ABSTRACT
Background:
Adverse childhood experiences (ACEs) are often associated with stress and anxiety-related disorders in adulthood, and learning and memory deficits have been suggested as a potential link between ACEs and psychopathology.
Objective:
In this preregistered study, the impact of social threat learning on the processing, encoding, and recognition of unknown faces as well as their contextual settings was measured by recognition performance and event-related brain potentials.
Method:
Sixty-four individuals with ACEs encoded neutral faces within threatening or safe context conditions. During recognition, participants had to decide whether a face was new or had been previously presented in what context (item-source memory), looking at old and new faces. For visual working memory, participants had to detect changes in low and high load conditions during contextual threat or safety.
Results:
Results showed a successful induction of threat expectation in persons with ACEs. In terms of face and source recognition, overall recognition of safe and new faces was better compared to threatening face-compounds, with more socially anxious individuals having an advantage in remembering threatening faces. For working memory, an effect of task load was found on performance, irrespective of threat or safety context. Regarding electrocortical activity, an old/new recognition effect and threat-selective processing of face–context information was observed during both encoding and recognition. Moreover, neural activity associated with change detection was found for faces in a threatening context, but only at high task load, suggesting reduced capacity for faces in potentially harmful situations when cognitive resources are limited.
Conclusion:
While individuals with ACE showed intact social threat and safety learning overall, threat-selective face processing was observed for item/source memory, and a threatening context required more processing resources for visual working memory. Further research is needed to investigate the psychophysiological processes involved in functional and dysfunctional memory systems and their importance as vulnerability factors for stress-related disorders.
HIGHLIGHTS
In this study, we show that while overall memory performance was not impaired in highly anxious participants with adverse childhood experiences, socially learned contextual threat modulated both the encoding, processing and recognition of unknown person identities.
Antecedentes: Las experiencias adversas en la infancia (ACEs, por su sigla en inglés) estan a menudo asociadas con trastornos relacionados con el estrés y la ansiedad en la edad adulta, y los déficits de aprendizaje y memoria han sido sugeridos como un vínculo potencial entre las ACEs y la psicopatología.
Objetivo: En este estudio previamente registrado, el impacto del aprendizaje de amenazas sociales en el procesamiento, la codificación y el reconocimiento de rostros desconocidos, así como sus entornos contextuales, se midió mediante el rendimiento y los potenciales cerebrales relacionados con los eventos.
Método: Sesenta y cuatro personas con ACEs codificaron rostros neutrales dentro de condiciones de contextos amenazantes o seguros. Durante el reconocimiento, los participantes tenían que decidir si una cara era nueva o se había presentado previamente en qué contexto (elemento-fuente de memoria), mirando caras antiguas y nuevas. Para la memoria de trabajo visual, los participantes tenían que detectar cambios en las condiciones de carga baja y alta durante la amenaza contextual o la seguridad.
Resultados: Los resultados mostraron una inducción exitosa de la expectativa de amenaza en personas con ACEs. En términos de reconocimiento de rostros y fuentes, el reconocimiento general de rostros seguros y nuevos fue mejor en comparación con los compuestos de rostros amenazantes, y las personas más ansiosas socialmente tenían una ventaja para recordar rostros amenazantes. Para la memoria de trabajo, se encontró un efecto de la carga de tareas en el rendimiento, independientemente de la amenaza o el contexto de seguridad. Con respecto a la actividad electrocortical, se observó un efecto de reconocimiento antiguo/nuevo y un procesamiento selectivo de amenazas de la información del contexto facial durante la codificación y el reconocimiento. Además, se encontró actividad neuronal asociada con la detección de cambios para rostros en un contexto amenazante, pero solo con una gran carga de tareas, lo que sugiere una capacidad reducida para rostros en situaciones potencialmente dañinas cuando los recursos cognitivos son limitados.
Conclusión: Si bien las personas con ACE mostraron un aprendizaje intacto de amenazas sociales y seguridad en general, se observó un procesamiento facial selectivo de amenazas para la memoria de elementos/fuentes, y un contexto amenazante requería más recursos de procesamiento para la memoria de trabajo visual. Se necesita más investigación para investigar los procesos psicofisiológicos involucrados en los sistemas de memoria funcional y disfuncional y su importancia como factores de vulnerabilidad para los trastornos relacionados con el estrés.
背景:童年不良经历(ACEs)通常与成年期的应激和焦虑相关疾病有关,学习和记忆缺陷被认为是 ACEs 和精神病之间的潜在联系。
目的:在这项预注册研究中,社交威胁学习对未知面孔的加工、编码和识别及其背景环境的影响是通过识别表现和事件相关的脑电位测量。
方法:64 名具有 ACE 的人在威胁或安全的环境条件下对中性面孔进行编码。在识别过程中,参与者必须通过查看新老面孔来决定一张面孔是新面孔还是之前在什么环境中呈现过的面孔(项目来源记忆)。对于视觉工作记忆,参与者必须在背景威胁或安全期间发现高低负荷条件的变化。
结果:结果显示,成功诱导了具有ACE 者的威胁预期。在面孔和来源识别方面,对安全面孔和新面孔的整体识别优于威胁面孔混合,社交焦虑的个体更善于记住威胁面孔。对于工作记忆,无论是威胁还是安全背景,都发现了任务负荷对性能的影响。关于皮层脑电活动,在编码和识别过程中都观察到了旧/新识别效果和面部背景信息的威胁选择性加工。此外,在威胁环境中发现与改变检测相关的神经活动,但仅在高任务负荷下,这表明在认知资源有限的可能有害情况下时,面孔能力降低。
结论:虽然具有 ACE 的个体总体上表现出完整的社交威胁和安全学习,在项目/源记忆中观察到威胁选择性面部加工,以及威胁背景需要更多的视觉工作记忆加工资源。需要进一步的研究来考查功能性和功能失调的记忆系统所涉及的心理生理过程及其作为应激相关疾病易感因素的重要性。
1. Introduction
Adverse Childhood Experiences (ACEs) such as sexual, physical, emotional abuse or neglect in childhood or adolescence are a lifelong burden for those affected, as well as for family members and society in general. Individual consequences often include a severely reduced quality of life, which manifests itself in a wide range of health, social, financial, and occupational problems (e.g. reduced work performance, sick leave). In addition, mental health impairments, including risk for mental disorders and comorbidities, impose enormous socioeconomic costs (Dube et al., Citation2003). Impaired cognitive functions such as attention, perception, and memory, which focus on arousing and threatening information, are considered vulnerability factors and precursors for the development and persistence of psychopathology (Bar-Haim et al., Citation2007). The present study examined the impact of emotionally arousing environmental information on the processing and recognition of unfamiliar faces in individuals with ACEs.
1.1. The impact of anxiety and arousal on memory processes
Anxious arousal has long been thought to modulate memory processes, and both facilitative and detrimental effects have been observed. In this regard, the fit of arousing conditions, memory system (e.g. short-term vs. long-term memory) and task characteristics (e.g. load) appear to critically modulate memory performance (Moran, Citation2016; Robinson et al., Citation2013). On the one hand, arousal can direct attention to salient stimuli and strengthen their mental representation (Mather & Sutherland, Citation2011). For instance, a memory-enhancing effect has been found for neutral and emotionally arousing items under arousing conditions, with weakened recall of the associative context in short-term memory tasks (Kensinger, Citation2009; Ventura-Bort et al., Citation2016). On the other hand, arousal consumes memory resources and therefore reduces the ability to actively suppress distracting information, resulting in a detrimental effect of arousal when greater recruitment of cognitive resources is required to maintain task performance (Eysenck et al., Citation2007; Moran, Citation2016). For instance, social anxiety as an arousal factor was associated with regular capacity of visual working memory only in the absence of task-irrelevant distractors and only in low-demand tasks (Moriya & Sugiura, Citation2012). Moreover, focusing on short-term and source memory in healthy participants, we found that contextual threat facilitated perceptual processing for both the central (neutral) item and peripheral (arousing) context information, but it did not affect recognition performance for neither (Schellhaas et al., Citation2020). Thus, the interplay between arousing conditions arising from a task and inter-individual differences, e.g. in trait anxiety and adverse childhood experiences, is poorly understood, and further studies are needed to examine different memory systems.
1.2. The role of social threat and safety learning in face perception and recognition
Anxious arousal can be triggered not only by one's own experiences but is also mediated by social cues in the environment. Specifically, humans learn vicariously by observing aversive experiences of others and through verbal communication (Haaker et al., Citation2017; Robinson et al., Citation2013). Although such social learning is considered extremely relevant in the development and maintenance of stress-related psychopathology (DSM-5; American Psychiatric Association [APA], Citation2013), only few studies have experimentally addressed this notion. Here, we examined social learning mechanisms to induce anxious arousal, focusing on person perception and recognition. Recognition of unfamiliar faces is generally poor (Burton & Jenkins, Citation2011), but arousing contextual features affect both perception and neural processing of these faces and the likelihood of recognizing them or the contextual settings.
Inherently emotional faces, such as those depicting loved familiar people or emotional expressions enhance perceptual, attentional and memory processes compared to viewing neutral unknown faces. A broad neural network has been associated to the perception and recognition of negative facial information (e.g. amygdala, hippocampus, extrastriate cortex, frontal and parietal cortices; Fusar-Poli et al., Citation2009; Keightley et al., Citation2011; Vuilleumier et al., Citation2001). Moreover, emotional faces trigger increased electrocortical processing already early in the visual processing stream. This is especially observed for threatening faces, whose processing is facilitated compared to non-threatening or neutral faces, as indicated by a pronounced N170 component (between 150-190ms after image onset) and early posterior negativity (EPN, 200–300 ms; Schupp et al., Citation2004; for a review see Schindler & Bublatzky, Citation2020). At later processing stages, facial threat signals have been shown to elicit increased P3 amplitudes and late positive potentials (LPP), indicating elaborate stimulus processing and memory updating (Bublatzky et al., Citation2020; Schupp et al., Citation2004). Interestingly, the modulatory effect of emotional arousal on face perception and recognition varied with interindividual differences. For example, individuals with depression showed enhanced P300 amplitudes for social threat information (Iffland et al., Citation2021), and veterans with posttraumatic stress disorder had reduced LPPs to angry faces in association with less accurate emotion identification (MacNamara et al., Citation2013).
Such neural threat-selective processing patterns presumably set the stage for behavioural response priming such as faster and more accurate detection rates for emotionally relevant information (Bublatzky et al., Citation2018; Kavcıoğlu et al., Citation2021; Öhman et al., Citation2001). Less is known, however, about a possible spill-over effect from a threatening (i.e. arousing) context to an otherwise neutral face. Is an unknown face with a neutral expression better remembered when previously encountered in threatening circumstances? For instance, preferential responding to neutral faces in a threatening context has been found for both familiar (even beloved) and unfamiliar faces, with a robust threat effect even over the course of several test days (Bublatzky et al., Citation2022). Here the question arises whether this preferential processing also translates to enhanced memory and recognition of (un)familiar faces.
1.3. Effects of adverse childhood experiences on neural processing
Understanding the deleterious effects of stress has been the subject of much neuroscience research. Severe stressful events such as ACEs can lead to prolonged and/or blunted response of the hypothalamus–pituitary–adrenal axis (McEwen, Citation2002), affecting brain morphology involved in learning and memory (e.g. hippocampus, prefrontal cortex; Bremner et al., Citation2003) and providing the basis for poor encoding of stressful situations. Moreover, stress-related neuronal and humoral responses have been linked to changes in information processing. For instance, biased attention and memory for negatively arousing information are associated with cognitive impairments such as distractibility or concentration problems (e.g. negativity bias; Letkiewicz et al., Citation2020).
Several components of the event-related brain potentials (ERP) have been identified as sensitive to early stress, trauma experiences, and post-traumatic stress disorder (e.g. N2, P200, P300; Karl et al., Citation2006). For instance, individuals with a history of abuse show reduced N2 and P300 for emotional stimuli, which presumably reflect problems in disengagement from emotional information (Letkiewicz et al., Citation2020). Importantly, combined attentional and learning deficits could serve as a link between adverse childhood experiences and severity of psychopathology. For instance, decreased discrimination between arousing and non-arousing cues may contribute to (over-)generalization of threat and related avoidance behaviours (Stegmann et al., Citation2020). Moreover, threat-selective processing patterns for several emotional valence categories have been observed in individuals with posttraumatic stress disorder (PTSD), which are similar to the processing of negative stimuli in healthy participants (e.g. enhanced P300 and late positivities; Saar-Ashkenazy et al., Citation2015). The temporal dynamics of cognitive processes in individuals with ACE are therefore likely to reveal changes in the perception, recognition, and memory of threatening situations.
In the context of adverse childhood experiences, abuse has been found to be associated with an increased bias towards negatively valenced emotional arousal, with an enhanced recognition and attention allocation towards threatening emotion expressions, even with very little information present (e.g. angry face; Assed et al., Citation2020; Pollak et al., Citation2000; Pollak & Sinha, Citation2002). This was evident in greater amygdala (re)activity for angry, fearful and sad faces and increased vmPFC activity for sad faces (Dannlowski et al., Citation2013; Doretto & Scivoletto, Citation2018; Tottenham et al., Citation2011; van Harmelen et al., Citation2013). Neglect, on the other hand, appears to result in a reduced ability to differentiate between emotional categories, probably due to a deprived environment (Pollak et al., Citation2000). Maltreatment was associated with an increased N170 amplitude for angry, fearful and happy faces, compared to a non-maltreated control group (Fang et al., Citation2019). Furthermore, children with a history of maltreatment show impaired attentional disengagement from angry facial stimuli apparent (Pollak & Tolley-Schell, Citation2003), especially apparent in early visual processing and structural face encoding (P100; N170; Curtis & Cicchetti, Citation2011; Fang et al., Citation2019) and early information-processing biases (Dodge et al., Citation1995; Weiss et al., Citation1992). However, whether these emotional processing biases continue into adulthood is less well understood. In terms of contextual threat factors, severely traumatized individuals show poorer recognition performance of face and contextual information, e.g. the background in which a stimulus is presented (Brewin, Citation2011; Kensinger, Citation2007; MacNamara et al., Citation2013). Explicit memory for context information (i.e. source memory), however, has rarely been explicitly studied in traumatized populations (see also Brewin et al., Citation2012; Tapia et al., Citation2012).
1.4. Study objective and hypotheses
This preregistered study (https://osf.io/gzpev) examined the influence of socially learned threat and safety on face memory, context memory, and visual working memory in participants with ACE as a potential mediator of psychopathology. To this end, an item/source memory and a change detection task were performed with unknown faces as task stimuli (Sessa et al., Citation2011; Weymar et al., Citation2013). Importantly, both tasks were completed within contextual settings serving as signal for shock threat or safety, and threat/safety associations were learned vicariously through observing others or by means of verbal instructions. Unless otherwise stated, hypotheses were preregistered.
1.4.1. Replication of threat-selective processing and aversive expectancy effects
Based on previous research on observational and instructional learning (Bublatzky et al., Citation2020; Olsson & Phelps, Citation2007), we predicted pronounced valence, arousal, and threat ratings for the threat relative to the safety context. Similarly, threat-selective electrocortical processing was predicted for early and late parieto-occipital and fronto-central negativity (i.e. enhanced N170, EPN, and LPP amplitudes relative to instructed/observed safety conditions; Bublatzky et al., Citation2010; Schellhaas et al., Citation2020). As an index of functional processes, this threat-selective processing was predicted regardless of anxiety levels or early maltreatment experiences, and no differences were expected between instructional and observational learning for any of the measures (Olsson & Phelps, Citation2007).
1.4.2. Memory performance with regard to threat effects
Regarding item/source memory, we predicted an overall enhanced recognition effect for faces from a threat context (i.e. independent of sample characteristics) with an either enhanced or possibly reduced recognition ability for the context itself (Bisby et al., Citation2018; Ventura-Bort et al., Citation2016). Visual working memory performance depends on the availability of cognitive resources and should be better if only one target stimulus is presented in comparison to two (i.e. different load conditions). Regarding the impact of threat, previous findings are mixed showing either reduced ability to actively inhibit the threatening information taking away attentional resources (Moran, Citation2016), null effects (Ward et al., Citation2020), or even better recruitment of cognitive resources (Moriya & Sugiura, Citation2012).
1.4.3. Early and late attentional and memory effects evident in electrocortical processing
Regarding electrocortical processing, we predicted differential processing of faces during threat relative to safe context conditions during the encoding session of the item-source memory task (i.e. enhanced N170, EPN, and LPP to threat-faces-compounds; Bublatzky et al., Citation2020; Schellhaas et al., Citation2020). For face recognition, we expected an old/new recognition ERP effect with enhanced positivity for previously presented faces in earlier (about 300-500ms parietal-occipital) and, depending on correctly assigned threat context, in a late time window (about 600-800ms fronto-central; Rugg & Curran, Citation2007; Schellhaas et al., Citation2020; Weymar et al., Citation2013), assumed to reflect familiarity and recollection processes, respectively. For visual working memory capacity, indexed by the contralateral delay activity (CDA), an increased amplitude was expected when the number of objects maintained in WM increases (Ikkai et al., Citation2010) and while a threat context as task irrelevant disrupting information is present (Ward et al., Citation2020). In addition, the N170, a component that is reliably elicited by facial stimuli (Schindler & Bublatzky, Citation2020), was exploratively analyzed (i.e. not preregistered) as an index of cognitive load and face perception in the working memory task (Morgan et al., Citation2008).
1.4.4. Impact of adverse childhood experiences and social anxiety
While the above hypotheses were predicted independent of sample characteristics, additional effects were expected regarding the impact of ACE and exploratory tested for social anxiety scores. We hypothesized that higher levels of traumatization were associated with increased neural processing of the threat but also the safe condition (especially at moderate-severe levels; Karl et al., Citation2006). It was therefore predicted that early differential processing of threat and safety conditions would decrease, as would the overall strength of processing (N170; P100; Galletly et al., Citation2001). With regard to later contextual (i.e. threat-related) ERP effects, it was assumed that threat reduces working memory capacity in highly traumatized individuals, resulting in an increased CDA during threat compared to safety. For recognition effects, threat was predicted to increase attention towards neutral faces in highly traumatized individuals, resulting in enhanced, expectancy-driven electrocortical processing of threat during recognition (LPP; Sandre et al., Citation2018). Regarding behavioural measures, we expected that perceived threat affects cognitive processing and memory and tends to inhibit adequate performance (Pechtel & Pizzagalli, Citation2011). Therefore, retrieval of face–context compounds under threat, as well as retention of these associations in working memory (Goodman et al., Citation2019; especially under high load), was expected to be worse with increasing ACE levels. Although a dimensional approach was pursued, exploratory group comparisons were conducted between low, medium, and high levels of anxiety and ACE, and their subgroups. This was done because some effects of early trauma on cognition may depend on type and severity (Herzog & Schmahl, Citation2018).
2. Methods
2.1. Participants
Sixty-four participants (59 females) between the age of 19–60 years (M = 32.45, SD = 11.05) were recruited from all over Germany. As an inclusion criterion, all participants had experienced adverse childhood experiences (from the age 0–18, abuse and neglect) as determined by the German version of the Childhood Trauma Questionnaire (CTQ; German Version, Bernstein et al., Citation1998). In a pre-screening, one item of every subscale (sexual abuse, physical neglect and abuse, emotional neglect and abuse) was presented with an overall value of ≥ 1 as cut-off criteria for participation. Sample size was determined by power analysis based on previous findings, detecting medium effects (Sessa et al., Citation2011) and statistical power of 1-β ≥ .80 (g*power, Erdfelder et al., Citation1996).
Exclusion criteria were acute and/or chronic physical diseases (e.g. cardiovascular, respiratory, or neurological diseases), psychotic disorders, use of psychotropic drugs (except selective serotonin and norepinephrine reuptake inhibitors [SSRIs and SNRIs]), and current (past 12 months) substance dependence and/or abuse. Inclusion/exclusion criteria were verified by means of an interview prior to participation which also served as diagnostics acquisition (Structured Clinical Interview for DSM-IV (APA, Citation2000), SCID-I (36 participants) or DSM-5 (APA, Citation2013), SCID-5 (28 participants)), done by PhD students trained in conducting psychological diagnostics (see supplements). For the diagnostics, data of five participants is missing due to technical errors in data collection and storing.
Participants were recruited via advertisements placed on various homepages (Central Institute of Mental Health Mannheim [CIMH]; Research Training Group GRK 2350), in local newspapers, and flyers at the CIMH and psychotherapist offices. Ethics approval was given by the local ethics committee (Ethik-Kommission II der Universität Heidelberg, Medizinische Fakultät Mannheim, 2018-633N-MA) and participants provided written informed consent to the study protocol and received monetary compensation (30€).
2.2. Materials and memory tasks
Participants performed two experimental tasks, a combined item/source memory (ISM) and visual working memory (VWM) task, both using face stimuli ((A)). Neutral face pictures of in total 150 actors (half females; 90 were chosen for the ISM task and 60 for the VWM; see supplements) were selected from the Karolinska Directed Emotional Faces (Lundqvist et al., Citation1998), the Radboud (Langner et al., Citation2010), and the NimStim database (Tottenham et al., Citation2009). Pictures (442 × 606 pixels) were transformed into grayscale, normalized for brightness, cropped with an elliptic mask to remove hair and ears, and a black frame was used to replace the original background (Adobe Photoshop CS2). For both tasks, face pictures were presented with contextual colours as a backdrop (1280 × 1024 pixels), using different colour combinations, either blue and green (RGB values: 0,0,255 and 0,255,0), or red and yellow (RGB values: 255,0,0 and 255,255,0); colour assignment to condition was counterbalanced.
Figure 1. (A) Schematic illustration of the experimental procedure. Background colours served as verbally instructed or observed threat-of-shock or safety cues in both following memory tasks, which were performed in a randomized order. (B) In the item/source memory task, during the encoding phase, 60 pictures of male and female faces displaying neutral expressions were presented for 6 s each (variable ITI) in front of a coloured background (green or blue source) that alternated in blocks of 10 faces, each block started with a coloured frame for 3 s. Participants’ task was to memorize the faces, without a mentioning of the colours. During the recognition phase, the 60 old and 30 new faces were presented intermixed without background colours. Participants’ task was to decide whether the face was shown before and with which colour (combined old/new item and source recognition task), or whether it was not previously presented. (C) For the visual working memory task (change detection), a cue indicated the spatial location to pay attention to. In a following memory array either four or two faces were presented for 200ms. After a 900ms retention interval, participants had to decide whether a face on the arrow indicated side had changed identity or not in the test array.
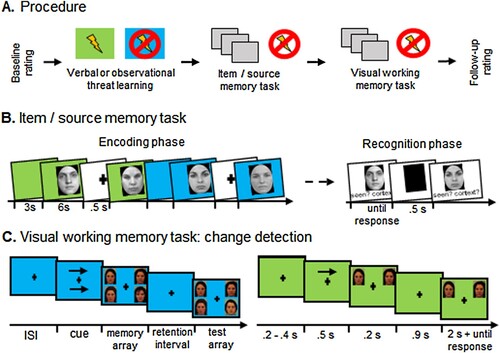
Item/source memory task. Participants were asked to recognize a previously presented face and to recall the specific contextual features of the face (see (B); Schellhaas et al., Citation2020). To this end, a random selection of 60 out of 90 face pictures was chosen, which were surrounded with two different coloured background frames (e.g. blue and green), 30 pictures for each colour. Participants’ task was to look carefully at the faces and memorize them while being instructed to recognize them later (explicit learning instruction for item memory). The additional source identification task was not mentioned (implicit learning of context information).
During an encoding session, the 60 pictures were presented for 6 s each (‘old’ faces), separated by an inter-trial interval (ITI) ranging between 620 and 1150ms, and the frame colours alternated in blocks of 10 pictures (i.e. 3 green or red and 3 blue or yellow blocks). Without delay, the recognition session started, and all 60 old faces (without colour frames) were presented intermixed with 30 additional new pictures in randomized order. Participants’ task was to indicate the contextual colour against which a face had been presented in the encoding phase (i.e. combined item and source memory), or whether it was a new face. Pictures remained on the screen until the participant responded by pressing one of three keyboard buttons. The behavioural options were either a blue (yellow) or a green (red) button (corresponding to the background, classified as old threat and safety faces), or a white button for newly presented pictures (new faces). If participants recognized a picture as being from the encoding phase but did not remember the context, they were instructed to guess the context. There was no time limit for responding and no feedback on accuracy was provided. Each choice was followed by a 1s ITI showing a black rectangle replacing the pictures and the next face was presented ().
Visual working memory task. A change detection task served to examine visual working memory (Sessa et al., Citation2011; Stout et al., Citation2013). Each trial consisted of a memory and a test array displaying 2 or 4 faces simultaneously (see (C)). Before the memory array was presented, two arrows (pointing 200ms to the left or right) indicated the position of the target faces, which were the face(s) to be remembered. The memory array was presented for 500ms and was followed by an empty retention interval (i.e. no faces) screen for 900ms in the same colour as the memory array. Following, the test array was presented and the participants had to decide whether (one of) the target face(s) had changed the identity by pressing one of two buttons (stating ‘the same’ or ‘different’). Regardless of the overall number of faces in the memory array (2 vs. 4) only one facès identity was changed. The change detection task was performed with two different background colours (either blue and green or red and yellow), and this context condition alternated every 10 trials.
Participants’ task was to focus on the arrow-indicated side and memorize only the face(s) in the memory array presented on this side. The number of target faces served to manipulate the task load (low vs. high load), and varied randomly within each context condition. In half of the trials, the faces on the memory and test array were identical, for the other trials one face on the arrow-cued side of the memory array was replaced with a different same-gender face in the test array. There was no response time limit and after the response, a fixation cross was presented (ITI 500ms) indicating the start of the next trial. Participants completed 16 practice trials without contextual colours (two for each combination of high- vs. low-load and changed vs. non-changed target). In the test phase, participants completed 6 runs without breaks in each colour, each with 64 trials (768 trials in total), presented in an evenly changing order and evenly distributed for high/low load and change/no change trials (). For both tasks, the change of the background colour was indicated by the presentation of a picture of the new colour without a face for 4s.
Stimuli were presented on a 22-inch computer screen placed approximately 1m in front of the participants using OpenSesame software (Mathôt et al., Citation2012).
2.3. Procedure
A first set of questionnaires was completed approximately 1–2 days before the testing day (CTQ and SCID). Upon arrival in the lab, the EEG sensor net was attached and questionnaires on social anxiety, and state anxiety were completed (Social Phobia Inventory, SPIN, Stangier & Steffens, Citation2001; State-Anxiety Inventory, STAI-S, Laux et al., Citation1981; ). Following, a baseline rating regarding the experimental background colours (blue/green, or yellow/red, serving later as threat/safety signals) was performed using valence, arousal (Self-Assessment Manikin, SAM; Bradley & Lang, Citation1994, on a scale from 1-9) and perceived threat scales (Likert scale from 0 to 10). To trigger aversive expectations about imminent shocks, a fake stimulation electrode was attached to the inner forearm of the non-dominant arm. Participants were then told that the expected shock intensity would be ‘maximally unpleasant but not yet painful’ and that they would receive a maximum of three electric shocks throughout the entire experiment.
Table 1. Questionnaire data for the total sample and split by social learning factor (observation and instruction).
Participants acquired threat and safety associations socially via either verbal instructions or observations. In the instruction group, participants were verbally instructed that specific background colours will serve as signals for shock threat (e.g. green) or safety from shocks (e.g. blue; Bublatzky et al., Citation2014; Schellhaas et al., Citation2020). The observation group learned threat/safety associations with contextual colours vicariously by viewing a video displaying another (fake) participant as a demonstrator (learning model) undergoing a differential threat conditioning experiment (Haaker et al., Citation2017). These videos consisted of pre-recorded experimental sessions with a female and male demonstrator within the same experimental environment. Videos contained 24 trials each (edited for brightness, trial length, and 4-minutes duration using Adobe Premiere Pro X). Two thirds of the video-threat trials were paired with a shock, indicated by the demonstrator twitching their arm and showing a frowning facial expression. The participants themselves were told that they would not receive shocks during the video; however, they should pay close attention to the demonstrator and the colours as they would receive shocks with the same colour in the next part of the experiment. Participants viewed videos with a same-sex demonstrator; assignment of colours to threat/safety conditions were balanced across participants.
The two memory tasks were completed in randomized order. At the end of the experiment, the videos were rated (using Likert scale forms 0-9) and revealed a medium-high demonstrator-observer agreement regarding naturalness (M = 4.98, SD = 2.91), identification (M = 5.06, SD = 2.95), expressiveness (M = 6.94, SD = 2.34), discomfort (M = 5.78, SD = 2.71), and empathy (M = 5.60, SD = 3.06). As a manipulation check, contextual colours were rated regarding valence, arousal, and perceived threat following both memory tasks. Indicating the successful learning of threat/safety contingencies, all participants were able to indicate the correct threat and safety colour at the end of the experiment. Finally, participants were debriefed.
2.4. Data recording and reduction
Electrocortical activity was recorded using a 65-channel system (BrainProducts, Munich, Germany). Ag/AgCl active electrodes were placed in a cap using a 10–10 electrode placement standard with FCz as reference electrode (Falk Minow Services, Herrsching, Germany). EEG was recorded continuously with a sampling rate of 500 Hz and filtered online from 0.1 to 100 Hz using Vision Recorder acquisition software and BrainAmp DC amplifiers (BrainProducts). Electrode impedance was kept below 20kΩ (manufacturer recommendation). Offline data analyses were done using VisionAnalyzer 2.0 (BrainProducts) and EMEGS (version 2.7; Peyk et al., Citation2011) including conversion to an average reference, 30 Hz low-pass filtering, artifact detection, sensor interpolation, and baseline correction (200 ms). Artifacts were rejected from trials exceeding ±70µV (e.g. eye blinks), on in average 1.29% of the trials for the ISM and 2.37% of the trials for the VWM.
Stimulus-synchronized epochs were extracted and lasted for the ISM task from 200ms before to 1000ms after stimulus onset (encoding and recognition session), and for the VWM task from 200ms before to 1200ms after stimulus onset of the memory array (including retention and test array). For the VWM task, difference waves for the contralateral-delay activity (CDA) were computed by subtracting the average activity recorded by electrodes ipsilateral to the arrow-cued visual field of the memory array from the average activity recorded at symmetrical electrodes contralateral to the arrow-cued visual field of the memory array (i.e. CP1/CP2; CP5/CP6). The CDA is classified as activity during the retention interval (500–900ms after memory array) and thought as reflecting working memory capacity (Sessa et al., Citation2011). Finally, separate average waveforms were calculated for each condition, for each sensor (difference of sensors for CDA) and participant.
One participant was excluded from all EEG analyses due to technical errors during recording (no data was collected). For the EEG analyses of the VWM task, additional four participants were discarded due to poor data quality (more than 30% of trials with artifacts). Due to missing values, two participants were excluded from the arousal ratings and seven from the perceived threat ratings. One and two participants for STAI-S and CTQ respectively with lack of data were excluded from covariation analyses.
2.5. Data analysis
The Greenhouse Geisser procedure was used to correct violations of sphericity and Bonferroni correction for multiple comparisons. As a measure of effect size the partial eta squared (ηp2) is reported. Statistical analyses were conducted using SPSS (version 25), for the MPT analyses the TreeBUGS package (Heck et al., Citation2018) in R studio (version 3.6.2, R Core Team, Citation2021). Significant effects were followed up by a separate two-tailed t-test with significance level set to p < .05. Due to our focus on adverse childhood experiences, social and general anxiety, we also included questionnaire scores of the SPIN, STAI-S and CTQTOT as well as the five subscales of the CTQ (emotional and physical abuse and neglect, sexual abuse) as exploratory covariates.
2.5.1. Self-report data
Separate 2 × 2 × 2 repeated measure ANOVAS were calculated for valence, arousal, and threat ratings. Within-subject factors were Time (baseline vs. follow-up) and Context (threat vs. safety), as well as the type of Learning (observation vs. instruction) as a between-subject factor.
2.5.2. Behavioural data
To quantify memory performance in the item/source memory task, hit rates (HIT, correct responses divided by all responses in target trials) and false alarm rates (FAR, incorrect responses divided by all responses in non-target trials) as well as item recognition (HIT-FAR) were computed. Source-memory for the encoding context of faces was analyzed using the average conditional source identification measure (ACSIM), which is the number of all correct source identifications divided by the overall number of faces that were identified as ‘old’ (Bell & Buchner, Citation2011). For the visual working memory task, HIT and FAR were also calculated and visual working memory was quantified with a standard index of sensitivity by subtracting the inverse-normal transform of the FAR from the inverse-normal transform of the HIT, deriving from signal-detection theory and reflecting change detection ability (d’ = ZHIT-ZFA). For HIT and FAR of zero a constant of .5 was added to the number of hits and false alarms and the number of detection and signal trials was increased by 1, as proposed by Hautus (Citation1995). Additionally, we calculated the number of encoded faces in each condition using Cowan’s k (k = load*(HIT-FA)), serving as an index of VWM capacity.
All item/source memory measures were analyzed by 2 × 2 ANOVAs, with Context (threat vs. safety) as a within-subject and Learning (observation vs. instruction) as a between-subject factor. Visual working memory was analyzed by a 2 × 2 × 2 ANOVA, with the additional within-subject factor of memory Load (low vs. high).
Hierarchical multinomial processing tree (MPT) modelling was used to disentangle item recognition, source recognition, and guessing biases for the item/source memory task. We used the two-high-threshold model of source monitoring (2HTSM; Bayen et al., Citation1996) with three decision trees (i.e. faces from threat or safety sources, or new faces; ), and individual questionnaire scores included in the MPT model (Arnold et al., Citation2015). As a between-factor, Learning (observation vs. instruction) was included, separate parameter estimates were calculated for each of the three decision trees. For details on MPT analyses see supplementary material.
Figure 2. Submodel 5d of the two-high-threshold model of source monitoring. The model parameters represent transition probabilities between latent cognitive states; DT = probability of detecting that an item is old from a threatening source; DS = probability of detecting that an item is old from a safe source; DN = probability of detecting that an item is new; dT = probability of correctly remembering the shock source of an item; dS = probability of correctly remembering the safe source of an item; g = probability of guessing that an item is from the shock source; b = probability of guessing that an item is old. Adapted from Arnold et al. Citation2013; originally by Bayen et al. (Citation1996).
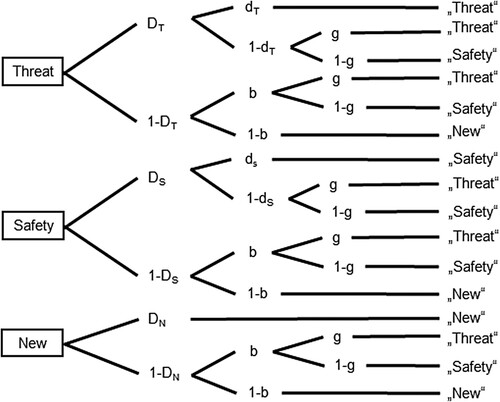
2.5.2.1. ERP data
Differential effects of the instructed/observed threat versus safety conditions on neural face processing and recognition were investigated for several ERP components. To this end, sensors and time-windows were selected based on previous research and visual data inspection (e.g. Bublatzky & Schupp, Citation2012; Schellhaas et al., Citation2020; Ventura-Bort et al., Citation2016; Weymar et al., Citation2013). Regarding the encoding session of the item/source memory task, statistical analyses were computed for an early time window (252–400ms) over parieto-occipital sensor sites (PO7, PO8) and for a late time window (540–800ms) over fronto-central sensors (C1, C2, FC1, FC2). For the recognition session, we used a three-step procedure with increasing informative value (cf. Schellhaas et al., Citation2020). First, for the classical Old/New recognition effect (Rugg & Curran, Citation2007), only trials with correct item recognition were included (regardless of source identification) and old versus new faces were compared in a late time window (660–800ms) over parieto-occipital sensors (PO9, PO10). Second, only trials with correct item and correct source identification were included for advanced item-source Old/New analyses (Schellhaas et al., Citation2020; Wilding & Rugg, Citation1996). Those were analyzed in a late time window (752–900ms) over central sensor sites (Cz, CPz, C1, C2, C3, C4). Third, trials based on correct item and source identification were included separately for old-threat, old-safe, and new faces. Here, a late time window (700–900ms) for parieto-occipital sensors (PO3, PO4, POz) was used.
Similar, for the visual working memory task, the contralateral-delay activity (i.e. difference waves), were computed between 500 and 900 ms after the onset of the memory array at CP5-CP6 and CP1-CP2 sensors, based on previous research and visual inspection (e.g. Sessa et al., Citation2011; Stout et al., Citation2013). For visualization, waveforms were low-pass filtered (10 Hz). Additionally, the N170 component was computed over parieto-occipital sites (PO9, PO10), within a ± 40ms window centered on the maximum peak of the grand-average means (147ms; e.g. Schindler & Bublatzky, Citation2020).
Separate repeated measure ANOVAs were computed based on the mean area scores for the selected sensors that considered the experimental Context (threat vs. safety; for ISM encoding and recognition, as well as VWM difference waves), Recognition (old vs. new; for ISM recognition), task Load (low vs. high; for VWM task), Learning group (observation vs. instruction; as between-subject factor), and Laterality (left vs. right hemisphere).
3. Results
3.1. Threat-related processing and aversive expectancy effects
Both observational and instructional learning groups successfully acquired threat and safety associations. Significant interactions emerged for Context × Time (valence: F(1,62) = 17.29, p < .001, ηp2 = .23 [.08,.35], arousal F(1,60) = 20.50, p < .001, ηp2 = .26 [.11, .39], threat F(1,55) = 14.14, p < .001, ηp2 = .21 [.06, .33]). After learning, the threat context was perceived as more unpleasant, arousing, and threatening compared to safety (valence: F(1,63) = 25.28, p < .001, ηp2 = .29 [.15, .45], arousal: F(1,63) = 37.07, p < .001, ηp2 = .37 [.21, .49], threat: F(1,60) = 32.60, p < .001, ηp2 = .35 [.19, .48]). Interestingly, no differences were found between learning groups (Fs < 1.48, ps > .23), and no interactions Learning × Context emerged (Fs < 1.14, ps > .29). Moreover, ratings did not co-vary with SPIN, STAI-S and CTQTOT. A significant positive correlation emerged between social anxiety and trauma scores (SPIN × CTQTOT, r(60) = .28, p < .05).
Electrocortical data confirmed successful threat induction as part of the encoding session of the item/source memory task (see ; Schellhaas et al., Citation2020). Differential processing of threat compared to safety contexts was observed during the encoding session, Context F(1,61) = 8.33, p < .01, ηp2 = .12 [.02, .27], with more pronounced parieto-occipital positivities (252-400ms) for a threat context over the right hemisphere, F(1,61) = 7.56, p < .01, ηp2 = .11 [.02, .24], indicating threat-enhanced discrimination of context features. This effect occurred simultaneously to and regardless of a high demanding memory task (i.e. unfamiliar face encoding; Schupp et al., Citation2007). The observation and instruction group did not differ, Learning F < 1. Moreover, a later threat-enhanced negativity emerged over fronto-central sites (540-800ms), Context F(1,62) = 3.13, p = .08, ηp2 = .05 [.00, .16], with more pronounced amplitudes over the right hemisphere, F(1,62) = 6.07, p < .05, ηp2 = .09 [.01, .21].
Figure 3. Illustration of the threat main effect in the encoding session of the item/source memory task. (A) Grand averaged ERPs prompted by faces presented within a threat-of-shock or safe context for an exemplary parieto-occipital sensor (PO8) and topographical difference maps (threat – safe) displaying the averaged time interval (230–300 ms) plotted on the back of a model head. (B) Grand averaged ERPs for faces presented within a threat-of-shock or safe context for an exemplary fronto-central sensor (FC1) and a topographical difference map (threat – safe) displaying the averaged time interval (472–632 ms) plotted on a top view of a model head.
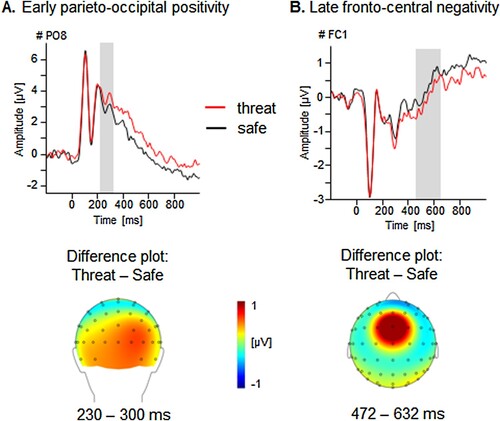
3.2. Memory performance with regard to threat effects
3.2.1. Item and source memory (ISM)
Contrary to our hypotheses, conventional measures of recognition performance revealed no effects of aversive anticipation on item (HIT-FAR; Context F(2, 62) < 1) or source identification [ACSIM; Context F(1,64) = 1.40, p = .24; HITT = .71 (SD = .16), FART = .23 (SD = .15), HITS = .72 (SD = .14), and FARS = .23 (SD = .15)]. As predicted, no differences emerged between observational and instructional learning (Fs < 1.51, ps > .22).
The hierarchical MPT model provides separate estimates for item and source recognition as well as the guessing parameters as a function of contextual settings (see ). While overlapping Bayesian confidence intervals (BCIs) indicate a non-meaningful difference, non-overlapping BCIs were observed between the recognition parameters for faces from the threat context (DT) and safety context/new faces (DS/DN). In other words, faces from a threat context were recognized less well than faces from a safe source or new faces. Regarding source recognition, overlapping and very large BCIs for the threat and safety sources (dT and dS) indicate no difference. Moreover, given a 2:1 ratio of old to new items, there was a slightly conservative tendency towards guessing that a face was new (b = .58). The source guessing parameter (g = .51) reflects the event probability that a face was from a threatening or safe context (1:1 ratio), thus at chance level. Finally, no differences were observed between the learning groups, and no covariation emerged with questionnaires. Model fit was assessed using Klaueŕs test statistic T1. The corresponding p-value was .39, indicating good model fit.
Table 2. Mean parameter estimates of the latent-trait MPT model for the recognition performance of the item/source memory task.
3.2.2. Visual working memory (VWM)
A summary of the VWM performance measures is reported in . No effects of contextual threat/safety or learning type were observed for hit rate, Fs(1,60) < 1, ps > .40. . High task load reduced hit rate indicating better performance for a set size of 2 relative to 4 faces, Load F(1,60) = 166.18, p < .001, ηp2 = .74 [.63, .79], the interaction of Context × Load, F(1,60) < 1, p = .58 was not significant.
Table 3. Behavioural performance measures for the visual working memory task (change detection), derived from signal detection theory.
For the false alarm rate (FAR), aversive anticipation and memory load did not play a role (Fs < 1, ps > .75). However, FAR was overall higher in the instructional learning group compared to the observational learning group, Learning F(1,60) = 5.13, p < 0.05, ηp2 = 0.08 [.01, .20].
For d‘ values (reflecting change detection ability), no main effects of threat/safety context or learning occurred, Context and Learning: Fs(1,60) < 1.35, ps > .25. However, high load reduced the detection of changes, Load F(1,60) = 99.66, p < .001, ηp2 = .63 [.49, .71], irrespective of context conditions, Context × Load F(1,60) < 1, p = .75. As indicated by k, VWM capacity was neither modified by Context, Learning, Load, nor Context × Load, Fs < 1, ps > .40. Interestingly, a significant interaction of Context × Learning emerged, F(1,60) = 5.21, p < .05, ηp2 = .08 [.01, .20], indicating that k was higher for the threatening compared to the safe context but only in the observational learning group.
3.3. Early and late attentional and memory effects evident in electrocortical processing (ERPs)
3.3.1. Item and source memory: old/new and context recognition
In a first step, we quantified the old/new effect using trials with correct face recognition only (i.e. regardless of correct or incorrect source allocation). Replicating our previous study in healthy participants (Schellhaas et al., Citation2020), correctly identified new trials were associated with a more pronounced positivity over parieto-occipital sensor sites (600-800ms), F(2,56) = 4.50, p < .05, ηp2 = .07 [.00, .18]. No effects were observed for Learning or Laterality, Fs(2,56) < 2.75, ps > .10. Thus, recognizing new faces was facilitated by enhanced neural processing, when correct context recognition was not considered.
In a second step, trials were selected based on correct item recognition and source allocation (see ). As in previous research and contrary to the above-reported results, old compared to new faces were associated with an enhanced positivity over central sensor sites (old/new ERP effect, 752–900 ms), F(2,56) = 3.60, p = .06, ηp2 = .06 [.00, .18], reflecting in parts our behavioural findings. No effects of Learning or Laterality occurred, Fs < 2.30, ps > .11. Thus, increased familiarity with the neutral faces (i.e. correct face and context identification) was mirrored in neural processing at later processing stages.
Figure 4. Illustration of the old/new recognition and contextual threat effects in the recognition session. (A) Grand averaged ERPs prompted by correctly classified old faces with correct source allocation, and new faces for an exemplary central sensor (C1) and a topographical difference map (old – new) displaying the averaged time interval (752–900 ms) plotted on a top view of a model head. Illustration of the main effect of Context in the recognition phase. (B) Grand averaged ERPs for faces which have been previously presented within threat-of-shock or safe context in the encoding session and new faces for an exemplary parieto-occipital sensor (PO4) and topographical difference maps (threat – new, threat – safe) displaying the averaged time interval (700–900 ms) plotted on a back view of a model head.
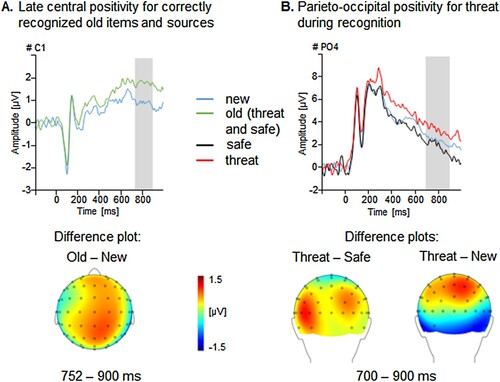
Third, this effect was further differentiated based on correct context recognition (i.e. threatening or safe context conditions relative to identifying a face as new). Specifically, context-dependent faces (compared to new faces) were associated with late parieto-occipital positivity (700-900ms), Context F(2,57) = 3.19, p < .05, ηp2 = .05 [.00, .22]. Post-hoc tests showed that this effect was driven by the threat context, revealing enhanced positivity for faces originating from a threat compared to a safe context or new faces (see (B)). Enhanced amplitudes were found over the right hemisphere, Laterality F(2,57) = 6.30, p < .01, ηp2 = .10 [.04, .31]. Learning groups did not differ, F < 1.
3.3.2. Visual working memory: N170 and contralateral delay activity
A more pronounced N170 was found for high- compared to low-load face presentations (i.e. four compared to two faces) over parieto-occipital sites, Load F(1,57) = 21.62, p < .001, ηp2 = .28 [.25, .54]. There was no main effect of Laterality, Learning or Context, Fs < 1.49, ps > .23 (see (A)).
Figure 5. Visual working memory: load and context effects for N170 and CDA. Illustration of the load effect in during change detection. (A) Grand averaged N170 plotted as a function of memory load (low (1 face) vs. high (2 faces)) and Context (threat vs. safety) for an exemplary parieto-occipital sensor and a topographical difference map (high – low load) displaying the averaged time interval of peak detection (120–190 ms) plotted on a back view of a model head. Illustration of the load and context effect during change detection. (B) CDA (contralateral minus ipsilateral), recorded at the CP5-CP6 sites, time-locked to the onset of the memory array, plotted as a function of set size (one face (low load) vs. two faces (high load)) and as a function of context (threat vs. safety). Waveforms were filtered with a high cutoff filter of 10 Hz for visual inspection only. Top views of topographical maps for each experimental condition (one vs. two faces and threat vs. safety contexts) recorded during a 500–900-ms interval following the onset of the memory array.
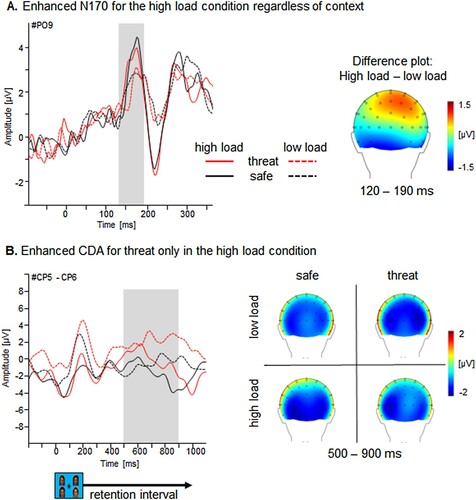
A main effect of Context emerged for CP1-CP2 (236–336ms), F(1,57) = 6.61, p < .05, ηp2 = .10 [.01, .24], showing an pronounced negative CDA for the safe compared to the threat context (see (B)), indicating that the safe context gained more access into working memory than the threatening context. Unexpectedly, there was no main effect of Load, F(1,57) = 1.19, p = .28, ηp2 = .02 [.00, .11], or interaction Context × Load, F(1,57) = 2.70, p = .12, ηp2 = .05 [.00, .16], suggesting that working memory capacity was not modulated by task difficulty and memory strain, even with changing context conditions. No main effect of Learning type occurred, F < 1. However, follow-up tests for the significant Context × Learning interaction, F(1,57) = 9.63, p < .01, ηp2 = .14 [.03, .28], revealed that the observation group had particular difficulty retaining the safety information from decreasing working memory capacity indicated by an enhanced negative CDA for the safety compared to the threat context for this group. There was no difference in the threat compared to the safe context for the instruction group. Laterality had no impact, Fs < 1. A marginal context effect emerged for CP6-CP5 (500-900ms), F(1,57) = 3.72, p = .059, ηp2 = .06 [.00, .18], with an enhanced CDA amplitude for the safe compared to the threat context. In this later time window, an enhanced CDA was observed for high- relative to low-load condition, F(1,57) = 5.81, p < .05, ηp2 = .09 [.01, .22], and this finding is in accordance with the hypotheses that higher load consumes working memory resources and reduces its capacity. No interaction effect between Context × Load, nor a main or interaction effect of learning type occurred, Fs < 3.72, p > .059.
3.4. Impact of adverse childhood experiences and social anxiety
The evaluation of the threat and safety contexts (i.e. valence, arousal, and perceived threat ratings) as well as the results regarding electrocortical threat processing (i.e. during encoding in the item/source memory task) were not altered by adverse childhood experiences. However, similar to our previous study (Schellhaas et al., Citation2020), a trend-level interaction Context × SPIN showed amplified electrocortical differences between threat and safety in more socially anxious participants during the encoding phase, F(1,61) = 3.37, p = .07, ηp2 = .05 [.00, .16].
Regarding ACE and behavioural performance in the item/source memory task (conventional measures; i.e. face recognition), a covariation of the hit rate emerged with the CTQ subscales sexual abuse and emotional neglect, F(1,62) = 4.83, p < .05, ηp2 = 0.08 [.00, .19] and F(1,62) = 4.31, p < .05, ηp2 = .07 [.00, .18]. This finding indicates that highly traumatized individuals had an increased hit rate for recognizing faces that were encoded during contextual threat. Moreover, several trend-level covariations emerged between state anxiety and hit rates (STAI-S × Context, F(1,62) = 2.68, p = .07, ηp2 = .05 [.00, .14]), as well as social anxiety and false alarm rates (SPIN × Context: F(1,62) = 3.47, p = .07, ηp2 = .05 [.00, .16]). Specifically, individuals with higher scores in state anxiety showed higher hit rates for recognizing safety items, and lower false alarm rates were found for threat items in more socially anxious participants, suggesting a better performance for highly anxious persons in a safe context.
For the change detection task (i.e. visual working memory capacity), covariations between Context and the CTQ subscales emotional abuse and emotional neglect emerged for the hit rate, F(1,54) = 6.74, p < .05, ηp2 = .11 [.01, .22] and F(1,54) = 5.68, p < .05, ηp2 = .10 [.01, .23]. These effects indicate that individuals with higher levels of emotional abuse had a higher chance of detecting change under threat, whereas highly emotionally neglected individuals performed better under safety than threat. Moreover, regarding false alarms, a covariation of task Load and emotional and physical neglect was found, F(1,54) = 5.50, p < .05, ηp2 = .09 [.01, .21] and F(1,54) = 4.92, p < .05, ηp2 = .08 [.00, .21], showing a higher FAR during high load for neglected participants. A trend level effect was found Context × SPIN (F(1,59) = 3.71, p = .06, ηp2 = .06 [.00, .19]), showing that the more socially anxious the participants were, the lower the FAR was in recognizing changes in threatening contexts. Also for the d‘ parameter, a significant interaction of context and social anxiety (SPIN) was found, F(1,59) = 4.96, p < .05, ηp2 = .08 [.00, .20]. Socially anxious participants were better in detecting a change within threatening compared to safe contexts.
With regard to the k parameter, an index of visual working memory capacity, there were significant interactions of task Load × SPIN, F(1,59) = 4.18, p < .05, ηp2 = .07 [.00, .18] as well as Load × Emotional Neglect, F(1,54) = 4.90, p < .05, ηp2 = .08 [.00, .20], and Load × Physical Neglect, F(1,54) = 4.28, p < .05, ηp2 = .07 [.00, .20]. These interactions indicate that participants with high levels of social anxiety and emotional neglect had a higher k for the low compared to the high load condition, whereas physically neglected individuals showed a higher k for high load. Moreover, trend level interactions emerged for Context × SPIN, F(1,59) = 3.35, p = .07, ηp2 = .05 [.00, .17] and Context × Emotional Abuse, F(1,54) = 5.10, p < .05, ηp2 = .09 [.01, .20]. These interactions indicate that highly anxious participants had lower VWM capacity during threat compared to safety conditions, whereas emotionally abused individuals seemed to benefit more from the threat with a higher k.
When using the preregistered dimensional approach, overall electrocortical processing did not vary with adverse childhood experiences or social anxiety scores. Additional exploratory analyses based on grouping participants by their CTQ and anxiety scores can be found in the Supplementary section.
4. Discussion
4.1. Summary of main findings
This preregistered study examined effects of social threat/safety learning – through verbal instructions or observing others – on face perception and memory in individuals with adverse childhood experiences. While participants experienced contextual threat of receiving shocks or safety, we focused on two memory modalities (item/source and visual working memory). As confirmed by self-reported ratings, the threat context was perceived as more arousing, threatening, and unpleasant relative to the safety condition. Interestingly, social threat induction was equally effective for both verbal and observational learning, and this was also evident for electrocortical processing. At the behavioural level, contextual safety seemed to facilitate face recognition in the item/source memory task, especially for the more socially anxious participants. In contrast, visual working memory was not affected by context conditions. However, the more socially anxious participants appear to perform better to threat than to safety and have a lower false alarm rate for detecting changes in the visual field. In addition, as expected, visual working memory was impaired in the more demanding task (high task load with four faces).
Regarding electrocortical activity, threat-selective processing patterns were observed during memory encoding in the item/source memory task. Confirming an old-new recognition effect, previously seen faces (old) revealed differential processing relative to unknown (new) faces. Interestingly, although behavioural recognition performance was overall poor, threat-selective face processing was observed during recognition (late parieto-occipital positivity for threat compared to safe and new faces; Schellhaas et al., Citation2020). Regarding visual working memory, task load reduced processing as revealed by N170 and CDA (late time window) in the high load condition. Consistent with the behavioural results, the presence of a threatening context gained more access to working memory and decreased its capacity, as evidenced by an increased CDA for the threat compared to the safe context. Overall, the present data suggest that regardless of the severity of childhood trauma, contextual threat alters face perception and reduces face recognition and storage, especially when cognitive demands are high. However, individuals with high social and state anxiety appear to benefit from the threatening contextual conditions and show improved detection of visual changes. Contrary to our expectations, the present sample of individuals with adverse childhood experiences did not show altered electrocortical processing as a function of trauma severity (i.e. CTQ scores) while performing memory tasks (i.e. face–context recognition task and visual working memory task). However, task performance in both memory tasks varied as a function of context conditions (i.e. threat and safety) and types of adverse childhood experiences (i.e. CTQ subscale scores).
4.2. Item and source memory: safety-enhanced face recognition but poor source memory
For the item/source memory task, participants were asked to indicate whether they have seen a face (i.e. the item) before and, if so, in what context (i.e. threat or safety source). On the electrocortical level, threat-selective face processing was observed in the encoding phase at two processing stages (parieto-occipital 250-400ms and fronto-central 540–800ms). In addition, we replicated an old/new recognition effect showing enhanced processing for correctly recognized old faces (parieto-occipital 660-800ms, and central 750-900ms), which also varied for threat compared to safe and new faces (parieto-occipital 700-900ms). Interestingly, this neural differentiation between threat- and safety-associated faces did not result in improved recognition memory, i.e. participants were unable to consciously identify the context in which they had previously encountered a face. This dissociation of neural measures and behavioural recognition performance is a direct replication of a previous study in a healthy sample (Schellhaas et al., Citation2020), and goes along with studies showing that neural processing is more sensitive to subtle context differentiation than behavioural performance (Luck et al., Citation1996; Sessa et al., Citation2011). It is therefore suggested that an observed dissociation between ERPs and behaviour is not due to the absence of processing modulations underlying a cognitive task (Wilkinson & Halligan, Citation2004), but may vary with subtle neural processing not resulting in overt behavioural changes.
Building upon previous research (Kensinger, Citation2009; Ventura-Bort et al., Citation2016), we predicted that an arousing context will enhance face memory. This hypothesis is based on the Arousal Biased Competition model (ABC; Mather & Sutherland, Citation2011), which assumes that processing of arousing and therefore salient stimuli is enhanced, whereas less salient stimuli must compete more vigorously for attentional resources. In contrast, our results showed a tendency for better recognition of faces encoded during safety, while the context was not remembered at all. Several alternative explanation may account for these diverging findings. Bisby et al. (Citation2018) suggest that the presence of a negative (context) element weakens the item-context association while leading to an enhancement in item recognition. However, this presupposes a temporal or conceptual link between face and context (item-source binding; Dunsmoor et al., Citation2015), which was not the case in the current study because face-context associations were not reinforced.
Alternatively, attentional resources may have been allocated exclusively to the threatening context during encoding, resulting in enhanced processing of threat versus safety/new information during both encoding and recognition, but impaired recognition of faces from the threatening context. Finally, new information and especially faces need time to consolidate (McGaugh, Citation2000). For instance, memory for faces is strongly depending on familiarity with a person (Burton & Jenkins, Citation2011) and neutral looking unknown faces are per se difficult to recognize (Arnold et al., Citation2021). Here, explicit learning instructions may enhance the strength of face-context association, and longer consolidation periods between encoding and recognition phase seem pertinent to boost item and source memory (e.g. a gap of one hour, day or even week; Ventura-Bort et al., Citation2016).
4.3. Visual working memory: reduced capacity with high load and threat
The visual working memory task required detection of changes in a visual scene displaying either two or four face identities (low and high task load). In accordance with our hypotheses, change detection performance was impaired under high task load, suggesting limited cognitive resources (Sessa et al., Citation2011). This effect was also found in electrocortical processing, which showed increased contralateral delay activity (CDA) and N170 for the high- compared to low-load condition, reflecting changes in working memory capacity (Morgan et al., Citation2008). Regarding the impact of threat, the contextual CDA modulation confirmed previous findings showing a reduced capacity for threat (Stout et al., Citation2013). Thus, threatening information plays a key role in the modulation of the CDA showing an increasing amplitude the more relevant the threatening information becomes (Ward et al., Citation2020).
Again, result patterns for perceptual processing and overt behavioural performance do not match, which may indicate low behavioural relevance of contextual threat in the present study. In contrast to that notion, individuals with ACE who showed more socially anxious symptomatology were better in detecting visual changes within a threatening context (lower false alarm rates, higher k and d’ parameter). This finding is in line with the Attentional Control Theory (Eysenck et al., Citation2007), which assumes that arousal consumes working memory resources and thereby reduces the ability to actively inhibit distracting information. This claim is supported by several studies showing that high state, trait and social anxiety is associated with attentional distraction by task irrelevant stimuli (Eysenck & Derakshan, Citation2011; Moriya & Sugiura, Citation2012). Thus, anxiety interferes with working memory processes (inhibition shifting, updating) and persons with high trait anxiety levels perform worse on demanding tasks, or alternatively, must put in compensatory efforts that lead to a good task performance with a trade-off in efficiency (slower reaction times; Edwards et al., Citation2015). In the present change detection task (without distracting elements), contextual threat may have led to increased attention and alertness in particularly anxious individuals with ACE, thereby boosting memory performance during threat (Robinson et al., Citation2013).
4.4. Social threat and safety learning: verbal and observed information is similarly effective
Contextual threat or safety was learned through verbal instructions or observing a model receiving electrical shocks. Similar to previous research (Bublatzky et al., Citation2020; Olsson & Phelps, Citation2007), such social means of learning were highly effective in establishing a threatening context, which was perceived as more threatening, unpleasant and arousing, and elicited threat-selective electrocortical processing compared to the safety context (Arnold et al., Citation2021; Schellhaas et al., Citation2020). Interestingly, however, these threat effects were independent of social learning type and did not vary with trauma severity or anxiety measures in individuals with adverse childhood experiences.
Specifically, no differences were found between learning through verbal instructions and observing others neither for ratings, behaviour or electrocortical measures. This finding is in line with recent research suggesting that both verbal and observational threat learning show indistinguishable behavioural effects (comparable to direct threat learning; Olsson & Phelps, Citation2007). However, regarding the underlying neural network partly different regions have been suggested to be involved in verbal and observational learning (e.g. ACC, anterior insula; Lindström et al., Citation2018). Exploring these differences and how to counteract maladaptive social threat and safety learning, for example, when it leads to pathological anxiety, is an important goal for future research. In particular, supporting correct recall of threatening and/or safe situations may prove helpful in overcoming psychopathological anxious arousal.
4.5. Adverse childhood experiences and inter-individual differences in anxiety
The impact of adverse childhood experiences (ACE) can be diverse and different types and timings can have distinct effects on neural development, behaviour, and psychopathology (Herzog & Schmahl, Citation2018; Sheridan & McLaughlin, Citation2014). In this study, we followed a trans-diagnostic approach with the childhood trauma questionnaire (CTQ) as a dimensional measure. This resulted in a unique and heterogeneous sample of participants who experienced varying degrees of childhood abuse and neglect, often simultaneously, ranging from milder CTQ scores to moderate and severe effects of childhood maltreatment. In this highly ecologically valid sample, we found intact memory processing despite the presence of sometimes severe ACE and comorbid psychopathology. Contrary to our preregistered hypotheses, we did not find overall altered or generalized threat processing associated with ACE, nor did we find changes in item/source memory or visual working memory, even independent of the cognitive load acting on the memory system (Goodman et al., Citation2019). Instead, we found that contextual safety improved recall of unknown neutral faces independent of recall of the context and severity or type of ACE. Exploratory analyses of the present ACE sample regarding inter-individual differences in social and state anxiety showed that this face recognition advantage increased in more anxious participants.
Regarding different subtypes of ACE, exploratory analyses showed that sexual abuse and emotional neglect were associated with improved recognition of faces from a threatening context. In addition, sexual and emotional abuse were associated with improved working memory during threat, possibly due to increased alertness in stressful situations. In contrast, emotional neglect scores were associated with better working memory under safe conditions (Robinson et al., Citation2013). This may reflect greater recruitment of cognitive resources in order to maintain task performance during low-stress tasks, resulting in equal or improved performance compared to individuals with ACE and low anxiety scores, but at the expense of highly effortful task performance (Moriya & Sugiura, Citation2012). Thus, when task demands are increased by distracting information, inhibition and filtering of these distractions are impaired and performance declines, especially when the distractions are associated with threat (Stout et al., Citation2013). While the tasks at hand were not designed to compare varying degrees of distraction and inhibition as part of the memory processes, however, future studies could be designed to include both threatening and neutral distractors into the memory task to investigate compensatory effects (MacNamara et al., Citation2011; Ward et al., Citation2020).
4.6. Limitations and future directions
First, because the tasks were demanding due to their difficulty (item and source memory) and effort (visual working memory), motivation plays an important role. Participants spent approximately 30 min on each task, with a short break in between, resulting in a long individual task and total study time. Motivation is related to cognitive control and load, and a lack of it can lead to decreased performance. To increase motivation, performance-based incentives or a time limit for responses could be introduced, especially in the visual working memory task, which in turn could lead to a reduced influence of the motivational effect.
Second, our ACE sample may consist of more high-functional individuals (in terms of memory functioning). Besides self-selection, our exclusion criteria were rather strict (e.g. no medication except SSRIs and SNRIs, no drug intake or comorbid addiction). Given that, the overall severity of adverse childhood experiences, was rather profound (medium-high CTQ score), and mainly driven by emotional abuse and neglect. However, the more severe cases of early traumatization, such as physical and sexual abuse, are more likely to develop severe psychiatric disorders and comorbidities leading to the use of psychotropic medications, the pool of severely traumatized participants in the current study is limited. This in turn could have led to comparable memory performance with regard to healthy participants (Schellhaas et al., Citation2020). Future studies may consider the inclusion of measures of resiliency and coping mechanisms to characterize the nature of high-functional maltreatment survivors and/or add more liberal inclusion criteria for including individuals with severe adverse childhood experiences.
Third, the results show a dissociation between perceptual/attentional processes and behavioural measures. Future research should address whether this dissociation is reflected in psychophysiological response priming, for instance, whether participants show autonomic arousal to faces encountered in a threatening context but are not retrieved from memory. This is also of clinical relevance, as identifying unconscious triggers of arousal is a key element of many treatments (e.g. for PTSD; Cahill & Foa, Citation2007). Moreover, in the age of a highly socially networked world (e.g. social media), the importance of socially mediated threat triggers is increasing in general and in psychopathology.
Fourth, both type and timing of adverse events during childhood play a critical role and have an impact on brain maturation. For instance, sensitive periods and specific ACE-subtypes influence the development of neurobiological alterations differentially (e.g. cortical thickness; Herzog & Schmahl, Citation2018), leading to differential effects of memory processes in adulthood. Therefore, more sensitive measures of the type and timing of ACEs should be included in future studies. For example, in using the CTQ scale as a measure of adverse childhood experiences, future studies should consider non-linear statistical approaches to gain more differentiated insights into the impact of childhood maltreatment on cognition. The focus of this study was on the total score of adverse childhood experiences. However, some of the subscales of the CTQ were not normally distributed, so the results regarding these subscales may be limited due to a conservative interpretation.
Finally, although most of our participants were between 25 and 37 years old, the age range in our sample was quite wide and unevenly distributed (19-60 years), and age-related differences could not be addressed with the data at hand. To address developmental aspects of adverse childhood experiences, future research will need to directly address younger and older populations. For example, ACEs have been linked to cognitive decline and impairments during adolescence (< 18 years of age) as well as older age (> 60 years; Blakemore, Citation2019; Halpin et al., Citation2021; Korten et al., Citation2014; Murman, Citation2015; Verhaeghen & Salthouse, Citation1997). However, the association between adverse childhood experiences, increasing age, and cognitive deficits is not well-understood (Gold et al., Citation2021; Ritchie et al., Citation2011).
4.7. Conclusions
In a unique sample of adult individuals with adverse childhood experiences, this study shows that contextual threat and safety lead to processing differences in face perception, recognition, and change detection. While overall intact social threat and safety learning was found, threat-selective face processing was observed in an item/source memory task and the threat context required more processing resources in a visual working memory tasks. Regarding behavioural performance, generally safety seemed to facilitate face recognition in low anxious individuals with low/moderate levels of ACEs. In contrast, highly anxious and severely maltreated individuals showed better task performance in aversive anticipatory contexts. More research is needed to examine the psychophysiological processes involved in functional and dysfunctional memory systems, and their relevance as vulnerability factors for stress-related disorders.
Supplemental Material
Download MS Word (93 KB)Acknowledgements
We are grateful to Tana Spethmann and Maximilian Frank for their help in data collection, Angelika Panizza, Marija Gligorijevic and the Team of the German Research Foundation (Deutsche Forschungsgemeinschaft, DFG) funded research training group GRK2350 for the help in recruitment and screening for eligibility.
Disclosure statement
No potential conflict of interest was reported by the author(s).
Data availability statement
The data that support the findings of this study are openly available in OSF at https://osf.io/u2nq7/?view_only = 59fd90797a184c7d8cac8ad194d20f0e.
Additional information
Funding
References
- American Psychiatric Association. (2000). Diagnostic and statistical manual of mental disorders (4th ed., text rev.). American Psychiatric Association.
- American Psychiatric Association. (2013). Diagnostic and statistical manual of mental disorders (5th ed.). American Psychiatric Association. https://doi.org/10.1176/appi.books.9780890425596
- Arnold, N. R., Bayen, U. J., & Böhm, M. F. (2015). Is prospective memory related to depression and anxiety? A hierarchical MPT modelling approach. Memory, 23(8), 1215–1228. https://doi.org/10.1080/09658211.2014.969276
- Arnold, N. R., Bayen, U. J., Kuhlmann, B. G., & Vaterrodt, B. (2013). Hierarchical modeling of contingency-based source monitoring: A test of the probability-matching account. Psychonomic Bulletin & Review, 20(2), 326–333.
- Arnold, N. R., González Cruz, H., Schellhaas, S., & Bublatzky, F. (2021). A multinomial modelling approach to face identity recognition during instructed threat. Cognition and Emotion, 35(7), 1302–1319. https://doi.org/10.1080/02699931.2021.1951175
- Assed, M. M., Khafif, T. C., Belizario, G. O., Fatorelli, R., Rocca, C. C. D. A., & de Pádua Serafim, A. (2020). Facial emotion recognition in maltreated children: A systematic review. Journal of Child and Family Studies, 29(5), 1493–1509. https://doi.org/10.1007/s10826-019-01636-w
- Bar-Haim, Y., Lamy, D., Pergamin, L., Bakermans-Kranenburg, M. J., & Van Ijzendoorn, M. H. (2007). Threat-related attentional bias in anxious and nonanxious individuals: a meta-analytic study. Psychological Bulletin, 133(1), 1–24. https://psycnet.apa.org/doi/10.10370033-2909.133.1.1. https://doi.org/10.1037/0033-2909.133.1.1
- Bayen, U. J., Murnane, K., & Erdfelder, E. (1996). Source discrimination, item detection, and multinomial models of source monitoring. Journal of Experimental Psychology: Learning, Memory, and Cognition, 22(1), 197–215. https://doi.org/10.1037/0278-7393.22.1.197
- Bell, R., & Buchner, A. (2011). Source memory for faces is determined by their emotional evaluation. Emotion, 11(2), 249–261. https://doi.org/10.1037/a0022597
- Bernstein, D. P., Fink, L., Handelsman, L., & Foote, J. (1998). Childhood trauma questionnaire. Assessment of family violence: A handbook for researchers and practitioners. APA PsycTests. https://doi.org/10.1037/t02080-000
- Bisby, J. A., Horner, A. J., Bush, D., & Burgess, N. (2018). Negative emotional content disrupts the coherence of episodic memories. Journal of Experimental Psychology: General, 147(2), 243–256. https://doi.org/10.1037/xge0000356
- Blakemore, S. J. (2019). Adolescence and mental health. The Lancet, 393(10185), 2030–2031. https://doi.org/10.1016/S0140-6736(19)31013-X
- Bradley, M. M., & Lang, P. J. (1994). Measuring emotion: The self-assessment manikin and the semantic differential. Journal of behavior Therapy and experimental Psychiatry, 25(1), 49–59. https://doi.org/10.1016/0005-7916(94)90063-9
- Bremner, J. D., Vythilingam, M., Vermetten, E., Southwick, S. M., McGlashan, T., Staib, L. H., Soufer, R., & Charney, D. S. (2003). Neural correlates of declarative memory for emotionally valenced words in women with posttraumatic stress disorder related to early childhood sexual abuse. Biological Psychiatry, 53(10), 879–889. https://doi.org/10.1016/S0006-3223(02)01891-7
- Brewin, C. R. (2011). The nature and significance of memory disturbance in posttraumatic stress disorder. Annual Review of Clinical Psychology, 7(1), 203–227. https://doi.org/10.1146/annurev-clinpsy-032210-104544
- Brewin, C. R., Huntley, Z., & Whalley, M. G. (2012). Source memory errors associated with reports of posttraumatic flashbacks: A proof of concept study. Cognition, 124(2), 234–238. https://doi.org/10.1016/j.cognition.2012.05.002
- Bublatzky, F., Flaisch, T., Stockburger, J., Schmälzle, R., & Schupp, H. T. (2010). The interaction of anticipatory anxiety and emotional picture processing: An event-related brain potential study. Psychophysiology, 47(4), 687–696. https://doi.org/10.1111/j.1469-8986.2010.00966.x
- Bublatzky, F., Gerdes, A. B., & Alpers, G. W. (2014). The persistence of socially instructed threat: Two threat-of-shock studies. Psychophysiology, 51(10), 1005–1014. https://doi.org/10.1111/psyp.12251
- Bublatzky, F., Guerra, P., & Alpers, G. W. (2018). Verbal instructions override the meaning of facial expressions. Scientific Reports, 8(1), 1–11. https://doi.org/10.1038/s41598-018-33269-2
- Bublatzky, F., Guerra, P., & Alpers, G. W. (2020). Watch out, he's dangerous! Electrocortical indicators of selective visual attention to allegedly threatening persons. Cortex, 131, 164–178. https://doi.org/10.1016/j.cortex.2020.07.009
- Bublatzky, F., Schellhaas, S., & Guerra, P. (2022). The mere sight of loved ones does not inhibit psychophysiological defense mechanisms when threatened. Scientific Reports, 12(1), 1–10. https://doi.org/10.1038/s41598-022-06514-y
- Bublatzky, F., & Schupp, H. T. (2012). Pictures cueing threat: Brain dynamics in viewing explicitly instructed danger cues. Social Cognitive and Affective Neuroscience, 7(6), 611–622. https://doi.org/10.1093/scan/nsr032
- Burton, A. M., & Jenkins, R. (2011). Unfamiliar face perception. In A. Calder, G. Rhodes, M. Johnson & J. Haxby (Eds.), The Oxford handbook of face perception (vol. 28, pp. 287–306). Oxford University Press.
- Cahill, S. P., & Foa, E. B. (2007). Psychological theories of PTSD. In M. J. Friedman, T. M. Keane & P. A. Resick (Eds.), Handbook of PTSD: Science and practice (pp. 55–77. Guilford Press.
- Curtis, W. J., & Cicchetti, D. (2011). Affective facial expression processing in young children who have experienced maltreatment during the first year of life: An event-related potential study. Development and Psychopathology, 23(2), 373–395. https://doi.org/10.1017/S0954579411000125
- Dannlowski, U., Kugel, H., Huber, F., Stuhrmann, A., Redlich, R., Grotegerd, D., Dohm, K., Sehlmeyer, C., Konrad, C., Baune, B. T., Arolt, V., Heindel, W., Zwitserlood, P., & Suslow, T. (2013). Childhood maltreatment is associated with an automatic negative emotion processing bias in the amygdala. Human brain Mapping, 34(11), 2899–2909. https://doi.org/10.1002/hbm.22112
- Dodge, K. A., Pettit, G. S., Bates, J. E., & Valente, E. (1995). Social information-processing patterns partially mediate the effect of early physical abuse on later conduct problems. Journal of Abnormal Psychology, 104(4), 632–643. https://doi.org/10.1037/0021-843X.104.4.632
- Doretto, V., & Scivoletto, S. (2018). Effects of early neglect experience on recognition and processing of facial expressions: A systematic review. Brain Sciences, 8(1), Article 10. https://doi.org/10.3390/brainsci8010010
- Dube, S. R., Felitti, V. J., Dong, M., Giles, W. H., & Anda, R. F. (2003). The impact of adverse childhood experiences on health problems: Evidence from four birth cohorts dating back to 1900. Preventive Medicine, 37(3), 268–277. https://doi.org/10.1016/S0091-7435(03)00123-3
- Dunsmoor, J. E., Murty, V. P., Davachi, L., & Phelps, E. A. (2015). Emotional learning selectively and retroactively strengthens memories for related events. Nature, 520(7547), 345–348. https://doi.org/10.1038/nature14106
- Edwards, E. J., Edwards, M. S., & Lyvers, M. (2015). Cognitive trait anxiety, situational stress, and mental effort predict shifting efficiency: Implications for attentional control theory. Emotion, 15(3), 350–359. https://doi.org/10.1037/emo0000051
- Erdfelder, E., Faul, F., & Buchner, A. (1996). GPOWER: A general power analysis program. Behavior Research Methods, Instruments, & Computers, 28(1), 1–11. https://doi.org/10.3758/BF03203630
- Eysenck, M. W., & Derakshan, N. (2011). New perspectives in attentional control theory. Personality and Individual Differences, 50(7), 955–960. https://doi.org/10.1016/j.paid.2010.08.019
- Eysenck, M. W., Derakshan, N., Santos, R., & Calvo, M. G. (2007). Anxiety and cognitive performance: Attentional control theory. Emotion, 7(2), 336–353. https://psycnet.apa.org/doi/10.10371528-3542.7.2.336. https://doi.org/10.1037/1528-3542.7.2.336
- Fang, J., Wang, S., Liu, J., & Gong, J. (2019). Early ERP components to emotional facial expressions in young adult victims of childhood maltreatment. Psychiatry Research, 275, 120–128. https://doi.org/10.1016/j.psychres.2019.03.024
- Fusar-Poli, P., Placentino, A., Carletti, F., Landi, P., Allen, P., Surguladze, S., Benedetti, F., Abbamonte, M., Gasparotti, R., Barale, F., Perez, J., McGuire, P., & Politi, P. (2009). Functional atlas of emotional faces processing: A voxel-based meta-analysis of 105 functional magnetic resonance imaging studies. Journal of Psychiatry and Neuroscience, 34(6), 418–432. https://www.jpn.ca/content/jpn/34/6/418.full.pdf
- Galletly, C., Clark, C. R., McFarlane, A. C., & Weber, D. L. (2001). Working memory in posttraumatic stress disorder—an event-related potential study. Journal of Traumatic Stress, 14(2), 295–309. https://doi.org/10.1023/A:1011112917797
- Gold, A. L., Meza, E., Ackley, S. F., Mungas, D. M., Whitmer, R. A., Mayeda, E. R., Miles, S., Eng, C. W., Gilsanz, P., & Glymour, M. M. (2021). Are adverse childhood experiences associated with late-life cognitive performance across racial/ethnic groups: Results from the Kaiser healthy aging and diverse life experiences study baseline. BMJ Open, 11(2), Article e042125. https://doi.org/10.1136/bmjopen-2020-042125
- Goodman, J. B., Freeman, E. E., & Chalmers, K. A. (2019). The relationship between early life stress and working memory in adulthood: A systematic review and meta-analysis. Memory, 27(6), 868–880. https://doi.org/10.1080/09658211.2018.1561897
- Haaker, J., Golkar, A., Selbing, I., & Olsson, A. (2017). Assessment of social transmission of threats in humans using observational fear conditioning. Nature Protocols, 12(7), 1378–1386. https://doi.org/10.1038/nprot.2017.027
- Halpin, A. B., MacAulay, R. K., Boeve, A. R., D’Errico, L. M., & Michaud, S. (2021). Are adverse childhood experiences associated with worse cognitive function in older adults? Journal of the International Neuropsychological Society, 1–10. https://doi.org/10.1017/S1355617721001272
- Hautus, M. J. (1995). Corrections for extreme proportions and their biasing effects on estimated values of d′. Behavior Research Methods, Instruments, & Computers, 27(1), 46–51. https://doi.org/10.3758/BF03203619
- Heck, D. W., Arnold, N. R., & Arnold, D. (2018). TreeBUGS: An R package for hierarchical multinomial-processing-tree modeling. Behavior Research Methods, 50(1), 264–284. https://doi.org/10.3758/s13428-017-0869-7
- Herzog, J. I., & Schmahl, C. (2018). Adverse childhood experiences and the consequences on neurobiological, psychosocial, and somatic conditions across the lifespan. Frontiers in Psychiatry, 9, Article 420. https://doi.org/10.3389/fpsyt.2018.00420
- Iffland, B., Klein, F., Schindler, S., Kley, H., & Neuner, F. (2021). “She finds you abhorrent”-The impact of emotional context information on the cortical processing of neutral faces in depression. Cognitive, Affective, & Behavioral Neuroscience, 21(2), 426–444. https://doi.org/10.3758/s13415-021-00877-x
- Ikkai, A., McCollough, A. W., & Vogel, E. K. (2010). Contralateral delay activity provides a neural measure of the number of representations in visual working memory. Journal of Neurophysiology, 103(4), 1963–1968. https://doi.org/10.1152/jn.00978.2009
- Karl, A., Malta, L. S., & Maercker, A. (2006). Meta-analytic review of event-related potential studies in post-traumatic stress disorder. Biological Psychology, 71(2), 123–147. https://doi.org/10.1016/j.biopsycho.2005.03.004
- Kavcıoğlu, F. C., Bublatzky, F., Pittig, A., & Alpers, G. W. (2021). Instructed threat enhances threat perception in faces. Emotion, 21(2), 419–429. https://doi.org/10.1037/emo0000708
- Keightley, M. L., Chiew, K. S., Anderson, J. A., & Grady, C. L. (2011). Neural correlates of recognition memory for emotional faces and scenes. Social Cognitive and Affective Neuroscience, 6(1), 24–37. https://doi.org/10.1093/scan/nsq003
- Kensinger, E. A. (2007). Negative emotion enhances memory accuracy: Behavioral and neuroimaging evidence. Current Directions in Psychological Science, 16(4), 213–218. https://doi.org/10.1111/j.1467-8721.2007.00506.x
- Kensinger, E. A. (2009). Remembering the details: Effects of emotion. Emotion Review, 1(2), 99–113. https://doi.org/10.1177/1754073908100432
- Korten, N. C., Penninx, B. W., Pot, A. M., Deeg, D. J., & Comijs, H. C. (2014). Adverse childhood and recent negative life events: Contrasting associations with cognitive decline in older persons. Journal of Geriatric Psychiatry and Neurology, 27(2), 128–138. https://doi.org/10.1177/0891988714522696
- Langner, O., Dotsch, R., Bijlstra, G., Wigboldus, D. H., Hawk, S. T., & Van Knippenberg, A. D. (2010). Presentation and validation of the Radboud Faces Database. Cognition & Emotion, 24(8), 1377–1388. https://doi.org/10.1080/02699930903485076
- Laux, L., Glanzmann, P., Schaffner, P., & Spielberger, C. D. (1981). Das State-Trait-Angstinventar [The state-trait anxiety inventory]. Beltz Test GmbH.
- Letkiewicz, A. M., Silton, R. L., Mimnaugh, K. J., Miller, G. A., Heller, W., Fisher, J., & Sass, S. M. (2020). Childhood abuse history and attention bias in adults. Psychophysiology, 57(10), Article e13627. https://doi.org/10.1111/psyp.13627
- Lindström, B., Haaker, J., & Olsson, A. (2018). A common neural network differentially mediates direct and social fear learning. NeuroImage, 167, 121–129. https://doi.org/10.1016/j.neuroimage.2017.11.039
- Luck, S. J., Vogel, E. K., & Shapiro, K. L. (1996). Word meanings can be accessed but not reported during the attentional blink. Nature, 383(6601), 616–618. https://doi.org/10.1038/383616a0
- Lundqvist, D., Flykt, A., & Öhman, A. (1998). Karolinska directed emotional faces [Database of standardized facial images]. Department of Clinical Neuroscience, Psychology section. Karolinska Institutet, 91, 630.
- MacNamara, A., Ferri, J., & Hajcak, G. (2011). Working memory load reduces the late positive potential and this effect is attenuated with increasing anxiety. Cognitive, Affective, & Behavioral Neuroscience, 11(3), 321–331. https://doi.org/10.3758/s13415-011-0036-z
- MacNamara, A., Post, D., Kennedy, A. E., Rabinak, C. A., & Phan, K. L. (2013). Electrocortical processing of social signals of threat in combat-related post-traumatic stress disorder. Biological Psychology, 94(2), 441–449. https://doi.org/10.1016/j.biopsycho.2013.08.009
- Mather, M., & Sutherland, M. R. (2011). Arousal-biased competition in perception and memory. Perspectives on Psychological Science, 6(2), 114–133. https://doi.org/10.1177/1745691611400234
- Mathôt, S., Schreij, D., & Theeuwes, J. (2012). Opensesame: An open-source, graphical experiment builder for the social sciences. Behavior Research Methods, 44(2), 314–324. https://doi.org/10.3758/s13428-011-0168-7
- McEwen, B. S. (2002). The neurobiology and neuroendocrinology of stress: Implications for post-traumatic stress disorder from a basic science perspective. Psychiatric Clinics of North America, 25(2), 469–494. https://doi.org/10.1016/S0193-953X(01)00009-0
- McGaugh, J. L. (2000). Memory–a century of consolidation. Science, 287(5451), 248–251. https://doi.org/10.1126/science.287.5451.248
- Moran, T. P. (2016). Anxiety and working memory capacity: A meta-analysis and narrative review. Psychological Bulletin, 142(8), 831–864. https://psycnet.apa.org/doi/10.1037bul0000051. https://doi.org/10.1037/bul0000051
- Morgan, H. M., Klein, C., Boehm, S. G., Shapiro, K. L., & Linden, D. E. (2008). Working memory load for faces modulates P300, N170, and N250r. Journal of Cognitive Neuroscience, 20(6), 989–1002. https://doi.org/10.1162/jocn.2008.20072
- Moriya, J., & Sugiura, Y. (2012). High visual working memory capacity in trait social anxiety. PloS one, 7(4), Article e34244. https://doi.org/10.1371/journal.pone.0034244
- Murman, D. L. (2015). The impact of age on cognition. Seminars in Hearing, 36(3), 111–121. https://doi.org/10.1055/s-0035-1555115
- Olsson, A., & Phelps, E. A. (2007). Social learning of fear. Nature Neuroscience, 10(9), 1095–1102. https://doi.org/10.1038/nn1968
- Öhman, A., Flykt, A., & Esteves, F. (2001). Emotion drives attention: Detecting the snake in the grass. Journal of Experimental Psychology: General, 130(3), 466–478. https://doi.org/10.1037/0096-3445.130.3.466
- Pechtel, P., & Pizzagalli, D. A. (2011). Effects of early life stress on cognitive and affective function: An integrated review of human literature. Psychopharmacology, 214(1), 55–70. https://doi.org/10.1007/s00213-010-2009-2
- Peyk, P., DeCesarei, A., & Junghöfer, M. (2011). Electro magneto encephalograhy software: Overview and integration with other EEG/MEG toolboxes. Computational Intelligence and Neuroscience, 2011, 1–10. https://doi.org/10.1155/2011/861705
- Pollak, S. D., Cicchetti, D., Hornung, K., & Reed, A. (2000). Recognizing emotion in faces: Developmental effects of child abuse and neglect. Developmental Psychology, 36(5), 679–688. https://doi.org/10.1037/0012-1649.36.5.679
- Pollak, S. D., & Sinha, P. (2002). Effects of early experience on children's recognition of facial displays of emotion. Developmental Psychology, 38(5), 784–791. https://doi.org/10.1037/0012-1649.38.5.784
- Pollak, S. D., & Tolley-Schell, S. A. (2003). Selective attention to facial emotion in physically abused children. Journal of Abnormal Psychology, 112(3), 323–338. https://doi.org/10.1037/0021-843X.112.3.323
- R Core Team (2021). R: A language and environment for statistical computing. R Foundation for Statistical Computing. https://www.R-project.org/
- Ritchie, K., Jaussent, I., Stewart, R., Dupuy, A. M., Courtet, P., Malafosse, A., & Ancelin, M. L. (2011). Adverse childhood environment and late-life cognitive functioning. International Journal of Geriatric Psychiatry, 26(5), 503–510. https://doi.org/10.1002/gps.2553
- Robinson, O. J., Vytal, K., Cornwell, B. R., & Grillon, C. (2013). The impact of anxiety upon cognition: Perspectives from human threat of shock studies. Frontiers in Human Neuroscience, 7, Article 203. https://doi.org/10.3389/fnhum.2013.00203
- Rugg, M. D., & Curran, T. (2007). Event-related potentials and recognition memory. Trends in Cognitive Sciences, 11(6), 251–257. https://doi.org/10.1016/j.tics.2007.04.004
- Saar-Ashkenazy, R., Shalev, H., Kanthak, M. K., Guez, J., Friedman, A., & Cohen, J. E. (2015). Altered processing of visual emotional stimuli in posttraumatic stress disorder: An event-related potential study. Psychiatry Research: Neuroimaging, 233(2), 165–174. https://doi.org/10.1016/j.pscychresns.2015.05.015
- Sandre, A., Ethridge, P., Kim, I., & Weinberg, A. (2018). Childhood maltreatment is associated with increased neural response to ambiguous threatening facial expressions in adulthood: Evidence from the late positive potential. Cognitive, Affective, & Behavioral Neuroscience, 18(1), 143–154. https://doi.org/10.3758/s13415-017-0559-z
- Schellhaas, S., Arnold, N., Schmahl, C., & Bublatzky, F. (2020). Contextual source information modulates neural face processing in the absence of conscious recognition: A threat-of-shock study. Neurobiology of Learning and Memory, 174, Article 107280. https://doi.org/10.1016/j.nlm.2020.107280
- Schindler, S., & Bublatzky, F. (2020). Attention and emotion: An integrative review of emotional face processing as a function of attention. Cortex, 130, 362–386. https://doi.org/10.1016/j.cortex.2020.06.010
- Schupp, H. T., Öhman, A., Junghöfer, M., Weike, A. I., Stockburger, J., & Hamm, A. O. (2004). The facilitated processing of threatening faces: An ERP analysis. Emotion, 4(2), 189–200. https://doi.org/10.1037/1528-3542.4.2.189
- Schupp, H. T., Stockburger, J., Codispoti, M., Junghöfer, M., Weike, A. I., & Hamm, A. O. (2007). Selective visual attention to emotion. Journal of Neuroscience, 27(5), 1082–1089. https://doi.org/10.1523/JNEUROSCI.3223-06.2007
- Sessa, P., Luria, R., Gotler, A., Jolicœur, P., & Dell'Acqua, R. (2011). Interhemispheric ERP asymmetries over inferior parietal cortex reveal differential visual working memory maintenance for fearful versus neutral facial identities. Psychophysiology, 48(2), 187–197. https://doi.org/10.1111/j.1469-8986.2010.01046.x
- Sheridan, M. A., & McLaughlin, K. A. (2014). Dimensions of early experience and neural development: Deprivation and threat. Trends in Cognitive Sciences, 18(11), 580–585. https://doi.org/10.1016/j.tics.2014.09.001
- Stangier, U., & Steffens, M. (2001). Social Phobia Inventory—deutsche Fassung [German version]. Psychologisches Institut der Universität Frankfurt am Main.
- Stegmann, Y., Ahrens, L., Pauli, P., Keil, A., & Wieser, M. J. (2020). Social aversive generalization learning sharpens the tuning of visuocortical neurons to facial identity cues. Elife, 9, Article e55204. https://doi.org/10.7554/eLife.55204
- Stout, D. M., Shackman, A. J., & Larson, C. L. (2013). Failure to filter: Anxious individuals show inefficient gating of threat from working memory. Frontiers in Human Neuroscience, 7, Article 58. https://doi.org/10.3389/fnhum.2013.00058
- Tapia, G., Clarys, D., Bugaiska, A., & El-Hage, W. (2012). Recollection of negative information in posttraumatic stress disorder. Journal of Traumatic Stress, 25(1), 120–123. https://doi.org/10.1002/jts.21659
- Tottenham, N., Hare, T. A., Millner, A., Gilhooly, T., Zevin, J. D., & Casey, B. J. (2011). Elevated amygdala response to faces following early deprivation. Developmental Science, 14(2), 190–204. https://doi.org/10.1111/j.1467-7687.2010.00971.x
- Tottenham, N., Tanaka, J. W., Leon, A. C., McCarry, T., Nurse, M., Hare, T. A., Marcus, D. J., Westerlund, A., Casey, B. J., & Nelson, C. (2009). The NimStim set of facial expressions: Judgments from untrained research participants. Psychiatry Research, 168(3), 242–249. https://doi.org/10.1016/j.psychres.2008.05.006
- van Harmelen, A. L., van Tol, M. J., Demenescu, L. R., van der Wee, N. J., Veltman, D. J., Aleman, A., van Buchem, M. A., Spinhoven, P., Penninx, B. W. J. H., & Elzinga, B. M. (2013). Enhanced amygdala reactivity to emotional faces in adults reporting childhood emotional maltreatment. Social Cognitive and Affective Neuroscience, 8(4), 362–369. https://doi.org/10.1093/scan/nss007
- Ventura-Bort, C., Löw, A., Wendt, J., Moltó, J., Poy, R., Dolcos, F., Hamm, A. O., & Weymar, M. (2016). Binding neutral information to emotional contexts: Brain dynamics of long-term recognition memory. Cognitive, Affective, & Behavioral Neuroscience, 16(2), 234–247. https://doi.org/10.3758/s13415-015-0385-0
- Verhaeghen, P., & Salthouse, T. A. (1997). Meta-analyses of age–cognition relations in adulthood: Estimates of linear and nonlinear age effects and structural models. Psychological Bulletin, 122(3), 231–249. https://doi.org/10.1037/0033-2909.122.3.231
- Vuilleumier, P., Armony, J. L., Driver, J., & Dolan, R. J. (2001). Effects of attention and emotion on face processing in the human brain: An event-related fMRI study. Neuron, 30(3), 829–841. https://doi.org/10.1016/S0896-6273(01)00328-2
- Ward, R. T., Lotfi, S., Sallmann, H., Lee, H. J., & Larson, C. L. (2020). State anxiety reduces working memory capacity but does not impact filtering cost for neutral distracters. Psychophysiology, 57(10), Article e13625. https://doi.org/10.1111/psyp.13625
- Weiss, B., Dodge, K. A., Bates, J. E., & Pettit, G. S. (1992). Some consequences of early harsh discipline: Child aggression and a maladaptive social information processing style. Child Development, 63(6), 1321–1335. https://doi.org/10.2307/1131558
- Weymar, M., Bradley, M. M., Hamm, A. O., & Lang, P. J. (2013). When fear forms memories: Threat of shock and brain potentials during encoding and recognition. Cortex, 49(3), 819–826. https://doi.org/10.1016/j.cortex.2012.02.012
- Wilding, E. L., & Rugg, M. D. (1996). An event-related potential study of recognition memory with and without retrieval of source. Brain, 119(3), 889–905. https://doi.org/10.1093/brain/119.3.889
- Wilkinson, D., & Halligan, P. (2004). The relevance of behavioural measures for functional-imaging studies of cognition. Nature Reviews Neuroscience, 5(1), 67–73. https://doi.org/10.1038/nrn1302