ABSTRACT
Despite its potential benefits, lutein availability can be limited due to inadequate dietary intake of fruits, vegetables, and seaweed. Additionally, lutein faces challenges related to gastrointestinal absorption due to its physicochemical characteristics. To tackle these issues, fortifying staple foods with lutein is proposed, but its low solubility and susceptibility to oxidative degradation present hurdles. Nanoencapsulation technology emerges as a promising solution for improving lutein stability during food processing, storage, and absorption. However, lutein’s stability remains a challenge, with susceptibility to degradation by temperature, UV-light, and oxygen exposure. Food processing involving high temperatures can cause degradation, while specific UV-wavelengths lead to cleavage of lutein molecules. This critical viewpoint carries substantial scientific significance by urging researchers globally to prioritize clinical investigations and the advancement of plant-based lutein nanoencapsulation in basic dietary items. Clinical studies focusing on lutein-plant-based nanoencapsulation approaches are essential to improve its stability and bioaccessibility, ultimately contributing to better vision health.
1. Introduction
Carotenoids are part of the terpenoids pigment family which are abundant in fruits, vegetables, and marine seaweeds, and are linked to several potential health benefits due to their antioxidant and anti-inflammatory properties (Mordi et al., Citation2020). Lutein (PubChem CID 5,281,243; https://pubchem.ncbi.nlm.nih.gov/compound/5281243) is one of the main carotenoids that can selectively accumulate in the eye, macula, and retina and is believed to have an eye protection effect because of its ability to extinguish reactive oxygen species (ROS) and absorb blue light (Balasubramaniam et al., Citation2020; Jain & Sirisha, Citation2020; Roberts & Dennison, Citation2015). According to a 2019 report from the World Health Organization (WHO), approximately 2.2 billion individuals worldwide are affected by visual impairments, with 1 billion of them not having received any form of treatment (Demmin & Silverstein, Citation2020; Tedros Adhanom Ghebreyesus, Citation2019). Following skin melanoma, the second most prevalent form of melanoma is ocular melanoma, which originates from melanocytes found in the conjunctival membrane and uveal tract within the eye (Jovanovic et al., Citation2013). Epidemiological data indicates that consuming lutein is associated with a reduced risk of age-related macular degeneration and cataracts (Pietro et al., Citation2016), as well as providing potential benefits in the fight against cancer and tumors (Kavalappa et al., Citation2021). Lutein is largely contained in carrots, corn, wheat cantaloupe, and orange/yellow paper, in addition to animal sources such as salmon and eggs (Abdel-Aal et al., Citation2013). Seaweed is another widely available lutein source that has not yet been commonly exploited (Fukushima et al., Citation2023). However, lutein is exclusively sourced from food, and its availability is often inadequate due to the limited intake of fruits and vegetables. Moreover, the consumption of seaweed is scarce (even though the lutein composition contained is more abundant). In addition to that, the effectiveness of lutein in biological processes relies heavily on how well it gets absorbed in the gastrointestinal system, and this absorption can be hindered by its physicochemical characteristics (Ciccone et al., Citation2013).
One way to address the challenge of insufficient lutein intake is to create staple foods fortified with lutein, ensuring a regular supply of this compound to the body. In accordance with the WHO guideline 2019, the interventions with fortified food are recommended to prevent the development of ocular diseases, especially for programs engaging large-scale communities (Tedros Adhanom Ghebreyesus, Citation2019). However, simply adding lutein to food offers minimal nutritional benefits because it has low solubility and is prone to oxidative degradation (Roberts & Dennison, Citation2015). Nanoencapsulation technology presents an appealing strategy for encapsulating bioactive compounds within a carrier, enhancing stability during food processing, storage, and gastrointestinal absorption (Pateiro et al., Citation2021; Zaher et al., Citation2022). Microfluidics has gained popularity in innovative food processing, and the emerging application of microfluidic engineering is nutrient nanoencapsulation (Ravichandran, Citation2010). This approach may revolutionize how we handle dispersed food systems and enable precise manipulation of structure at the micro level. Due to its hydrophobic nature, lutein needs to be incorporated into mixed micelles for effective gastrointestinal absorption.
The existing body of research on nutrient nanoencapsulation in conjunction with emulsion-based delivery systems is somewhat limited. Therefore, this critical perspective holds significant scientific importance as it encourages researchers worldwide to focus on clinical research and the development of plant-based lutein nanoencapsulation in staple foods as a means to address nutritional issues related to eye health, particularly in the context of combating ocular melanoma. This initiative aligns with the objectives of the WHO World Report from 2019, which aimed to tackle vision-related challenges and drive action (Demmin & Silverstein, Citation2020), including through innovative research. We hope that this concise critical perspective will support endeavors aimed at reducing the burden of eye-related conditions and vision impairment, ultimately contributing to the achievement of Sustainable Development Goals (SDGs), with a particular emphasis on SDG 3.8, which focuses on universal health coverage through clinical studies on lutein-plant-based nanoencapsulation conducted by researchers worldwide. In essence, this endeavor recognizes the significance of each individual’s vision in the broader context of global health.
2. Search strategy and selection criteria
References for this review were identified via searches of PubMed for articles published until January 2024, using the search scope of “Articles published in English.” Relevant references cited in those articles were reviewed. The terms were as follows: (eye) OR (vision) AND (nanoencapulation) OR (nanotechnology) (melanoma) OR (cancer) OR (ocular melanoma) OR (oncology) AND (lutein)) OR (carotenoids). Relevant articles were identified by searching the authors’ files in Google Scholar, Scopus, ScienceDirect, and the Springer Online Archives Collection.
3. Results and discussions
3.1. Molecular structure of lutein
As a member of xanthophylls in the carotenoids family, lutein molecular structure is derived from a tetraterpene containing two oxygens in the form of hydroxyl (OH) moieties. The molecule contains 40 carbon with conjugated double bonds and has a close similarity with its xanthophyll counterpart – zeaxanthin (Demmig-Adams et al., Citation2022). The molecular structure of lutein in 2D and 3D conformations has been presented in . Lutein is biosynthesized by photosynthesis-dependent organisms or genetically modified bacteria, such as Synechocystis sp. (Lehmann et al., Citation2021), where its pure crystalline isolates tend to have – an orange color appearance. The hydroxyl moieties in the compound could experience esterification, and in the case of marigold-sourced isolate, lutein is commonly found in its esterified form with palmitic acid (Clowutimon et al., Citation2018). In this esterified form, lutein becomes more susceptible to degradation (Martínez-Delgado et al., Citation2017). Thus, to obtain the non-esterified or free form of lutein, a reaction with a strong base using aqueous alcohols is required (Clowutimon et al., Citation2018).
Figure 1. Molecular structure of lutein in 2D (a), and 3D conformations (b). Oxidated derivatives of lutein (c), and SwissADME-generated bioavailability radar (d) (http://www.swissadme.ch/index.php; accessed on 12 March 2023).
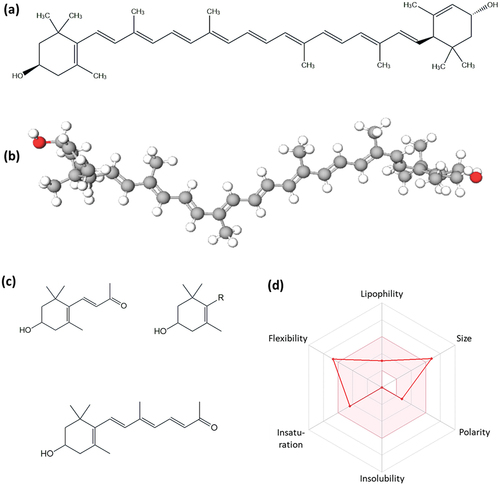
3.2. Stability, bioaccessibility, and bioavailability of lutein
Intake of lutein-rich foods (such as paprika, spinach, parsley, lettuce, kale, and peas) has been evidenced to yield ocular health benefits against, but not limited to, age-related macular degeneration (AMD) and cataracts (Buscemi et al., Citation2018). However, the benefits of lutein from the food source are limited by its bioaccessibility. Upon its entering the gastrointestinal tract, emulsification of lutein into small lipid particles occurs followed by its merging into mixed micelles facilitated by bile salts and biliary phospholipids, where the enterocytes, mediated by scavenger receptor class B type I protein, then plays its role in the micelles absorption (Xavier et al., Citation2018). The bioaccessibility of lutein has been studied in vitro using gastrointestinal digestion models, where the values ranged from 5% to 100% depending on the types of food or drink (Ochoa Becerra et al., Citation2020). Fortification of lutein into a fermented milk provides 100% bioaccesibility of lutein (Granado-Lorencio et al., Citation2010).
The stability of this compound is the limitation of lutein in exerting its health benefits, as the molecule is prone to alteration by the effect of temperature, UV-light exposure, and oxygen exposure. Food processing that requires high temperatures (cooking, drying, frying pasteurization, canning, and so on) could cause the degradation and isomerization in lutein structure (Maiani et al., Citation2009). Degradation of lutein in wheat grain has been observed to occur at temperatures of as low as 40°C, and it increases significantly after ≥80°C (Ahmad et al., Citation2013). Exposure to UV-length at certain wavelengths, namely 200–400 nm, 463 nm, and 365 nm has been reported to cause major cleavage of lutein into smaller molecules (Khalil et al., Citation2012; Kline et al., Citation2011). Lutein may undergo auto-oxidation owing to the exposure to atmospheric oxygen. Moreover, the oxidation of lutein may also take place during the drying process of the lutein-sourced foods. Hence, it is pretty common for lutein to lose its stability owing to oxidation resulting in the production of apocarotenoids (carotenoids with <40 C atoms) (González et al., Citation2011), as presented in .
The bioavailability of lutein is found abundant in the retina, where it can exert its bioactive effects to protect and improve the ocular organ. Other than that, due to its low stability and bioaccessibility, lutein has molecular properties that contribute to its low bioavailability. Based on the bioavailability radar generated by SwissADMET (http://www.swissadme.ch/index.php), lutein has poor bioavailability especially due to its large molecular size and number of rotatable bonds exceeding 9 (). Based on the experimental data, lutein is not soluble in water (logP = 7.9) with a polarity of 40.46 Å (). As the data were derived from in vitro experimental, though it can stimulate the small intestinal digestion, it may fail to make a distinction between bioaccessibility and bioavailability efficiency (Mohammadnezhad et al., Citation2023; Vlaicu et al., Citation2023). In human studies, the bioavailability of Lutein (administered as lutein-fortified milk 200 mL/day for 14 days) was found to be as low as 0.56 µmol/L (Granado-Lorencio et al., Citation2010). Broccoli intake of 200 g/day for 7 days yielded a lutein concentration of 0.30 µM in the plasma and retinal lutein content of 0.91 ± 0.31 ng/g (Granado-Lorencio et al., Citation2007). Nonetheless, researchers have been developing strategies, including nanoencapsulation, to improve the stability, bioaccessibility, and bioavailability of lutein (Kamil et al., Citation2016).
Table 1. Molecular properties of lutein based on SwissADME*.
3.3. General and emerging sources of lutein
Pigmented plant-based foods such as carrots, corn, wheat cantaloupe, and orange/yellow paper, along with animal meats such as salmon and eggs have been suggested as good sources of lutein (Abdel-Aal et al., Citation2013). Eggs contain lutein and other carotenoids, and their contents can be increased by feeding the laying hens with alfalfa meal (Englmaierová et al., Citation2019). Today, lutein could be isolated from other alternative sources as presented in . Marigold flower petals are not traditionally considered the source of nutrients, but recent studies suggest the presence of high lutein in the flower (Dharani et al., Citation2022; Maheshwari et al., Citation2023; Shaikh et al., Citation2023). Lutein content in marigold flowers could reach as high as 35.49% w/w after the supercritical fluid extraction (SCFE) [17]. Endangered Cyclamen sp. flowers have also been investigated for their potential as lutein sources, where the lutein yield reached 44.92—143.91 mg/g dried extract (Cornea-Cipcigan et al., Citation2022). Extraction techniques contribute to the proportion of lutein contained in the extract. More eco-friendly approaches using magnetic nanoparticles (Savvidou et al., Citation2022) and non-ecotoxic solvents (such as the combination of choline chloride and glucose) have been employed (Dharani et al., Citation2022; Savvidou et al., Citation2022).
Table 2. Updated research on lutein isolation from different plant-based sources and its medicinal benefits in vitro.
Moreover, large bodies of evidence have suggested that microalgae could be utilized as the source of carotenoids, including lutein. Chlorella sp. is the commonly reported microalgae used to obtain lutein (Georgiopoulou et al., Citation2022; Ova et al., Citation2022; Patel et al., Citation2022; Wang et al., Citation2023), while others include Haematococcus pluvialis (Savvidou et al., Citation2022), Coccomyxa enuresis (Ruiz-Domínguez et al., Citation2022), and Tetraspora sp (Maswanna & Maneeruttanarungroj, Citation2022). Diatom Phaeodactylum tricornutum is also among the alternative sources of lutein that have been reported (Wang et al., Citation2023). Other than optimizing the extraction parameters, a higher yield could be obtained by changing the algal cultivation parameter (Maswanna & Maneeruttanarungroj, Citation2022; Patel et al., Citation2022). Which, alga could be cultivated in nitrogen-deprived conditions to optimize the biosynthesis of lutein (Maswanna & Maneeruttanarungroj, Citation2022). In the pre-treatment stage, the physical force could be applied to create smaller powder particles that could allow higher extraction yield (Marlina et al., Citation2020). Several examples of this approach are ball-milling (Patel et al., Citation2022) and cryogrinding (Cvitković et al., Citation2022).
Lutein or lutein-rich extracts are good antioxidant and anti-inflammatory agents as proven by multiple studies (Belyagoubi et al., Citation2022; Cornea-Cipcigan et al., Citation2022; Georgiopoulou et al., Citation2022, Citation2023; Liang et al., Citation2023; Maheshwari et al., Citation2023; Ruiz-Domínguez et al., Citation2022; Shaikh et al., Citation2023). A study even reported the anti-inflammatory activity of lutein in the human retinal pigment epithelial cell line (ARPE-19) that was induced by interleukin (IL)-1β. Chlorella sp. extract with lutein of 1.70 mg/g dried extract was shown to inhibit various cancer cell lines in vitro (Sawasdee et al., Citation2023). Because this nutrient is highly abundant in plants and microalgae, more studies should be developed to exploit its medicinal benefits.
3.4. Lutein in the cancer and tumor research (in Vivo/in vitro)
Antitumor activities of lutein were witnessed in 2003 by Chew and colleagues who used mouse models injected with mammary tumor cells (B. P. Chew et al., Citation2003). They found that lutein induces selective apoptosis toward tumor cell and inhibit its angiogenesis () (B. P. Chew et al., Citation2003). Similar to the research performed by other research groups, the viabilities of in vitro cancer cells were found reduced in the lutein-treated sample (Gansukh et al., Citation2019; Liu et al., Citation2016; Luan et al., Citation2021; Omar et al., Citation2021; Rafi et al., Citation2015; Ruiling, Citation2019; Yamagata et al., Citation2017). Lutein-induced apoptosis is mostly evidenced by the upregulation of Bax and downregulation of B-cell lymphoma 2 (Bcl-2) along with the downregulation of Bcl-2/Bax ratio () (B. P. Chew et al., Citation2003; Eom et al., Citation2023; Gansukh et al., Citation2019; Sawasdee et al., Citation2023; Yamagata et al., Citation2017). Downregulation of Bcl-2 is an important step in the initiation of the caspase signaling cascade during apoptosis as the protein is responsible for the formation of apoptosome () (Ola et al., Citation2011). Caspase-3, as the dominant executioner dominant caspase in cell apoptosis (Brentnall et al., Citation2013), has been found positively affected by the introduction of lutein (Eom et al., Citation2023; Gansukh et al., Citation2019; Kavalappa et al., Citation2021; Yamagata et al., Citation2017). Nonetheless, the precursor of this caspase, procaspase-3, has been reported to be overexpressed in various malignancies and contributes positively to the oncogenic transformation as its low-level activity induces subapoptosis instead of apoptosis (Boudreau et al., Citation2019). In this light, lutein has been found as a promising activator of procaspase-3, −8, and −9 and a promoter of caspase enzymatic activities (Sawasdee et al., Citation2023). Furthermore, the presence of lutein impacts positively to the activation of pro-apoptotic nuclear transcription factor p53 () (B. P. Chew et al., Citation2003; Luan et al., Citation2021).
Lutein is also known to induce apoptosis against cancer and tumor cells via oxidative pathways. Photo-oxidized form of lutein could reduce endogenous antioxidants, namely glutathione and malondialdehyde (MDA), that increase the oxidative stress that could inhibit the growth of HeLa cells (Lakshminarayana et al., Citation2010). A study found that exposure of lutein to human buccal mucosa squamous carcinoma BICR10 cells could increase the expression of oxidative stress-responsive kinase-1 (OXSR1) which is a member of serine or threonine protein kinase family (Enășescu et al., Citation2021). Clear evidence of oxidative stress-related apoptosis by lutein in a recent study using gastric cancer AGS cells (Eom et al., Citation2023). The level of reactive oxygen species (ROS) was found to increase concomitant to the activation of NADPH oxidase resulting in apoptosis (based on bax, bcl-2, caspase-3, and cell viability profiles) (Eom et al., Citation2023). The foregoing apoptosis could be reversed by the introduction of N-acetylcysteine acting as an antioxidant with NADPH oxidase inhibition as its mechanism of action (Eom et al., Citation2023). The formation of ROS as a means of lutein-induced apoptosis has also been witnessed in another study using HeLa cell lines (Gansukh et al., Citation2019). Nonetheless, ROS is beneficial in cancer cells during a hypoxic state that stabilizes hypoxia-inducible factor-1α (HIF-1α) further implicating the malignancy (Chandel et al., Citation2000). Treatment using lutein in this state could attenuate the ROS and consequently downregulate the HIF-1α (Y. Li et al., Citation2018).
Additionally, studies have suggested that lutein may impair the growth of cancer and tumor cells through other pathways. Upregulations of nuclear factor erythroid 2-related factor-2 (Nrf-2) and heme oxygenase-1 (HO-1) have been witnessed in lutein-treated HT29 cells, in which these findings are associated with the antiproliferative activity of lutein (Liu et al., Citation2016). A study using multiple cancer cell lines revealed that lutein reduces phosphorylated forms of protein kinase B (AKT) and the mammalian target of rapamycin (mTOR), thereby inhibiting the AKT/mTOR survival signaling (Sawasdee et al., Citation2023). Lutein also disrupts cancer growth by altering the expressions of cancer stem cell-related markers namely SOX-2, NANOG, CD44, CD133, SLCA11, and ABCB1 (Yamagata et al., Citation2017). Lutein could affect the expression of long non-coding RNAs (lncRNAs) and micro RNAs (miRNAs) that are associated with tumor progression (Y. Zhang et al., Citation2021). As a combinatorial therapy with doxorubicin, lutein may increase its efficacy whilst reducing its adverse inflammatory effects (Luan et al., Citation2021). Findings of lutein as anticancer and antitumor agents as reported by multiple in vitro/in vivo studies have been summarized in .
Table 3. Recent studies (in vivo/in vitro) of lutein as an anticancer or antitumor.
3.5. Evidence from clinical studies
In clinical studies, researchers have explored multiple clinical benefits of lutein. In a systematic review, diatery lutein was found to increase cognitive function among healthy adults (Nouchi et al., Citation2020) but a more recent meta-analysis conclude that the improvement was not significant (J. Li & Abdel‐Aal, Citation2021). In a small-scale randomized controlled trial, supplementation of dietary lutein was proven to increase eye health among healthy subjects based on macular pigment optical density, contrast sensitivity, as well as glare sensitivity (Machida et al., Citation2020). Food enrichment with lutein, in a long-term intervention, was found to be significant in increasing macular pigment optical density (Schnebelen-Berthier et al., Citation2021), as well as preventing age-related macular degeneration (E. Y. Chew et al., Citation2022). As previously discussed, lutein has potent activity against cancer and tumor cells. Therefore, its potential as a preventive and therapeutic agent against cancer and tumors is worth receiving a spotlight.
In human subjects, a study suggests that the risk of colorectal cancer could be avoided by consuming lutein, where the mechanism is thought to be interplayed with DICER1 rs3742330 polymorphism (Kim et al., Citation2019). Since lutein is among the only two dietary carotenoids (the other one being zeaxanthin) that could be accumulated in the retina, it is thought to be a valuable bioactive compound to treat ocular melanoma (Buscemi et al., Citation2018). Unfortunately, there is still little evidence on the role of lutein as a therapeutic or preventive agent against ocular melanoma. Zeaxanthin, an isomer of lutein, has been found active against uveal melanoma C918 cells by showing inhibitory activities against the productions of matrix metalloproteinase (MMP)-2 and NF-κB (Bi et al., Citation2016). The evidence from an in vivo study also suggests zeaxanthin’s preventive effect against uveal melanoma cell growth (Xu et al., Citation2015). A randomized controlled trial (n = 42) revealed that supplementation of lutein, zeaxanthin, and meso-zeaxanthin could improve the macular pigment optical density (MPOD) in patients with glaucoma (Loughman et al., Citation2021). However, the evidence is considered insufficient as the sample size was too small for statistical interpretation and the duration was too short for visual field assessment (Loughman et al., Citation2021). Moreover, the benefit of lutein supplementation against ocular melanoma might be limited by its bioaccessibility and bioavailability. Future research should employ strategies that could overcome the foregoing limitations, which is through nanoencapsulation. Lutein in the form of nanoparticles has been shown with higher efficacy against buccal mucosa squamous BICR10 carcinoma cells as compared to its normal-sized form (Enășescu et al., Citation2021).
Finally, it is critical to create more efforts in improving clinical studies that focus on the stability and bioaccessibility of lutein for health vision via lutein-plant-based nanoencapsulation approaches, especially to combating ocular melanoma.
3.6. Potential of nanoencapsulation technologies for lutein delivery
Being acknowledged as a modern food technology, nano encapsulation has in fact been reported to improve the stability and bioavailability of various bioactive compounds including fruit extracts, ferulic acid, epigallocatechin-3-gallate, quercetin, curcumin, and other phenolic compounds (Tahir et al., Citation2021). In a recent study, essential oils extracted from Origanum glandulosum Desf. was evidenced to have increased cytotoxicity against Hep-G2 cells in its nanocapsules form, though its DPPH antioxidant activity was reduced (Ali et al., Citation2020). In a more comprehensive study, the encapsulation approach through complexation with pea protein nanofibrils resulted in higher chemical stability and water stability (Yi et al., Citation2022). More importantly, its anticancer activity against Caco-2 cells was improved significantly (Yi et al., Citation2022).
In terms of carotenoids, encapsulation has been used to increase the efficacy of lycopene where it has been proven in vitro against breast cancer cells (Vasconcelos et al., Citation2020). Interestingly, the lipid-based nanoencapsulated lycopene did not exhibit hemolytic activity against erythrocytes (Vasconcelos et al., Citation2020). Lutein was reported to have exceptional increases in bioaccessibility along with hepatic and ocular accumulations in rat model after encapsulated in a mixed nanoemulsion of oleic and linoleic acid (Toragall et al., Citation2021). Nano-scale delivery system of lutein, which was derived from combination of liposomes and chitosan, was able to increase the endogenous antioxidant activity in the liver of mice (G. Zhang et al., Citation2023). A study reported that a nanoencapsulated lutein has a cytotoxicity against Caco-2 cells which was negatively correlated with the lutein solubility (Luo et al., Citation2024). The role of balancing the solubility and permeability of lutein via nanoencapsulation in optimizing its anticancer activity has been reported in a study using Caco-2 cells in vitro (Luo et al., Citation2024). Strategies in encapsulating lutein can be performed through various nano-scale systems including that employing nanoemulsions, polymeric nanoparticles, polymer-lipid nanoparticles, or limposomes, where more details on these strategies have been discussed previously (Algan et al., Citation2022; Y. Li et al., Citation2024; Vieira & Conte-Junior, Citation2022). Taken altogether, nanoencapsulation could increase the bioaccessibility, bioavailability, and bioaccumulation of lutein but its efficacy is varied, hence requiring further studies.
4. Conclusions
Lutein, a member of the carotenoid family, has gained attention for its potential health benefits, particularly in the context of eye health and its role in combating ocular melanoma. Lutein, which can be found in various fruits, vegetables, and marine seaweeds, is known for its antioxidant and anti-inflammatory properties. It selectively accumulates in the eye, particularly in the macula and retina, offering potential protection against oxidative damage and blue light exposure. Research and epidemiological data have suggested that lutein intake may lower the risk of age-related macular degeneration, cataracts, and potentially even certain cancers and tumors. However, lutein is primarily sourced from food, and its availability can be limited due to dietary choices. Additionally, its effectiveness in biological processes depends on efficient gastrointestinal absorption (Graphical Abstract), which can be hindered by its physicochemical properties.
To address the challenge of insufficient lutein intake, the development of staple foods fortified with lutein has been proposed, to ensure a consistent supply of this compound to individuals. However, simply adding lutein to food may not provide significant nutritional benefits due to its poor solubility and vulnerability to oxidative degradation. Nanoencapsulation technology emerges as a promising strategy to enhance the stability, bioavailability, and bioaccessibility of lutein in food products. Furthermore, microfluidic engineering is gaining traction as an innovative approach to nutrient nanoencapsulation, offering the potential for precise control of micro-level structures in dispersed food systems. This critical perspective underscores the importance of clinical research and the development of plant-based lutein nanoencapsulation in staple foods to address nutritional challenges related to eye health, including the prevention and treatment of ocular melanoma. This aligns with the objectives of the WHO World Report from 2019, which emphasized the importance of research and innovation in tackling vision-related issues. The hope is that this endeavor will contribute to reducing the burden of eye-related conditions and vision impairment, ultimately supporting the achievement of Sustainable Development Goal 3.8, which focuses on universal health coverage through clinical studies on lutein-plant-based nanoencapsulation conducted by researchers worldwide.
Furthermore, this text provides insight into the molecular structure of lutein, its sources, stability, and bioavailability. It highlights the potential benefits of lutein as an antioxidant and anti-inflammatory agent, as well as its role in inducing apoptosis in cancer and tumor cells through various pathways. While there is promising evidence of lutein’s therapeutic potential, especially in preventing and treating certain cancers and ocular health issues, further research is needed to address issues related to its stability and bioavailability. In summary, lutein shows great promise as a bioactive compound with a wide range of potential health benefits, and efforts to improve its delivery and efficacy through nanoencapsulation are essential for future research and clinical applications. Lutein intake is recommended to maintain eye health with possibility of preventing ocular melanoma. The absence of clinical studies on nanoecapsulated lutein for ocular melanoma management present the opportunity for further research in this topic.
Author contributions
Conceptualization, Fahrul Nurkolis, Muhammad Iqhrammullah, Hardinsyah Hardinsyah and Nurpudji Astuti Taslim; Data curation, Fahrul Nurkolis, Rony Abdi Syahputra and Andi Yasmin Syauki; Formal analysis, Fahrul Nurkolis, Muhammad Iqhrammullah, Rudy Kurniawan, Dionysius Subali, Happy Kurnia Permatasari and Andi Yasmin Syauki; Investigation, Rudy Kurniawan and Dionysius Subali; Methodology, Fahrul Nurkolis and Muhammad Iqhrammullah; Software, Reggie Surya and Vika Anggari; Supervision, Dian Aruni Kumalawati, Happy Kurnia Permatasari, Raymon R. Tjandrawinata, Nelly Mayulu, Hardinsyah Hardinsyah, Nurpudji Astuti Taslim and Bonglee Kim; Validation, Happy Kurnia Permatasari, Raymon R. Tjandrawinata, Nelly Mayulu, Hardinsyah Hardinsyah, Nurpudji Astuti Taslim and Bonglee Kim; Visualization, Fahrul Nurkolis, Muhammad Iqhrammullah, Faqrizal Ria Qhabibi and Vika Anggari; Writing – original draft, Fahrul Nurkolis, Muhammad Iqhrammullah, Rudy Kurniawan, Hardinsyah Hardinsyah and Nurpudji Astuti Taslim; Writing – review & editing, Fahrul Nurkolis, Muhammad Iqhrammullah, Rony Abdi Syahputra, Dionysius Subali, Reggie Surya, Dian Aruni Kumalawati, Faqrizal Ria Qhabibi, Raymon R. Tjandrawinata and Bonglee Kim. More specific, Dr. Happy Kurnia Permatasari is an expertise in Molecular and Biochemistry of Cancer and is responsible for writing section of cancer mechanism.; Prof. Dr. Bonglee Kim has a strong background in Anticancer of Herbal Medicine and is responsible for writing and revising section 5 and edited main article.; Prof. Dr. Hardinsyah Hardinsyah., Prof. Dr. Nurpudji Astuti Taslim., Dr. Nelly Mayulu, and Dr. Andi Yasmin Syauki has a strong background in Nutrition and Functional Food from Natural Product and is responsible for writing and revising Lutein. All authors have read and agreed to the published version of the manuscript.
Acknowledgment
We offer a great thank you to the Chairman of the Indonesian Association of Clinical Nutrition Physicians, Professor Nurpudji Astuti Taslim, MD., MPH., PhD., Sp.GK(K), and the President of the Federation of Asian Nutrition Societies (FANS), Professor Hardinsyah, Ph.D., for reviewing and providing suggestions, as well as input on the draft of this opinion article.
Disclosure statement
No potential conflict of interest was reported by the author(s).
Data availability statement
There is no data related to this review article. The data were only sourced from the literature listed in this article (Data included in article/supp. material/referenced in article).
Additional information
Funding
References
- Abdel-Aal, E. S. M., Akhtar, H., Zaheer, K., & Ali, R. (2013). Dietary sources of lutein and zeaxanthin carotenoids and their role in eye health. Nutrients, 5(4), 1169–11. MDPI AG. https://doi.org/10.3390/nu5041169
- Ahmad, F. T., Asenstorfer, R. E., Soriano, I. R., & Mares, D. J. (2013). Effect of temperature on lutein esterification and lutein stability in wheat grain. Journal of Cereal Science, 58(3), 408–413. https://doi.org/10.1016/j.jcs.2013.08.004
- Algan, A. H., Gungor-Ak, A., & Karatas, A. (2022). Nanoscale delivery systems of lutein: An updated review from a pharmaceutical perspective. Pharmaceutics, 14(9), 1852. Multidisciplinary Digital Publishing Institute. https://doi.org/10.3390/pharmaceutics14091852
- Ali, H., Al-Khalifa, A. R., Aouf, A., Boukhebti, H., & Farouk, A. (2020). Effect of nanoencapsulation on volatile constituents, and antioxidant and anticancer activities of Algerian Origanum glandulosum Desf. essential oil. Scientific Reports, 10(1), 1–9. https://doi.org/10.1038/s41598-020-59686-w
- Balasubramaniam, V., June Chelyn, L., Vimala, S., Mohd Fairulnizal, M. N., Brownlee, I. A., & Amin, I. (2020). Carotenoid composition and antioxidant potential of Eucheuma denticulatum, Sargassum polycystum and Caulerpa lentillifera. Heliyon, 6(8), e04654. https://doi.org/10.1016/j.heliyon.2020.e04654
- Belyagoubi, L., Belyagoubi-Benhammou, N., Atik-Bekkara, F., & Abdelouahid, D. E. (2022). Influence of harvest season and different polarity solvents on biological activities, phenolic compounds and lipid-soluble pigment contents of Spirogyra sp. from Algeria. Advances in Traditional Medicine, 22(2), 359–369. https://doi.org/10.1007/s13596-021-00551-0
- Bi, M.-C., Hose, N., Xu, C.-L., Zhang, C., Sassoon, J., & Song, E. (2016). Nonlethal levels of zeaxanthin inhibit cell migration, invasion, and secretion of MMP-2 via NF-κB pathway in cultured human uveal melanoma cells. Journal of Ophthalmology, 2016, 1–8. https://doi.org/10.1155/2016/8734309
- Boudreau, M. W., Peh, J., & Hergenrother, P. J. (2019). Procaspase-3 overexpression in cancer: A paradoxical observation with therapeutic potential. ACS Chemical Biology, 14(11), 2335–2348. https://doi.org/10.1021/acschembio.9b00338
- Brentnall, M., Rodriguez-Menocal, L., De Guevara, R. L., Cepero, E., & Boise, L. H. (2013). Caspase-9, caspase-3 and caspase-7 have distinct roles during intrinsic apoptosis. BMC Cell Biology, 14(1), 32. https://doi.org/10.1186/1471-2121-14-32
- Buscemi, S., Corleo, D., DiPace, F., Petroni, M. L., Satriano, A., & Marchesini, G. (2018). The effect of lutein on eye and extra-eye health. Nutrients, 10(9). https://doi.org/10.3390/nu10091321
- Chandel, N. S., McClintock, D. S., Feliciano, C. E., Wood, T. M., Melendez, J. A., Rodriguez, A. M., & Schumacker, P. T. (2000). Reactive Oxygen Species Generated at Mitochondrial Complex III Stabilize Hypoxia-inducible Factor-1α during Hypoxia: A mechanism of O2 sensing*. Journal of Biological Chemistry, 275(33), 25130–25138. https://doi.org/10.1074/jbc.M001914200
- Chew, B. P., Brown, C. M., Park, J. S., & Mixter, P. F. (2003). Dietary lutein inhibits mouse mammary tumor growth by regulating angiogenesis and apoptosis. Anticancer Research, 23(4), 3333–3339.
- Chew, E. Y., Clemons, T. E., Agrón, E., Domalpally, A., Keenan, T. D. L., Vitale, S., Weber, C., Smith, D. C., Christen, W., SanGiovanni, J. P., Ferris, F. L., Danis, R. P., Blodi, B. A., Ruby, A. J., Antoszyk, A., Klein, M., Kim, I., Fish, G. E. & DiLoreto, D. (2022). Long-term outcomes of adding lutein/zeaxanthin and ω-3 fatty acids to the AREDS supplements on age-related macular degeneration progression: AREDS2 report 28. JAMA Ophthalmology, 140(7), 692–698. https://doi.org/10.1001/jamaophthalmol.2022.1640
- Ciccone, M. M., Cortese, F., Gesualdo, M., Carbonara, S., Zito, A., Ricci, G., De Pascalis, F., Scicchitano, P., & Riccioni, G. (2013). Dietary intake of carotenoids and their antioxidant and anti-inflammatory effects in cardiovascular care. Mediators of Inflammation, 2013, 1–11. Hindawi Publishing Corporation. https://doi.org/10.1155/2013/782137
- Clowutimon, W., Shotipruk, A., Boonnoun, P., & Ponpesh, P. (2018). Development of mass transfer model for chromatographic separation of free lutein and fatty acids in de-esterified marigold lutein. Food and Bioproducts Processing, 110, 6–15. https://doi.org/10.1016/j.fbp.2018.04.003
- Cornea-Cipcigan, M., Bunea, A., Bouari, C. M., Pamfil, D., Páll, E., Urcan, A. C., & Mărgăoan, R. (2022). Anthocyanins and carotenoids characterization in flowers and leaves of cyclamen genotypes linked with bioactivities using multivariate analysis techniques. Antioxidants, 11(6), 1126. https://doi.org/10.3390/antiox11061126
- Cvitković, D., Lisica, P., Zorić, Z., Pedisić, S., Repajić, M., Dragović-Uzelac, V., & Balbino, S. (2022). The influence of cryogrinding on essential oil, phenolic compounds and pigments extraction from Myrtle (Myrtus communis L.) leaves. Processes, 10(12), 2716. https://doi.org/10.3390/pr10122716
- Demmig-Adams, B., Polutchko, S. K., & Adams, W. W., III. (2022). Structure-function-environment relationship of the isomers zeaxanthin and lutein. Photochem, 2(2), 308–325. https://doi.org/10.3390/photochem2020022
- Demmin, D. L., & Silverstein, S. M. (2020). Visual impairment and mental health: Unmet needs and treatment options. Clinical Ophthalmology, 14, 4229–4251. https://doi.org/10.2147/OPTH.S258783
- Dharani, S., Ramalingam, P., Basavaraju, S., & Saraswathy, N. (2022). Extraction of lutein from targetes erecta using deep eutectic solvents. AIP Conference Proceedings, 2446. https://doi.org/10.1063/5.0110158
- Enășescu, D. A., Moisescu, M. G., Imre, M., Greabu, M., Ripszky Totan, A., Stanescu-Spinu, I., Burcea, M., Albu, C., & Miricescu, D. (2021). Lutein treatment effects on the redox status and metalloproteinase-9 (MMP-9) in oral cancer squamous cells—are there therapeutical hopes? Materials, 14(11), 2968. https://doi.org/10.3390/ma14112968
- Englmaierová, M., Skřivan, M., & Vít, T. (2019). Alfalfa meal as a source of carotenoids in combination with ascorbic acid in the diet of laying hens. Czech Journal of Animal Science, 64(1), 17–25. https://doi.org/10.17221/116/2018-CJAS
- Eom, J. W., Lim, J. W., & Kim, H. (2023). Lutein induces reactive oxygen species-mediated apoptosis in gastric cancer AGS cells via NADPH oxidase activation. Molecules, 28(3), 1178. https://doi.org/10.3390/molecules28031178
- Fukushima, Y., Taguchi, C., Kishimoto, Y., & Kondo, K. (2023). Japanese carotenoid database with α- and β-carotene, β-cryptoxanthin, lutein, zeaxanthin, lycopene, and fucoxanthin and intake in adult women. International Journal for Vitamin and Nutrition Research, 93(1), 42–53. https://doi.org/10.1024/0300-9831/a000707
- Gansukh, E., Mya, K. K., Jung, M., Keum, Y.-S., Kim, D. H., & Saini, R. K. (2019). Lutein derived from marigold (tagetes erecta) petals triggers ROS generation and activates Bax and caspase-3 mediated apoptosis of human cervical carcinoma (HeLa) cells. Food and Chemical Toxicology, 127, 11–18. https://doi.org/10.1016/j.fct.2019.02.037
- Georgiopoulou, I., Tzima, S., Louli, V., & Magoulas, K. (2022). Supercritical CO2 extraction of high-added value compounds from chlorella vulgaris: Experimental design, modelling and optimization. Molecules, 27(18), 5884. https://doi.org/10.3390/molecules27185884
- Georgiopoulou, I., Tzima, S., Louli, V., & Magoulas, K. (2023). Process optimization of microwave-assisted extraction of chlorophyll, carotenoid and phenolic compounds from chlorella vulgaris and comparison with conventional and supercritical fluid extraction. Applied Sciences, 13(4), 2740. https://doi.org/10.3390/app13042740
- González, I. A., Osorio, C., Meléndez-Martínez, A. J., González-Miret, M. L., & Heredia, F. J. (2011). Application of tristimulus colorimetry to evaluate colour changes during the ripening of Colombian guava (Psidium guajava L.) varieties with different carotenoid pattern. International Journal of Food Science & Technology, 46(4), 840–848. https://doi.org/10.1111/j.1365-2621.2011.02569.x
- Granado-Lorencio, F., Herrero-Barbudo, C., Olmedilla-Alonso, B., Blanco-Navarro, I., & Pérez-Sacristán, B. (2010). Lutein bioavailability from lutein ester-fortified fermented milk: In vivo and in vitro study. The Journal of Nutritional Biochemistry, 21(2), 133–139. https://doi.org/10.1016/j.jnutbio.2008.12.002
- Granado-Lorencio, F., Olmedilla-Alonso, B., Herrero-Barbudo, C., Pérez-Sacristán, B., Blanco-Navarro, I., & Blázquez-García, S. (2007). Comparative in vitro bioaccessibility of carotenoids from relevant contributors to carotenoid intake. Journal of Agricultural and Food Chemistry, 55(15), 6387–6394. https://doi.org/10.1021/jf070301t
- Jain, A., & Sirisha, V. L. (2020). Algal Carotenoids. Encyclopedia of Marine Biotechnology, 33–64. John Wiley & Sons, Ltd. https://doi.org/10.1002/9781119143802.ch2
- Jovanovic, P., Mihajlovic, M., Djordjevic-Jocic, J., Vlajkovic, S., Cekic, S., & Stefanovic, V. (2013). Ocular melanoma: An overview of the current status. International Journal of Clinical and Experimental Pathology, 6(7), 1230–1244. e-Century Publishing Corporation. /pmc/articles/PMC3693189/
- Kamil, A., Smith, D. E., Blumberg, J. B., Astete, C., Sabliov, C., & Oliver Chen, C.-Y. (2016). Bioavailability and biodistribution of nanodelivered lutein. Food Chemistry, 192, 915–923. https://doi.org/10.1016/j.foodchem.2015.07.106
- Kavalappa, Y. P., Gopal, S. S., & Ponesakki, G. (2021). Lutein inhibits breast cancer cell growth by suppressing antioxidant and cell survival signals and induces apoptosis. Journal of Cellular Physiology, 236(3), 1798–1809. https://doi.org/10.1002/jcp.29961
- Khalil, M., Raila, J., Ali, M., Islam, K. M. S., Schenk, R., Krause, J.-P., Schweigert, F. J., & Rawel, H. (2012). Stability and bioavailability of lutein ester supplements from tagetes flower prepared under food processing conditions. Journal of Functional Foods, 4(3), 602–610. https://doi.org/10.1016/j.jff.2012.03.006
- Kim, J., Lee, J., Oh, J. H., Chang, H. J., Sohn, D. K., Kwon, O., Shin, A., & Kim, J. (2019). Dietary lutein plus zeaxanthin intake and DICER1 rs3742330 a > G polymorphism relative to colorectal cancer risk. Scientific Reports, 9(1). https://doi.org/10.1038/s41598-019-39747-5
- Kline, M. A., Duncan, S. E., Bianchi, L. M., Eigel William Nicholas, I. I. I., & O’Keefe, S. F. (2011). Light wavelength effects on a lutein-fortified model colloidal beverage. Journal of Agricultural and Food Chemistry, 59(13), 7203–7210. https://doi.org/10.1021/jf200740c
- Koraneeyakijkulchai, I., Phumsuay, R., Thiyajai, P., Tuntipopipat, S., & Muangnoi, C. (2023). Anti-inflammatory activity and mechanism of sweet corn extract on il-1β-induced inflammation in a human retinal pigment epithelial cell line (ARPE-19). International Journal of Molecular Sciences, 24(3), 2462. https://doi.org/10.3390/ijms24032462
- Lakshminarayana, R., Sathish, U. V., Dharmesh, S. M., & Baskaran, V. (2010). Antioxidant and cytotoxic effect of oxidized lutein in human cervical carcinoma cells (HeLa). Food and Chemical Toxicology, 48(7), 1811–1816. https://doi.org/10.1016/j.fct.2010.04.011
- Lehmann, M., Vamvaka, E., Torrado, A., Jahns, P., Dann, M., Rosenhammer, L., Aziba, A., Leister, D., & Rühle, T. (2021). Introduction of the carotenoid biosynthesis α-branch into Synechocystis sp. PCC 6803 for lutein production. Frontiers in Plant Science, 12, 699424. https://doi.org/10.3389/fpls.2021.699424
- Li, J., & Abdel‐Aal, E. S. M. (2021). Dietary lutein and cognitive function in adults: A meta‐analysis of randomized controlled trials. Molecules, 26(19), 5794. https://doi.org/10.3390/molecules26195794
- Li, Y., Zhang, Y., Liu, X., Wang, M., Wang, P., Yang, J., & Zhang, S. (2018). Lutein inhibits proliferation, invasion and migration of hypoxic breast cancer cells via downregulation of HES1. International Journal of Oncology, 52(6), 2119–2129. https://doi.org/10.3892/ijo.2018.4332
- Li, Y., Zhao, Y., Zhang, H., Ding, Z., & Han, J. (2024). The application of natural carotenoids in multiple fields and their encapsulation technology: A review. Molecules, 29(5), 967. https://doi.org/10.3390/MOLECULES29050967
- Liang, Z., Zhang, P., Xiong, Y., Johnson, S. K., & Fang, Z. (2023). Phenolic and carotenoid characterization of the ethanol extract of an Australian native plant haemodorum spicatum. Food Chemistry, 399, 133969. https://doi.org/10.1016/j.foodchem.2022.133969
- Liu, Z.-F., Wu, F.-X., Wang, L.-P., Wang, M.-C., & Fu, L. (2016). Lutein suppresses cell proliferation in human colon cancer cell line HT29 via Nrf-2/ARE signal transduction pathway. World Chinese Journal of Digestology, 24(6), 858–865. https://doi.org/10.11569/wcjd.v24.i6.858
- Loughman, J., Loskutova, E., Butler, J. S., Siah, W. F., & O’Brien, C. (2021). Macular pigment response to lutein, zeaxanthin, and meso-zeaxanthin supplementation in open-angle glaucoma: A randomized controlled trial. Ophthalmology Science, 1(3), 100039. https://doi.org/10.1016/j.xops.2021.100039
- Luan, R.-L., Wang, P.-C., Yan, M.-X., & Chen, J. (2021). Effect of lutein and doxorubicin combinatorial therapy on S180 cell proliferation and tumor growth. European Review for Medical and Pharmacological Sciences, 22(5), 1514–1520. https://doi.org/10.26355/eurrev_201803_14501
- Luo, S., Zhang, Y., Song, J., Li, Y., Wu, C., & Zhang, C. (2024). Solubility-permeability interplay of a supersaturated lutein delivery system constructed by glycosylated stevioside and hydroxypropyl-methylcellulose. International Journal of Biological Macromolecules, 258, 128791. https://doi.org/10.1016/j.ijbiomac.2023.128791
- Machida, N., Kosehira, M., & Kitaichi, N. (2020). Clinical effects of dietary supplementation of lutein with high bio-accessibility on macular pigment optical density and contrast sensitivity: A randomized double-blind placebo-controlled parallel-group comparison trial. Nutrients, 12(10), 1–12. https://doi.org/10.3390/nu12102966
- Maheshwari, N., Arya, R. K., Verros, G. D., Dhamole, P. B., & Kannan, A. (2023). Surfactant-enhanced extraction of lutein from marigold petals using an aqueous two-phase system. Separations, 10(2), 133. https://doi.org/10.3390/separations10020133
- Maiani, G., Periago Castón, M. J., Catasta, G., Toti, E., Cambrodón, I. G., Bysted, A., Granado-Lorencio, F., Olmedilla-Alonso, B., Knuthsen, P., Valoti, M., Böhm, V., Mayer-Miebach, E., Behsnilian, D., & Schlemmer, U. (2009). Carotenoids: Actual knowledge on food sources, intakes, stability and bioavailability and their protective role in humans. Molecular Nutrition & Food Research, 53(S2), S194–S218. https://doi.org/10.1002/mnfr.200800053
- Marlina, I., Saleha, M., Fathurrahmi, S., Maulina, F. P., & Idroes, R. (2020). Polyurethane film prepared from ball-milled algal polyol particle and activated carbon filler for NH3–N removal. Heliyon, 6(8), e04590. https://doi.org/10.1016/j.heliyon.2020.e04590
- Martínez-Delgado, A. A., Khandual, S., & Villanueva–Rodríguez, S. J. (2017). Chemical stability of astaxanthin integrated into a food matrix: Effects of food processing and methods for preservation. Food Chemistry, 225, 23–30. https://doi.org/10.1016/j.foodchem.2016.11.092
- Maswanna, T., & Maneeruttanarungroj, C. (2022). Identification of major carotenoids from green alga Tetraspora sp. CU2551: Partial purification and characterization of lutein, canthaxanthin, neochrome, and β-carotene. World Journal of Microbiology and Biotechnology, 38(8), 129. https://doi.org/10.1007/s11274-022-03320-6
- Mohammadnezhad, P., Valdés, A., & Álvarez-Rivera, G. (2023). Bioactivity of food by-products: An updated insight. In Current opinion in food science (Vol. 52, p. 101065). Elsevier. https://doi.org/10.1016/j.cofs.2023.101065
- Mordi, R. C., Ademosun, O. T., Ajanaku, C. O., Olanrewaju, I. O., & Walton, J. C. (2020). Free radical mediated oxidative degradation of carotenes and xanthophylls. Molecules, 25(5), 1038. Multidisciplinary Digital Publishing Institute. https://doi.org/10.3390/molecules25051038
- Nouchi, R., Suiko, T., Kimura, E., Takenaka, H., Murakoshi, M., Uchiyama, A., Aono, M., & Kawashima, R. (2020). Effects of lutein and astaxanthin intake on the improvement of cognitive functions among healthy adults: A systematic review of randomized controlled trials. Nutrients, 12(3), 617. Multidisciplinary Digital Publishing Institute. https://doi.org/10.3390/nu12030617
- Ochoa Becerra, M., Mojica Contreras, L., Hsieh Lo, M., Mateos Díaz, J., & Castillo Herrera, G. (2020). Lutein as a functional food ingredient: Stability and bioavailability. Journal of Functional Foods, 66, 103771. https://doi.org/10.1016/j.jff.2019.103771
- Ola, M. S., Nawaz, M., & Ahsan, H. (2011). Role of Bcl-2 family proteins and caspases in the regulation of apoptosis. Molecular and Cellular Biochemistry, 351(1), 41–58. https://doi.org/10.1007/s11010-010-0709-x
- Omar, W. M., Ahmed, A. E., Raslan, M., El-Nesr, K., Ali, M. M., De Abdelmaksoud, M., & El Dahshan, D. (2021). Effect of lutein-rich extract on human cancer cells. Middle East Journal of Cancer, 12(1), 147–150.
- Ova, O. D., Ata, A., & Ovez, B. (2022). Identification of photosynthetic pigments extracted from phaeodactylum tricornutum as high-value bioactive compounds. Research Journal of Biotechnology, 17(12), 91–99. https://doi.org/10.25303/1712rjbt91099
- Pateiro, M., Gómez, B., Munekata, P. E. S., Barba, F. J., Putnik, P., Kovačević, D. B., & Lorenzo, J. M. (2021). Nanoencapsulation of promising bioactive compounds to improve their absorption, stability, functionality and the appearance of the final food products. Molecules, 26(6), 1547. Multidisciplinary Digital Publishing Institute. https://doi.org/10.3390/molecules26061547
- Patel, A. K., Vadrale, A. P., Tseng, Y.-S., Chen, C.-W., Dong, C.-D., & Singhania, R. R. (2022). Bioprospecting of marine microalgae from Kaohsiung Seacoast for lutein and lipid production. Bioresource Technology, 351, 126928. https://doi.org/10.1016/j.biortech.2022.126928
- Pietro, D., Tomo, D. D., & Pandolfi, P. (2016). Carotenoids in cardiovascular disease prevention. JSM Atheroscler, 1(1). https://doi.org/10.13140/RG.2.1.2635.9921
- Rafi, M. M., Kanakasabai, S., Gokarn, S. V., Krueger, E. G., & Bright, J. J. (2015). Dietary lutein modulates growth and survival genes in prostate cancer cells. Journal of Medicinal Food, 18(2), 173–181. https://doi.org/10.1089/jmf.2014.0003
- Ravichandran, R. (2010). Nanotechnology applications in food and food processing: Innovative green approaches, opportunities and uncertainties for global market. International Journal of Green Nanotechnology: Physics and Chemistry, 1(2), P72–P96. Taylor & Francis Group. https://doi.org/10.1080/19430871003684440
- Roberts, J. E., & Dennison, J. (2015). The photobiology of lutein and zeaxanthin in the eye. Journal of Ophthalmology, 2015, 1–8. https://doi.org/10.1155/2015/687173
- Ruiling, D. (2019). Lutein regulates proliferation and apoptosis of gastric cancer stem cells through PI3K/Akt signaling pathway. Chinese Journal of Tissue Engineering Research, 23(29), 4593–4598. https://doi.org/10.3969/j.issn.2095-4344.1810
- Ruiz-Domínguez, M. C., Medina, E., Salinas, F., Bugueño, W., Fuentes, J.-L., Vílchez, C., Garbayo, I., & Cerezal-Mezquita, P. (2022). Methodological optimization of supercritical fluid extraction of valuable bioactive compounds from the acidophilic microalga Coccomyxa onubensis. Antioxidants, 11(7), 1248. https://doi.org/10.3390/antiox11071248
- Savvidou, M. G., Tsiaka, T., Zoumpoulakis, P., Maggiorou, E., Tyrovolas, K., Molino, A., Hristoforou, E., & Ferraro, A. (2022). Separation and concentration of astaxanthin and lutein from microalgae liquid extracts using magnetic nanoparticles. Magnetochemistry, 8(8), 80. https://doi.org/10.3390/magnetochemistry8080080
- Sawasdee, N., Jantakee, K., Wathikthinnakon, M., Panwong, S., Pekkoh, J., Duangjan, K., Yenchitsomanus, P., & Panya, A. (2023). Microalga Chlorella sp. extract induced apoptotic cell death of cholangiocarcinoma via AKT/mTOR signaling pathway. Biomedicine & Pharmacotherapy, 160, 114306. https://doi.org/10.1016/j.biopha.2023.114306
- Schnebelen-Berthier, C., Acar, N., Simon, E., Thabuis, C., Bourdillon, A., Mathiaud, A., Dauchet, L., Delcourt, C., Benlian, P., Crochet, M., Defoort, S., Tailleux, A., Staels, B., Bretillon, L., & Lecerf, J. M. (2021). The algovue clinical trial: Effects of the daily consumption of eggs enriched with lutein and docosahexaenoic acid on plasma composition and macular pigment optical density. Nutrients, 13(10), 3347. https://doi.org/10.3390/nu13103347
- Shaikh, A. A., Ray, A., & Singhal, R. S. (2023). Co-extraction of marigold flowers (Tagetes erecta L.) and dried coconut (Cocos nucifera L.) shreds using supercritical carbon dioxide: Characterization and functional food formulations. Food Chemistry Advances, 2, 100189. https://doi.org/10.1016/j.focha.2023.100189
- Tahir, A., Shabir Ahmad, R., Imran, M., Ahmad, M. H., Kamran Khan, M., Muhammad, N., Nisa, M. U., Tahir Nadeem, M., Yasmin, A., Tahir, H. S., Zulifqar, A., & Javed, M. (2021). Recent approaches for utilization of food components as nano-encapsulation: A review. International Journal of Food Properties, 24(1), 1074–1096. Taylor & Francis. https://doi.org/10.1080/10942912.2021.1953067
- Tedros Adhanom Ghebreyesus. (2019). World report on vision. World Health Organisation, 214(14). https://www.who.int/publications/i/item/9789241516570
- Toragall, V., Srirangam, P., Jayapala, N., & Baskaran, V. (2021). Lutein encapsulated oleic - linoleic acid nanoemulsion boosts oral bioavailability of the eye protective carotenoid lutein in rat model. Materials Today Communications, 28, 102522. https://doi.org/10.1016/j.mtcomm.2021.102522
- Vasconcelos, A. G., Valim, M. O., Amorim, A. G. N., Do Amaral, C. P., de Almeida, M. P., Borges, T. K. S., Socodato, R., Portugal, C. C., Brand, G. D., Mattos, J. S. C., Relvas, J., Plácido, A., Eaton, P., Ramos, D. A. R., Kückelhaus, S. A. S., & Leite, J. R. S. A. (2020). Cytotoxic activity of poly-ε-caprolactone lipid-core nanocapsules loaded with lycopene-rich extract from red guava (Psidium guajava L.) on breast cancer cells. Food Research International, 136, 109548. https://doi.org/10.1016/j.foodres.2020.109548
- Vieira, I. R. S., & Conte-Junior, C. A. (2022). Nano-delivery systems for food bioactive compounds in cancer: Prevention, therapy, and clinical applications. Critical Reviews in Food Science and Nutrition, Taylor & Francis. https://doi.org/10.1080/10408398.2022.2106471
- Vlaicu, P. A., Untea, A. E., Varzaru, I., Saracila, M., & Oancea, A. G. (2023). Designing nutrition for health—incorporating dietary by-products into poultry feeds to create functional foods with insights into health benefits, risks, bioactive compounds, food component functionality and safety regulations. Foods, 12(21), 4001. Multidisciplinary Digital Publishing Institute. https://doi.org/10.3390/foods12214001
- Wang, M., Morón-Ortiz, Á., Zhou, J., Benítez-González, A., Mapelli-Brahm, P., Meléndez-Martínez, A. J., & Barba, F. J. (2023). Effects of pressurized liquid extraction with dimethyl sulfoxide on the recovery of carotenoids and other dietary valuable compounds from the microalgae spirulina, chlorella and phaeodactylum tricornutum. Food Chemistry, 405, 134885. https://doi.org/10.1016/j.foodchem.2022.134885
- Xavier, A. A. O., Carvajal-Lérida, I., Garrido-Fernández, J., & Pérez-Gálvez, A. (2018). In vitro bioaccessibility of lutein from cupcakes fortified with a water-soluble lutein esters formulation. Journal of Food Composition and Analysis, 68, 60–64. https://doi.org/10.1016/j.jfca.2017.01.015
- Xu, X. L., Hu, D.-N., Iacob, C., Jordan, A., Gandhi, S., Gierhart, D. L., & Rosen, R. (2015). Effects of zeaxanthin on growth and invasion of human uveal melanoma in nude mouse model. Journal of Ophthalmology, 2015, 1–8. https://doi.org/10.1155/2015/392305
- Yamagata, K., Fujiwara, A., Onodera, D., & Motoki, T. (2017). Lutein regulates the expression of apoptosis-related genes and stem cell markers in A549 human lung cancer cells. Natural Product Communications, 12(6), X19345781701200616–X19345781701200616. https://doi.org/10.1177/1934578X1701200616
- Yi, J., He, Q., Peng, G., & Fan, Y. (2022). Improved water solubility, chemical stability, antioxidant and anticancer activity of resveratrol via nanoencapsulation with pea protein nanofibrils. Food Chemistry, 377, 131942. https://doi.org/10.1016/j.foodchem.2021.131942
- Zaher, S., Soliman, M. E., Elsabahy, M., & Hathout, R. M. (2022). Protein nanoparticles as natural drugs carriers for cancer therapy. Advances in Traditional Medicine, 1–30. Springer. https://doi.org/10.1007/s13596-022-00668-w
- Zhang, G., Zhang, M., Pei, Y., Qian, K., Xie, J., Huang, Q., Liu, S., Xue, N., Zu, Y., & Wang, H. (2023). Enhancing stability of liposomes using high molecular weight chitosan to promote antioxidative stress effects and lipid-lowering activity of encapsulated lutein in vivo and in vitro. International Journal of Biological Macromolecules, 253, 126564. https://doi.org/10.1016/j.ijbiomac.2023.126564
- Zhang, S., Lu, Y., He, X., Su, Y., Hu, F., Wei, X., Pan, M., Zhou, Q., & Yang, W. (2022). Lutein inhibits tumor progression through the ATR/Chk1/p53 signaling pathway in non-small cell lung cancer. Phytotherapy Research, 37(4), 1260–1273. https://doi.org/10.1002/ptr.7682
- Zhang, Y., Chang, J., Jiang, W., Ye, X., & Zhang, S. (2021). Long non‑coding RNA CASC9/microRNA‑590‑3p axis participates in lutein‑mediated suppression of breast cancer cell proliferation. Oncology Letters, 22(1), 1–8. https://doi.org/10.3892/ol.2021.12805