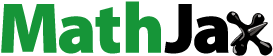
ABSTRACT
This research focused on the preparation in microcapsules of mackerel protein hydrolysates by spray-drying, and their characteristics and stability were analyzed. The result indicated that the optimum conditions for preparing microcapsules were as follows: maltodextrin as the wall material, the ratio of mackerel protein hydrolysates to microporous starch was 2.2:1, and solid concentration was 12% at a temperature of 200°C. The prepared microcapsules had lower water content, better fluidity and persistency. In addition, the microcapsules had less foul odor, higher acceptance, better shape, and more stability.
Introduction
Microcapsule technology can effectively prevent the core material from losing functional activity due to external influences while reducing undesirable characteristic odor or taste (D. L. Li et al., Citation2019). Microencapsulation technology is widely used in food, medicine and agriculture, especially in the food processing industry. The choice of wall material directly impacts the encapsulation effect of microcapsules (Q. H. Yu et al., Citation2017), as maltodextrin, a common natural polymer material, is widely used in encapsulating natural extracts for its high safety. There are many preparation methods for microcapsules, among them, the spray drying embedding method is widely used in the production of functional products for its high embedding rate and compliance with industrial production requirements (Carra et al., Citation2021), such as Tremella fuciformis polysaccharides (Niu et al., Citation2021), nattokinase (G. L. Li et al., Citation2021) and salvianolic acid (Wang et al., Citation2021). It has been reported that highly stable microcapsules can be made of soy skim by spray drying (H. Wang et al., Citation2019).
Mackerel (Pneumatophorus japonicus), a genus of mackerel in the family Scombridae, has a sweet taste and could be used for food and medicine. In recent years, there has been a growing interest in the exploitation of mackerel for its medicinal properties (Afonso et al., Citation2023). Currently, mackerel is mainly used in the production of low-value-added products with a limited amount of further processing (Zhou et al., Citation2015). Low molecular peptides from the enzymatic hydrolysis of mackerel have antioxidant properties (X. Q. Wang et al., Citation2015). However, such low molecular bioactive peptides are sensitive to various environmental influences, which limits their applications (L. Chen et al., Citation2014). With the introduction of microencapsulation technology, these low molecular peptides could effectively maintain their biological activity. At present, there have been reports of peptide microcapsules such as oyster peptides (Zhang et al., Citation2017), casein hydrolysate (Rocha et al., Citation2009), and Phaseolus lunatus peptides (Raúl et al., Citation2019). According to Atefe et al. (Citation2020), maltodextrin and whey protein concentrate encapsulated bee pollen protein hydrolysate by spray drying can form microcapsules that maintain high antioxidant activity after 48 hours of UV irradiation. Meanwhile, the antioxidant activity of mackerel protein hydrolysates microcapsules has not yet been reported.
The following study focuses on preparing mackerel protein hydrolysate microcapsules and their related characteristics and antioxidative stability while also aiming to provide a reference for developing and applying highly active antioxidant peptide products from mackerel.
Materials and methods
Raw materials and chemicals
Mackerel was purchased from the local market (Ningbo, China), with a 300 ± 20 g mass and an average body length of 25 ± 2 cm. Acid protease (5 × 104U/g), pepsin (2.5 × 105 U/g), and trypsin (2.5 × 105 U/g) were purchased from Beijing Solarbio Co., Ltd. Microporous starch of food grade was purchased from Baichuankangze Biotechnology Co., Ltd (Xianyang, China). Maltodextrin of food grade was purchased from Xitangwang Biotechnology Co., Ltd(Xianyang, China). β-cyclodextrin of food grade, NaCl, and absolute ethanol were obtained from Sinopharm Chemical Reagent Co., Ltd (Shanghai, China). 2,2-Diphenyl-1- picrylhydrazyl (DPPH) was purchased from Sigma (St Louis, MO, U.S.A.). All other reagents and organic solvents used were of analytical grade.
Preparation of mackerel protein hydrolysates (MPH)
Mackerel meat (with bones and skin removed) was mixed and homogenized in distilled water (10.65:100, w/w). The hydrolysis process involved adding 0.21% acid protease (based on the weight of acid protease to the weight of the meat, w/w) in a shaking water bath at 53°C, maintaining a pH of 3.0, and allowing the hydrolysis to proceed for 5 h. The enzymatic hydrolysis was then inactivated by boiling the mixture for 15 min, followed by cooling to room temperature. Subsequently, the hydrolysate was centrifuged at 15,000 g for 15 minutes at 4°C, and the supernatant was collected using an centrifuge (5804 R, Eppendorf, Germany). After centrifugation, the resulting material was freeze-dried using an freeze dryer (ALPHA2–4,Christ, Germany) and stored at −20°C for further preparation (Ma et al., Citation2022).
Selection of the microcapsule wall material
The MPH and microporous starch were dispersed in water at 50°C and a ratio of 2:1 (w/w) homogenized for 10 min to form a core material mixture. Then, separately added into the same gelatin mass, β-cyclodextrin and maltodextrin, into the above core materials solution and homogenized for 10 min. The total solid content was kept at 10%. The flow rate of the spray dryer was 20 mL/min, and the inlet air temperature was 200°C to produce microcapsules with different wall materials. The microcapsule powder was stored in plastic tubes at 4°C for encapsulation efficiency analysis.
Optimization of the microencapsulation preparation process
Based on the single-factor experiments, we considered the ratio of MPH and microporous starch (w/w, A), solid content (B, %), and inlet air temperature (C, °C) as independent factors influencing the encapsulation efficiency (EE, %). Each factor was tested at three levels (−1, 0, 1), specifically A (1:1, 2:1, and 3:1), B (5%, 10%, and 15%), and C (190°C, 200°C, and 210°C). A three-level, three-factor Box-Behnken model was employed for the response surface test to optimise the microencapsulation conditions.
Determination of encapsulation efficiency
Encapsulation efficiency (EE) was evaluated as the encapsulated to total peptide content ratio in microcapsule powder. We take an appropriate amount of microcapsule powder in water, centrifuged at 15,000 g for 10 min, and discard the supernatant to remove the mackerel protein hydrolysates that were not embedded on the surface of the microcapsule, and then put the residue in water and ultrasonication for 10 min (PM3-900TL Ultrasound machine, Prima, UK) to fully swell and rupture completely, centrifuged at 15,000 g for 10 min, the supernatant was taken to measure the peptide content in the microcapsules, prepared a solution with the same mass concentration as MPH at the microcapsules. The encapsulation efficiency of the microencapsulated was calculated using the following equation:
Physicochemical characters of microcapsules
Determination of water content in microcapsules
An appropriate amount of microcapsules (m1) was weighed and baked in an oven at 105°C until a constant weight was reached (m2). The water content of the microcapsules was calculated using the following equation:
Determination of Hausner ratio in microcapsules
The Hausner ratio (HR) was determined according to the method of Goyal et al. (Citation2015) with slight modifications. An appropriate weight of microcapsules (m1) was filled in a 10 mL glass cylinder, and the cylinder was slightly tapped to sink the powder sticking to the walls. Directly read the volume (v1) from the glass cylinder, and the bulk density (ρB) was calculated using the following equation:
The tapped density (ρT) determination of the glass cylinder was repeatedly tapped to sink microcapsules power measure tapped volume (v2). The ρT of the microcapsules was calculated using the following equation:
The HR was evaluated as the ratio of the bulk density to tapped density in microcapsule powder and calculated according to the following formula equation:
Determination of angle of repose in microcapsules
The angle of repose (AOR) was determined following the method of Liu and Li (Citation2016) with slight modifications. A measured weight of microcapsules (5 g) was poured through a funnel at a fixed distance from the ground to form a conical heap, and a glass rod was used on the top of the funnel to stir and ensure even distribution of the microcapsules continuously. The height and base radius of the microcapsule heap were then measured, and the AOR was calculated using the following formula:
Determination of hygroscopicity of microcapsules
The hygroscopicity ratio of the microcapsules was determined by referring to the method of D. R. Lin et al. (Citation2022) with slight modifications. An appropriate amount of microcapsules (m1) was weighed and placed in a gas-tight container containing saturated NaCl solution (75% RH) at 25°C. After one week, the sample was weighed (m2). The hygroscopic ratio of the microcapsules was calculated using the following equation:
Determination of particle size and particle size distribution
The particle size distribution of the microcapsule was measured by a laser particle size analyzer (HELOS-OASIS, Sympatec, Germany). The microcapsules were dispersed with pure water, the dispersion pressure was 2.5 bar, the vacuum degree was 82 mbar, and the injection rate was 60%, referring to the method of Shi et al. (Citation2021) and with slight modifications.
Morphology of microcapsules by scanning electron microscope (SEM)
The morphology of microcapsules was determined by referring to the method of Akram and Zhang (Citation2020) with slight modifications. Under a vacuum, the microcapsules were mounted on the double-sided adhesive tape, sprayed with gold, and observed by S-4800 SEM (Hitachi, Japan).
Flavor analysis by electronic nose
Flavor differences between microcapsules and MPH were measured by a PEN 3 electronic nose system(Airsense, Germany), and the method was slightly modified by referring to Ming et al. (Citation2017). 0.5 g samples were accurately weighed, and placed in a 10 mL headspace bottle to enrich odor, and then sealed at room temperature for 60 min before analysis. Detection parameters: self-cleaning time was 60 s, zeroing was 5 s, measurement time was 120 s, and the total sample flow rate was 300 mL/min.
Sensory evaluation by fuzzy mathematics
Refer to Yu et al. ‘s fuzzy mathematics sensory evaluation method (M. Yu et al., Citation2022) with a slight modification for sensory evaluation. Ten assessors selected to form a sensory assessment team carried out the sensory evaluation of the samples. Each factor was taken at three levels/scores (excellent/10 ~ 7, medium/6 ~ 3, and poor/2 ~ 0). The color standard was a uniform color, without black or brown. The odor standard was a pleasing odor without rancidity and a peculiar smell. Moreover, the state standard was an excellent tissue with no foreign matter and agglomeration.
Set of factors and evaluation: taking color (U1), smell (U2), and state (U3) as factors, factors were U = {U1, U2, U3}, the evaluation level of excellent (V1), medium (V2), poor (V3), and the evaluation was V = {V1, V2, V3}. The weight was determined by the combined empowerment method, the importance of each factor was scored before the sensory evaluation, and the weight set of the factors was obtained after the normalization method to X = {X1, X2, X3}.
Establishment of fuzzy matrix and fuzzy transformation, the fuzzy matrix Ri was obtained by calculating the scores of all evaluators for each factor and the fuzzy relationship evaluation as Yi = X×Ri. Establishing the evaluation grade takes the middle value of the three grades as the evaluation grade was K = {8.5,4.5,1}, and the total score of the comprehensive rating Ti = K×Yi.
Stability of microcapsules
Thermogravimetric (TG) analysis of microcapsules
TG analysis was determined by referring to the method of L. Wang et al. (Citation2020) with slight modifications. The thermal stability of microcapsules was determined at 30 ~ 800°C using a thermal gravimetric analyzer (Pyris Diamond TG/DTA, Perkin-Elmer, U.S.A.). The rate of nitrogen gas warming was 10°C/min, and the flow rate was 20 mL/min.
Simulation of in vitro gastrointestinal digestion of microcapsules
Simulation of in vitro gastrointestinal digestion of microcapsules was determined by referring to the method of Cai et al. (Citation2022) with slight modifications. A sample solution with a 10 mg/mL concentration was prepared with the artificial gastric solution and was heat-preserved at 37°C for 10 min. In the gastric phase (6 h), 1% (w/w) pepsin and samples were added every hour. In the intestinal phase (4 h), an equal volume of artificial intestinal solution and 2% (w/w) trypsin were added, and samples were taken every hour. The sample solution was inactivated in boiling water for 10 min and then cooled to room temperature. The supernatant was collected after centrifuged at 15,000 g for 15 min at 4°C.
Refer to the method of Wang et al. (Citation2021) with slight modifications for the determining of DPPH radical scavenging rate. The sample was mixed with an equal volume of DPPH ethanol solution (1 × 10−4 mmol/L) at room temperature for 30 min to determine the absorption value Ay at 517 nm (721 G spectrometer, Jinghua, China). The absorption value A0 was determined when ethanol was instead of DPPH, and the absorption value Ax was determined after water instead of the sample. The DPPH radical scavenging rate was calculated using the following equation:
The antioxidant activity retention rate was calculated using the following equation:
Storage stability of microcapsules
Microcapsules and MPH were stored in a dry place at room temperature (20°C). Samples were taken every 24 h for the first 7 days and every 15 days for the subsequent 90 days storage. The samples’ antioxidant activity retention rate and whiteness index (WI) were compared.
The color of the samples was measured using a CR-400 colorimeter (Konica Minolta, Japan), and the determination of WI referred to the method of Gao et al. (Citation2021) with slight modifications. After calibrating the colorimeter, spread the sample evenly and measure its L*, a*, and b* values at room temperature. The WI was calculated using the following equation:
Statistical analysis
Data were repeated three times, and the results were described as mean±standard deviation (SD) using GraphPad Prism 7 software. Statistical analysis was performed using the Design-Expert software (Version 10.0.7) and SPSS 21 software. A value of p < .05 was considered statistically significant.
Results and discussion
Optimization of the microcapsule embedding process
In the study, the encapsulation efficiency was evaluated by the encapsulation efficiency. The encapsulation efficiency of maltodextrin was 76.90 ± 0.84%, which was significantly higher than that of the other wall materials (Gelatin was 54.79 ± 0.26%, β-cyclodextrin was 41.02 ± 1.05%) (p < .05). Therefore, maltodextrin was selected as the wall material of microcapsules. Mousavi and Ghandiha (Citation2022) used maltodextrin as the wall material to encapsulate the nettle extract by spray drying and prepared microcapsules with high stability under optimal process conditions. FT-IR confirmed that there was no chemical reaction between the core material and maltodextrin.
The porous structure of microporous starch could effectively load active substances and form a mosaic core material. The homogenization process enhanced the dispersion of maltodextrin in the preparation solution. After spray drying, it uniformly covers the core material’s surface to form a low permeability protective layer. So, microporous starch and maltodextrin have higher entrapment rates for mackerel protein hydrolysates.
The design and results of the response surface methodology (RSM) are shown in . The response surface experimental results were analyzed by Design Expert 10.0.7 to obtain the regression equation: EE/% = –7.74A2–6.98B2–4.91C2–1.50AB + 2.45AC + 0.46BC + 3.30A+6.35B–1.34C + 77.06. showed that factors A, B and C significantly affected EE. The p value of the model was highly significant (p < .01), and the p value of the lack of fit was not significant (p = .4736 > .05), which indicated that the model was reliable. In addition, F (B)>F (A)>F (C), indicating that factor B (solid content) had the most significant effect on the embedding rate, followed by factor A (the ratios of MPH and microporous starch) and factor C (inlet air temperature).
Table 1. Design and results of RSM.
Table 2. ANOVA for optimization of the microcapsule embedding process.
The contour and response surface of each factor are shown in . The results showed that the EE of microcapsules increased first and then decreased with the increase of the ratio of peptide, solid content, and inlet air temperature. The response surface of factor A (the ratios of MPH and microporous starch) and factor C (inlet air temperature) was more convex than the interaction of other factors, p = .0045 < .01. The results indicated that the interaction of the two factors had the most significant influence on the EE, followed by factors A and B (solid content) and factors B and C.
Figure 1. Interaction between factor A (the ratios of MPH and microporous starch) and B (solid content), factor A and C (inlet air temperature), factor B and C.
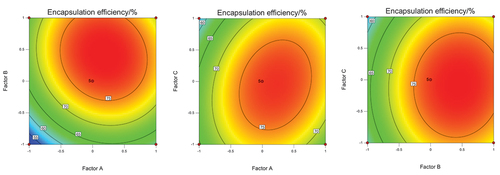
The best parameters of the microcapsule preparation process obtained by the Box-Behnken model were as follows: the ratios of MPH to microporous starch 2.167:1 (w/w), the solid content of 12.06%, and inlet air temperature at 199.58°C. Under these conditions, the EE of microcapsules was 78.61%. In order to facilitate the practical operation, the embedding process of mackerel protein hydrolysate was adjusted to 2.2:1, the ratio of peptide to microporous starch, 12% solid concentration and 200°C temperature. Through three parallel validation tests, the embedding rate was 77.52 ± 0.23%, which was not significantly different from the prediction results of the model, indicating that the model had a high accuracy in predicting the embedding process parameters of mackerel protein hydrolysates.
Analysis of the physicochemical characters of microcapsules
The water content of the microcapsules was 3.00 ± .12%, which met the requirements of 2%~5% water content of the microcapsules products (X. N. Lin, Citation2018). The water was entirely lost during the spray-drying, and the obtained microcapsules had a low water content. This process effectively prevented microorganisms from destroying their structures and helped the microcapsules to be stored for a long time.
The bulk density of the microcapsules was 0.42 ± 0.01 g/cm3, and the tap density was .40 ± .01 g/cm3. The Hausner ratio (HR) was evaluated as the ratio of bulk density to tapped density. The HR and the angle of repose were essential parameters to characterize the fluidity of microcapsules. The fluidity of microcapsules was better when the Hausner ratio was close to 1.0 or the repose angle was minor. The Hannas ratio and the angle of repose of this study were 1.10 and 29.05 ± 0.42 °, which conformed to the characteristics of good liquidity (angle of repose = 29.05 ± 0.42 °<30°) (Liu & Li, Citation2016). That may be because the microporous starch could fully adsorb the mackerel protein hydrolysate to saturation and form a relatively plump core material. After being embedded in maltodextrin, the surface of the microcapsule was relatively smooth, so the microcapsule had good fluidity and a better protection effect on active substances. The hygroscopicity of the microcapsules was 16.27 ± 1.74%; therefore, moisture-proof treatment should be carried out during storage.
Analysis of particle size and particle size distribution
The particle size and distribution of microcapsules are essential parameters for evaluating the quality of microcapsules, which are mainly determined by the properties of core materials, wall materials and preparation process. As shown in , the particle size distribution of the prepared microcapsules was uniform, with 7.50 μm surface mean particle size and 14.04 μm volume mean particle size, which conformed to the Gaussian distribution. The shape of the particle size distribution showed that the particle size uniformity of the microcapsules was high, which was consistent with the analysis results of the Hausner ratio and angle of repose. The particle size and distribution of the microcapsules were affected by the spray dryer’s inlet air temperature and nozzle size. The preparation process of microcapsules included homogenization and atomization treatment. Because of the high degree of dispersion and the formation of smaller droplets through atomization, the microcapsule particle size was smaller than the peony seed polypeptide microcapsules prepared by the sharp hole method (0.7 mm, Qian et al., Citation2022) and the sunflower seeds ACE inhibitory peptide microcapsules prepared by ultrasonic method (80 μm, Luo et al., Citation2020).
Analysis of microcapsules by SEM
The empty microcapsules were irregular and shrivelled in shape, with many surface depressions and voids (). The microcapsules were roughly elliptical and full shape, and the surface was smooth with no obvious cracks and no adhesion of the microcapsule particles (). The morphological comparison between the empty microcapsules and microcapsules could intuitively show that the obtained product was not a simple physical superposition of MPH, microporous starch, and maltodextrin but formed microcapsules with a core-wall structure and a nearly ellipsoidal shape. Due to the high temperature of spray drying, the microencapsulation lost water quickly and led to the formation of slight surface shrinkage, but no obvious deep cracks were found. In conclusion, the microcapsules prepared by spray drying had a high embedding degree to the core material.
Flavor analysis of microcapsules
The flavor differences between microcapsules and MPH were analyzed by electronic nose technology. The contribution of the first principal component of microcapsules (left) and MPH (right) was 99.74%. The contribution of the second principal component was .25%, and the total contribution of the two principal components was 99.99% (), which indicated that the two principal components could effectively reflect the information on the overall odors of microcapsules and MPH (Mo et al., Citation2010). The overall odor of the two substances did not overlap, which indicated that the odor of MPH was significantly changed after microencapsulation.
Sensory evaluation was a comprehensive evaluation of products. Determining chemical indicators was a partial evaluation of product characteristics. Sensory evaluation of products was an indispensable step. Sensory perception is the main factor affecting consumers’ purchase intention. The improved products could better meet the needs of consumers with the sensory evaluation method. The color, odor, and state of microcapsules and MPH were sensory evaluated by a 10-person evaluation team, and the scores were processed by fuzzy mathematics. The microcapsules’ colour was uniform, with no blackening or browning, and its tissue was in a good state with no lumps or odors. However, the color of the MPH was darker than that of the microcapsules. The tissue state of MPH was medium, but the distribution was uneven.
By analyzing the importance ratings of the participants for each index, the quality factor weight sets X = {0.30, 0.40, 0.30}, combined with the fuzzy comprehensive discriminant mathematical model to analyze the participants’ sample ratings: the comprehensive evaluation set of microcapsules Y1 = {0.32, 0.68, 0}, that is the comprehensive score T1 = 5.78; and the comprehensive evaluation set of MPH Y2 = {0.1, 0.76, 0.14} with the comprehensive score T2 = 4.41. Therefore, the microencapsulation of MPH could mask its lousy odor and improve its acceptability.
Stability of microcapsules
TG analysis of microcapsules
Thermogravimetric (TG) analysis of microcapsules refers to the change of the mass of microcapsules with the increase of temperature under the programmed temperature rise, which can characterize the thermal stability of microcapsules in the temperature range. The falling point of the TG weight loss curve is the weight loss area of microcapsules, and the flat point is the platform area of mass. DTG curve obtained by the first derivative of the TG curve, which characterizes the relationship between the weight loss rate of microcapsules and temperature. According to the TG curve in , before the temperature rose to 123.23°C, the mass loss of the mackerel protein hydrolysate microcapsule was about 3.6%, which was close to its water content (3.00 ± 0.12%). Before this temperature, the microcapsule structure was relatively stable, possibly related to the low water content property formed after a short-term high temperature (X. Y. Li et al., Citation2016). The DTG curve was obtained after the first derivation of the TG curve. From the DTG curve, it can be seen that the thermal decomposition of microcapsules consists of three parts. The first part was heated from 123.23°C to 195.73°C, and the maltodextrin in the outer layer began to decompose and reacted violently. In the second part, the temperature rose from 195.73°C to 362.82°C, the core material mixture disintegrated, and its weight loss accelerated. The temperature rose from 362.82°C to 800°C in the third part. In this stage, the core material completely disintegrates and carbonizes with the temperature increase (Y. Chen et al., Citation2022, and the change in microcapsule quality tends to be gentled. To sum up, the microcapsule structure remained stable before it rose to 123.23°C, and the temperature change at this stage had little effect on its stability. In the process of heating from 123.23°C to 800°C, the structure of microcapsules gradually disintegrated until complete carbonization with the increase in temperature.
Simulation of in vitro gastrointestinal digestion of microcapsules
The in vitro gastrointestinal simulation digestion aims to simulate the main digestive enzymes and pH environment human gastrointestinal digestion. The digestive stability of microcapsules can be effectively evaluated by in vitro gastrointestinal simulation digestion. Compared with the in vivo digestion method, the in vitro simulation method has higher safety and repeatability. Therefore, the in vitro gastrointestinal simulation digestion method was used to evaluate the digestive stability of the microcapsules in this study. As shown in , the gastrointestinal simulation digestion in vitro was divided into gastric digestion (1 ~ 6 h) and intestinal digestion (7 ~ 10 h). In the gastric digestion stage, the anti-oxidation activity retention rate of microencapsulated and mackerel protein hydrolysates was not significantly changed (p > .05). In the intestinal digestion stage, the antioxidant activity of microcapsules and mackerel protein hydrolysates decreased significantly with the increase in digestion time (p > .05). However, the decrease in microcapsule activity was much lower than that of mackerel protein hydrolysates, which may be due to the alkaline instability of the hydrolysate, which unfolds the peptide chain under the action of trypsin and further degrades into small peptides or amino acids, resulting in a decrease in its activity (Nongonierma & Fitzgerald, Citation2016). Microcapsules are coated with maltodextrin and microporous starch, which somewhat avoids direct contact between the active substance and intestinal fluid, thus retaining good antioxidant activity. However, under the action of an alkaline environment, trypsin, and shear force, the microcapsule structure is gradually destroyed, and active substances are released. After complete in vitro simulated gastrointestinal digestion (10 hours), the microcapsule activity still decreased, the antioxidant activity of the microencapsulated mackerel protein hydrolysate and the mackerel protein hydrolysate after simulated gastrointestinal digestion maintained 67.48 ± 2.71% and 30 ± 0.67% respectively, indicating that the microencapsulated mackerel protein hydrolysate could significantly improve its digestive stability.
Analysis of storage stability
Storage stability is an important index to evaluate the microcapsules quality. After a long time of storage, the microcapsules will gradually undergo physical and chemical changes, which may lead to the collapse of the microcapsule structure and loss of its related biological activity. The color change of the microcapsule may indicate that the outer wall material of the microcapsule is affected by the storage environment, and consumers’ purchase intention is closely related to the product’s appearance, color and properties. This study discussed the microcapsules and mackerel protein hydrolysates’ antioxidant activity retention rate and color change during storage. It can be seen from that after 7 days of storage (microcapsules and MPH were stored in a dry place at room temperature (20°C) in storage stability research), the antioxidant activity of the microcapsules had no significant change. The antioxidant activity retention rate was 97.70%, while the activity of mackerel protein hydrolysates decreased significantly from the third day (p < .05). The antioxidant activity retention rate was only 40.15% on the seventh day. With the extension of storage time, the antioxidant activity and whiteness of the microcapsules showed a downward trend but still maintained a high antioxidant activity. After 90 days of storage, the antioxidant retention rate was still 75.81 ± 3.78%, indicating that microencapsulation could effectively improve the storage stability of mackerel protein hydrolysates ().
The previous research showed that the microcapsules had low water content (3.00 ± 0.12%), good fluidity (HR 1.10 and angle of repose 29.05 ± 0.42 °), and small particle size (micron level), which could prevent the adhesion between microcapsules and the microorganisms influence during storage. SEM, sensory evaluation and electronic nose analysis showed that the core material was entirely coated by the microencapsulated maltodextrin, which could retain the activity of the core material and effectively improve its storage stability. Thermogravimetric analysis indicated that the microcapsules could maintain the structure integrity before 123.23°C. Even if the storage temperature changed, its stability would be less affected. It was speculated that the outer layer of the microcapsules formed by spray drying at high temperatures was relatively compact, effectively ensuring its core materials’ activity. This result was consistent with the research of Mousavi and Saurabh (Mousavi & Ghandiha, Citation2022; Saurabh et al., Citation2021), who used maltodextrin as the composite wall material and prepared microcapsules with high storage stability through spray drying.
Conclusions
In this study, microcapsules were prepared by spray drying with maltodextrin as the wall material and microporous starch as the adsorption carrier, and their characteristics and stability were also analyzed. The results showed that the optimum conditions for preparing the microcapsules of mackerel protein hydrolysates were at the ratio of peptide to microporous starch 2.2:1, the concentration of solid matter 12% and the temperature 200°C. Under these conditions, the microcapsules were full in shape, and the embedding rate was 77.52 ± 0.23%. In addition, the prepared microcapsules have low water content, good fluidity, good smell, high acceptability, and thermal stability. After microencapsulation, the digestive and storage stability of mackerel protein hydrolysates were significantly improved. The research results provide a specific technical reference for developing and applying highly active peptide products.
Disclosure statement
No potential conflict of interest was reported by the author(s).
Additional information
Funding
References
- Afonso, C., Cardoso, C., Gomes-Bispo, A., Ferreira, I., Rego, A., Coelho, I., Motta, C., Prates, J., Castanheira, I., & Bandarra, N. M. (2023). Fatty acids, selenium, and vitamin B12 in chub mackerel (Scomber colias) as nourishment considering seasonality and bioaccessibility as factors. Food Chemistry, 403, 134455. https://doi.org/10.1016/j.foodchem.2022.134455
- Akram, A. N., & Zhang, C. (2020). Effect of ultrasonication on the yield, functional and physicochemical characteristics of collagen-II from chicken sternal cartilage. Food Chemistry, 307, 125544. https://doi.org/10.1016/j.foodchem.2019.125544
- Atefe, M., Alireza, S. M., Hossein, M., & Vahid, K. (2020). Stability and structural properties of bee pollen protein hydrolysate microencapsulated using maltodextrin and whey protein concentrate. Heliyon, 6(5), e03731. https://doi.org/10.1016/j.heliyon.2020.e03731
- Cai, J. X., Xia, S. S., Ma, J. W., Wang, J. Y., Cao, S. Q., & Qi, X. Y. (2022). Preparation, isolation and stability of collagen ace inhibitory peptides from the skin of Navodon Septentrionalis. Journal of Chinese Institute of Food Science and Technology, 3(22), 225–10. https://d.wanfangdata.com.cn/periodical/ChlQZXJpb2RpY2FsQ0hJTmV3UzIwMjMwODMxEg96Z3NweGIyMDIyMDMwMjUaCGo1ZGRzeGN4
- Carra, J. B., Matos, R. L. N. D., Novelli, A. P., Couto, R. O. D., Yamashita, F., Ribeiro, M. A. D., Meurer Eduardo, C., Verri, W. A., Casagrande, R., Georgetti, S. R., Arakawa, N. S., & Baracat, M. M. (2021). Spray-drying of casein/pectin bioconjugate microcapsules containing grape (Vitis labrusca) by-product extract. Food Chemistry, 368, 130817. https://doi.org/10.1016/j.foodchem.2021.130817
- Chen, L., Zhang, Y., Tan, Y. C., & Lin, Q. L. (2014). Response surface methodology for the optimization of preparation process for rice antioxidant peptide microcapsules by piercing method. Food Science, 35(22), 97–103. https://www.spkx.net.cn/EN/Y2014/V35/I22/97
- Chen, Y., He, N., Yang, T., Cai, S., Zhang, Y., Lin, J., Huang, M., Chen, W., Zhang, Y., & Hong, Z. (2022). Fucoxanthin loaded in palm stearin- and cholesterol-based solid lipid nanoparticle-microcapsules, with improved stability and bioavailability in vivo. Marine Drugs, 20(4), 237–256. https://doi.org/10.3390/md20040237
- Gao, T. X., Deng, S. L., Zhao, X., & Zhou, G. H. (2021). Synergistic effects of polysaccharide and ultrasonic treatment on gel properties of low-salt minced chicken meat. Food Science, 42(19), 148–156. https://www.spkx.net.cn/EN/10.7506/spkx1002-6630-20201102-016
- Goyal, A., Sharma, V., Sihag, M. K., Tomar, S. K., Arora, S., Sabikhi, L., & Singh, A. K. (2015). Development and physico-chemical characterization of microencapsulated flaxseed oil powder: A functional ingredient for omega-3 fortification. Powder Technology, 286, 527–537. https://doi.org/10.1016/j.powtec.2015.08.050
- Li, D. L., Zhu, M. J., Liu, X. M., Wang, Y. T., & Cheng, J. R. (2019). Insight into the effect of microcapsule technology on the processing stability of mulberry polyphenols. LWT, 126, 109144. https://doi.org/10.1016/j.lwt.2020.109144
- Li, G. L., Li, T., He, F., Chen, C., Xu, X., Tian, W. L., Yang, Y., He, X., Li, H., Chen, K. Q., Hao, N., & Ouyang, P. K. (2021). Microencapsulation of nattokinase from fermentation by spray drying: Optimization, comprehensive score, and stability. Food Science and Nutrition, 9(7), 3906–3916. https://doi.org/10.1002/fsn3.2378
- Li, X. Y., Wang, L., Wan, Y., & Xiong, Z. H. (2016). Effect of drying method on physicochemical properties and antioxidant activities of hohenbuehelia serotina polysaccharides. Process Biochemistry, 51(8), 1100–1108. https://doi.org/10.1016/j.procbio.2016.05.006
- Lin, D. R., Xiao, L. J., Li, S. Q., Qin, W., Loy, D. A., Chen, H., & Zhang, Q. (2022). Effects of fructooligosaccharide and soybean protein isolate in the microencapsulation of walnut oil. Industrial Crops and Products, 177, 114431. https://doi.org/10.1016/j.indcrop.2021.114431
- Lin, X. N. (2018). Preparation and microencapsulation of heme iron peptides. Guangzhou, South China Agricultural University, 65–72. https://kns.cnki.net/kcms/detail/detail.aspx?FileName=1018770869.nh&DbName=CMFD2020
- Liu, A. R., & Li, Z. J. (2016). Different wall materials preparation camellia seed oil microcapsules. Journal of the Chinese Cereals and Oils Association, 31(3), 69–73. https://d.wanfangdata.com.cn/periodical/ChlQZXJpb2RpY2FsQ0hJTmV3UzIwMjMwODMxEg96Z2x5eGIyMDE2MDMwMTMaCHk5dmYyNGRs
- Luo, P., Pan, S. Y., & He, D. P. (2020). Preparation of microcapsule of ACE inhibitory peptides derived from sunflower seeds. Cereals & Oils, 33(2), 84–87. https://d.wanfangdata.com.cn/periodical/ChlQZXJpb2RpY2FsQ0hJTmV3UzIwMjMwODMxEg5sc3l5ejIwMjAwMjAyMhoIN3lyMzg4c2E%3D
- Ma, J. W., He, L. Y., Cai, J. X., Xu, L. B., Cao, S. Q., & Qi, X. Y. (2022). Mackerel protein peptide preparation and characteristics of pneumatophorus japonicus. Journal of Food and Fermentation Industry, 48(21), 204–212. http://sf1970.cnif.cn/CN/10.13995/j.cnki.11-1802/ts.029039
- Ming, T. H., Qiu, D. H., Zhou, J., Li, Y., Zhang, C. D., Zhang, D. J., & Su, X. R. (2017). Analysis of the deodorization and aroma during fermentation of grass carp by lactobacillus plantarum. Journal of Chinese Institute of Food Science and Technology, 17(10), 202–210. https://d.wanfangdata.com.cn/periodical/ChlQZXJpb2RpY2FsQ0hJTmV3UzIwMjMwODMxEg96Z3NweGIyMDE3MTAwMjgaCGh0NG5wZjhh
- Mo, X. L., Xu, Y., & Fan, W. L. (2010). Characterization of aroma compounds in Chinese rice wine qu by solvent-assisted flavor evaporation and headspace solid-phase microextraction. Journal of Agricultural and Food Chemistry, 58(4), 2462–2469. https://doi.org/10.1021/jf903631w
- Mousavi, K. S. E., & Ghandiha, S. (2022). Optimization of spray drying parameters for encapsulation of nettle (urtica dioica L.) extract. LWT-Food Science and Technology, 158, 113149. https://doi.org/10.1016/j.lwt.2022.113149
- Niu, B., Feng, S. M., Xuan, S. Q., & Shao, P. (2021). Moisture and caking resistant tremella fuciformis polysaccharides microcapsules with hypoglycemic activity. Food Research International, 146, 110420. https://doi.org/10.1016/j.foodres.2021.110420
- Nongonierma, A. B., & Fitzgerald, R. J. (2016). Strategies for the discovery, identification and validation of milk protein-derived bioactive peptides. Trends in Food Science and Technology, 50(4), 26–43. https://doi.org/10.1016/j.tifs.2016.01.022
- Qian, S. H., Li, F. K., Tu, X. M., Zheng, P., Wang, R. N., & Zhao, S. G. (2022). Preparation, characterization and release characteristics of peony seed polypeptide microcapsule. China Oils & Fats, 1–11. http://kns.cnki.net/kcms/detail/61.1099.TS.20211126.1742.012.html
- Raúl, E. C., Andrea, C. S., Luis, C. G., Silvina, R. D., & David, B. A. (2019). Bioactive Phaseolus lunatus peptides release from maltodextrin/gum Arabic microcapsules obtained by spray drying after simulated gastrointestinal digestion. International Journal of Food Science and Technology, 54(6), 2002–2009. https://doi.org/10.1111/ijfs.14031
- Rocha, G. A., Rocha, G. A., Trindade, M. A., Netto, F. M., & Favaro-Trindade, C. S. (2009). Microcapsules of a casein hydrolysate: Production, characterization, and application in protein bars. Food Science & Technology International, 15(4), 407–413. https://doi.org/10.1177/1082013209346042
- Saurabh, S. P., Heartwin, A. P., Magdaline, E. E. F., Surendra, N. B., & Palanimuthu, V. (2021). Microencapsulation of curcumin by spray drying: Characterization and fortification of milk. Journal of Food Science and Technology, 59(4), 1–15. https://doi.org/10.1007/s13197-021-05142-0
- Shi, Z. D., Jiang, Y. P., Sun, Y. J., Li, F. J., Min, D. D., Zhang, X. H., & Li, X. A. (2021). Preparation and characterization of oregano essential oil microcapsules and its effect on quality preservation of apricot fruit during storage. Food Science, 42(11), 186–194. https://www.spkx.net.cn/EN/Y2021/V42/I11/186
- Wang, H., Sun, Y. F., Li, Y., Tong, X. H., Regenstein, J. M., Huang, Y. Y., Ma, W. J., Sami, R., Qi, B. K., & Jiang, L. Z. (2019). Effect of the condition of spray-drying on the properties of the polypeptide-rich powders from enzyme-assisted aqueous extraction processing. Drying Technology, 37(16), 2105–2115. https://doi.org/10.1080/07373937.2018.1563104
- Wang, L., Li, T., Xin, B., Liu, Y., & Zhang, F. L. (2020). Preparation and characterization of wormwood-oil-contained microcapsules. Journal of Microencapsulation, 37(4), 324–331. https://doi.org/10.1080/02652048.2020.1749320
- Wang, S., Li, Y., Meng, X. Y., Chen, S. W., Huang, D. J., Xia, Y. M., & Zhu, S. (2021). Antioxidant activities of chlorogenic acid derivatives with different acyl donor chain lengths and their stabilities during in vitro simulated gastrointestinal digestion. Food Chemistry, 357, 129904. https://doi.org/10.1016/j.foodchem.2021.129904
- Wang, X. Q., Xing, R. E., Liu, S., Yu, H. H., Li, K. C., & Li, P. C. (2015). Purification and characterization of novel antioxidant peptides of different molecular weights from mackerel pneumatophorus japonicus protein hydrolysate. Chinese Journal of Oceanology and Limnology, 33(1), 159–168. https://doi.org/10.1007/s00343-014-3369-y
- Wang, Y. H., Qu, T., Wang, X. L., & Yang, R. P. (2021). In vitro preparation and evaluation of sustained-release microcapsules of salvianolic acid. Drug Design, Development and Therapy, 15, 1623–1631. https://doi.org/10.2147/DDDT.S294314
- Yu, M., Ma, J. H., Wang, X. H., Lu, M., Fu, X., Zhang, L. C., Shi, T. Y., Xu, L. W., Zhang, L., & Xie, T. M. (2022). Peanut sprout yogurt: Increased antioxidant activity and nutritional content and sensory evaluation by fuzzy mathematics. Journal of Food Processing and Preservation, 46(7), 16663. https://doi.org/10.1111/jfpp.16663
- Yu, Q. H., Cheng, H., Wang, N., Zhi, Z. J., Chen, S. G., & Ye, X. Q. (2017). Research progress on microencapsulation of flavonoids and application in food industry. Journal of Chinese Institute of Food Science and Technology, 17(7), 175–183. https://d.wanfangdata.com.cn/periodical/ChlQZXJpb2RpY2FsQ0hJTmV3UzIwMjMwODMxEg96Z3NweGIyMDE3MDcwMjIaCHRmMXlybnZy
- Zhang, X. K., Zhang, L., Xu, M. Q., Li, Q., & Miao, R. K. (2017). Synthesis of microencapsulated oyster peptides and its effect on inflammatory cytokines and enzyme levels in mice. Journal of Food Measurement and Characterization, 11(2), 610–622. https://doi.org/10.1007/s11694-016-9429-6
- Zhou, J. P., Zhang, W. T., Meng, M., Wang, Y. M., Liu, A. J., & Zheng, J. (2015). Extraction and volatile components of mackerel water-soluble proteins. Food Science, 36(8), 12–18. https://www.spkx.net.cn/EN/Y2015/V36/I8/12