ABSTRACT
Trehalose is a disaccharide formed from two glucose molecules. This sugar molecule can be isolated from a range of organisms including bacteria, fungi, plants and invertebrates. Trehalose has a variety of functions including a role as an energy storage molecule, a structural component of glycolipids and plays a role in the virulence of some microorganisms. There are many metabolic pathways that control the biosynthesis and degradation of trehalose in different organisms. The enzyme trehalase forms part of a pathway that converts trehalose into glucose. In this study we set out to investigate whether trehalase plays a role in both stress adaptation and virulence of Burkholderia pseudomallei. We show that a trehalase deletion mutant (treA) had increased tolerance to thermal stress and produced less biofilm than the wild type B. pseudomallei K96243 strain. We also show that the ΔtreA mutant has reduced ability to survive in macrophages and that it is attenuated in both Galleria mellonella (wax moth larvae) and a mouse infection model. This is the first report that trehalase is important for bacterial virulence.
Introduction
Trehalose is a disaccharide, composed from two glucose molecules, which can be found in many species of bacteria, fungi, plants and invertebrates.Citation1 It is a very stable molecule, typically requiring the presence of a trehalase enzyme for degradation into glucose. The properties of this molecule make it an atypical sugar with many varied functions and the stability of trehalose maybe central to many of its unique biological properties. Trehalose was first proposed to function as an energy storage molecule. This is certainly true in many insects where trehalose provides an easily mobilisable energy reserve, which is consumed during flight.Citation2 It is also thought that trehalose provides an energy reserve in fungal spores while spore germination is accompanied by trehalose hydrolysis.Citation3,4 In Mycobacteria and Corynebacteria, trehalose plays a role as a structural component of many cell wall glycolipids. In Mycobacterium tuberculosis the cell wall “cord factor” (trehalose 6,6-dimycolate) provides both impermeability to drugs and protection against phagocyte killing.Citation1,5 Additionally, high levels of trehalose are found in organisms that can survive desiccation. In this situation the trehalose serves to stabilize macromolecules and especially proteins and membrane lipids in the cell. By forming a glass-like structure around these molecules they are protected from damage caused by dehydration and oxidation.Citation1,6 The ability of trehalose to stabilize biological molecules is now being exploited widely to extend the shelf life of a wide range of products, including enzymes, antibodies and vaccines.Citation6 Finally, trehalose and trehalose derivatives such as trehalose-6-phosphate have been shown to regulate some biological processes such as metabolism and growth in plants and in fungi.Citation1,6,Citation7
The roles of trehalose in microbial virulence have been studied in fungal and bacterial pathogens. Mutations that abolish trehalose production in a range of fungi including Candida albicans, Magnaporthe oryzae, Stagonospora nodorum, Cryptococcus neoformans, and Cryptococcus gattii result in reduced virulence.Citation5 In M. tuberculosis the inactivation of the TreYZ pathway for trehalose biosynthesis reduced the ability of the bacterium to establish chronic disease.Citation8 The situation is also complicated by the findings that the role of trehalose in virulence may be host species dependent. For example, in Pseudomonas aeruginosa the disruption of trehalose biosynthesis reduced virulence toward plants, but not virulence toward Caenorhabditis elegans, Drosophila melanogaster, or mice.Citation9 Trehalose degradation, by endogenous trehalase, has been reported to play a role in virulence in some fungal pathogens including M. oryzae and C. albicans, but had no effect on virulence in C. gattii.Citation5 Trehalase has previously been identified as a possible virulence associated gene in bacteria, since it is present in bacterial pathogens but absent or uncommon in non-pathogens.Citation10 Orthologues have been identified in 7 pathogenic bacteria including Burkholderia pseudomallei,Citation10 [personal communications. In B. pseudomallei this gene is annotated as treA and the encoded protein TreA has sequence identity with proteins in Salmonella typhimurium (55%), Klebsiella pneumoniae (56%), Shigella fexneri (52%), E. coli 0157:H7 (56%) and Pseudomonas aeruginosa (60%). This has lead us to hypothesize that TreA could be important during mammalian infection.
Burkholderia pseudomallei is the causative agent of melioidosis, a serious and often fatal disease of humans. The disease is frequently reported in Southeast Asia and Northern Australia and there is increasing evidence that melioidosis occurs in many, and possibly all, tropical countries.Citation11 A recent study has found that the incidence of this disease is likely to have been significantly underestimatedCitation12 and global deaths from melioidosis are comparable to those due to measles and much higher than fatalities due to leptospirosis or dengue fever.Citation12 In many of these countries the disease is currently mis-diagnosed as tuberculosis. Although melioidosis can occur in apparently healthy individuals, conditions such as diabetes predispose individuals.Citation13 The manifestations of disease range from an asymptomatic infection, to a fatal sepsis.Citation13 The bacterium has the ability to establish persistent but asymptomatic infections in humans for up to 60 y.Citation14 Therapy is not always successful because the bacterium is resistant to many antibiotics. Consequently, a protracted course of treatment with a combination of antibiotics is required and relapse following treatment is common.Citation13 Currently there is no licensed vaccine available for the prevention of disease. In endemic regions, B. pseudomallei can be readily isolated from soil and water. Melioidosis in humans is a consequence of exposure to this bacterium, via cuts and abrasions, inhalation or ingestion. The lifecycle of the bacterium in the environment is poorly understood but it is known that it can survive for years in hostile environments lacking nutrients and can survive exposure to a wide range of temperatures and dehydration.Citation15,16 In this study we have set out to investigate whether trehalase plays a role in virulence of B. pseudomallei.
Results
Construction of B. pseudomallei treA mutant
We identified a single trehalase gene, encoding a 565 amino acid protein, located on chromosome 2 (BPSS0671) in B. pseudomallei K96243. This gene was also present in all of the reported B. pseudomallei genome sequences. The B. pseudomallei trehalase was similar to the E. coli neutral trehalase (treA) with 56% amino acid identity. We constructed a treA deletion mutant using vector pMo130.Citation17 The mutant was genome sequenced to confirm that treA was deleted and we did not find additional mutations in the genome. To complement the mutant we cloned the treA gene downstream of the native promoterdownstream of a strong constitutive (dhfr) promoter or downstream of a regulatable promoter (rhaB). The plasmids (pBHRnat-treA pDA17-treA or pSCrhaB2-treA respectively) were transformed into B. pseudomallei ΔtreA. One or more of these plasmids were then used in subsequent assays.
In M9 glucose both wild type B. pseudomallei and B. pseudomallei ΔtreA grew similarly (). In contrast, when the strains were grown in M9 trehalose, the ΔtreA mutant failed to grow whereas the wild type strain reached a final optical density of OD590nm 1.2 (). The complemented strains B. pseudomallei ΔtreA/pBHR-nattreA and B. pseudomallei ΔtreA/pDA17-treA were able to grow in M9 trehalose, although it should be noted that this complementation assay was only performed once ().
Figure 1. Growth of B. pseudomallei wild type, ΔtreA mutant or complemented mutants in M9 medium containing 0.4% glucose with agitation (A) or 0.4% trehalose with agitation (B). The ability of complemented mutants (ΔtreA/pBHR-nattreA and ΔtreA/pDA17-treA) to grow in M9 medium containing 0.4% trehalose, in a static 96 well plate format, was recorded after 48 hours (C). Values represent the mean from one experiment performed in triplicate. Error bars show standard error of the mean (SEM).
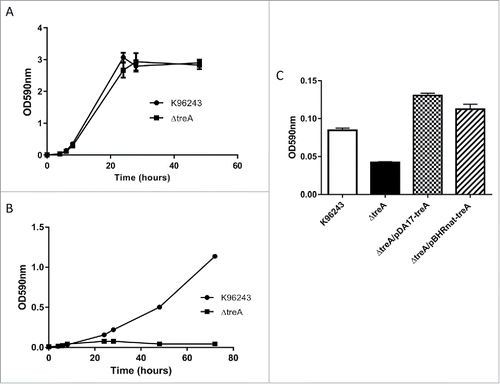
ΔtreA had increased tolerance to thermal stresses
Bacterial trehalases have previously been shown to have a role in thermal stress.Citation18 As a consequence, we assayed the role of B. pseudomallei treA in survival after heat and cold stress. Overnight cultures of B. pseudomallei, B. pseudomallei ΔtreA or B. pseudomallei ΔtreA/pSCrhaB2-treA were standardized to OD590nm 0.1 in LB broth before heating at 65°C for 2 hours and enumerating survivors on LB agar. The wild type and complemented mutant strains had survival frequencies of approximately 10−3 (). In comparison the survival frequency of the ΔtreA mutant was significantly higher at approximately 10−2 (p = 0.015 compared to WT). We also tested survival following exposure to cold stress. Overnight cultures of B. pseudomallei, B. pseudomallei ΔtreA, B. pseudomallei ΔtreA/pBHRnat-treA or B. pseudomallei ΔtreA/pDA17-treA were standardized to 100 CFU and incubated at 4°C for 5 d before enumeration on LB agar at 37°C. On average the survival frequency was 10−0.8 (). In contrast the survival frequency of the ΔtreA mutant was 10−0.3 (p = 0.004 compared to wild type). The B. pseudomallei ΔtreA/pBHRnat-treA strain had a survival frequency of approximately 10−1, whereas B. pseudomallei ΔtreA/pDA17-treA had no survival.
Figure 2. Survival of B. pseudomallei wild type ΔtreA mutant, pSCrhaB2-treA complement, pDA17-treA complement or pBHR-nattreA complement after exposure to heat stress at 65°C (A) or cold stress at 4°C (B) Values represent the mean from 5 independent experiments performed in triplicate or 2 independent experiments with 9 replicates respectively. Error bars show SEM. * = p < 0.05, *** = p < 0.001, **** = p < 0.0001 following one way Anova, Tukey post-test.
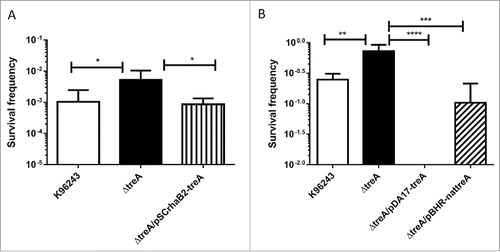
ΔtreA had reduced ability to survive inside macrophages
The intracellular survival of B. pseudomallei wild type, the ΔtreA mutant or the pDA17-treA complemented mutant were determined by counting the number of bacteria at each time point. The overall patterns of infection by the wild type and ΔtreA mutant were similar; the numbers of intracellular bacteria decreased between 2 and 4 hours post infection but increased by 6 to 8 hours post infection. However, even though the J774.A.1 macrophages were infected with wild type and ΔtreA mutant at an MOI of 10 the numbers of ΔtreA mutant bacteria were significantly lower than the parental strain at 2, 4, 6, and 8 hours post infection (p = 0.018, 0.085, 0.0003, and 0.0008 respectively). This defect in intracellular survival was restored in the complemented strain ().
Figure 3. Intracellular survival of B. pseudomallei wild type, ΔtreA mutant or pDA17-treA complemented mutant in J774.A.1 macrophages. Macrophages were infected with bacteria at an MOI of 10 and intracellular bacteria enumerated on LB agar. Values represent the mean from 3 independent experiments, performed in triplicate, with error bars showing the SEM s. * = p < 0.05, *** = p < 0.001 following students t-test.
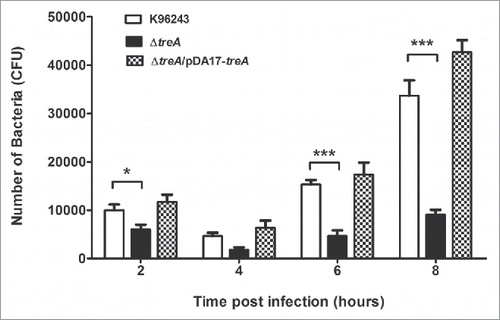
Effects of biochemical-induced stress conditions on growth
Next, stresses that B. pseudomallei may encounter in macrophages were tested in vitro. We saw no difference in survival of the mutant, compared to the wild type, after exposure to pH 4 for up to 60 min, or with 1 M hydrogen peroxide (data not shown). Compared with the wild-type, sensitivity of the ΔtreA mutant was not significantly different either to intracellularly or to extracellularly generated superoxide using paraquat or xanthine/xanthine oxidase, respectively (data not shown). However, the mutant was more susceptible to killing by 0.04 M tert-butyl hydroperoxide (tBOOH) with p = 0.016 and the phenotype was restored in the pDA17-treA complemented strain ().
treA is important in biofilm formation
B. pseudomallei, B. pseudomallei ΔtreA or B. pseudomallei ΔtreA/pDA17-treA were inoculated into TSB and biofilms were grown on peg lids inserted into the cultures. Following 48 hours growth, pegs were stained with crystal violet and the optical density measured following an ethanol wash to quantify biofilm levels. The wild type B. pseudomallei and B. pseudomallei ΔtreA/pDA17-treA strains had similar levels of staining giving standardized optical density readings (OD490 nm/OD595 nm) of 0.1203 ± 0.01 and 0.0984 ± 0.01 respectively (). In contrast the B. pseudomallei ΔtreA mutant had significantly reduced staining and the standardized optical density of the released crystal violet solution was (OD490nm/OD595nm) 0.06 ± 0.01.
Figure 5. Relative biofilm formation by B. pseudomallei wild type, ΔtreA mutant or pDA17-treA complement measured as crystal violet (CV) bound (OD490nm) / turbidity of bacterial culture (OD590nm). Values represent the mean from 3 independent experiments each with 12 replicates. Error bars show SEM. *** = p < 0.001, **** = p < 0.0001 following one-way Anova, Tukey post-test.
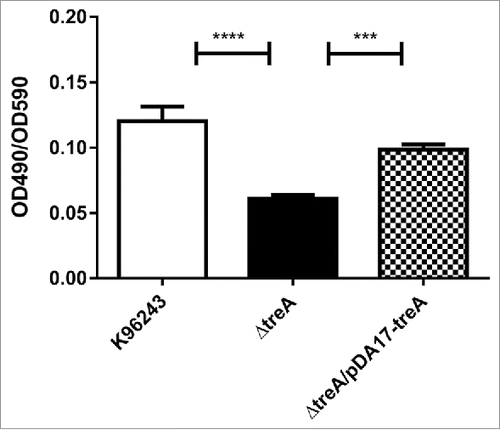
To determine if the difference in biofilm formation was due to differences in capsular polysaccharide production, the bacteria were incubated with an anti-capsule antibody and binding was quantified using a GFP labeled secondary antibody and fluorescence microscopy. Labeled B. pseudomallei, B. pseudomallei ΔtreA or B. pseudomallei ΔtreA/pDA17-treA all had similar pixel intensities following image quantification (Fig. S1) indicating that similar amounts of capsule polysaccharide were present on the bacterial cell surface. Incubating each strain with an anti-LPS antibody and quantifying with a GFP labeled secondary antibody as a control, did not result in fluorescence (data not shown). This evidence is consistent with the presence of a CPS layer on all the strains blocking binding of the anti-LPS antibody.
ΔtreA is attenuated in the G. mellonella and mouse infection model
After challenging G. mellonella with 103 Colony forming units (CFU), all larvae infected with wild type B. pseudomallei were dead at 27 hours, whereas 40% of larvae challenged with the ΔtreA mutant were alive (). We were unable to complement the wild type phenotype when challenging G. mellonella with B. pseudomallei ΔtreA/pDA17-treA or B. pseudomallei ΔtreA/pBHRnat-treA and speculate this could be due to the lack of antibiotic selection to retain the complementation plasmids during infection (Data not shown). When mice were challenged with wild type B. pseudomallei, death of the mice was recorded from day 4 until day 42. In contrast, all mice challenged with B. pseudomallei ΔtreA were still alive at the end of the study (). However, when the ΔtreA infected mice were culled, bacteria were isolated from lungs, livers, and spleens with average CFU counts of 2.3 × 104, 4.9 × 103 and 7.5 × 102 CFU/ml respectively. Our results suggest a marked reduction in virulence of the B. pseudomallei ΔtreA mutant in both G. mellonella and mice.
Figure 6. Virulence of B. pseudomallei wild type or ΔtreA mutant in G. mellonella larvae or in mice. (A) Groups of 10 G. mellonella larvae were challenged with 103 CFU of B. pseudomallei K96243 or the ΔtreA mutant. Values represent the mean from 3 independent experiments. Error bars show SEM. * = p < 0.05 following 2 way Anova, Sidak's multiple comparisons test. (B) Groups of 6 BALB/c mice challenged via i.p. route with 3.4 × 104 CFU of B. pseudomallei K96243 (solid line) or 6.7 × 104 CFU of the ΔtreA mutant (dotted line).
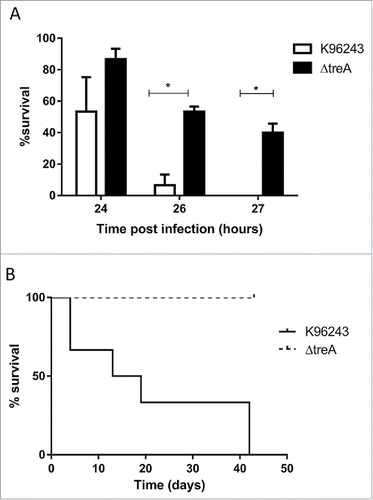
Discussion
Trehalose plays a number of distinct functions in plants, invertebrate and microbial cells from serving as an energy storage carbohydrate, to protecting membranes and proteins from stress-induced damage and to a regulator of the glycolytic path and signaling pathways.Citation19 In this study we have identified a single trehalase encoding gene in B. pseudomallei. The protein was similar to the E. coli TreA trehalase, which is located in the periplasmic space of the bacterium. E. coli also possesses a second trehalase (TreC) which is cytoplasmic, and a TreB transporter which is located in the inner membrane and is responsible for import of trehalose into the cell. Neither TreB nor TreC were identified in the genome sequences of B. pseudomallei. However at high osmolarity the normal pathway for the uptake and utilization of trehalose is blocked.Citation20 E. coli can also utilize periplasmic trehalose by splitting it into glucose molecules, which are subsequently taken up by the phosphotransferase- mediated uptake system.Citation20 This may be the same mechanism that B. pseudomallei uses to utilize trehalose without having a trehalose transporter. B. pseudomallei also has no apparent cytoplasmic trehalase within its genome. However, it does contain a putative trehalose synthase (treS) gene. TreS catalyzses the reversible interconversion of trehalose and maltose within the cytoplasm.Citation21 Like many bacteria B. pseudomallei possess an otsBA operon, which encodes trehalose sythetase/phosphatase. Therefore, it appears that B. pseudomallei may both produce and metabilize trehalose within the bacterial cell. The biosynthetic pathway is broadly paralleled in fungi. In fungi, acid and neutral trehalases are involved in trehalose degradation. The former are found in vacuoles within the fungal cell, while neutral trehalases are cytoplasmically located.Citation22
In this study we have shown that the treA gene plays a role in mitigating stress tolerance and in virulence of B. pseudomallei in murine and insect infection models. Whole genome transcriptome profiling of B. pseudomallei, has previously shown that treA is expressed in many different conditions but importantly appears to be upregulated in conditions of nutrient deprivation aswell as in normal human serum.Citation23 The ability of trehalose to protect cells against stress is well documented in invertebrates, plants and fungi.Citation6 The protective properties of trehalose are attributed to different mechanisms ranging from the formation of an inert “glass” around macromolecules to the ability of the molecule to replace or exclude water molecules.Citation24 As a consequence of the protective properties of trehalose the oxidation and degradation of macromolecules is minimized.Citation24 Deletion of the treA gene increased the ability of the bacterium to survive exposure to both heat and cold stress. Deletion of the Listeria monocytogenes trehalase (treA) has previously been shown to increase heat tolerance Citation18 and was associated with increased intracellular trehalose. Like E. coli, treA in L. monocytogenes is predicted to encode a cytoplasmic protein. Fungal trehalase mutants have also been reported to show enhanced thermal stress.Citation25 There is less evidence linking intracellular trehalose levels to cold stress, but trehalose is believed to act as a cryoprotectant in insects Citation26 and the addition of trehalose to media during prolonged cold storage has been shown to increase the long term survival of Salmonella enterica.Citation27 Our finding that the constitutive expression of treA (in the B. pseudomallei ΔtreA/pDA17-treA strain) abolished the ability of the bacterium to withstand cold shock, is consistent with increased levels of trehalase and consequently reduced levels of trehalose in the bacterial cell. However, quantification of trehalose levels would be needed to validate this. Furthermore, we can't rule out the possibility that deletion of trehalase is also having an effect upon the regulation and biosynthesis of trehalose via the genes otsBA. It's possible that the ΔtreA mutant is instead upregulated for trehalose production. Including a B. psedomallei ΔtreAΔotsBA mutant in our assays would be needed to exclude this possibility.
Further investigations into the role of treA might reveal whether our findings are linked to the observations that B. pseudomallei is only found in soils in tropical or sub-tropical regions of the world.
We also showed the B. pseudomallei ΔtreA mutant had decreased biofilm formation and our results are similar to the findings with a T6P hydrolase (treC) mutant of Klebsiella pneumonia.Citation28 The reduction in biofilm by the K. pneumoniae ΔtreC mutant was associated with reduced CPS production. Biofilm and CPS production were restored by the addition of glucose to the growth media. Previous studies have shown that adding glucose to B. pseudomallei growth media have also shown increased biofilm formation.Citation29 However our studies failed to reveal a difference in capsular polysaccharide (CPS) production by B. pseudomallei, B. pseudomallei ΔtreA or the complemented strains. Additionally we were unable to complement for the loss of biofilm with the addition of glucose in the growth media. Overall our findings indicate that different mechanisms link trehalose metabolism and biofilm production in B. pseudomallei and K. pneumonia.
While trehalose metabolism has previously been linked to the virulence of Burkholderia glumae and Pseudomonas aeruginosa in plants, Citation9,30 it has not been linked to virulence of bacterial pathogens in mammals. The P. aeruginosa ΔtreYZΔtreS trehalose biosynthesis mutant, which was attenuated in plants, retained full virulence in mice and insects.Citation9 Our results indicate a marked reduction in virulence of the B. pseudomallei ΔtreA mutant in G. mellonella and in mice (although complementation would ideally be needed to provide further evidence) and this was reflected in the reduced ability of the ΔtreA mutant to grow in murine macrophages.The ability to resist intracellular killing by oxidative stress is not related to trehalase because we found no difference in H2O2 response between B pseudomallei wild-type and the ΔtreA mutant. Our data did show a difference in resistance to tert-butyl hydroperoxide (tBOOH). tBOOH is an organic hydroperoxide that decomposes to alkoxyl and peroxyl radicals that can further react to metal ions to generate reactive oxygen species (ROS) such as H2O2.Citation31 Citation32 tBOOH is more stable than H202 and additionally decomposition of tBOOH accelerates lipid peroxidation and depletes cell glutathione, so has additional effects to just hydrogen peroxide.Citation33 Furthermore, the proteins that respond to each stressor are different. H2O2 requires catalase, but tBOOH requires Ohr or AhpC.Citation34 This may explain the different outcome of both experiments.
While trehalase has not previously been linked to bacterial virulence of mammals or insects, there are reports of trehalase playing a role in fungal virulence.Citation25,35,Citation36 In the study by Sanchez-Fresneda et al, the virulence potential of Candida parapsilosis trehalase mutants were also reduced in a murine model, as determined by fewer CFU numbers isolated from kidney tissue compared to wild type and complemented strains.Citation25 Attenuation of the B. pseudomallei ΔtreA mutant in mice may also be due to lower cell numbers. This could be due to slower growth or increased bacterial clearance by the host immune system. Alternatively it may be linked to reduced ability to form biofilm, sinceB. pseudomallei that produce low levels of biofilm have previously been shown to have reduced virulence in a mouse infection model.Citation37
Proteins involved in trehalose biosynthesis have been proposed as novel drug targets. This pathway is absent in mammalian cells and the enzymes involved in this pathway are highly specific.Citation19 Our finding that trehalase plays a role in virulence of B. pseudomallei suggests that that this pathway merits further investigation, as a drug target for the treatment of melioidosis.
Materials and methods
Bacterial stains, macrophage cell line, culture conditions, and reagents
E. coli strains DH5α, S17–1 λ pir, and B. pseudomallei K96243 were grown at 37°C in Luria Bertani broth (LB). Where indicated B. pseudomallei was gown in M9 minimal medium (pH 7.4) containing 0.4% w/v glucose or trehalose. Chloramphenicol (30 μg/ml for E. coli and 50 μg/ml for B. pseudomallei) and kanamycin (50 μg/ml for E. coli and 400 μg/ml for B. pseudomallei) were added as required. LB agar without sodium chloride but containing 10% (w/v) sucrose was used in the final step of mutant selection. Bacterial growth was determined by measuring the optical density at 600 nm in triplicate. The mouse macrophage cell line J774A.1 was obtained from the American Type Culture Collection (ATCC, Manasssas, Va.) and cells were maintained in Dulbecco's modified Eagle's medium (DMEM) supplemented with 10% (v/v) fetal bovine serum (HYCLONE) ), 1% L-glutamine (250 mM) (Hyclone) and 1% penicillin / streptomycin (Hyclone) at 37°C with 5% CO2. For infection with B. pseudomallei, macrophages were cultured in Gibco L-15 media (Invitrogen) at 37°C without CO2. Reagents used in this study were obtained from Sigma-Aldrich unless stated otherwise.
Construction of treA mutant
A DNA fragment that included 540-bp upstream and 440-bp downstream regions of the B. pseudomallei K96243 treA coding region and flanked by BgllI and NheI restriction sites was synthesized by GENEART. The DNA fragment was ligated into suitably digested pMo130 Citation17 and electroporated into E. coli DH5α. Recombinant plasmids were selected on LB agar containing 400μg/ml kanamycin. The recombinant plasmid was isolated and electroporated into E. coli S17-1 λpir, then conjugated with B. pseudomallei K96243. The conjugation and sacB counter selection was carried out as previously described.Citation38 Transconjugants were selected on LB agar containing 50 μg/ml chloramphenicol. Colonies were selected and sub cultured into LB broth and an overnight culture was diluted 104 fold and plated onto LB agar without salt containing 10% sucrose. After incubation at 24°C for 2–3 d colonies were picked and the treA deletion verified by PCR using primers F1 (5′- GTGCGATGAAAGCGTAGAGG-3′ binds 700 bp upstream of treA ORF) and R2 (5′-AATACGGACCGCTCCATGC 3′ binds 550 bp downstream of the treA ORF) or F2 (5′- CGCTCAAGCTGCTCGATCTG-3′ which binds within the last 30 bp at the 3′ end of treA) and R2.
Genome sequencing of the ΔtreA mutant
B pseudomallei genomic DNA was harvested by the method described previously.Citation39 Rehydrated DNA was checked for quality by running on an agarose gel and running on a bioanalyser. The DNA was sequenced by the University of Exeter sequencing service and output files were aligned and analyzed against the published K96243 genome using artemis genome browser and annotation tool.
Construction of complementation plasmids
The treA gene was PCR amplified using Failsafe polymerase and the primers treA_fwd 5′- TTATGGATCCATGGTCACGCCGCGGCATCGCCCGCTTCAT-3′ and treA_rv 5′- GCTAAAGCTTTCAGCCGCCGTACAGATCGAGCAGCTTGAG-3′. The purified PCR product was then cloned into the BamHI and HindIII sites of pSCrhaB2 Citation40 or pDA17.Citation41 For cloning treA and the predicted promoter region into pBHR4, the treA open reading frame and a region 300-bp upstream and 200-bp downstream was PCR amplified using primers treA_FWD_Nat 5′-TTAAGAGCTCCACGGTCATTTGCGCGAAGTGTGGGAAG-3′ and treA_RV_Nat 5′-ATTAGGATCCCGGTTTCCGACGGCGCATC-3′. The purified product was cloned into the SacI and BamHI sites of pBHRCitation42 removing the GroES promoter.
Biofilm assay
Bacterial cultures were grown on tryptic soy agar (TSA) plates at 37°C for 16 hour and then inoculated into PBS standardizing to OD590nm 1.0. Cultures were then diluted 1:2000 in fresh tryptic soy broth (TSB). 150µl aliquots were added to a 96-well peg lidded plate (12 wells for each culture) and incubated at 37°C. After 24 hours the peg lid was transferred into fresh TSB media for a further 24 hours before measuring the optical density of the plate at 590 nm. The peg lids were then washed in PBS before baking at 65°C for 30 minutes. Biofilms were stained with crystal violet for 30 minutes and then washed 3x with PBS. Crystal violet stain was released with ethanol treatment for 20 minutes and measured at OD490 nm. Relative biofilm levels were quantified by comparing OD490nm/OD590nm.
CPS staining
Overnight cultures of B. pseudomallei were diluted to OD590nm 0.1 in 1 ml of PBS containing 4 % Paraformaldehyde (PFA) and incubated for 15 minutes on the bench. Cultures were then centrifuged at 13000 × g for 7 minutes and cells resuspended in 500 µl 5 % Bovine serum albumin (BSA) / phosphate buffer saline (PBS) and incubated for 1 hour. The IgG 2b isotype anti-CPS I monoclonal antibodyMab 4VIH12 Citation43 was then added to each strain at a final concentration of 25 µg/ml and incubated for 1 hour at 37°C. The cells were then harvested by centrifugation and washed twice in 500 µl PBS. The cells were then resuspended in 500 µl 5 % BSA/PBS containing anti- goat anti-rabbit GFP tagged secondary antibody (Licor) at a 1:400 dilution and incubated at 1 hour at 37°C. The cells were then harvested by centrifugation and washed twice in PBS. Finally the cells were suspended in 100 µl PBS. Ten microlitre µl aliquots were spotted onto a microscope slide, air-dried and overlaid with vector shield. Slides were visualized under the GFP filter on a fluorescence microscope (100 × objective) and imaged. Quantification of pixel intensity was carried out using image J software. The fluorescence intensity of all bacteria in at least 3 fields of view per strain was calculated.
Heat stress
Overnight cultures were standardized to OD590nm 0.1 in LB broth and heated at 65°C for 2 hours. Cells were serial diluted and enumerated on LB agar. Survival frequency was calculated by the number of bacteria post heat treatment compared to pre-treatment.
Cold stress
Overnight cultures were standardized and serial diluted in LB before spread plating 100 bacteria onto LB agar plates. Half the plates were incubated at 37°C overnight (non-treated) and half incubated at 4°C for 5 d. After 5 d these plates were then incubated at 37°C for 1 day to enumerate survivors. Percentage survival was calculated by the number of CFU following cold stress compared to the number of CFU for non-treated samples.
Hydrogen peroxide and tBOOH stress
Overnight cultures were standardized to OD590nm 0.1 in LB broth and 100 µl of culture was spread plated onto LB agar plates. Five millimeter diameter paper discs were then placed onto the plates and 5 µl of 2 M H2O2 (Sigma-Aldrich) or 6 µl of 0.04 M tert-butyl hydroperoxide (t-BOOH, Sigma-Aldrich) was applied to each disc. Plates were then incubated at 37°C for 24 hours before measuring the area of growth inhibition around the discs.
Superoxide stress
To investigate the effect of intracellular superoxide on viability, a paraquat assay was used. The optical density (OD590nm) of an overnight bacterial culture was adjusted to 0.1 and 130 μl of the culture was spread onto LB agar. Paraquat solutions (100, 50, 10 and 5 mM) were pipetted onto either the surface of the inoculated agar or onto 6 mm sterile filter discs on the plates. After incubation at 37°C for 1 day or 20°C for 3 d the zones of inhibition were measured.
To determine sensitivity to extracellular superoxide, a xanthine/xanthine oxidase assay was used. Overnight cultures were diluted to OD590nm of 0.1 (∼108 CFU/mL) and 1 ml of the culture was incubated with xanthine/xanthine oxidase (250 mM/0.14 U final concentrations, respectively). Catalase (100 U) was added to protect cells from H2O2 generated as a superoxide degradation product. After 30, 60, 90 or 120 minutes, bacteria were enumerated on LB agar. Colonies were counted after 1 day incubation at 37°C.
Acid stress assay
Bacterial overnight cultures were washed with PBS and adjusted to OD590nm 0.1 in LB at pH 4, (acidified with 1 M Hydrochloric acid) and incubated at 37°C for 0, 10, 20, 30 or 60 minutes. Following incubation, bacteria were washed in PBS and enumerated. The percentage of survival was calculated by comparing against the number of bacteria at T0.
Galleria mellonella killing assay
Bacterial virulence toward G. mellonella (waxmoth) larvae was assessed by killing assays as described previously.Citation42 Briefly, 103 CFU in a volume of 10 μl were injected into the hemocoel of 10 larvae per bacterial strain. Larvae were incubated at 37°C and the number of dead larvae scored after 24, 26, and 27 hours.
Survival in macrophages
Prior to infection, J774.A.1 macrophages were cultured in DMEM medium. Overnight cultures of B. pseudomallei were diluted to 1 × 106 CFU/ml in DMEM medium, then added to wells seeded with 1 × 105 macrophages (MOI of 10). After incubation at 37°C for 2 h extracellular bacteria were killed by replacing growth medium with DMEM medium containing 250 μg/ml kanamycin for 2 h, followed by maintenance in DMEM containing 10 μg/ml kanamycin. At 2, 4, 6 or 8 h post infection, cells were washed with pre-warmed PBS to remove the antibiotic. Viable intracellular bacteria were released from the infected cells by adding 0.1% (v/v) Triton X-100. The cell lysates were serially diluted with sterile distilled water and appropriate dilutions plated on LB agar. The numbers of colony forming unit (CFU) were counted after incubation at 37°C for 24 to 36 hours.
Virulence studies in mice
Female BALB/c age-matched mice, approximately 6 weeks old, were used in this study. The mice were grouped together in cages of 5 with free access to food and water and subjected to a 12 hour light/dark cycle. For challenge the animals were handled under bio-safety level III containment conditions. All investigations involving animals were carried out according to the requirements of the Animal (Scientific Procedures) Act 1986. In two separate experiments, groups of 6 mice were challenged with wild type B. pseudomallei strain K96243 or ΔtreA by the intraperitoneal (i.p.) route and the infection monitored for 5 weeks. Humane endpoints were strictly observed and animals deemed incapable of survival were humanely killed by cervical dislocation.
Disclosure of potential conflicts of interest
No potential conflicts of interest were disclosed.
KVIR_S_1199316.docx
Download MS Word (26.9 KB)Funding
This work was supported by Defense Science and Technology Laboratory, grant DSTLX-1000060221 (WP2), Transformational Medical Technologies program contract W911NF-08-C-0023 from the Department of Defense Chemical and Biological Defense program through the Defense Threat Reduction Agency (DTRA), Thailand research fund (TRF), Commission of Higher Education (CRE) and Mahidol University. MV is a grantee of TRF MRG5480076 and ICTM grant from The Faculty of Tropical Medicine, Mahidol University, Thailand.
References
- Elbein AD, Pan YT, Pastuszak I, Carroll D. New insights on trehalose: a multifunctional molecule. Glycobiology 2003; 13:17R-27R; PMID:12626396; http://dx.doi.org/10.1093/glycob/cwg047
- Becker A, Schloder P, Steele JE, Wegener G. The regulation of trehalose metabolism in insects. Experientia 1996; 52:433-9; PMID:8706810; http://dx.doi.org/10.1007/BF01919312
- Thevelein JM, den Hollander JA, Shulman RG. Changes in the activity and properties of trehalase during early germination of yeast ascospores: correlation with trehalose breakdown as studied by in vivo 13C NMR. Proc Natl Acad Sci USA 1982; 79:3503-7; PMID:6954495; http://dx.doi.org/10.1073/pnas.79.11.3503
- Rousseau P, Halvorson HO, Bulla LA, Jr., St Julian G. Germination and outgrowth of single spores of Saccharomyces cerevisiae viewed by scanning electron and phase-contrast microscopy. J Bacteriol 1972; 109:1232-8; PMID:4551750
- Tournu H, Fiori A, Van Dijck P. Relevance of trehalose in pathogenicity: some general rules, yet many exceptions. PLoS Pathog 2013; 9:e1003447; PMID:23966851; http://dx.doi.org/10.1371/journal.ppat.1003447
- Iturriaga G, Suarez R, Nova-Franco B. Trehalose metabolism: from osmoprotection to signaling. Int J Mol Sci 2009; 10:3793-810; PMID:19865519; http://dx.doi.org/10.3390/ijms10093793
- Ponnu J, Wahl V, Schmid M. Trehalose-6-phosphate: connecting plant metabolism and development. Front Plant Sci 2011; 2:70; PMID:22639606; http://dx.doi.org/10.3389/fpls.2011.00070
- Murphy HN, Stewart GR, Mischenko VV, Apt AS, Harris R, McAlister MS, Driscoll PC, Young DB, Robertson BD. The OtsAB pathway is essential for trehalose biosynthesis in Mycobacterium tuberculosis. The J Biol Chem 2005; 280:14524-9; PMID:15703182; http://dx.doi.org/10.1074/jbc.M414232200
- Djonovic S, Urbach JM, Drenkard E, Bush J, Feinbaum R, Ausubel JL, Traficante D, Risech M, Kocks C, Fischbach MA, et al. Trehalose biosynthesis promotes Pseudomonas aeruginosa pathogenicity in plants. PLoS Pathog 2013; 9:e1003217; PMID:23505373; http://dx.doi.org/10.1371/journal.ppat.1003217
- Stubben CJ, Duffield ML, Cooper IA, Ford DC, Gans JD, Karlyshev AV, Lingard B, Oyston PC, de Rochefort A, Song J, et al. Steps toward broad-spectrum therapeutics: discovering virulence-associated genes present in diverse human pathogens. BMC Genomics 2009; 10:501; PMID:19874620; http://dx.doi.org/10.1186/1471-2164-10-501
- Birnie E, Wiersinga WJ, Limmathurotsakul D, Grobusch MP. Melioidosis in Africa: should we be looking more closely? Future Microbiol 2015; 10:273-81; PMID:25689538; http://dx.doi.org/10.2217/fmb.14.113
- Limmathurotsakul D, Golding N, Dance DA, Messina JP, Pigott DM, Moyes CL, Rolim DB, Bertherat E, Day NP, Peacock SJ, et al. Predicted global distribution of and burden of melioidosis. Nat Microbiol 2016; 1: 1-5; PMID:26877885; http://dx.doi.org/10.1038/nmicrobiol.2015.8
- White NJ. Melioidosis. Lancet 2003; 361:1715-22; PMID:12767750; http://dx.doi.org/10.1016/S0140-6736(03)13374-0
- Ngauy V, Lemeshev Y, Sadkowski L, Crawford G. Cutaneous melioidosis in a man who was taken as a prisoner of war by the Japanese during World War II. J Clin Microbiol 2005; 43:970-2; PMID:15695721; http://dx.doi.org/10.1128/JCM.43.2.970-972.2005
- Chen YS, Chen SC, Kao CM, Chen YL. Effects of soil pH, temperature and water content on the growth of Burkholderia pseudomallei. Folia Microbiol (Praha) 2003; 48:253-6; PMID:12800512; http://dx.doi.org/10.1007/BF02930965
- Wuthiekanun V, Smith MD, White NJ. Survival of Burkholderia pseudomallei in the absence of nutrients. Trans R Soc Trop Med Hyg 1995; 89:491; PMID:8560519; http://dx.doi.org/10.1016/0035-9203(95)90080-2
- Hamad MA, Zajdowicz SL, Holmes RK, Voskuil MI. An allelic exchange system for compliant genetic manipulation of the select agents Burkholderia pseudomallei and Burkholderia mallei. Gene 2009; 430:123-31; PMID:19010402; http://dx.doi.org/10.1016/j.gene.2008.10.011
- Ells TC, Truelstrup Hansen L. Increased thermal and osmotic stress resistance in Listeria monocytogenes 568 grown in the presence of trehalose due to inactivation of the phosphotrehalase-encoding gene treA. Appl Environ Microbiol 2011; 77:6841-51; PMID:21821737; http://dx.doi.org/10.1128/AEM.00757-11
- Eleutherio E, Panek A, De Mesquita JF, Trevisol E, Magalhaes R. Revisiting yeast trehalose metabolism. Curr Genet 2015; 61:263-74; PMID:25209979; http://dx.doi.org/10.1007/s00294-014-0450-1
- Gutierrez C, Ardourel M, Bremer E, Middendorf A, Boos W, Ehmann U. Analysis and DNA sequence of the osmoregulated treA gene encoding the periplasmic trehalase of Escherichia coli K12. Mol Gen Genet 1989; 217:347-54; PMID:2671658; http://dx.doi.org/10.1007/BF02464903
- Pan YT, Koroth Edavana V, Jourdian WJ, Edmondson R, Carroll JD, Pastuszak I, Elbein AD. Trehalose synthase of Mycobacterium smegmatis: purification, cloning, expression, and properties of the enzyme. Eur J Biochem 2004; 271:4259-69; PMID:15511231; http://dx.doi.org/10.1111/j.1432-1033.2004.04365.x
- Jules M, Beltran G, Francois J, Parrou JL. New insights into trehalose metabolism by Saccharomyces cerevisiae: NTH2 encodes a functional cytosolic trehalase, and deletion of TPS1 reveals Ath1p-dependent trehalose mobilization. Appl Environ Microbiol 2008; 74:605-14; PMID:18065618; http://dx.doi.org/10.1128/AEM.00557-07
- Ooi WF, Ong C, Nandi T, Kreisberg JF, Chua HH, Sun G, Chen Y, Mueller C, Conejero L, Eshaghi M, et al. The condition-dependent transcriptional landscape of Burkholderia pseudomallei. PLoS Genet 2013; 9:e1003795; PMID:24068961; http://dx.doi.org/10.1371/journal.pgen.1003795
- Jain NK, Roy I. Effect of trehalose on protein structure. Protein Sci 2009; 18:24-36; PMID:19177348
- Sanchez-Fresneda R, Martinez-Esparza M, Maicas S, Arguelles JC, Valentin E. In Candida parapsilosis the ATC1 gene encodes for an acid trehalase involved in trehalose hydrolysis, stress resistance and virulence. PLoS One 2014; 9:e99113; PMID:24922533; http://dx.doi.org/10.1371/journal.pone.0099113
- Wharton DA. Cold tolerance of New Zealand alpine insects. J Insect Physiol 2011; 57:1090-5; PMID:21397607; http://dx.doi.org/10.1016/j.jinsphys.2011.03.004
- Gruzdev N, Pinto R, Sela Saldinger S. Persistence of Salmonella enterica during dehydration and subsequent cold storage. Food Microbiol 2012; 32:415-22; PMID:22986208; http://dx.doi.org/10.1016/j.fm.2012.08.003
- Wu MC, Lin TL, Hsieh PF, Yang HC, Wang JT. Isolation of genes involved in biofilm formation of a Klebsiella pneumoniae strain causing pyogenic liver abscess. PLoS One 2011; 6:e23500; PMID:21858144; http://dx.doi.org/10.1371/journal.pone.0023500
- Ramli NS, Eng Guan C, Nathan S, Vadivelu J. The effect of environmental conditions on biofilm formation of Burkholderia pseudomallei clinical isolates. PLoS One 2012; 7:e44104; PMID:22970167; http://dx.doi.org/10.1371/journal.pone.0044104
- Kim S, Park J, Lee J, Shin D, Park DS, Lim JS, Choi IY, Seo YS. Understanding pathogenic Burkholderia glumae metabolic and signaling pathways within rice tissues through in vivo transcriptome analyses. Gene 2014; 547:77-85; PMID:24949534; http://dx.doi.org/10.1016/j.gene.2014.06.029
- Chance B, Sies H, Boveris A. Hydroperoxide metabolism in mammalian organs. Physiol Rev 1979; 59:527-605; PMID:37532
- Guidarelli A, Cattabeni F, Cantoni O. Alternative mechanisms for hydroperoxide-induced DNA single strand breakage. Free Radic Res 1997; 26:537-47; PMID:9212348; http://dx.doi.org/10.3109/10715769709097825
- Masaki N, Kyle ME, Farber JL. tert-butyl hydroperoxide kills cultured hepatocytes by peroxidizing membrane lipids. Arch Biochem Biophys 1989; 269:390-9; PMID:2919876; http://dx.doi.org/10.1016/0003-9861(89)90122-7
- Fontenelle C, Blanco C, Arrieta M, Dufour V, Trautwetter A. Resistance to organic hydroperoxides requires ohr and ohrR genes in Sinorhizobium meliloti. BMC Microbiol 2011; 11:100; PMID:21569462; http://dx.doi.org/10.1186/1471-2180-11-100
- Botts MR, Huang M, Borchardt RK, Hull CM. Developmental cell fate and virulence are linked to trehalose homeostasis in Cryptococcus neoformans. Eukaryot Cell 2014; 13:1158-68; PMID:25001408; http://dx.doi.org/10.1128/EC.00152-14
- Pedreno Y, Gonzalez-Parraga P, Martinez-Esparza M, Sentandreu R, Valentin E, Arguelles JC. Disruption of the Candida albicans ATC1 gene encoding a cell-linked acid trehalase decreases hypha formation and infectivity without affecting resistance to oxidative stress. Microbiology 2007; 153:1372-81; PMID:17464051; http://dx.doi.org/10.1099/mic.0.2006/003921-0
- Chin CY, Hara Y, Ghazali AK, Yap SJ, Kong C, Wong YC, Rozali N, Koh SF, Hoh CC, Puthucheary SD, et al. Global transcriptional analysis of Burkholderia pseudomallei high and low biofilm producers reveals insights into biofilm production and virulence. BMC Genomics 2015; 16:471; PMID:26092034; http://dx.doi.org/10.1186/s12864-015-1692-0
- Logue CA, Peak IR, Beacham IR. Facile construction of unmarked deletion mutants in Burkholderia pseudomallei using sacB counter-selection in sucrose-resistant and sucrose-sensitive isolates. J Microbiol Methods 2009; 76:320-3; PMID:19150470; http://dx.doi.org/10.1016/j.mimet.2008.12.007
- Grimberg J, Maguire S, Belluscio L. A simple method for the preparation of plasmid and chromosomal E. coli DNA. Nucleic Acids Res 1989; 17:8893; PMID:2587246; http://dx.doi.org/10.1093/nar/17.21.8893
- Milton DL, O'Toole R, Horstedt P, Wolf-Watz H. Flagellin A is essential for the virulence of Vibrio anguillarum. J Bacteriol 1996; 178:1310-9; PMID:8631707
- Flannagan RS, Linn T, Valvano MA. A system for the construction of targeted unmarked gene deletions in the genus Burkholderia. Environ Microbiol 2008; 10:1652-60; PMID:18341581; http://dx.doi.org/10.1111/j.1462-2920.2008.01576.x
- Wand ME, Muller CM, Titball RW, Michell SL. Macrophage and Galleria mellonella infection models reflect the virulence of naturally occurring isolates of B. pseudomallei, B. thailandensis and B. oklahomensis. BMC Microbiol 2011; 11:11; PMID:21241461; http://dx.doi.org/10.1186/1471-2180-11-11
- Atkins T, Prior R, Mack K, Russell P, Nelson M, Prior J, Ellis J, Oyston PC, Dougan G, Titball RW. Characterisation of an acapsular mutant of Burkholderia pseudomallei identified by signature tagged mutagenesis. J Med Microbiol 2002; 51:539-47; PMID:12132769; http://dx.doi.org/10.1099/0022-1317-51-7-539