ABSTRACT
Adhesion of Helicobacter pylori to the gastric mucosa is a prerequisite for the pathogenesis of H. pylori related diseases. In this study, we investigated the ganglioside composition of human stomach as the target for attachment mediated by H. pylori SabA (sialic acid binding adhesin). Acid glycosphingolipids were isolated from human stomach and separated into subfractions, which were characterized by mass spectrometry and by binding of antibodies, bacteria, and Solanum tuberosum lectin. H. pylori SabA binding gangliosides were characterized as Neu5Acα3-neolactohexaosylceramide and Neu5Acα3-neolactooctaosylceramide, while the other acid human stomach glycosphingolipids characterized (sulfatide and the gangliosides GM3, GD3, GM1, Neu5Acα3-neolactotetraosylceramide, GD1a and GD1b) were not recognized by the bacteria. Defining H. pylori binding glycosphingolipids of the human gastric mucosa will be useful to specifically target this microbe-host interaction for therapeutic intervention.
Introduction
Helicobacter pylori is a successful bacterium, which is exceptionally equipped to persistently colonize the human stomach. Colonization by this pathogen can lead to severe gastric diseases, ranging from chronic gastritis and peptic ulcer disease to malignant neoplastic diseases (gastric cancer and mucosa-associated lymphoid tissue (MALT) lymphoma). The adherence of H. pylori to the gastric epithelium is a virulence factor facilitating the colonization of the stomach. A number of different carbohydrate receptor candidates for H. pylori have been described [Citation1]. However, despite the multitude of candidate H. pylori receptors, only three carbohydrate binding adhesins, the blood group antigen binding BabA adhesin [Citation2], the sialic acid binding SabA adhesin [Citation3], and the lacdiNAc-binding LabA adhesin [Citation4], have been characterized to date. In addition, the interaction of HopQ with human CEACAMs was recently reported [Citation5,Citation6]. HopQ is thus the first protein binding adhesin of H. pylori. The adherence-associated lipoprotein A and B (AlpA/B) and the outer inflammatory protein A (OipA) are also involved in H. pylori adhesion [Citation7–9].
BabA, which binds Leb and other related fucosylated antigens, is the primary adhesin responsible for attachment of H. pylori to the human gastric mucosa. SabA binds to N-acetyllactosamine-based gangliosides with terminal α3-linked Neu5Ac, with a preferential binding to gangliosides with long N-acetyllactosamine chains, and also binds to gangliosides with fucose substitutions of the N-acetyllactosamine core chain, as e.g. the dimeric sialyl-Lex ganglioside [Citation10].
Another ganglioside binding protein of H. pylori is the soluble neutrophil activating protein HP-NAP, which specifically binds to gangliosides with a terminal NeuAcα3Galβ4GlcNAcβ3Galβ4GlcNAc sequence [Citation11]. HP-NAP is to some extent associated with the bacterial cell surface [Citation12], and could thus be involved in the ganglioside recognition by H. pylori bacterial cells. However, the ganglioside binding pattern of mutant H. pylori with knock-out of the gene coding for HP-NAP was identical to that of the parental wild type strain. Thus HP-NAP is not involved in the binding of H. pylori bacterial cells to gangliosides [Citation10].
In non-Leb individuals an enhanced H. pylori colonization density is obtained by interactions between the SabA adhesin and sialylated gastric glycoconjugates [Citation13]. Still, the content of sialylated glycoconjugates is low in the normal human gastric epithelium [Citation14–16]. The major acid glycosphingolipids of human stomach have been characterized as sulfatide and the gangliosides GM3, GM1, GD3, and GD1a [Citation17], but the detailed ganglioside composition has not yet been determined.
In the present study total acid glycosphingolipids were isolated from human stomach, and separated into subfractions, which were characterized by mass spectrometry and by binding of monoclonal antibodies, bacteria, and Solanum tuberosum lectin in chromatogram binding assays. Two minor H. pylori SabA binding gangliosides were characterized as Neu5Acα3-neolactohexaosylceramide and Neu5Acα3-neolactooctaosylceramide, while the other acid human stomach glycosphingolipids characterized (sulfatide and the gangliosides GM3, GD3, Neu5Acα3-neolactotetraosylceramide, GD1a and GD1b) were not recognized by H. pylori SabA.
Results
Binding of SabA expressing H. pylori to total acid glycosphingolipids of human stomach
To identify human gastric gangliosides to which H. pylori SabA binds, acid glycosphingolipids isolated from human stomach were separated on thin-layer chromatograms and tested for binding of BabA and SabA expressing H. pylori wild type strain J99, and the deletion mutant strains lacking SabA (J99/SabA-) or the H. pylori neutrophil activating protein (J99/NAP-). Chemical staining of the human stomach acid glycosphingolipids showed four major bands migrating as sulfatide and the gangliosides GM3, GD3, and GD1a (, lane 2). All three strains showed BabA mediated binding to the reference Leb hexaosylceramide (, lane 4), and to compounds migrating at the same level in the non-acid glycosphingolipid fraction from human stomach (, lane 3). The SabA expressing H. pylori strain J99 also bound to a number of minor slow-migrating compounds in the acid glycosphingolipid fraction from human stomach (, lane 2). The same binding pattern was obtained with the deletion mutant strain lacking the H. pylori neutrophil activating protein (, lane 2), demonstrating that HP-NAP has no role in the binding to human stomach gangliosides by H. pylori bacterial cells. However, no binding to these compounds was obtained with the J99/SabA deletion mutant strain (, lane 2), demonstrating that the binding of H. pylori to the slow-migrating acid glycosphingolipids of human stomach is mediated by SabA.
Figure 1. Binding of H. pylori to the total acid glycosphingolipids of human stomach. Thin-layer chromatogram after detection with anisaldehyde (A), and autoradiograms obtained by binding of H. pylori strain J99 (B), H. pylori strain J99/NAP- (C), and H. pylori strain J99/SabA- (D). The glycosphingolipids were separated on aluminum-backed silica gel plates, using chloroform/methanol/water 60:35:8 (by volume) as solvent system, and the binding assays were performed as described under "Materials and methods”. Autoradiography was for 12 h. The lanes were: Lane 1, total acid glycosphingolipids of human granulocytes, 40 μg; Lane 2, total acid glycosphingolipids of human stomach, 40 μg; Lane 3, total non-acid glycosphingolipids of human stomach from a blood group A individual, 40 μg; Lane 4, reference Leb hexaosylceramide (Fucα2Galβ3(Fucα4)GlcNAcβ3Galβ4Glcβ1Cer), 4 μg. In lane 2 # denotes the migration of sulfatide, ## the migration of the GM3 ganglioside, ### the migration of the GD3 ganglioside, and #### the migration of the GD1a ganglioside.
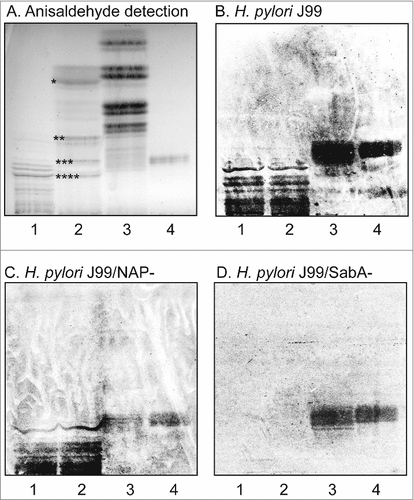
Characterization of the total acid glycosphingolipids of human stomach by LC-ESI/MS
The total acid glycosphingolipids of human stomach were next characterized by LC-ESI/MS. shows the base peak chromatogram obtained, and the glycosphingolipids identified by MS2 of the molecular ions are given in the chromatogram. Thus, MS2 of the [M-H+]− ion at m/z 778 identified sulfatide (not shown), while MS2 the [M-H+]− ion at m/z 1151 () identified the GM3 ganglioside, both with d18:1-16:0 ceramide. In the same manner, MS2 of the [M-2H+]2− ion at m/z 721 () identified the GD3 ganglioside with d18:1-16:0 ceramide, while Neu5Ac-neolactotetraosylceramide was demonstrated by MS2 of the [M-2H+]2− ion m/z 758 (), also with d18:1-16:0 ceramide. The GD1a ganglioside with d18:1-24:0 was identified by MS2 of the [M-2H+]2− ion at m/z 959 eluting at RT 22.4 min (), while the GD1b ganglioside with d18:1-24:0 was characterized by MS2 of the [M-2H+]2− ion at m/z 959 eluting at RT 23.4 min ().
Figure 2. LC-ESI/MS of the total acid glycosphingolipids of human stomach. (A) Base peak chromatogram from LC-ESI/MS of the total acid glycosphingolipids from human stomach. Sulfatide, SO3-3Galβ1Cer; GM3, Neu5Acα3Galβ4Glcβ1Cer; NeuAc-nLc4, Neu5Acα3Galβ4GlcNAcβ3Galβ4Glcβ1Cer; GD3, Neu5Acα8Neu5Acα3Galβ4Glcβ1Cer; GD1a, Neu5Acα3Galβ3GalNAcβ4(Neu5Acα3)Galβ4Glcβ1Cer; GD1b, Galβ3GalNAcβ4(Neu5Acα8Neu5Acα3)Galβ4Glcβ1Cer. (B) MS2 of the ion at m/z 1151 (retention time 17.0 min). (C) MS2 of the ion at m/z 721 (retention time 21.2 min). (D) MS2 of the ion at m/z 758 (retention time 19.4 min). (E) MS2 of the ion at m/z 959 (retention time 22.4 min). (F) MS2 of the ion at m/z 959 (retention time 23.4 min). See Supplemental Figure S1 for interpretation formulas.
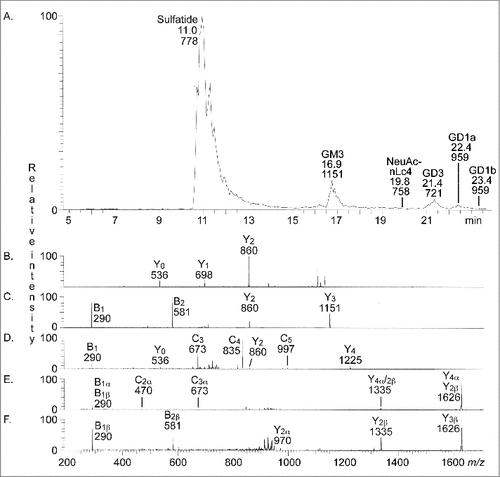
Characterization of the acid glycosphingolipids of human stomach by bacteria and antibody binding
To substantiate the data from mass spectrometry the binding of a number of carbohydrate binding ligands to the acid glycosphingolipids of human stomach was thereafter tested (). Again binding of H. pylori strain J99 to slow-migrating gangliosides of human stomach was obtained (, lane 1). The binding of sulfatide binding CS6 expressing Escherichia coli [Citation18] to a fast-migrating compound of the acid fraction of human stomach (, lane 1) supported the presence of sulfatide, while the binding of anti-Neu5Acα3-neolacto antibodies (, lane 1) to a more slow-migrating compound was in line with the presence of Neu5Acα3-neolactotetraosylceramide. Slow-migrating compounds were also recognized by the anti-GD1a (, lane 1) and anti-GD3 (, lane 1) antibodies, supporting the presence of the GD1a and GD3 gangliosides, respectively. Finally, no binding of monoclonal antibodies directed against the Neu5Acα3-Lea determinant (, lane 1), the Neu5Acα3-Lex determinant (, lane 1), or Neu5Acα6-neolacto (not shown), to the acid glycosphingolipids of human stomach occurred.
Figure 3. Characterization of the acid glycosphingolipids of human stomach by bacteria and antibody binding. Thin-layer chromatogram after detection with anisaldehyde (A), and autoradiograms obtained by binding of H. pylori strain J99 (B), sulfatide binding CS6 expressing Escherichia coli (C), monoclonal antibodies directed against Neu5Acα3-neolacto (D), the GD1a ganglioside (E), the GD3 ganglioside (F), Neu5Acα3-Lea (G), and Neu5Acα3-Lex (H). The glycosphingolipids were separated on aluminum-backed silica gel plates, using chloroform/methanol/water 60:35:8 (by volume) as solvent system, and the binding assays were performed as described under "Materials and methods”. Autoradiography was for 12 h. The lanes were: Lane 1, total acid glycosphingolipids of human stomach, 40 μg; Lane 2, reference total acid glycosphingolipids of human liver cancer, 40 μg; Lane 3, reference calf brain gangliosides, 40 μg. To allow comparison between the chromatograms in the figures the migration level of NeuAc5α3-neolactohexaosylceramide is indicated by the designation SnLc6.
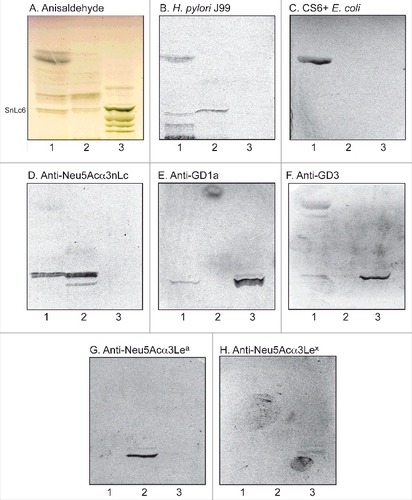
Isolation of H. pylori SabA binding gangliosides from human stomach
In order to characterize the minor slow-migrating H. pylori SabA binding gangliosides of human stomach, the total acid glycosphingolipid fraction (60 mg) was separated by repeated chromatographies on silicic acid and Iatrobeads columns, and the preparative procedure was monitored by binding of radiolabeled SabA-expressing H. pylori on thin-layer chromatograms. The first separation gave one fraction (5.6 mg) containing compounds migrating in the sulfatide region on thin-layer chromatograms, and one fraction (2.8 mg) with compounds migrating as the GM3 ganglioside. No binding of H. pylori to these fractions was obtained. After a number of further chromatographies six subfractions containing gangliosides migrating as Neu5Acα3-neolactotetraosylceramide and below were obtained (designated fractions S1-S6; , lanes 1–6). These ganglioside fractions were tested for binding of SabA-expressing H. pylori strain J99. Fractions S1-S3 were non-binding (, lanes 1–3), while binding of H. pylori to fractions S4, S5 and S6 was obtained (, lanes 4–6).
Figure 4. Binding of SabA-expressing H. pylori to acid glycosphingolipid subfractions from human stomach. Thin-layer chromatogram after detection with anisaldehyde (A), and autoradiograms obtained by binding of H. pylori strain J99 (B and C), Solanum tuberosum lectin (D) and monoclonal anti-Neu5Acα3-neolacto antibodies (E). The glycosphingolipids were separated on aluminum-backed silica gel plates, using chloroform/methanol/water 60:35:8 (by volume) as solvent system, and the binding assays were performed as described under "Materials and methods”. Autoradiography was for 12 h. The lanes were on A and B: Lanes 1–6, acid subfractions S1-S6 isolated from human stomach, 4 μg/lane; Lane 7, reference Neu5Acα3-neolactotetraosylceramide (Neu5Acα3Galβ4GlcNAcβ3Galβ4Glcβ1Cer) of human erythrocytes, 4 μg; Lane 8, reference NeuAc-G-10 ganglioside (Neu5Acα3Galβ4GlcNAcβ6 (Neu5Acα3Galβ4GlcNAcβ3)Galβ4GlcNAcβ3Galβ4Glcβ1Cer) of human erythrocytes, 1 μg. The lanes were on C-E: Lane 1, total acid glycosphingolipids of human neutrophils, 40 μg; Lanes 2–4, acid subfractions SI-SIII isolated from human stomach, 1 μg/lane. To allow comparison between the chromatograms in the figures the migration level of NeuAc5α3-neolactohexaosylceramide is indicated by the designation SnLc6.
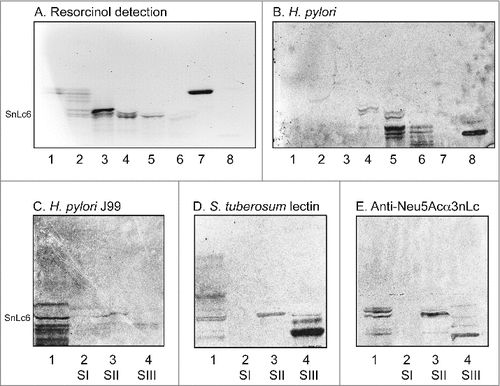
The three H. pylori binding fractions S4-S6 were pooled, and again separated an Iatrobeads column. After pooling the subfractions obtained according to their mobility on thin-layer chromatograms and their H. pylori binding activity, three fractions were obtained (designated fraction SI, SII and SIII). H. pylori SabA binding compounds were present in fractions SII and SIII (, lanes 3–4), whereas fraction SI was non-binding (, lane 2). Binding of the Neu5Acα3Galβ4GlcNAcβ3Galβ4GlcNAc recognizing lectin from Solanum tuberosum [Citation19] (, lanes 3–4), and anti-Neu5Acα3-neolacto antibodies (, lanes 3–4), to fractions SII and SIII was also obtained, whereas no binding of anti-Neu5Acα6-neolacto antibodies occurred (not shown). Taken together this demonstrated that the gangliosides in fractions SII and SIII had N-acetyllactosamine core chains and terminal α3-linked Neu5Ac.
Characterization of the H. pylori SabA binding fraction SII and fraction SIII of human stomach by LC-ESI/MS
The base peak chromatogram of fraction SII had a major [M-2H+]2− ion at m/z 941 corresponding to a [M-H+]− ion at m/z 1882, and indicating a ganglioside with one Neu5Ac, two HexNAc, four Hex, and d18:1-16:0 ceramide. MS2 of the [M-2H+]2− ion at m/z 941 () gave a series of Y ions (Y0 at m/z 536, Y3 at m/z 1063, Y5 at m/z 1428, and Y6 at m/z 1590), which along with the B- and C-type ions, demonstrated a ganglioside with Neu5Ac-Hex-HexNAc-Hex-HexNAc-Hex-Hex sequence and d18:1-16:0 ceramide.
Figure 5. LC-ESI/MS of fraction S-II and fraction S-III of human stomach. (A) MS2 of the ion at m/z 941 (retention time 28.2 min). (B) MS2 of the ion at m/z 1123 (retention time 30.3 min).
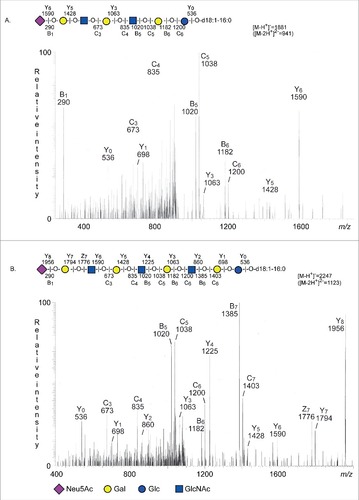
A [M-2H+]2− ion at m/z 1123 was present in the base peak chromatogram of fraction SIII.
This [M-2H+]2− ion at m/z 1123 corresponds to a [M-H+]− ion at m/z 2247, and indicated a ganglioside with one Neu5Ac, three HexNAc, five Hex, and d18:1-16:0 ceramide. Here MS2 () gave a Y ion series (Y0 at m/z 536, Y1 at m/z 698, Y2 at m/z 860, Y3 at m/z 1063, Y4 at m/z 1225, Y5 at m/z 1428, Y6 at m/z 1590, Y7 at m/z 1794, and Y8 at m/z 1956), which along with the B- and C-type ions, identified a ganglioside with Neu5Ac-Hex-HexNAc-Hex-HexNAc-Hex-HexNAc-Hex-Hex sequence and d18:1-16:0 ceramide.
Thus, LC-ESI/MS and the binding of the Neu5Acα3Galβ4GlcNAcβ3Galβ4GlcNAc recognizing lectin from S. tuberosum allowed identification of Neu5Acα3-neolactohexaosylceramide in fraction SII and Neu5Acα3-neolactooctaosylceramide in fraction SIII.
Binding of SabA-expressing H. pylori to the total acid glycosphingolipids of human gastric mucosa
Since the glycosphingolipids from human stomach were isolated from whole stomach tissue, and thus also could be derived from the submucosal tissue, we next examined the binding of H. pylori to acid glycosphingolipids from human gastric mucosal scrapings. Once again the same binding pattern was obtained, i.e. the bacteria bound distinctly to a number of slow migrating compounds (, lane 2), demonstrating that the H. pylori SabA binding gangliosides were present in human gastric mucosal cells. Binding of the Neu5Acα3Galβ4GlcNAcβ3Galβ4GlcNAc recognizing lectin from S. tuberosum to the acid glycosphingolipids from human gastric mucosa was also obtained (, lane 2), confirming the presence of gangliosides with N-acetyllactosamine core chains with terminal α3-linked Neu5Ac.
Figure 6 . Binding of SabA-expressing H. pylori to the total acid glycosphingolipids of human gastric mucosa. Thin-layer chromatogram after detection with anisaldehyde (A), and autoradiograms obtained by binding of H. pylori strain J99 (B), and S. tuberosum lectin (C). The glycosphingolipids were separated on aluminum-backed silica gel plates, using chloroform/methanol/water 60:35:8 (by volume) as solvent system, and the binding assays were performed as described under "Materials and methods”. Autoradiography was for 12 h. The lanes were: Lane 1, total acid glycosphingolipids of human neutrophils, 40 μg; Lane 2, total acid glycosphingolipids of mucosal scrapings from human stomach, 40 μg; Lane 3, total acid glycosphingolipids of non-mucosal residue from human stomach, 40 μg; Lane 4, reference NeuAcα3-neolactotetraosylceramide (Neu5Acα3Galβ4GlcNAcβ3Galβ4Glcβ1Cer) of human erythrocytes, 4 μg; Lane 5, reference Neu5Ac-G-10 ganglioside (Neu5Acα3Galβ4GlcNAcβ6(Neu5Acα3Galβ4GlcNAcβ3)Galβ4GlcNAcβ3Galβ4Glcβ1Cer) of human erythrocytes, 4 μg. To allow comparison between the chromatograms in the figures the migration level of NeuAc5α3-neolactohexaosylceramide is indicated by the designation SnLc6.
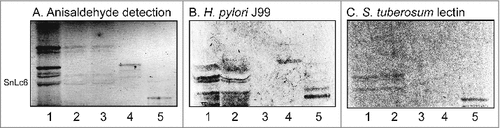
Inhibition assay
Next the potential blocking effect of the Neu5Acα3Galβ4GlcNAcβ3Galβ4GlcNAc binding lectin from S. tuberosum on the binding of H. pylori to human stomach gangliosides was evaluated by incubating chromatograms with unlabeled lectin before binding of radiolabeled bacteria. By this procedure the binding of H. pylori strain J99 to the gangliosides of human stomach was completely abolished (Fig. S3B, lane 2). The lectin also interfered with the binding of the bacteria to the gangliosides of human granulocytes (Fig. S3B, lane 1). However, in this case the binding was diminished, but several binding active compounds were still detected.
Characterization of sulfatides and the GM3 gangliosides by LC-ESI/MS
LC-ESI/MS of the fraction containing compounds migrating in the sulfatide region identified a battery of sulfatides with variant ceramide composition (Fig. S2). The predominant molecular species had long hydroxy fatty acids (h24:0 and h26:0), as reported previously [Citation17]. There were also minute amounts of sulfated dihexosylceramides.
GM3 with mainly sphingosine and non-hydroxy 16:0-24:0 fatty acids was characterized by LC-ESI/MS of the fraction having compounds migrating as the GM3 ganglioside (data not shown).
The acid glycosphingolipids of human stomach characterized in this study, and their H. pylori binding activity, are summarized in . In the binding experiments using mixtures of acid glycosphingolipids from human stomach or gastric mucosa a binding of the bacteria in the sulfatide region (e.g. , lane 1) was sometimes obtained. This binding could not be reproduced when using defined amounts of reference sulfatide, and was judged to be unspecific and caused by the relative high amounts of these compounds in the glycosphingolipid mixtures. An occasional binding at the level of Neu5Acα3-neolactotetraosylceramide, and also to reference Neu5Acα3-neolactotetraosylceramide, was also obtained (compare , lane 7 and , lane 4). Occasional binding to this ganglioside has been reported previously [Citation10], and is most likely due to suboptimal exposure of the Neu5Acα3Gal head group on this relatively short N-acetyllactosamine chain.
Table 1. Acid glycosphingolipids of human stomach identified by mass spectrometry and binding of carbohydrate recognizing ligands, and results on Helicobacter pylori binding
Discussion
In this study we have characterized the acid glycosphingolipids from human stomach and found that the major acid glycosphingolipids of human stomach are sulfatide and the GM3 ganglioside. In addition, the gangliosides GD3, GD1a, GD1b, Neu5Acα3-neolactotetraosylceramide, Neu5Acα3-neolactohexaosylceramide, and Neu5Acα3-neolactooctaosylceramide, were identified.
Neu5Acα3-neolactohexaosylceramide and Neu5Acα3-neolactooctaosylceramide, isolated from human hepatoma and human colon cancer, were identified as high affinity ligands in our previous study of the structural requirements for H. pylori ganglioside binding [Citation10]. A preferential binding to Neu5Acα3-neolactooctaosylceramide over Neu5Acα3-neolactohexaosyl-ceramide was observed, which most likely is due to a better access to the Neu5Acα3Gal group on the longer carbohydrate chain. Comparative binding studies using dilutions of gangliosides on thin-layer plates demonstrated an increased affinity for gangliosides carrying fucose residues on the core chain, since the bacteria bound better to the VIM-2 (Neu5Acα3Galβ4GlcNAcβ3Galβ4(Fucα3)GlcNAcβ3Galβ4Glcβ1Cer) and sialyl-dimeric-Lex (Neu5Acα3Galβ4(Fucα3)GlcNAcβ3Galβ4(Fucα3)GlcNAcβ3Galβ4Glcβ1Cer) gangliosides than to Neu5Acα3-neolactohexaosylceramide. Increased binding to the divalent G-10 ganglioside (Neu5Acα3Galβ4GlcNAcβ6(Neu5Acα3Galβ4GlcNAcβ3)Galβ4GlcNAcβ3Galβ4Glcβ1Cer), having two Neu5Acα3Galβ4GlcNAc branches was also observed.
Here, two minor H. pylori SabA binding gangliosides from human stomach were characterized as sialyl-neolactohexaosylceramide (Neu5Acα3Galβ4GlcNAcβ3Galβ4GlcNAcβ3Galβ4Glcβ1Cer), and sialyl-neolactooctaosylceramide (Neu5Acα3Galβ4GlcNAcβ3Galβ4GlcNAcβ3Galβ4GlcNAcβ3Galβ4Glcβ1Cer). Binding studies using acid glycosphingolipids from human gastric mucosal cells indicated that these gangliosides were indeed present in target cells of H. pylori.
The expression of the high affinity SabA binding sialyl-LeX determinant is low in the healthy non-inflamed human gastric mucosa [Citation14,Citation16]. In line with this, there was no binding of anti-sialyl-LeX antibodies to the acid glycosphingolipids of human stomach, and despite a targeted search for molecular ions of the sialyl-LeX and dimeric-sialyl-LeX gangliosides in the LC-ESI/MS study, these compounds could not be identified.
However, the gastric inflammation elicited by H. pylori infection leads to an alteration in the glycosylation of the gastric mucosa with upregulation of inflammation-associated sialyl-Lex antigens, promoting SabA-mediated attachment of H. pylori [Citation3]. Thus, high levels of sialylated glycoconjugates are present in the gastric mucosa of H. pylori infected individuals [Citation20]. The increased expression of sialyl-Lex and sialyl-Lea is due to transcriptional up-regulation of the B3GNT5, B3GALT5 and FUT3 genes [Citation21]. Thus, enhanced adhesion of SabA positive H. pylori to the inflamed gastric epithelium is supported by the increased expression of high affinity SabA binding glycoconjugates, allowing bacterial persistence after successful colonization and establishment of gastric inflammation [Citation3].
The expression of SabA is highly variable and subject to regulation via phase variation in the promoter and coding regions, as well as repression by the two-component system ArsRS [Citation22–25]. Furthermore, sabA can recombine with its related allele sabB, and to some extent with hopQ [Citation26]. Thus, in the face of a changing gastric microenvironment with remodeling of the glycome, an enhanced SabA expression may allow H. pylori attachment, whereas down-regulation of SabA may allow escape from areas with strong host immune defenses.
In addition to mediating gastric adhesion, SabA is involved in the interplay between H. pylori and human erythrocytes and neutrophils [Citation27,Citation28]. Thus, SabA is the sialic acid-dependent hemagglutinin of H. pylori, as demonstrated by hemagglutination studies using sabA deletion mutants. Protease treatment of the erythrocytes showed that H. pylori-mediated hemagglutination mainly occurs through interactions with erythrocyte glycosphingolipids [Citation27].
In H. pylori induced gastritis a prominent feature is the infiltration of the gastric lamina propria by neutrophil granulocytes, and neutrophils are also seen permeating between the epithelial cells. In addition H. pylori are in direct contact with immune cells, including granulocytes, of the lamina propria in the majority of cases of gastritis and gastric cancer [Citation29]. Neutrophils are activated by H. pylori, leading to an oxidative burst reaction with release of reactive oxygen metabolites [Citation30], which over time leads to an accumulation of oxidative DNA damage [Citation31,Citation32]. Deletion of the neutrophil activating protein HP-NAP, the vacuolating cytotoxin VacA, or the BabA adhesin had no effect on H. pylori neutrophil activating capacity [Citation28]. However, deletion of the SabA adhesin gave significant defects in neutrophil activation, and the neutrophil adherence, phagocytosis and agglutination were also severely impaired. Preincubation of SabA-expressing H. pylori with sialylated oligosaccharides mirrored the effect caused by SabA deletion [Citation33]. Thus, H. pylori-induced activation of human neutrophils is initiated by the SabA-mediated binding to sialic acid-carrying neutrophil receptors.
Upon binding of H. pylori to the acid glycosphingolipids of human granulocytes and human stomach very similar binding patterns were obtained, which might lead to the suspicion that the minor H. pylori binding gangliosides characterized were derived from infiltrating neutrophils, and not from the gastric epithelial cells. However, the ganglioside composition of human neutrophils is distinctly different from the human stomach gangliosides characterized here. The gangliosides of human neutrophils are a complex mixture [Citation34–37], and with the exception of the GM3 ganglioside, these gangliosides have one or several N-acetyllactosamine moieties, where one or more of the N-acetylglucosamines may be substituted with α3-linked fucose(s), and there are gangliosides with both α6- or α3-linked Neu5Ac. As stated above we did a targeted search for fucosylated gangliosides, as e.g. sialyl-LeX and dimeric-sialyl-LeX, in the LC-ESI/MS analyses. We also searched for gangliosides with α6-linked Neu5Ac, which may be readily identified by diagnostic 0,2X ions [Citation37]. However, none of these compounds could be identified among of the gangliosides from human stomach.
Interestingly, the crystal structures of both BabA and SabA have recently been reported [Citation38,Citation39]. Both adhesins have an ectodomain topology with seven α helices organized in a joined 4 + 3 helix bundle. In BabA there is also a 4-stranded β sheet insertion which is involved in Leb binding. This insertion is not present in SabA, and the location of the carbohydrate binding site in SabA has not been identified yet.
The distribution of Neu5Acα3-neolactohexaosylceramide and Neu5Acα3-neolactooctaosylceramide in the human stomach has not been investigated. However, several immunohistochemistry studies have demonstrated that ABH and Le blood group determinants based on type 1 core chain are present in the foveolar epithelium in the normal gastric mucosa, while ABH and Le blood group determinants based on type 2 core are found both in the foveolar epithelium and in deep gastric glands [Citation14,Citation40–43]. The precursor of Neu5Acα3-neolactohexaosylceramide and Neu5Acα3-neolactooctaosylceramide, i.e. the blood group i antigen (Galβ4GlcNAcβ3Galβ4GlcNAc) is mainly present in the gastric glands, but also found in the foveolar epithelium [Citation44].
The minor SabA binding gangliosides of human gastric mucosa might play different roles in different stages of H. pylori infection. During the initial colonization by H. pylori, which is primarily mediated by BabA, Neu5Acα3-neolactohexaosylceramide and Neu5Acα3-neolactooctaosylceramide might function as additional adhesion factors for SabA-expressing H. pylori. The H. pylori induced gastric inflammation leads to a transcriptional activation of the human FUT3 gene [Citation21], and Neu5Acα3-neolactohexaosylceramide and Neu5Acα3-neolactooctaosylceramide are targets for the FUT3 gene product Fuc-TIII, a fucosyltransferase capable of catalyzing addition of a fucose in the sialyl-LeX-characteristic α3-linkage, the last step in sialyl-LeX synthesis. Thus, Neu5Acα3-neolactohexaosylceramide and Neu5Acα3-neolactooctaosylceramide have a role in the increased expression of sialyl-LeX and related antigens, which H. pylori can exploit for binding to the inflamed gastric mucosa.
The identification of the minor SabA binding gangliosides of the human gastric mucosa, Neu5Acα3-neolactohexaosylceramide and Neu5Acα3-neolactooctaosylceramide may further contribute to our understanding of the molecular mechanisms by which H. pylori establish successful infections in human hosts, and could hopefully guide the development of novel and efficacious anti-adhesive therapies that specifically target this bacterium-host interaction.
Materials and methods
H. pylori strains, culture conditions and labeling
The generalist H. pylori strain J99, and the construction of the J99/SabA- mutant sabA::cam were described by Mahdavi et al [Citation3]. The construction of HP-NAP knock-out mutant, designated J99/NAP- (napA::kan) strain was described by Unemo et al [Citation28]. The wild type J99 strain was used since it binds to sialyl-LeX, dimeric-sialyl-LeX, sialyl-Lea and sialyl-lactosamine with similar affinities [Citation27].
For chromatogram binding experiments the bacteria were grown in a microaerophilic atmosphere at 37°C for 48 h on Brucella medium (Fisher Scientific; BD 211086) containing 10% fetal calf serum (Fisher Scientific; A15-101) inactivated at 56°C, and BBL IsoVitaleX Enrichment (Fisher Scientific; BD 211876). The mutant strains J99/SabA- and the J99/NAP- were cultured on the same medium supplemented with chloramphenicol (20 μg/ml). Bacteria were radiolabeled by the addition of 50 μCi 35S-methionine (PerkinElmer; NEG77207MC) diluted in 0.5 ml phosphate-buffered saline (PBS) pH 7.3, to the culture plates. After incubation for 12–72 h at 37°C under microaerophilic conditions, the bacteria were harvested, centrifuged three times and thereafter suspended to 1 × 108 CFU/ml in PBS. The specific activities of the suspensions were approximately 1 cpm per 100 H. pylori organisms.
Reference glycosphingolipids
Total acid and non-acid glycosphingolipid fractions were isolated as described [Citation45]. Individual glycosphingolipids were isolated by repeated chromatography on silicic acid columns and by HPLC, and identified by mass spectrometry [Citation46,Citation47], and 1H-NMR spectroscopy [Citation48].
Isolation of H. pylori binding gangliosides from human stomach
The isolation of total acid and total non-acid glycosphingolipids from human stomach was done during the 1970ies by the method described by Karlsson [Citation45]. The stomach was collected in 1974 at Sahlgrenska University Hospital, Göteborg, Sweden (before the hospital had an ethics committee). The stomach was dissected out at autopsy of an 84 year old patient who died of heart failure due to a myocardial abscess, and frozen at −70°C. The tissue was then lyophilized, and thereafter extracted in two steps in a Soxhlet apparatus with chloroform and methanol (2:1 and 1:9, by volume, respectively). The material thereby obtained was subjected to mild alkaline hydrolysis and dialysis, followed by separation on a silicic acid column. Acid and non-acid glycosphingolipid fractions were obtained by chromatography on a DEAE-cellulose column. In order to separate the non-acid glycosphingolipids from alkali-stable phospholipids, the non-acid fraction was acetylated and separated on a second silicic acid column, followed by deacetylation and dialysis. Final purifications were done by chromatographies on DEAE-cellulose and silicic acid columns. A total acid glycosphingolipid fraction of 59.7 mg was isolated from 31.7 g dry weight tissue. The glycosphingolipids were dissolved in chloroform/methanol (2:1, by volume), and kept at -20°C.
After the initial test for H. pylori binding, non-glycosphingolipid impurities in the total acid glycosphingolipid fraction were removed by chromatography on a silicic acid column eluted with increasing concentrations of methanol in chloroform. Thereby a fraction (40 mg) containing resorcinol positive compounds and a compound migrating in the sulfatide region on thin-layer plates was obtained. This fraction was separated on a 40 g Iatrobeads column (Iatron Laboratories Inc.; 6RS-8060) eluted with chloroform/methanol/water (65:24:4, by volume) 4 × 10 ml, chloroform/methanol/water (60:35:8, by volume) 5 × 10 ml, and chloroform/methanol/water (40:40:12, by volume) 10 × 10 ml, and finally chloroform/methanol/water (40:40:12, by volume) 40 ml. The fractions obtained were analyzed by thin-layer chromatography and anisaldehyde and resorcinol staining, and the H. pylori binding activity was assessed using the chromatogram binding assay (see below). The fractions were pooled according to the mobility on thin-layer chromatograms and their H. pylori binding activity. Thereby one fraction (5.6 mg) containing compounds migrating in the sulfatide region, one fraction (2.8 mg) with compounds migrating as the GM3 ganglioside, and one fraction (14 mg) containing slow migrating H. pylori binding gangliosides, were obtained.
The latter fraction was separated on a 15 g Iatrobeads column eluted in a similar manner. This resulted in six fractions (designated fractions S1-S6) containing resorcinol positive compounds migrating as Neu5Acα3-neolactotetraosylceramide and below. Pooling of the three H. pylori binding fractions S4-S6 gave 2.8 mg. This material was separated on a 2 g Iatrobeads column eluted with chloroform/methanol/water (60:35:8, by volume) 60 × 0.5 ml, and finally 10 ml. After pooling of the subfractions according to their mobility on thin-layer chromatograms and their H. pylori binding activity, three fractions were obtained (designated fraction SI, SII and SIII).
Isolation of acid glycosphingolipids from human gastric mucosa
The acid glycosphingolipids from human gastric mucosa were from a previous study of H. pylori binding non-acid glycosphingolipids [Citation49]. The materials used were stomach tissue (10 × 10-cm pieces) obtained from the fundus region from patients undergoing elective surgery for morbid obesity. After washing with 0.9% NaCl (w/v), the mucosal cells were gently scraped off and kept at −70°C. The material was lyophilized, and acid and non-acid glycosphingolipids were isolated as described [Citation49].
Thin-layer chromatography
Thin-layer chromatography was done on aluminum- or glass-backed silica gel 60 high performance thin-layer chromatography plates (Merck; 105641/105547). Glycosphingolipid mixtures (40 μg) or pure glycosphingolipids (1-4 μg) were applied to the plates, and eluted with chloroform/methanol/water (60:35:8, by volume) as solvent system. Chemical detection was done with anisaldehyde [Citation50], or the resorcinol reagent [Citation51].
Chromatogram binding assays
Binding of 35S-labeled H. pylori to glycosphingolipids on thin-layer chromatograms was done as previously reported [Citation10]. Dried chromatograms were dipped for 1 min in diethylether/n-hexane (1:5, by volume) containing 0.5% (w/v) polyisobutylmethacrylate (Sigma-Aldrich; 181544.). After drying, the chromatograms were soaked in PBS/BSA (PBS containing 2% bovine serum albumin (w/v), 0.1% NaN3 (w/v) and 0.1% Tween 20 (by volume)) for 2 h at room temperature. The chromatograms were subsequently covered with radiolabeled bacteria diluted in PBS/BSA (2-5 × 106 cpm/ml). Incubation was done for 2 h at room temperature, followed by repeated washings with PBS. The chromatograms were thereafter exposed to XAR-5 X-ray films (Carestream; 8941114) for 12 h.
The mouse monoclonal antibodies tested for binding to the acid glycosphingolipids in the chromatogram binding assay are given in . Binding of antibodies to glycosphingolipids separated on thin-layer chromatograms was performed as described [Citation52], using 125I-labeled monoclonal anti-mouse antibodies (DakoCytomation Norden A/S; Z0259) for detection.
Table 2. Monoclonal antibodies used in chromatogram binding assays.
Binding of 35S-labeled CS6 expressing E. coli, and 125I-labeled S. tuberosum lectin (Sigma-Aldrich; L7885) on thin-layer chromatograms was done as described [Citation18,Citation19].
Inhibition assay
To evaluate the potential blocking of H. pylori ganglioside binding, thin-layer chromatograms with separated glycosphingolipids were treated with polyisobutylmethacrylate and blocked with PBS/BSA, as described above. The chromatograms were then incubated with unlabeled S. tuberosum lectin (diluted to 50 μg/ml in PBS/BSA) for 2 h at room temperature, followed by repeated washings with PBS. The chromatograms were thereafter incubated with radiolabeled H. pylori J99, as described above.
LC-ESI/MS of glycosphingolipids
LC-ESI/MS of the native acid glycosphingolipids was done as described [Citation53]. The glycosphingolipids were dissolved in methanol:acetonitrile in proportion 75:25 (by volume) and separated on a 200 × 0.150 mm column, packed in-house with 5 μm polyamine II particles (YMC Europe GmbH; PB12S05). An autosampler, HTC-PAL (CTC Analytics AG) equipped with a cheminert valve (0.25 mm bore) and a 2 µl loop, was used for sample injection. An Agilent 1100 binary pump (Agilent technologies) delivered a flow of 250 µl/min, which was splitted down in an 1/16” microvolume-T (0.15 mm bore) (Vici AG International) by a 50 cm x 50 µm i.d. fused silica capillary before the injector of the autosampler, allowing approximately 2–3 µl/min through the column. Samples were eluted with an aqueous gradient (A:100% acetonitrile to B: 10 mM ammonium bicarbonate). The gradient (0-50% B) was eluted for 40 min, followed by a wash step with 100% B, and equilibration of the column for 20 min. The samples were analyzed in negative ion mode on a LTQ linear quadropole ion trap mass spectrometer (Thermo Electron), with an IonMax standard ESI source equipped with a stainless steel needle kept at –3.5 kV. Compressed air was used as nebulizer gas. The heated capillary was kept at 270°C, and the capillary voltage was –50 kV. Full scan (m/z 500–1800, two microscan, maximum 100 ms, target value of 30,000) was performed, followed by data-dependent MS2 scans (two microscans, maximun 100 ms, target value of 10.000) with normalized collision energy of 35%, isolation window of 2.5 units, activation q = 0.25 and activation time 30 ms). The threshold for MS2 was set to 500 counts. Data acquisition and processing were conducted with Xcalibur software (Version 2.0.7). Manual assignment of glycosphingolipid sequences was done with the assistance of the Glycoworkbench tool (Version 2.1), and by comparison of retention times and MS2 spectra of reference glycosphingolipids.
Abbreviations
HP-NAP | = | H. pylori neutrophil activating protein |
LC-ESI/MS | = | liquid chromatography/electrospray ionization mass spectrometry |
Disclosure of potential conflicts of interest
The authors report no conflict of interest.
1440171_zip.pdf
Download PDF (4.5 MB)Acknowledgements
The anti-Neu5Acα3-neolacto and anti-Neu5Acα6-neolacto antibodies were a kind gift from Dr. M. Blomqvist, Institute of Biomedicine, Department of Clinical Chemistry and Transfusion Medicine, The Sahlgrenska Academy at University of Gothenburg, Sweden.
Additional information
Funding
References
- Teneberg S. The multiple carbohydrate binding specificities of Helicobacter pylori. In: Lindhorst TK, Oscarson S, editors. Glycoscience and Microbial Adhesion/ Topics in Current Chemistry. Springer-Verlag, Berlin Heidelberg; 2009. p. 109–138.
- Ilver D, Arnqvist A, Ögren J, et al. Helicobacter pylori adhesin binding fucosylated histo-blood group antigens revealed by retagging. Science. 1998;279:373–7. doi:10.1126/science.279.5349.373. PMID:15273394.
- Mahdavi J, Sondén B, Hurtig M, et al. Helicobacter pylori SabA adhesin in persistent infection and chronic inflammation. Science. 2002;297:573–8. doi:10.1126/science.1069076. PMID:12142529.
- Rossez Y, Gosset P, Bonega IG, et al. The LacdiNAc-specific adhesin LabA mediates adhesion of Helicobacter pylori to human gastric mucosa. J Infect Dis. 2014;210:1286–95. doi:10.1093/infdis/jiu239. PMID:24755437.
- Königer V, Holsten L, Harrison U, et al. Helicobacter pylori exploits human CEACAMs via HopQ for adherence and translocation of CagA. Nat Microbiol. 2016;2:16188. doi:10.1038/nmicrobiol.2016.188. PMID:27748756.
- Javaheri A, Kruse T, Moonens K, et al. Helicobacter pylori adhesin HopQ engages in a virulence-enhancing interaction with human CEACAMs. Nat Microbiol. 2016;2:16189. doi:10.1038/nmicrobiol.2016.189. PMID: 27748768.
- Odenbreit S, Till M, Hofreuter D, et al. Genetic and functional characterization of the alpAB gene locus essential for the adhesion of Helicobacter pylori to human gastric tissue. Mol Microbiol. 1999;31:1537–48. doi:10.1046/j.1365-2958.1999.01300.x. PMID:10200971.
- Odenbreit S, Kavermann H, Püls J, et al. CagA tyrosine phosphorylation and interleukin-8 induction by Helicobacter pylori are independent from alpAB, HopZ and bab group outer membrane proteins. Int J Med Microbiol. 2002;292:257–66. doi:10.1078/1438-4221-00205. PMID:12398216.
- Dossumbekova A, Prinz C, Mages J, et al. Helicobacter pylori HopH (OipA) and bacterial pathogenicity: genetic and functional genomic analysis of hopH gene polymorphisms. J Infect Dis. 2006;194:1346–55. doi:10.1086/508426. PMID: 17054063.
- Roche N, Ångström J, Hurtig M, et al. Helicobacter pylori and complex gangliosides. Infect Immun. 2004;72:1519–29. doi:10.1128/IAI.72.3.1519-1529.2004. PMID:14977958.
- Teneberg S, Miller-Podraza H, Lampert HC, et al. Carbohydrate binding specificity of the neutrophil-activating protein of Helicobacter pylori. J Biol Chem. 1997;272:19067–71. doi:10.1074/jbc.272.30.19067. PMID: 9228091.
- Blom K, Lundin S, Bölin I, et al. Flow cytometric analysis of the localization of H. pylori antigens during different growth phases. FEMS Immunol Med Microbiol. 2001;30:173–9. doi:10.1111/j.1574-695X.2001.tb01567.x. PMID: 11335135.
- Sheu BS, Odenbreit S, Hung KH, et al. Interaction between host gastric Sialy-Lewis-X and H. pylori SabA enhances H. pylori density in patients lacking gastric Lewis B antigen. Am J Gastroenterol. 2006;101:36–44. doi:10.1111/j.1572-0241.2006.00358.x. PMID:16405531.
- Sakamoto S, Watanabe T, Tokumaru T, et al. Expression of Lewisa, Lewisb, Lewisx, Lewisy, siayl-Lewisa, and sialyl-Lewisx blood group antigens in human gastric carcinoma and in normal gastric tissue. Cancer Res. 1989;49:745–52. PMID:2910493.
- Madrid JF, Ballesta J, Castells MT, et al. Glycoconjugate distribution in the human fundic mucosa revealed by lectin- and glycoprotein-gold cytochemistry. Histochemistry. 1990;95:179–87. doi:10.1007/BF00266591. PMID:2081692.
- Murata K, Egami H, Shibata Y, et al. Expression of blood group-related antigens, ABH, Lewis(a), Lewis(b), Lewis(x), Lewis(y), CA19-9, and CSLEX1 in early cancer, intestinal metaplasia, and uninvolved mucosa of the stomach. Am J Clin Pathol. 1992;98:67–75. doi:10.1093/ajcp/98.1.67. PMID:1615929.
- Natomi H, Saitoh T, Sugano K, et al. Systematic analysis of glycosphingolipids in the human gastrointestinal tract: enrichment of sulfatides with hydroxylated longer-chain fatty acids in the gastric and duodenal mucosa. Lipids. 1993;28:737–42. doi:10.1007/BF02535996. PMID:8377590.
- Jansson L, Tobias J, Jarefjäll C, et al.. Sulfatide recognition by colonization factor antigen CS6 from enterotoxigenic Escherichia coli. PLoS ONE. 2009;4:e4487. doi:10.1371/journal.pone.0004487. PMID:19242561.
- Ciopraga J, Ångström J, Bergström J, et al. Isolectins from Solanum tuberosum with different detailed carbohydrate binding specificities: Unexpected recognition of lactosylceramide by N-acetyllactosamine-binding lectins. J Biochem. 2000;128:855–867. doi:10.1093/oxfordjournals.jbchem.a022824. PMID:11056399.
- Ota H, Nakayama J, Momose M, et al. Helicobacter pylori infection produces reversible glycosylation changes to gastric mucins. Virchows Arch. 1998;433:419–26. doi:10.1007/s004280050269. PMID:9849856.
- Magalhaes A, Marcos-Pinto R, Nairn AV, et al. Helicobacter pylori chronic infection and mucosal inflammation switches the human gatric glycosylation pathways. Biochim Biophys Acta. 2015;1852:1928–1939. doi:10.1016/j.bbadis.2015.07.001. PMID:26144047.
- Goodwin AC, Weinberger DM, Ford CB, et al. Expression of the Helicobacter pylori adhesin SabA is controlled via phase variation and the ArsRS signal transduction system. Microbiology. 2008;154:2231–40. doi:10.1099/mic.0.2007/016055-0. PMID:18667556.
- Kao CY, Sheu SM, Sheu BS, et al. Length of thymidine homopolymeric repeats modulates promoter activity of sabA in Helicobacter pylori. Helicobacter. 2012;17:203–9. doi:10.1111/j.1523-5378.2012.00936.x. PMID:22515358.
- Åberg A, Gideonsson P, Vallström A, et al. A repetitive DNA element regulates expression of the Helicobacter pylori sialic acid binding adhesin by a rheostat-like mechanism. PLoS Pathog. 2014;10:e1004234. doi:10.1371/journal.ppat.1004234. PMID:24991812.
- Harvey VC, Acio CR, Bredehoft AK, et al. Repetitive sequence variations in the promoter region of the adhesin-encoding gene sabA of Helicobacter pylori affect transcription. J Bacteriol. 2014;196:3421–9. doi:10.1128/JB.01956-14. PMID:25022855.
- Talarico S, Whitefeld SE, Fero J, et al. Regulation of Helicobacter pylori adherence by gene conversion. Mol Microbiol. 2012;84:1050–61. doi:10.1111/j.1365-2958.2012.08073.x. PMID:22519812.
- Aspholm-Hurtig M, Olfat FO, Nordén J, et al. SabA is the H. pylori hemagglutinin and is polymorphic in binding to sialylated glycans. PLoS Pathog. 2006;2:989–1001. PMID:17121461.
- Unemo M, Aspholm-Hurtig M, Ilver D, et al. The sialic acid binding SabA adhesin of Helicobacter pylori is essential for nonopsonic activation of human neutrophils. J Biol Chem. 2005;280:15390–7. doi:10.1074/jbc.M412725200. PMID:15689619.
- Necchi V, Candusso ME, Tava F, et al. Intracellular, intercellular, and stromal invasion of gastric mucosa, preneoplastic lesions, and cancer by Helicobacter pylori. Gastroenterology. 2007;132:1009–23. doi:10.1053/j.gastro.2007.01.049. PMID:17383424.
- Rautelin H, Blomberg B, Fredlund H, et al. Incidence of Helicobacter pylori strains activating neutrophils in patients with peptic ulcer disease. Gut. 1993;34:599–603. doi:10.1136/gut.34.5.599. PMID:8504958.
- Baik SC, Youn HS, Chung MH, et al. Increased oxidative DNA damage in Helicobacter pylori-infected human gastric mucosa. Cancer Res. 1996;56:1279–82. PMID:8640814.
- Handa O, Naito Y, Yoshikawa T. Helicobacter pylori: a ROS-inducing bacterial species in the stomach. Inflamm Res. 2010;59:997–1003. doi:10.1007/s00011-010-0245-x. PMID:20820854.
- Teneberg S, Jurstrand M, Karlsson K, et al. Inhibition of Helicobacter pylori-induced activation of human neutrophils by sialylated oligosaccharides. Glycobiology. 2000;10:1171–81. doi:10.1093/glycob/10.11.1171. PMID:11087709.
- Stroud MR, Handa K, Salyan ME, et al.Monosialogangliosides of human myelogenous leukemia HL60 cells and normal human leukocytes. 1. Separation of E-selectin binding from nonbinding gangliosides, and absence of sialosyl-Lex having tetraosyl to octaosyl core. Biochemistry. 1996;35:758–69. doi:10.1021/bi951600r. PMID:8547256.
- Stroud MR, Handa K, Salyan ME, et al. Monosialogangliosides of human myelogenous leukemia HL60 cells and normal human leukocytes. 2. Characterization of E-selectin binding fractions, and structural requirements for physiological binding to E-selectin. Biochemistry. 1996;35:770–8. doi:10.1021/bi952461g. PMID:8547257.
- Metelmann W, Peter-Katalinic j, Müthing J. Gangliosides from human granulocytes: A nano-ESI QTOF mass spectrometry fucosylation study of low abundance species in complex mixtures. J Am Soc Mass Spectrom. 2001;12:964–73. doi:10.1016/S1044-0305(01)00276-8. PMID:11506229.
- Meisen I, Peter-Katalinic j, Müthing J. Discrimination of neolacto-series gangliosides with alpha2-3- and alpha2-6-linked N-acetylneuraminic acid by nanoelectrospray ionization low-energy collision-induced dissociation tandem quadropole TOF MS. Anal Chem. 2003;75:5719–25. doi:10.1021/ac0347617. PMID: 14588011.
- Moonens K, Gideonsson P, Subedi S, et al. Structural insights into polymorphic ABO glycan binding by Helicobacter pylori. Cell Host Microbe. 2016;19:55–66. doi:10.1016/j.chom.2015.12.004. PMID:26764597.
- Pang SS, Nguyen ST, Perry AJ, et al. The three-dimensional structure of the extracellular adhesion domain of the sialic acid-binding adhesin SabA from Helicobacter pylori. J Biol Chem. 2014;289:6332–40. doi:10.1074/jbc.M113.513135. PMID:4375407.
- Mollicone R, Bara J, Le Pendu J, Oriol R. Immunological pattern of type 1 (Lea, Leb) and type 2 (X, Y, H) blood group-related antigens in the human pyloric and duodenal mucosae. Lab Invest. 1985;53:219–27. PMID: 2410664.
- Mollicone R, Le Pendu J, Bara J, Oriol R. Heterogeneity of the ABH antigenic determinants expressed in human pyloric and duodenal mucosae. Glycoconj J. 1986;3:187–202. doi:10.1007/BF01049376.
- Torrado J, Blasco E, Cosme A, et al. Expression of type 1 and type 2 blood group-related antigens in normal and neoplastic gastric mucosa. Am J Clin Pathol. 1989;91:249–54. doi:10.1093/ajcp/91.3.249. PMID: 2923092.
- Murata K, Egami H, Shibata Y, et al. Expression of blood group-related antigens, ABH, Lewis(a), Lewis(b), Lewis(x), Lewis(y), CA19-9, and CSLEX1 in early cancer, intestinal metaplasia, and uninvolved mucosa of the stomach. Am J Clin Pathol. 1992;98:67–75. doi:10.1093/ajcp/98.1.67. PMID: 1615929.
- Kapadia A, Feizi T, Jewell D, et al. Immunocytochemical studies of blood group A, H, I, and i antigens in gastric mucosae of infants with normal gastric histology and of patients with gastric carcinoma and chronic benign peptic ulceration. J Clin Pathol. 1981;34:320–37. doi:10.1136/jcp.34.3.320. PMID: 7014654.
- Karlsson KA. Preparation of total non-acid glycolipids for overlay analysis of receptors for bacteria and viruses and for other studies. Meth Enzymol. 1987;138:212–20. doi:10.1016/0076-6879(87)38018-8. PMID:3600323.
- Samuelsson BE, Pimlott W, Karlsson KA. Mass spectrometry of mixtures of intact glycosphingolipids. Meth Enzymol. 1990;193:623–46. doi:10.1016/0076-6879(90)93442-N. PMID:3600323.
- Karlsson H, Halim A, Teneberg S. Differentiation of glycosphingolipid-derived glycan structural isomers by liquid chromatography-mass spectrometry. Glycobiology. 2010;20:1103–16. doi:10.1093/glycob/cwq070. PMID:20466655.
- Koerner Jr TAW, Prestegard JH, Demou PC, et al. High-resolution proton NMR studies of gangliosides. 1. Use of homonuclear spin-echo J-correlated spectroscopy for determination of residue composition and anomeric configurations. Biochemistry. 1983;22:2676–87. doi:10.1021/bi00280a014. PMID:6871155.
- Teneberg S, Leonardsson I, Karlsson H, et al. Lactotetraosylceramide, a novel glycosphingolipid receptor for Helicobacter pylori, present in human gastric epithelium. J Biol Chem (.2002;).277:19709–19. doi:10.1074/jbc.M201113200. PMID:11914375.
- Waldi D. Sprühreagentien für die dünnschicht-chromatographie In: Stahl E, editor. Dünnschicht-Chromatographie. Springer-Verlag; 1962. p. 496–515.
- Svennerholm L, Fredman P. A procedure for the quantitative isolation of brain gangliosides. Biochim Biophys Acta. 1980;617:97–109. doi:10.1016/0005-2760(80)90227-1. PMID:7353026.
- Barone A, Säljö K, Benktander, J, et al. Sialyl-lactotetra: a novel cell surface marker of undifferentiated human pluripotent stem cells. J Biol Chem. 2014;289:18846–59. doi:10.1074/jbc.M114.568832. PMID:24841197.
- Johansson MM, Dedic B, Lundholm K, et al. Characterization of moose intestinal glycosphingolipids. Glycoconj J. 2015;32:393–412. doi:10.1007/s10719-015-9604-8. PMID:26104834.
- Nilsson O, Månsson JE, Lindholm L, et al. Sialosyllactotetraosylceramide, a novel ganglioside antigen detected in human carcinomas by a monoclonal antibody. FEBS Lett. 1985;182:398–402. doi:10.1016/0014-5793(85)80341-0. PMID:2984045.
- Nilsson O, Lindholm L, Holmgren J, et al. Monoclonal antibodies raised against NeuAcα2-6neolactotetraosylceramide detect carcinoma-associated gangliosides. Biochim Biophys Acta. 1985;835:577–83. doi:10.1016/0005-2760(85)90127-4. PMID:2410031.