruit. Journal of Food Quality, 2022, 2090562. https://doi.org/10.1155/2022/2090562
- Yousuf, B., & Srivastava, A. K. (2017). Flaxseed gum in combination with lemongrass essential oil as an effective edible coating for ready-to-eat pomegranate arils. International Journal of Biological Macromolecules, 104(Pt A), 1030–1038. https://doi.org/10.1016/j.ijbiomac.2017.07.025
- Zhang, Y., Zhang, M., & Yang, H. (2015). Postharvest chitosan-g-salicylic acid application alleviates chilling injury and preserves cucumber fruit quality during cold storage. Food Chemistry, 174, 558–563. https://doi.org/10.1016/j.foodchem.2014.11.106