ABSTRACT
In this paper, we examine the exchange of crops and livestock through the application of strontium (87Sr/86Sr) isotope analysis on cereal grains and faunal tooth enamel from the regional center of Uppåkra and three nearby settlements in Scania, southern Sweden, dating to the first millennium AD. Around a third of the fauna have non-local 87Sr/86Sr values, indicating the import of livestock from several different regions. After cleaning, almost all of the cereal grains have non-local 87Sr/86Sr values, which is surprising given the nearby abundance of fertile agricultural soils. We therefore suggest considering non-locally grown crops to be those whose 87Sr/86Sr values fall outside the normal distribution; if this approach is used, around 20% of the analyzed crop samples are interpreted as having grown non-locally. This study demonstrates the potential of combining strontium isotopic data of archaeobotanical and zooarchaeological material for gaining insights into the movement of agricultural products in prehistory.
GRAPHICAL ABSTRACT
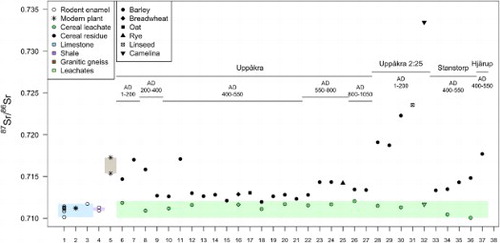
1. Introduction
Several major settlements developed in the Scandinavian Iron Age (500 BC–AD 1050), which came to have central economic, political and religious functions (Hedeager Citation2002; Jørgensen Citation2009). The regional center of Uppåkra, situated in southern Sweden, was one of these high-status settlements with an occupational history lasting over a millennium (c. 100 BC–AD 1000).
The artifactual record from Uppåkra has revealed a highly developed artisanship (Lindell Citation2001; Hårdh Citation2001). A fairly continuous occurrence of bronze casting and large amounts of by-products from bone- and antler-working indicate that production not only fulfilled the needs of the inhabitants at this regional center, but was intended to supply an established network of trade and contacts well outside the settlement (Helgesson Citation2002). The material culture from the site, including finds such as Roman denarii, millefiori, fibulae, objects of glass from the European continent and Arabic silver coins, demonstrates extensive trade relations that expanded across the continent well beyond the local region. Acquaintance with other cultures is further reflected in finds of Roman origin, such as gaming pieces and surgical instruments, that probably attest to a knowledge of board games and surgical practices, respectively. The presence of weighing scales and hack silver further underlines Uppåkra’s role as a trading center (Hårdh Citation2002, Citation2010).
The abundance and quality of its artisanal products and exotic luxury items, reflecting a concentration of wealth not found at contemporary settlements in the region, probably allowed Uppåkra to develop into a centralized and affluent settlement in the first centuries AD. The more ordinary settlements in the surrounding areas appear to have been wealthy settlements as well. However, the economy of the surrounding settlements appears to have been focused on agrarian production and they lack the evidence of large-scale artisan production seen at the regional center (Söderberg Citation2018; Bolander and Söderberg Citation2019).
The large size of Uppåkra (40 hectares) raises the question of how it was supplied with food. Moreover, if the settlement had an economic focus on craft production and trade, along with taking a role in ceremonial and administrative activities for a larger population, an emerging hierarchical society could have shifted the economy of the settlement to be less reliant on its own agriculture, developing a dependence on farmers from outside for its food supplies. Alternatively, growing prosperity at the settlement could have encouraged the development of a particularly strong local agricultural base. Our knowledge of the organization of farming and of the handling of food and other agricultural products at the site is, however, limited.
At present, a few studies on archaeobotanical remains from Uppåkra have envisaged that the handling of plant products involved extended networks of contacts. A system with crop production from the periphery supplying Uppåkra was suggested due to the observed size differences in barley grains (Larsson Citation2018). Larger grains were more frequent at the regional center when compared to sites in the surrounding area, indicating that the regional center could have had access to high-quality crop products from other farming communities. The introduction to Uppåkra of vegetables and condiments in the second century AD, based on finds of seeds from plants typically grown in gardens in the Roman empire, demonstrates that a range of garden plants was cultivated locally (Larsson and Ingemark Citation2015).
Economic classification of sites using the relative proportion of cereal grains, chaff and weed seeds from archaeobotanical assemblages, as an indication of different stages of crop processing, has been used to differentiate between producer and consumer sites and thus identify the presence of trade networks (e.g. Hillman Citation1984; Jones Citation1985). This method, however, requires good preservation of chaff and weed seeds and an understanding of taphonomic processes (Van der Veen Citation2007). Crop by-products and weed ecological attributes, to identify possible trade of grain between Uppåkra and its environs, have not yet been thoroughly analyzed, primarily due to the lack of chaff in archaeobotanical assemblages from Scandinavian Iron Age settlements.
This study will focus on the agricultural base of the regional center Uppåkra by exploring whether the settlement handled crops and livestock from the local area or whether produce originated from other regions. Strontium (Sr) isotope analysis was employed as a potential method to trace the provenence of agricultural products, examining the Sr isotope composition (specifically the mass fractionation corrected ratio of 87Sr/86Sr) of cereal crops and oil plants, and tooth enamel of livestock from a study area consisting of Uppåkra and three more ordinary settlements situated nearby, dating to the first millennium AD. An earlier study based on Sr isotope analysis of the tooth enamel of cattle has indicated the presence of non-local livestock at Uppåkra (Price Citation2013), but this was a smaller study of 14 samples derived only from Uppåkra and the question of livestock mobility in this region therefore merits further investigation.
First, the strontium isotope composition expected for crops growing and animals grazing in the local area was established, based on the 87Sr/86Sr values of archaeological rodent tooth enamel and modern plant samples from the surrounding region, as well as material leached from archaeological cereal grains recovered from Uppåkra and the nearby sites. The 87Sr/86Sr values of archaeological rodent tooth enamel and modern plants from sites located in other regions on bedrock geologies different from the early Tertiary limestone on which Uppåkra is situated were also determined to reveal the variation in 87Sr/86Sr values to be expected in the wider area. The 87Sr/86Sr values of archaeological cereal grain residues (the material remaining after cereal grains were leached in acid) and livestock tooth enamel could then be used to distinguish between local versus non-local sources of agricultural products.
This study aims to further understanding and discuss economic aspects of the agricultural base at the regional center of Uppåkra and the settlements which surrounded it, focusing on the relationship between production and consumption of crops and raising of livestock that constituted the everyday life of individual households. By combining isotopic investigation of both archaeobotanical and zooarchaeological material, this work provides a more holistic insight into past subsistence than previous studies of faunal remains alone. This novel methodological approach thus offers an opportunity to understand aspects of past subsistence strategies, while greater insight into the wider subsistence base and the connectedness of agricultural production contributes to a broader understanding of social interactions within an Iron Age society.
2. Archaeological site area
This study investigates four Iron Age settlements located in the province of Scania in southern Sweden (). The regional center of Uppåkra is the largest archaeological site in the study area. Three sites located within 6 km of Uppåkra – Uppåkra 2:25, Stanstorp and Hjärup – represent settlements in its nearby hinterland. These sites are relatively small in comparison with Uppåkra and were contemporary with the main site at different periods of the Swedish Iron Age (). All sites are found on similar soil types, consisting of uniform Quaternary deposits that are dominated by clay till and smaller areas of sandy soil, overlying limestone bedrock (Daniel, Malmberg Persson, and Persson Citation2000).
Table 1. Chronology of the Swedish Iron Age.
3. Technical background
3.1. The geology of Scania
Scania is located on the border between the very old Fennoscandian bedrock that dominates much of Sweden and Finland and the younger sedimentary bedrock that dominates across Denmark and much of continental Europe (Wikman and Bergström Citation1987).
The central and northern part of Scania consists of Precambrian Fennoscandian bedrock, mainly granitoids and gneisses, between 1870 and 1000 million years in age (see ). This Precambrian bedrock can also be found in smaller ridges further south. In a tectonic zone running from northwest to southeast, there is a mixed bedrock of limestone, shale and sandstone, mainly dating to the Cambrian/Ordovician and Triassic/Jurassic periods, about 570–65 million years old. In the northeast there is a region with Cretaceous limestone, and in the southwest a region with Tertiary limestone, which is the youngest bedrock in Scania and Sweden, formed about 65–55 million years ago. This Tertiary limestone continues in Denmark to the west.
The archaeological sites in southwestern Scania presented in this study are all situated on Tertiary limestone.
3.2. Strontium isotope baselines
Previous studies, using the 87Sr/86Sr values of various biological materials – such as bones and teeth of rodents, wool, modern plants and shells of land snails – have established a framework of Sr isotope baselines for different regions of southern Scandinavia, Denmark and northern Germany (Frei and Price Citation2012; Sjögren, Price, and Ahlström Citation2009; Price Citation2013; Sjögren and Price Citation2013; Arcini Citation2018; Kjällquist and Price Citation2019). In this study, additional 87Sr/86Sr values of rodent tooth enamel, modern plant samples and material leached from archaeological grains have been included to obtain a higher resolution Sr isotope baseline for the area local to Uppåkra (see Section 5.1).
3.3. Strontium in plants and animals
During growth, plants take up Sr from the bioavailable fraction in the soil, which is mainly Sr dissolved in soil pore water (Ericson Citation1985). This Sr derives from a combination of in situ weathering of bedrock during soil formation and a variable input of Sr from atmospheric sources, namely precipitation and airborne particles (Bentley Citation2006). Plants therefore have 87Sr/86Sr values that tend to reflect their geographic origin. Indeed, the 87Sr/86Sr values of modern plants are quite often used in archaeological mobility studies to define the isotopic signature of the local area (e.g. Brönnimann et al. Citation2018; Willmes et al. Citation2014).
Sr isotope analysis of archaeological crop remains have been few until now, due to concerns about contamination with Sr from the burial environment. A number of studies have now shown that while charred cereal grains do adsorb exogenous Sr from the soil in which they are buried, it is possible to remove at least some of this Sr by leaching (soaking) in acid and thus to determine whether the 87Sr/86Sr value of the residual material overlaps or not with the Sr isotope signature of the local area (Heier, Evans, and Montgomery Citation2009; Benson Citation2012; Bogaard et al. Citation2014; Styring et al. Citation2019). Although there is a possibility that not all of the exogenous Sr contamination is removed during leaching, incomplete removal is only likely to result in an underestimation of whether cereals were grown outside of the local area (Styring et al. Citation2019). The determination of the Sr isotope composition of archaeological cereal grains and the Sr that is removed during leaching them in acid can therefore provide a tentative means of identifying crops unlikely to have been cultivated in the local area. Charring itself has been found to result in a small decrease of 0.0001 in cereal grain 87Sr/86Sr values, although this observation is posited to be a result of inherent variability between different cereal grains or contamination during the charring process rather than fractionation due to the charring process itself (Heier, Evans, and Montgomery Citation2009).
Isotope ratios of Sr in hydroxyapatite, the primary mineral component of tooth enamel, have been shown to be particularly useful signatures for the place of origin of humans and animals. The formation of tooth enamel incorporates nutrients in the water and foods an individual consumed during their childhood and is not subsequently remodeled during life. Thus, the 87Sr/86Sr value of tooth enamel reflects the Sr isotope composition of the local environment where an individual was born and lived as a juvenile. Furthermore, tooth enamel seems not to adsorb exogenous Sr from the soil during burial (Ericson Citation1985; Price, Burton, and Bentley Citation2002; Montgomery Citation2010). In the last 20 years numerous examples of the application of Sr isotope ratios to archaeological questions have been published, which have mainly focused on the mobility of humans (Price et al. Citation1994; Grupe et al. Citation1997; Montgomery, Evans, and Neighbour Citation2003; Price and Gestsdóttir Citation2006; Arcini Citation2018). However, in the last decade several studies have also shown that the isotopic composition of Sr in tooth enamel can be useful to reveal mobility in animals and particularly livestock in the past (Evans et al. Citation2007; Viner et al. Citation2010; Price Citation2013; Sjög
Table 2. Number of samples of plant and faunal remains selected for strontium isotope analysis from each archaeological site.
Thirty samples of charred archaeological cereal grains were selected. These comprised 27 hulled barley (Hordeum vulgare) grain samples and one sample each of bread wheat (Triticum aestivum), rye (Secale cereale) and oat (Avena sativa). Two additional samples of seeds from flax (Linum usitatissimum) and gold-of-pleasure (Camelina sativa) were also included. Each sample comprises 10 grains/seeds from the same archaeological context.
Enamel was sampled from molariform teeth from pigs (Sus domesticus), cattle (Bos taurus) and horse (Equus caballus); 45 samples in total. Because the crown of molariform teeth forms when animals are growing, it represents where the animal was grazing and feeding at a young age. With only a few suitable teeth found in the study area, different molariform teeth were selected. Thus, depending on which teeth were analyzed, their 87Sr/86Sr values reflect different periods of the life history of the animals.
Ten teeth from pigs included first molars (M1) and permanent premolars, which are formed in piglets at 1–4 months and 6–12 months, respectively (Magnell and Carter Citation2007). The 31 teeth from cattle included 2 deciduous premolars and 2 first molars (M1), in which the crown of the tooth is formed in utero. Fourteen samples were from second molars (M2), formed at 6–12 months of age, and 13 third molars and permanent premolars, formed at 12–18 months of age (Brown et al. Citation1960).
To evaluate the local Sr isotopic baseline, 11 samples of enamel from incisors and molars of rodents, i.e. water vole (Arvicola amphibius), black rat (Rattus rattus) and yellow-necked mouse (Apodemus flavicollis), were selected from several archaeological sites located on various bedrock types in Scania. Modern bread wheat (T. aestivum) cereal grains and straw, pinecone (Pinus sylvestris) and hazelnut shell (Corylus avellana) were collected from three locations ( and and and ). For sample details, see Supplementary material 3. Additionally, the 87Sr/86Sr values of material removed from archaeological cereal grains after leaching/soaking in 6 M hydrochloric acid for 24 h (leachates) were used to estimate the local range of 87Sr/86Sr values.
Table 3. 87Sr/86Sr values of archaeological cereal grains and seeds of oil plants (residues and leachates) from Uppåkra and its surrounding sites and the 87Sr/86Sr values of archaeological rodent tooth enamel and modern plant samples from different bedrock types in Scania.
Table 4. 87Sr/86Sr values of archaeological faunal tooth enamel from Uppåkra and the nearby sites of Hjärup and Stanstorp and the 87Sr/86Sr values of archaeological rodent tooth enamel and modern plant samples from various bedrock types in Scania.
4.2. Lab methods
4.2.1. Plants
Each of the charred grain samples, comprising 10 grains/seeds and weighing c. 0.2–0.3 g, was ground to a powder using an agate mortar and pestle. This material was then leached in 10 mL Teflon distilled 6 M hydrochloric acid (HCl) at room temperature overnight, following method 2 of Styring et al. (Citation2019). All samples reacted, producing carbon dioxide gas, suggesting the presence of carbonate. The samples were then centrifuged and the supernatant fluid (leachate) decanted off. The sample was washed twice in de-ionized water, centrifuged and the supernatant water added to the acid leachate. The leachate and washed samples were stored in separate test tubes.
Samples were prepared for Sr isotope analysis following the protocol in Styring et al. (Citation2019): The residual charred material and the modern plant samples were placed in a clean dissolution vessel. Four mL 8 M nitric acid (HNO3) and 1 mL hydrogen peroxide (H2O2) were added and the vessels were placed on a hotplate at 60°C overnight with lids on loosely to allow any early gas produced through reaction with acid to escape. The caps were then screwed down tightly and the samples were microwaved at 170°C for 30 min. When cool, this material was then transferred to Savillex beakers and reduced in volume on a hot place in a HEPA filtered laminar flow hood. Ultrapure H2O2 was added until the samples were a light straw yellow color. This required about 10 mL H2O2 per sample. The charred grain samples, the modern plant samples and approximately half of the leachate solutions were then dried down, converted to chloride using 6 M HCl (Teflon distilled), dried down and taken up in calibrated 2.5 M HCl. Sr was separated from the samples using Eichrom AG50 X8 cation resin.
Strontium was loaded onto a single Re filament following the method of Birck (Citation1986) and the isotopic composition was determined by Thermal Ionisation Mass spectroscopy (TIMS) using a Thermo Triton multi-collector mass spectrometer at NEIF, British Geological Survey. All samples were run using a peak jumping routine of 100 * 8 s integrations. The average total Sr beam size was 5 V. Sr ratios were normalized for instrumental mass discrimination using a fixed 86Sr/88Sr value of 0.1194. The samples were run at a time when the international standard for 87Sr/86Sr, NIST 987, gave 0.710258 ± 0.000016 (2 σ, n = 36) and data are corrected to the accepted value for this standard of 0.710250. Procedural Sr blanks were approximately 70 pg. Procedural reproducibility of plant dissolutions were monitored using NIST 1515 apple leaf standard, which gave a 87Sr/86Sr value of 0.713950 ± 0.00004 (2 σ, n = 18) and a Sr concentration of 25.5 ± 3.9 ppm (2 σ, n = 18).
4.3.2. Fauna
Faunal samples were analyzed at two laboratories, in which different methods were used for preparation and analysis. The samples from Uppåkra were analyzed at the Department of Geological Sciences, University of North Carolina at Chapel Hill, the samples from Hjärup and Stanstorp at NIGL, British Geological Survey.
Laboratory methods at Department of Geological Sciences, University of North Carolina at Chapel Hill (Sjögren, Price, and Ahlström Citation2009; Price Citation2013): Tooth enamel was separated with a drill and pre-cleaned in high purity water. The samples were further pre-cleaned by mechanical abrasion with a Dremel tool fitted with a sanding bit to remove the outer surface and potential contamination. Samples weighing 2–5 mg were then dissolved in 5 M HNO3. The Sr fraction was purified using EiChrom Sr-Spec resin and eluted with HNO3 followed by water.
Isotopic ratios were obtained on the strontium fraction using a VG (Micromass) Sector 54 TIMS in the Department of Geological Sciences, University of North Carolina at Chapel Hill. This is a single focusing, magnetic sector instrument equipped with multiple Faraday collectors. Strontium was placed on single Re filaments and analyzed using a quintuple-collector dynamic mode of data collection. Internal precision for 87Sr/86Sr analyses is typically 0.0006–0.0009% standard error, based on 100 dynamic cycles of data collection, i.e. ±0.000006. Recent analyses of the strontium NIST 987 standard average 0.710260 ± 0.000010 (2 σ, n = 30) and data are corrected to the accepted value for this standard of 0.710250. Sr ratios were normalized for instrumental mass discrimination using a fixed 86Sr/88Sr value of 0.1194. Total procedural blanks for strontium are typically below 100 μg, which is insignificant relative to the amounts of Sr in the samples.
Laboratory methods at NIGL, British Geological Survey: The enamel surface of the tooth was abraded from the surface to a depth of >100 microns using a tungsten carbide dental bur and the removed material discarded. An enamel sample was cut from the tooth using a flexible diamond edged rotary dental saw. All surfaces were mechanically cleaned with a diamond bur to remove adhering dentine. The resulting sample was transferred to a clean (class 100, laminar flow) working area for further preparation. In a clean laboratory, the sample was first cleaned ultrasonically in high purity water to remove dust, rinsed twice, and then soaked for an hour at 60°C, rinsed twice, then dried and weighed into pre-cleaned Teflon beakers. The sample was mixed with 84Sr tracer solution and dissolved in Teflon distilled 8 M HNO3 before being converted to its chloride form using 6 M HCl.
Strontium was collected using Eichrom AG50 X8 resin columns. Strontium was loaded onto a single Re filament following the method of Birck (Citation1986) and the isotope composition was determined by TIMS using a Thermo Triton multi-collector mass spectrometer. Samples ran at about 1350–1450 degrees °C with a beam size of 2–4 Volts. All samples were run in static mode for 100–200 scans and to an internal run precision average of ±0.00012 (1SE). The international standard for 87Sr/86Sr, NBS987, gave a value of 0.710270 ± 0.000006 (2 σ, n = 8) during the analysis and data were corrected to the accepted value of 0.710250.
5. Results
5.1. Establishing a “local” environmental strontium isotope signature
and show the 87Sr/86Sr values of carbonized cereal grains from Uppåkra and its surrounding sites compared to the 87Sr/86Sr values of archaeological rodent tooth enamel and modern plant samples from various bedrock types in Scania. The 87Sr/86Sr values of the material removed from the archaeological grains after leaching in 6 M HCl for 24 h (leachates) range from 0.71005 to 0.71205 and average 87Sr/86Sr = 0.71134 ± 0.00054 (1 σ, n = 15). This range encompasses the 87Sr/86Sr values of archaeological (Iron Age to Medieval period) rodent enamel samples, which range from 0.71013 to 0.71142 and average 0.71101 ± 0.00041 (1 σ, n = 8), and two modern plant samples (87Sr/86Sr = 0.71118 and 0.71123) from areas of limestone bedrock in southwest Scania (see – sampling locations).
Figure 3. 87Sr/86Sr values of archaeological cereal grains (residues and leachates) from Uppåkra and its surrounding sites compared to the 87Sr/86Sr values of archaeological rodent tooth enamel and modern plant samples from various bedrock types in Scania. Shading represents the range in 87Sr/86Sr values of each group.
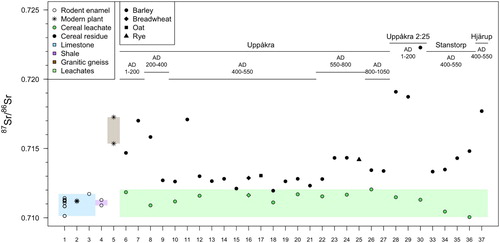
Enamel from an archaeological rodent recovered in an area of limestone bedrock in northeast Scania () has a slightly higher 87Sr/86Sr value of 0.71172 than the values of the rodents and plants from southwest Scania. The 87Sr/86Sr values of enamel from rodents recovered near Lake Ringsjön in central Scania, on an area with shale bedrock, also fall within the range of cereal grain leachate values, whereas the 87Sr/86Sr values of modern plants sampled in central Scania east of Lake Ringsjön, in an area with granitic gneiss bedrock, are much higher (average 0.71631 ± 0.00135, 1 σ, n = 2).
The 87Sr/86Sr values of the leachates are normally distributed (Shapiro–Wilk test: W = 0.89, p = .063), although visual inspection of the data in a normal probability plot (Q–Q plot), which compares the data by quantiles to the expected normal curve, indicates that the two leachates of cereal grains from Stanstorp are slightly lower than the reference line ((a)).
5.2. Identifying locally grown cereals
The 87Sr/86Sr values of the material remaining after leaching the cereal grain samples (i.e. excluding oil seed crops, L. usitatissimum and C. sativa) in 6 M HCl for 24 h (residues) are consistently higher than the 87Sr/86Sr values of the leachates, ranging from 0.71195 to 0.72228 (n = 14) (). The 87Sr/86Sr values of the residues are not normally distributed (Shapiro–Wilk test: W = 0.81, p < .001). Visual inspection of the data in a normal probability plot indicates that there is a positive skew to the data and that seven of the samples clearly fall outside of the normal distribution ((b)). These are samples with 87Sr/86Sr values ≥ 0.71582. Of the archaeological cereal grain samples from Uppåkra identified as falling outside the normal distribution, one is dated to the Early Roman Iron Age, one to the Late Roman Iron Age and one to the Migration Period. All three of the cereal grain samples from Roman Iron Age site Uppåkra 2:25 and the one from Migration period Hjärup also fall outside the normal distribution. The 87Sr/86Sr values of L. usitatissimum and C. sativa seed residues after leaching are notably higher than those of the cereal grains (87Sr/86Sr = 0.72354 and 0.73345, respectively). Both samples of oil seeds were sampled from the same context, a refuse pit at Uppåkra 2:25, from which cereal grains were also taken and whose 87Sr/86Sr value falls outside the normal distribution.
5.3. 87Sr/86Sr values of animals
and show the 87Sr/86Sr values of animal tooth enamel from the regional center Uppåkra and the nearby sites of Hjärup and Stanstorp compared to the 87Sr/86Sr values of archaeological rodent tooth enamel and modern plant samples from various bedrock types in Scania and leachates from archaeological cereal grains from Uppåkra and surrounding sites. The 87Sr/86Sr values of pig tooth enamel range from 0.71052 to 0.71184 and average 87Sr/86Sr = 0.71136 ± 0.00044 (1 σ, n = 10). The 87Sr/86Sr values for pigs from all three settlements are within the range of the local baseline defined by the 87Sr/86Sr values of local rodent teeth and modern plants and cereal grain leachates (). The 87Sr/86Sr values of cattle tooth enamel range from 0.70974 to 0.72406 and average 87Sr/86Sr = 0.71221 ± 0.00259 (1 σ, n = 31). One of the cattle tooth enamel samples from Uppåkra has a lower 87Sr/86Sr value than the range of archaeological cereal grain leachate values, whereas six are higher than the range, i.e. have more radiogenic 87Sr/86Sr values (). This indicates that a relatively large proportion (35%) of the cattle may not have been local, although most 87Sr/86Sr values only differ slightly from the local baseline and thus could be from nearby areas with slightly different bedrock.
Figure 5. 87Sr/86Sr values of archaeological faunal tooth enamel from Uppåkra and the nearby sites of Hjärup and Stanstorp, compared to the 87Sr/86Sr values of archaeological rodent tooth enamel and modern plant samples from various bedrock types in Scania and archaeological cereal grain leachates from Uppåkra and surrounding sites. Shading represents the range in 87Sr/86Sr values of each group defining a local baseline.
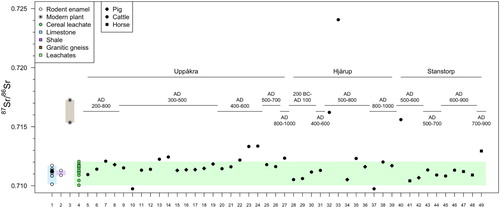
Four cattle tooth enamel samples from Hjärup have 87Sr/86Sr values either lower or higher than the local baseline. From Stanstorp, tooth enamel samples from one of the cattle and one horse have 87Sr/86Sr values inconsistent with the local baseline. Among these samples, two cattle teeth from Hjärup and one from Stanstorp have highly radiogenic values, corresponding to more distant regions with Precambrian bedrock ().
6. Discussion
6.1. Establishing a “local” environmental strontium isotope signature
The 87Sr/86Sr values of archaeological bones (mean ± 2 σ) are sometimes used to provide a baseline range of local 87Sr/86Sr values, based on the assumption that groundwater Sr in the soil exchanges with biogenic Sr in the bone matrix, altering the biogenic Sr signature and even entirely overwriting it with the Sr isotopic composition of the local soil (see Bentley Citation2006). Bone tissue is therefore usually not sampled, but instead tooth enamel, which due to its denser structure and mainly inorganic composition does not absorb Sr from surrounding soil as readily (Balasse Citation2002; Montgomery Citation2010). The same principle regarding bones can be applied to charred cereal grains, since plant remains have also been found to adsorb Sr from the burial environment (Styring et al. Citation2019). In an experimental setup, leaching of buried charred grains in 6 M HCl gave leachates with 87Sr/86Sr values similar to those of buried grains before leaching, which is likely a mix of the 87Sr/86Sr values of a water leach of the soil and the original, unburied grains (Styring et al. Citation2019). If the same is the case here, the 87Sr/86Sr value of the local soil is likely to be slightly lower than the values of the leachates and thus to be in close agreement with the 87Sr/86Sr values of the rodent tooth enamel samples from the area. It can therefore be proposed that the range of mean ± 2 σ of archaeological cereal grain leachate 87Sr/86Sr values (0.71134 ± 0.00108, or 0.71026–0.71242), is consistent with the local Sr isotopic signature. This range in 87Sr/86Sr values also encompasses 87Sr/86Sr values of tooth enamel samples of rodents from southwest Sweden, which range from 0.71013 to 0.71172 (). Since all the values of the samples of rodents and modern plants falls within an expected range in relation to the bedrock of Cretaceous limestone in the region, the established local strontium isotope signature can be considered reliable.
6.2. Mobility of agricultural products
6.2.1. Identifying locally grown cereals
Only three of the archaeological cereal grain residues fall within the range in 87Sr/86Sr values of rodent tooth enamel samples from southwest Scania and the range of mean ± 2 σ of archaeological cereal grain leachates. If we were to define locally grown cereals as only those with 87Sr/86Sr values within this range, then only these three samples would be interpreted as having grown locally. This is surprising, given the suitable agricultural soils in the local area (Ekström Citation1950). It is therefore possible that: (1) the 87Sr/86Sr values of the cereal grain residues are not representative of the Sr value of the original biogenic Sr, or (2) the local range in 87Sr/86Sr values defined by rodent enamel and archaeological cereal grain leachates differs from the Sr isotopic composition of bioavailable Sr taken up by crops in the past.
Leaching of buried charred cereal grains in 6 M HCl has been found to remove some but not all contaminant Sr derived from the soil, and also to remove biogenic Sr (Heier, Evans, and Montgomery Citation2009; Styring et al. Citation2019). It is therefore likely that the 87Sr/86Sr values of the archaeological cereal grain residues represent a mix of contaminant Sr from the soil and residual biogenic Sr from the grains. Since the cereal grain residues all have more radiogenic 87Sr/86Sr values than the material leached out by the acid and the local tooth enamel, any remaining contaminant soil Sr likely has a lower 87Sr/86Sr value than the original biogenic Sr. If some of this contaminant Sr remains in the grain residues after leaching, it means that the 87Sr/86Sr values of the original unburied grains could in fact be even higher than those of the leached residues. If this is the case, none of the grains would fall within the local Sr signature defined by rodent enamel and cereal grain leachates. Biased removal of biogenic Sr with a particular isotopic composition during leaching is unlikely to account for the more radiogenic 87Sr/86Sr values of cereal grains residues, since leaching of uncontaminated charred cereal grains in 6 M HCl was only found to alter their 87Sr/86Sr values by up to ±0.0001 (Heier, Evans, and Montgomery Citation2009; Styring et al. Citation2019).
It is possible, then, that the Sr isotopic composition of the samples used to define the environmental baseline (archaeological rodent enamel, modern plants, and archaeological cereal grain leachates) differs from that of ancient crops grown in the local area. The choice of proxies to define a local bioavailable Sr isotopic signature has been the subject of much debate and has not yet been fully resolved (e.g. Bentley Citation2006; Grimstead, Nugent, and Whipple Citation2017; Maurer et al. Citation2012). A recent study aiming to establish a Sr isotope baseline for southwestern Sweden (north of the study area in this paper) chose to sample surface waters, enamel from small mammals from archaeological contexts and modern snail shells, due to concerns that modern plants could be affected by wind-blown dust and that the Sr isotopic composition of soil is difficult to interpret in terms of bioavailable Sr (Blank et al. Citation2018). Frei and Frei (Citation2011) found that the 87Sr/86Sr values of soil extracts and snail shells were on average 0.001 lower than those of lake and stream waters and sheep hair sampled from the same area on Zealand. Denmark. They tentatively attributed this to wash-out of Sr with an elevated 87Sr/86Sr value from topsoil and preferential uptake of the remaining Sr with a lower 87Sr/86Sr value by small herbivores. Blank et al. (Citation2018), on the other hand, found no significant difference between the 87Sr/86Sr values of surface waters, small mammals and snail shells. Sr studies to date have tended to focus on defining baselines for comparison with human and faunal tooth enamel, which will be an average of consumed food and water sources from multiple areas. However, significant variability in individual plant 87Sr/86Sr values (e.g. Snoeck et al. Citation2020), which will depend upon the soil composition of the particular patch in which a plant is growing, makes it difficult to interpret archaeological crop 87Sr/86Sr values as local or not using conventionally defined isotopic baselines. Future work should focus on further defining this variability (up to 0.0052 between plants growing at a single location in Ireland; Snoeck et al. Citation2020) in order to provide an appropriate local baseline for comparison with archaeological crop remains.
Leaching in 6 M HCl extracts Sr associated with hydrous iron-manganese oxides adsorbed on clays and occurring in carbonates and sulfides (Négrel, Grosbois, and Kloppmann Citation2000). Indeed, the bubbling observed during acid leaching of the archaeological cereal grains in this study testifies to the dissolution of carbonates. This leaching process could therefore have released relatively recalcitrant Sr that would not be bioavailable to plants, suggesting that the leachates could have a different isotopic composition from those of locally growing plants. The fact, however, that the 87Sr/86Sr values of rodent tooth enamel from the local area and two modern plant samples growing close to the site overlap with the leachate 87Sr/86Sr values suggests that dissolution of non-bioavailable Sr does not result in a notable difference in the Sr isotopic composition. It cannot be excluded that collection of more environmental baseline samples, including water and more modern plants, might help to determine whether the range in 87Sr/86Sr values of the Iron Age fields for crops is in fact wider than that of the cereal grain leachates and rodent enamel, which are all from the settlements themselves and therefore represent a very restricted area.
An alternative method of identifying non-locally grown cereals, without relying on an independently defined local Sr isotope signature, could be to treat those cereal grain residues whose 87Sr/86Sr values fall outside of the normal distribution as having grown outside of the local area. Wright (Citation2005) used a similar statistical method for human individuals from Tikal, Guatemala, first excluding 87Sr/86Sr values that were clear outliers and then identifying samples that differed from the normal distribution using a normal probability plot. (b) is a normal probability plot of the 87Sr/86Sr values of cereal grain residues in this study and shows that seven of the cereal grain residues fall outside of the normal distribution and could therefore be interpreted as having grown outside of the local area.
In regional center Uppåkra, these potentially non-locally grown cereals were recovered from a house, from contexts dating to the Early Roman Iron Age and Migration Period, and from a hall building, dating to the Late Roman Iron Age. These samples have 87Sr/86Sr values ranging from 0.71583 to 0.71709. 87Sr/86Sr values around 0.7160 have been established for samples from the central parts of Scania located on granitic gneiss, some 40–60 km northeast of Uppåkra and the grain could possibly originate from this area. One cereal grain sample from the more ordinary settlement of Hjärup, dating to the Migration Period, could also originate from Central Scania, although its 87Sr/86Sr value is slightly higher at 0.71770. All the cereal grain samples analyzed from the more ordinary settlement of Uppåkra 2:25, dating to the Early Roman Iron Age, were potentially non-local, ranging from 0.71873 to 0.72228. The closest region where similar values have been recorded are in the neighboring provinces of Blekinge and Småland about 120–250 km northeast of the Uppåkra region (Glykou et al. Citation2018). There is also the Romeleåsen ridge, c. 15 km southeast of the archaeological sites, which has similar bedrock and could therefore support plants with higher 87Sr/86Sr values as well.
6.2.2. Mobility of livestock
A relatively large proportion (35%) of the cattle have 87Sr/86Sr values that fall outside the range of the local baseline and therefore seem to have been brought to the Uppåkra area prior to slaughter. This is in accordance with results based on kill-off patterns of cattle from Uppåkra, which show a larger proportion of fully-grown animals (2.5–4 years) and adults over 4 years than would be expected for a locally-raised population, indicating the import of slaughter animals to the regional center (Magnell, Boethius, and Thilderqvist Citation2013). Seven of the cattle from Uppåkra, one from Hjärup and one horse from Stanstorp have 87Sr/86Sr values, ranging from 0.71218 to 0.71336, which are only slightly more radiogenic than the local baseline. These animals could originate from areas with slightly older bedrock than that found in the Uppåkra area. It is difficult to pin-point with certainty from which area these animals originate. Possibly these cattle and horses were raised on pastures north of the city of Lund, where the bedrock of Jurassic and Triassic shale differs from the Tertiary limestone in the Uppåkra area (SGU Ser Ba nr 40). Corresponding 87Sr/86Sr values (0.71120–0.7216) of tooth enamel have been measured from pigs from Rya in northwest Scania, an area with similar bedrock to the area north of Lund. This area is only about 5 km from Uppåkra and the settlement’s main pastures could have been situated in this area.
Two cattle teeth, from Uppåkra and Hjärup, respectively, have slightly lower values (0.70974) than the local baseline. Similar values have been established for Zealand in Denmark and could represent imports of cattle over the Öresund strait (Frei and Price Citation2012). Another possibility is that cattle were grazing on meadows by the sea just some 4–6 km west of the settlements, and that the lower 87Sr/86Sr values could result from sea spray, since seawater has a 87Sr/86Sr value of 0.7092 (Whipkey et al. Citation2000; Snoeck Citation2014). Two cattle from Hjärup and one from Stanstorp have 87Sr/86Sr values (0.71621–0.72406) that clearly differ from the local baseline and indicate the possibility of imports of livestock from areas with older bedrock. Possibly cattle were herded from central parts of Scania about 40–60 km from Uppåkra, where 87Sr/86Sr values are around 0.7160. 87Sr/86Sr values as high as 0.7241 have only been found in the regions of Västergötland and Östergötland, over 300 km north of the study area (Arcini Citation2018). However, we have few baseline samples from northern Scania and the neighboring province of Småland, and it is possible that the cattle originate from this area.
All the tooth enamel samples from pigs from the settlements Uppåkra, Hjärup and Stanstorp have 87Sr/86Sr values within the range of rodent enamel samples from the local study area. This indicates that pigs were not moved over any great distance, but were kept close to these settlements. Thus, pigs do not seem to have been included in any exchange system involving imports of grain and cattle herded to the Uppåkra area. It therefore seems possible, at least for the Iron Age in Scandinavia, to use the 87Sr/86Sr values of tooth enamel from pigs as an approximation for the local Sr isotope signature at a site.
6.2.3. Implications for long-distance movement of livestock and grain
This Sr isotope study of cereal grain and faunal remains shows that some grain and faunal products found in the study area around Uppåkra are of non-local origin. A third of the fauna analyzed exhibited non-local 87Sr/86Sr values and for now, we assume that those cereal grain samples that fall outside the normal 87Sr/86Sr value distribution (c. 20% of the total) were non-local to the sites. To address these results we need to consider both practical aspects of farming and its preconditions in the study area, and how social interactions within an Iron Age society may have played a role in the movement of agricultural products. Concerning the 87Sr/86Sr values of cereal grains, we additionally need to consider methodological aspects (see above discussion).
The investigated sites are situated in an area of southwestern Scania with mostly fertile soils, providing ideal conditions for arable production. This would have given farmers the possibility to produce crops with high yields, likely providing sufficient grain supplies to meet the local demand for staple grain. These circumstances differ from parts of Scania with older bedrock and poorer soils, where arable production was lower and agriculture was more focused on animal herding.
A recently published compilation and modeling of pollen data shows that the fertile soils of southwestern Scania during the Iron Age were characterized by a very open, almost treeless landscape, and that arable land covered c. 20–35% of the land area (Lagerås and Fredh Citation2019). Another recent study determined nitrogen isotope values of archaeological cereal grains recovered from Uppåkra and six nearby sites (Larsson, Bergman, and Lagerås Citation2019). Larsson, Bergman, and Lagerås (Citation2019) found that the grains had high nitrogen isotope values and attributed these to systematic addition of manure to arable fields during most of the first millennium AD. These studies both point to intensive crop production in the area, and for that reason, it seems unlikely that farmers in the investigated area had the need to import large quantities of grain from regions outside. Large areas around the settlements were potentially used for crop production, leaving fewer areas for pastures. Smaller livestock, such as pigs, and some of the cattle stock were likely kept in the area around the settlement. About a third of the cattle in this study may have been included in transhumance systems, where distant pastures were located either by the sea or in areas north of Uppåkra.
Culture and traditions may on the other hand have influenced agricultural aspects of social life and the mobility of agricultural products. For example, in the Germania, Tacitus writes that it was customary for settlements and individual households to make voluntary contributions of cattle and crops to their leaders (Tacitus, Germania 15). A payment in agricultural produce or other valuables was accepted by the leaders as a token of honor, and protection or security to farmers may have been given in return. A similar system may have been in place around the regional center of Uppåkra, and if so, it could explain some of the non-local produce found in the archaeological record. According to later Old Norse sources, rulers of the Late Iron Age society in Scandinavia had a central role as leaders for cult and communal gatherings, which included sacrifices and feasting, called blót. These ritual feasts had significant social importance in consolidating social structures and it seems that the people were obliged to give gifts, such as animals for slaughter and barley to produce beer, to the leading aristocracy at the ritual feast (Sundqvist Citation2000). Uppåkra has been assumed to have been a religious center based on finds of a ceremonial building, ritual objects and evidence of feasting (Helgesson Citation2002; Larsson Citation2011; Magnell, Boethius, and Thilderqvist Citation2013). The ceremonial building at Uppåkra was rebuilt several times according to the same ground plan and stood on the same place from the Roman Iron Age until the Viking Period (Larsson and Lenntorp Citation2004). One possibility is therefore that imported grains and cattle to the regional center could have been tributes to ritual feasts and the rulers of Uppåkra. Moreover, large-scale production of malt used for beer-making has previously been identified in an area within the regional center, and this further underlines the likelihood of activities that may be linked to feasting and/or trade (Larsson, Svensson, and Apel Citation2018).
Another aspect of social life may have involved exchange of agricultural products. Previous studies investigating kill-off patterns of cattle and metric analyses of barley grains at Uppåkra and settlements in its surrounding area indicate the possibility of exchange of agricultural products (Magnell, Boethius, and Thilderqvist Citation2013; Larsson Citation2018). That non-local cattle and grains were also found at the settlements of Uppåkra 2:25, Hjärup and Stanstorp, in the surroundings of the regional center, indicates that these settlements were part of an exchange system of agricultural products with other regions as well. Alternatively, Hjärup and Stanstorp, which were relatively large, prosperous settlements, accessed agricultural products from the nearby regional center, possibly via exchange or redistribution within the farming society.
If we consider the chronology of the indicated non-local 87Sr/86Sr values, we see that the cereal grain residues that fall outside of the normal distribution are more frequent (five of the seven 87Sr/86Sr values) in the early periods (c. AD 0–400), compared to later periods (c. AD 400–1050; 2 of the 23 87Sr/86Sr values). Changes in agricultural practices over time are indicated by a number of archaeobotanical investigations in the study area. Macrofossil records show that crop production changed at different times (Larsson Citation2015). Although hulled barley was the principle crop in the first millennium AD, in the early periods (c. AD 0–400), cereal cultivation appears to have been more diverse, including wheats, naked barley and broomcorn millet, and different settlements or farmsteads in the environs of Uppåkra had specialized cereal cultivation. In later periods (c. AD 600–1050) rye gradually became a staple crop along with barley, reducing the economic importance of other cereals. Investigations of manuring intensity in the study area, based on nitrogen isotope analysis of ancient barley grains, show that manuring inputs in crop cultivation varied during the initial period (AD 0–200), reflecting mixed manuring regimes, but were generally high during the later periods (AD 200–1000), indicating systematic input of manure (Larsson Citation2019). Such changes in cultivation practice could also have involved a change in the kinds of soils that were cultivated: glaciofluvial sediments with sand on older bedrock could have been used in the early periods and later, there may have been more systematic cultivation on clay. This could account for the lower variability of cereal grain 87Sr/86Sr values in later periods.
The 87Sr/86Sr values of cattle tooth enamel indicate an opposite trend for imports of livestock. There are only three samples dating to the Pre-Roman/Roman Iron Age (500 BC–AD 400), but all have 87Sr/86Sr values corresponding to the local Sr isotopic baseline, providing no evidence for imports of livestock during this time. From the Late Iron Age (AD 400–1050), 13 of the 28 cattle seem to originate from areas with different bedrock to that found in the Uppåkra area.
Identifying long-distance movement of livestock and grain in the study area, we also need to consider the archaeological contexts that were sampled. At Uppåkra, archaeological excavations have over the years largely focused on the central area of the site. This central area has revealed hall-buildings, a ceremonial house, grain storage and areas for ritual activities, and along with an abundance of high-status artifacts, it has been suggested that this area of the site was associated with the social elite, but functioned also as a place of communal use (Larsson and Söderberg Citation2012; Magnell, Boethius, and Thilderqvist Citation2013; Larsson Citation2019). Because very few “ordinary” households on the site have been excavated and analyzed, we consider samples used in this study to be from archaeological contexts belonging to the aristocracy and ritual areas. Concerning the surrounding sites, these are smaller than the regional center, but are still large compared to other contemporary settlements in the region, comprising numerous farmsteads. The sites of Stanstorp and Hjärup also appear to have been wealthy. Thus, the central position of economic and political power at Uppåkra probably also provided economic advantages to surrounding settlements, which according to this study, likely involving access to non-local produce.
7. Conclusion
This study is one of the first to examine the Sr isotope composition of both crop and animal remains recovered on archaeological sites to obtain a more holistic insight into the movement of agricultural products in the past. The novel approach of determining the 87Sr/86Sr values of archaeological crop remains is nonetheless not without its challenges. Sampling of material to assess biogenic Sr isotope signatures of locally grown crops needs to be carefully considered, since unlike animals and humans, plant 87Sr/86Sr values are not an average of the isotopic composition of Sr consumed over a [variably] wide area. In this study we therefore use the normal distribution of archaeological cereal grain 87Sr/86Sr values to identify likely non-locally cultivated crops, but the validity of this approach needs to be further assessed. Some uncertainties also remain regarding the extent to which the 87Sr/86Sr value of leached cereal grains reflects the isotopic composition of the original biogenic Sr prior to burial and thus how these 87Sr/86Sr values can be interpreted in terms of locally versus non-locally sourced crops.
The results from the strontium isotope analysis has found that some cereal grain samples have particularly high 87Sr/86Sr values, ≥0.71582, which fall outside the normal distribution and are therefore highly likely to have grown outside the local area. These 87Sr/86Sr values are more radiogenic than the local baseline and are similar to those of some cattle and a horse from the same sites, suggesting that some cereals and livestock were imported to Uppåkra and its surrounding settlements from more distant areas on granitic bedrock, likely to the north of the site. This builds on existing knowledge of Uppåkra as a center with extensive trade relations, by showing that crops and livestock were also an integral part of its social networks. This study has therefore contributed to widening our understanding of social interactions within Scandinavian Iron Age society in terms of the movement of agricultural products. What occasions or traditions were involved in this movement between farming communities is difficult to say, but it is possible that livestock and grain were brought to large communal feasting events as voluntary contributions to leaders, or played a role in trade and exchange relations.
Supplemental Material
Download Zip (31.5 KB)Disclosure statement
No potential conflict of interest was reported by the author(s).
Notes on contributors
Mikael Larsson is a researcher at the Department of Archaeology and Ancient History at Lund University where he specializes in archaeobotany. His research has focused on prehistoric plant use in Scandinavia and has aimed to understand agricultural aspects of social life with attention to the handling and distribution of food and other agricultural plant product. He completed his Ph.D. in Archaeology at Lund University (2015), previous academic achievements are from Simon Fraser University, Vancouver.
Ola Magnell is a zooarchaeologist and researcher at the National Historical Museum, Lund. He holds a Ph.D. in Osteology from the Department of Archaeology and Ancient History, Lund University (2006). His research has centered on various topics of human and animal relations ranging from Mesolithic hunting to Iron Age animal husbandry and ritual practices.
Amy Styring is an Associate Professor of Archaeological Science in the School of Archaeology at the University of Oxford. As an archaeological chemist, her interest is in advancing scientific methods that reveal a direct and detailed picture of everyday life in the past, particularly in relation to food production and consumption practices and associated land use change. She obtained her Ph.D. in Chemistry from the University of Bristol (2012), applying nitrogen isotopic analysis of amino acids as a novel approach for the reconstruction of past diet and agricultural practices.
Per Lagerås is a paleoecologist and Associate Professor at the National Historical Museum, Lund. He obtained his Ph.D. in Geoscience and Environmental studies at the Department of Geology, Lund University (1996), applying palynological data to investigate long-term vegetation and land-use in the Holocene. His research has recently focused on periods of decline and abandonment in prehistoric and historic times using palaecological analyses combined with archaeological data.
Jane Evans is a research scientist at the National Environmental Isotope Facility, British Geological Survey, where she is the Head of Science Based Archaeology. She is a geologist by training (Ph.D. 1990) but now focuses on the application of isotopes to archaeological problems such as migration and provenance. She holds Honorary Professorships in Archaeology at Nottingham and Leicester Universities.
Additional information
Funding
References
- Arcini, C. 2018. The Viking Age. A Time of Many Faces. Oxford: Oxbow Books.
- Balasse, M. 2002. “Reconstructing Dietary and Environmental History From Enamel Isotopic Analysis: Time Resolution of Intra-Tooth Sequential Sampling.” International Journal of Osteoarchaeology 12 (3): 155–165.
- Benson, L. V. 2012. “Development and Application of Methods Used to Source Prehistoric Southwestern Maize: A Review.” Journal of Archaeological Science 39: 791–807.
- Bentley, R. A. 2006. “Strontium Isotopes from the Earth to the Archaeological Skeleton: A Review.” Journal of Archaeological Method and Theory 13: 135–187.
- Birck, J. L. 1986. “Precision K-Rb-Sr Isotopic Analysis: Application to Rb-Sr Chronology.” Chemical Geology 56: 73–83.
- Blank, M., K.-G. Sjögren, C. Knipper, K. M. Frei, and J. Storå. 2018. “Isotope Values of the Bioavailable Strontium in Inland Southwestern Sweden—A Baseline for Mobility Studies.” PLoS One 13 (10): e0204649. doi:10.1371/journal.pone.0204649.
- Bogaard, A., E. Henton, J. Evans, K. C. Twiss, M. P. Charles, P. Vaiglova, and N. Russell. 2014. “Locating Land use at Neolithic Çatalhöyük, Turkey: the Implications of 87Sr/86Sr Signatures in Plants and Sheep Tooth Sequences.” Archaeometry 56: 860–877.
- Bolander, A., and B. Söderberg. 2019. Hjärup 7:1 och 22:1. Järnåldersboplats och senneolitiskt gravfält – del 1. Arkeologisk undersökning 2017. Del 1 – Bakgrund, genomförande, tolkning och utvärdering. Skåne län, Skåne, Staffanstorps kommun, Uppåkra socken, Hjärup 7:1 och 22:1, Uppåkra 37. Arkeologerna, Statens historiska museum Rapport 2019:33.
- Brönnimann, D., C. Knipper, S. Pichler, B. Röder, H. Rissanen, B. Stopp, Martin Rosner, et al. 2018. “The Lay of Land: Strontium Isotope Variability in the Dietary Catchment of the Late Iron Age Proto-Urban Settlement of Basel-Gasfabrik, Switzerland.” Journal of Archaeological Science: Reports 17: 279–292.
- Brown, W. A. B., P. V. Christofferson, M. Massler, and M. B. Weiss. 1960. “Postnatal Tooth Development in Cattle.” American Journal of Veterinary Research 21: 7–34.
- Daniel, E., K. Malmberg Persson, and M. Persson. 2000. “Quaternary Deposits of Skåne, Scale 1:250 000.” Sveriges geologiska undersökning. SGU serie Ba, 0373–2657; 55 Ba 55; 2000.
- Ekström, A. 1950. “Skånes åkerjordsområden.” Socker, Handlingar 6 (3): 53–61.
- Ericson, J. E. 1985. “Strontium Isotope Characterization in the Study of Prehistoric Human Ecology.” Journal of Human Evolution 14: 503–514. doi:10.1016/S0047-2484(85)80029-4.
- Evans, J., M. Parker Pearson, R. Madgwick, H. Sloane, and U. Albarella. 2019. “Strontium and Oxygen Isotope Evidence for the Origin and Movement of Cattle at Late Neolithic Durrington Walls, UK.” Archaeological and Anthropological Sciences 11: 5181–5197. doi:10.1007/s12520-019-00849-w.
- Evans, J., S. Tatham, S. Chenery, and C. Chenery. 2007. “Anglo-Saxon Animal Husbandry Techniques Revealed Through Isotope and Chemical Variations in Cattle Teeth.” Applied Geochem 22 (9): 1994–2005.
- Frei, K. M., and R. Frei. 2011. “The Geographic Distribution of Strontium Isotopes in Danish Surface Waters—A Base for Provenance Studies in Archaeology, Hydrology and Agriculture.” Applied Geochemistry 26: 326–340.
- Frei, K. M., and T. D. Price. 2012. “Strontium Isotopes and Human Mobility in Prehistoric Denmark.” Journal of Anthropological Archaeology of Science 4: 103–114.
- Glykou, A., G. Eriksson, J. Storå, M. Schmitt, E. Kooijman, and K. Linén. 2018. “Intra- and Inter-Tooth Variation in Strontium Isotope Ratios from Prehistoric Seals by Laser Ablation Multi-Collector Inductively Coupled Plasma Mass Spectrometry.” Rapid Communication in Mass Spectrometry 32: 1215–1224.
- Grimstead, N. D., S. Nugent, and J. Whipple. 2017. “Why a Standardization of Strontium Isotope Baseline Environment Data Is Needed and Recommendations for Methodology.” Advances in Archaeological Practices 5 (2): 184–195. DOI: 10.1017/aap.2017.6.
- Grupe, G., T. D. Price, P. Schröter, F. Söllner, C. M. Johnson, and B. L. Beard. 1997. “Mobility of Bell Beaker People Revealed by Strontium Isotope Ratios of Tooth and Bone. A Study of Southern Bavarian Skeletal Remains.” Applied Geochemistry 12: 517–525.
- Hårdh, B. 2001. “Produktion och Spridning. Näbbfibulor i Skåne.” In Uppåkra – Centrum och Sammanhang, edited by B. Hård. Uppåkrastudier 3. Acta Archaeologica Lundensia, Series in 80, No. 34, 187–204.
- Hårdh, B. 2002. “Uppåkra in the Migration and Merovingian Periods.” In Central Places in the Migration and Merovingian Periods, edited by B. Hårdh and L. Larsson. Uppåkrastudier 6. Acta Archaeologica Lundensia Series in 8o, No. 39, 41–54.
- Hårdh, B. 2010. “Viking Age Uppåkra.” In Från Romartida Skalpeller Till Senvikingatida Urnesspännen, edited by B. Hårdh. Uppåkrastudier 11. Acta Archaeologica Lundensia, Series in 8°, No. 61, 247–316.
- Hedeager, L. 2002. “Scandinavian ‘Central Places’ in a Cosmological Setting.” In Central Places in the Migration and Merovingian Periods, edited by B. Hårdh and L. Larsson. Uppåkrastudier 6. Acta Archaeologica Lundensia, Series in 8°, No. 39, 3–18.
- Heier, A., J. A. Evans, and J. Montgomery. 2009. “The Potential of Carbonized Grain to Preserve Biogenic 87Sr/86Sr Signatures Within the Burial Environment.” Archaeometry 51: 277–291.
- Helgesson, B. 2002. “Uppåkra in the 5th to 7th Centuries. The Transformation of a Central Place and Its Hinterland.” In Central Places in the Migration and the Merovingian Periods, edited by B. Hårdh and L. Larsson. Uppåkrastudier 6. Acta Archaeologica Lundensia, Series in 80, No. 39.
- Hillman, G. 1984. “Interpretation of Archaeological Plant Remains: The Application of Ethnographic Models From Turkey.” In Plants and Ancient Man – Studies in Paleoethnobotany, edited by W. Van Zeist and W. A. Casparie, 1–41. Rotterdam: A.A. Balkema.
- Jones, M. 1985. “Archaeobotany Beyond Subsistence Reconstruction.” In Beyond Domestication in Prehistoric Europe, edited by G. Barker and C. Gamble, 107–128. London: Academic Press.
- Jørgensen, L. 2009. “Pre-Christian Cult at Aristocratic Residences and Settlement Complexes in Southern Scandinavia in the 3rd–10th Centuries AD.” In Glaube, Kult und Herrschaft. Phänomene des Religiösen im 1. Jahrtausend n. Chr. in Mittel- und Nordeuropa. Akten des 59. Internationalen Sachsensymposions und der Grundprobleme der frühgeschichtlichen Entwicklung im Mitteldonauraum herausgeben, edited by von U. Freeden, H. Friesinger, and E. Warmers, 329–354. Bonn: Dr. Rudolf Habelt.
- Kjällquist, M., and D. Price. 2019. “Mesolithic Mobility and Social Contact Networks in South Scandinavia Around 7000 BCE: Lithic Raw Materials and Isotopic Proveniencing of Human Remains from Norje Sunnansund, Sweden.” Journal of Anthropological Archaeology 53: 186–201.
- Lagerås, P., and D. E. Fredh. 2019. “Long-term Development of Landscape Openness and Arable Land use in an Agricultural Region of Southern Sweden: The Potential of REVEALS Estimates Using Pollen Records from Wells.” Vegetation History and Archaeobotany. doi:10.1007/s00334-019-00756-z.
- Larsson, L. 2011. “A Ceremonial Building as a ‘Home of the Gods’? Central Buildings in the Central Place of Uppåkra.” In The Gudme/Gudhem Phenomenon. Schriften des Archäologischen Landesmuseum Band 6, edited by O. Grimm and A. Pesch. Neumünster, 189–206.
- Larsson, M. 2015. “Agrarian Plant Economy at Uppåkra and the Surrounding Area: Archaeobotanical Studies of an Iron Age Regional Center.” Acta Archaeol Lundensia. Series in 4°, 33.
- Larsson, M. 2018. “Barley Grain at Uppåkra, Sweden: Evidence for Selection in the Iron Age.” Vegetation History and Archaeobotany 27: 419–435.
- Larsson, L. 2019. “Uppåkra. A Central Site in Southern Scandinavia Iron Age: Stability and Change Through More Than a Millennium.” Acta Archaeologica 90: 13–42. doi:10.1111/j.1600-0390.2019.12211.x.
- Larsson, M., J. Bergman, and P. Lagerås. 2019. “Manuring Practices in the First Millennium AD in Southern Sweden Inferred from Isotopic Analysis of Crop Remains.” PLoS One 14 (4): e0215578. doi:10.1371/journal.pone.0215578.
- Larsson, M., and D. Ingemark. 2015. “Roman Horticulture Beyond the Frontier: Garden Cultivation at Iron Age Uppåkra (Sweden).” Journal of Roman Archaeology 28: 393–402.
- Larsson, L., and K.-M. Lenntorp. 2004. “The Enigmatic House.” In Continuity for Centuries. A Ceremonial Building and its Context at Uppåkra, Southern Sweden, edited by L. Larsson. Uppåkrastudier 10. Stockholm, 3–48.
- Larsson, L., and B. Söderberg. 2012. “Bland Mordbrännare och Flygande Smeder: en Sekvens av Hallbyggnader i Uppåkra.” Ale 4: 1–15.
- Larsson, M., A. Svensson, and J. Apel. 2018. “Botanical Evidence of Malt for Beer in Iron Age Uppåkra, Sweden.” Journal of Archaeological and Anthropological Science 11 (5): 1961–1972. doi:10.1007/s12520-018-0642-6.
- Lindberg, S., and K. Schmidt Sabo. 2019. Bytomt och boplats i Fjelie, inför ombyggnad av väg E6.02. Arkeologisk undersökning 2016, Skåne län, Skåne, Lomma kommun, Fjelie socken, fastighet Fjelie 15:1 (9, 10), fornlämning Fjelie 13:1, Fjelie 45:1 och Fjelie 55. Arkeologerna, Statens historiska museum Rapport 2019:10.
- Lindell, M. 2001. “Utgrävningar i Uppåkra – en översiktlig Beskrivning av de Inledande Utgrävningarna Utförda 1996–1998.” In Uppåkra – Centrum i Analys och Rapport, edited by L. Larsson. Uppåkrastudier 4. Acta Archaeologica Lundensia, Series in 8°, No. 28, Lund, 3–22.
- Magnell, O., A. Boethius, and J. Thilderqvist. 2013. “Fest i Uppåkra. En Studie av Konsumtion och Djurhållning Baserad på Djurben Från Ceremonihus och Vapendeposition.” In Folk, fä och Fynd, edited by B. Hårdh and L. Larsson. Uppåkrastudier 12. Acta Archaeologica Lundensia, Series in 8°, No. 64, 85–132.
- Magnell, O., and R. Carter. 2007. “Age Estimation of Wild Boar (Sus scrofa) Based on Molariform Mandibular Tooth Development and Its Application to Seasonality at the Mesolithic Site of Ringkloster.” In Pigs and Humans. 10,000 Years of Interaction, edited by U. Albarella, K. Dobney, A. Ervynck, and P. Rowley-Conwy, 197–217. Oxford: Oxford University Press.
- Maurer, A.-F., S. Galer, C. Knipper, L. Beierlein, E. V. Nunn, D. Peters, Thomas Tütken, Kurt W. Alt, and Bernd R. Schöne. 2012. “Bioavailable 87Sr/86Sr in Different Environmental Samples – Effects of Anthropogenic Contamination and Implications for Isoscapes in Past Migration Studies.” Science of the Total Environment 443: 216–229.
- Montgomery, J. 2010. “Passports From the Past. Investigating Human Dispersals Using Strontium Isotope Analysis of Tooth Enamel.” Annals of Human Biology 37: 325–346. doi:10.3109/03014461003649297.
- Montgomery, J., J. Evans, and T. Neighbour. 2003. “Sr Isotope Evidence for Population Movement Within the Hebridean Norse Community NW Scotland.” Journal of the Geological Society 160: 649–653.
- Négrel, P., C. Grosbois, and W. Kloppmann. 2000. “The Labile Fraction of Suspended Matter in the Loire River (France): Multi-Element Chemistry and Isotopic (Rb–Sr and C–O) Systematics.” Chemical Geology 16: 271–285.
- Price, T. D. 2013. “Human Mobility at Uppåkra. A Preliminary Report on Isotopic Proveniencing.” In Folk, fä och Fynd, edited by B. Hårdh and L. Larsson. Uppåkrastudier 12. Acta Archaeologica Lundensia. Series in 80, No. 64, 163–176.
- Price, T. D., J. H. Burton, and R. Bentley. 2002. “Characterization of Biologically Available Strontium Isotope Ratios for the Study of Prehistoric Migration.” Archaeometry 44: 117–135.
- Price, T. D., and H. Gestsdóttir. 2006. “The First Settlers of Iceland. An Isotopic Approach to Colonization.” Antiquity 80: 130–144.
- Price, T. D., C. M. Johnson, J. A. Ezzo, J. H. Burton, and J. A. Ericson. 1994. “Residential Mobility in the Prehistoric Southwest United States. A Preliminary Study Using Strontium Isotope Analysis.” Journal of Archaeological Science 24: 315–330.
- Sjögren, K.-G., and T. D. Price. 2013. “A Complex Neolithic Economy. Isotope Evidence for the Circulation of Cattle and Sheep in the TRB of Western Sweden.” Journal of Archaeological Science 40: 690–704.
- Sjögren, K.-G., T. D. Price, and T. Ahlström. 2009. “Megaliths and Mobility in South-Western Sweden. Investigating Relationships Between a Local Society and Its Neighbors Using Strontium Isotopes.” Journal of Anthropological Archaeology 28: 85–101.
- Snoeck, C. 2014. “Impact of Strontium sea Spray Effect on the Isotopic Ratio (87Sr/86Sr) of Plants in Coastal Ireland.” Quaternary Newsletter 134: 37–39.
- Snoeck, C., S. Ryan, J. Pouncett, M. Pellegrini, P. Claeys, A. Wainwright, N. Mattielli, J. A. Lee-Thorp, and R. Schulting. 2020. “Towards a Biologically Available Strontium Isotope Baseline for Ireland.” Science of the Total Environment 712: 136248.
- Söderberg, B. 2018. Stanstorp 5:1 och 6:1. Boplats och offerplats från yngre järnålder. Skåne, Staffanstorps kommun, Nevishögs socken, Stanstorp 5:1 och 6:1, Nevishög 41. Arkeologisk undersökning 2016. Arkeologerna, Statens historiska museum Rapport 2018:137.
- Styring, A. K., J. A. Evans, E. K. Nitsch, J. A. Lee-Thorp, and A. Bogaard. 2019. “Revisiting the Potential of Carbonized Grain to Preserve Biogenic 87Sr/86Sr Signatures Within the Burial Environment.” Archaeometry 61: 179–193.
- Sundqvist, O. 2000. An Arena for Higher Powers. Ceremonial Builidings and Religious Strategies for Ruleship in Late Iron Age Scandinavia. Leiden: Koninklije Brill.
- Van der Veen, M. 2007. “Formation Processes of Desiccated and Carbonized Plant Remains – The Identification of Routine Practice.” Journal of Archaeological Science 34: 968–990.
- Viner, S., J. Evans, U. Albarella, and M. Parker Pearson. 2010. “Cattle Mobility in Prehistoric Britain: Strontium Isotope Analysis of Cattle Teeth From Durrington Walls (Wiltshire, Britain).” Journal of Archaeological Science 37 (11): 2812–2820.
- Whipkey, C. E., R. C. Capo, O. A. Chadwick, and B. W. Stewart. 2000. “The Importance of Sea Spray to the Cation Budget of a Coastal Hawaiian Soil: A Strontium Isotope Approach.” Chemical Geology 168: 37–48.
- Wikman, H., and J. Bergström. 1987. “Beskrivning till provisoriska översiktliga berggrundskartan Malmö.” Sveriges Geologiska Undersökning, Serie Ba 40.
- Willmes, M., L. McMorrow, L. Kinsley, R. Armstrong, M. Aubert, S. Eggins, C. Falguères, B. Maureille, and I. Moffat. 2014. “The IRHUM (Isotopic Reconstruction of Human Migration) Database–Bioavailable Strontium Isotope Ratios for Geochemical Fingerprinting in France Earth Syst.” Scientific Data 6: 117–122. www.earth-syst-sci-data.net/6/117/2014/.
- Wright, L. E. 2005. “Identifying Immigrants to Tikal, Guatemala: Defining Local Variability in Strontium Isotope Ratios of Human Tooth Enamel.” Journal of Archaeological Science 32: 555–566. doi:10.1016/j.jas.2004.11.011.