ABSTRACT
To investigate the role of adrenergic signalling (AS) in the host immune response and Porphyromonas gingivalis virulence, we compared norepinephrine (NE) and isoproterenol (ISO) responses in Galleria mellonella. P. gingivalis infection was evaluated by survival; humoral immune responses (i.e. melanization and cecropin and gloverin mRNA expression); cellular immune responses (i.e. haemocyte count, nodulation by histology); and P. gingivalis recovery (CFU/mL). P. gingivalis was cultivated in the presence of ISO (PgISO) or NE and injected into the larvae for survival evaluation. Finally, we co-injected ISO and PgISO to evaluate the concomitant effects on the immune response and bacterial virulence. None of the ligands were toxic to the larvae; ISO increased haemocyte number, even after P. gingivalis infection, by mobilizing sessile haemocytes in a β-adrenergic-specific manner, while NE showed the opposite effect. ISO treatment reduced larval mortality and the number of recovered bacteria, while NE increased mortality and showed no effect on bacterial recovery. ISO and NE had similar effects on melanization and decreased the expression of cecropin. Although co-cultivation with NE and ISO increased the gene expression of bacterial virulence factors in vitro, only the injection of PgISO increased larval death, which was partially reversed by circulating ISO. Therefore, α- and β-adrenergic signalling had opposite effects after P. gingivalis infection. Ultimately, the catecholamine influence on the immune response overcame the effect of more virulent strains. The effect of AS directly on the pathogen found in vitro did not translate to the in vivo setting.
Introduction
Stress contributes to the development and progression of various diseases due to the activation of several responses, including those of the sympathetic nervous system (SNS). SNS acts through α- and β-adrenergic receptors (AR), which are activated by the catecholamines epinephrine and norepinephrine (NE). Periodontitis, a chronic inflammatory disease of the supporting tissues of teeth, and the main cause of tooth loss in adults worldwide , is affected by SNS on its two pathogenesis pillars: host immune response and the microbiota [Citation1,Citation2].
Regarding the host response, the activation of the β-AR signalling leads to an increase in bone loss in rats with periodontitis [Citation3], resulting in a pro-inflammatory profile [Citation4,Citation5] and decreased periodontal fibroblast proliferation [Citation6]. Conversely, the blockage of the SNS by sympathectomy or propranolol (a β-AR blocker) inhibits osteoclastic differentiation and stress-induced interleukin (IL)-1β, IL-6, and IL-8 release, resulting in decreased bone loss [Citation5,Citation7–9].
In the microbiota, catecholamines have been shown to exhibit in vitro activity in some microorganisms. Epinephrine and NE interfere with the growth of some periodontopathogenic bacteria such as Fusobacterium nucleatum and Tannerella forsythia [Citation10–14], while also increasing the virulence of other bacteria such as Porphyromonas gingivalis, the main pathogen associated with chronic periodontitis [Citation15Citation16,Citation17]. NE increases the expression of gingipain (rgpb), an important virulence factor of P. gingivalis [Citation16,Citation17]. This action can be reversed by the administration of propranolol, suggesting that a catecholaminergic receptor is expressed in the pathogen [Citation18]. Although the current literature shows a direct action of catecholamines on P. gingivalis, the high concentrations used in these studies are not comparable to the concentrations in physiological or stress conditions, making the translation from bench to bedside a challenge.
Even though in vitro studies show the potential influence of adrenergic signalling directly on the main pathogen of periodontitis [Citation16–18], and studies in rodents and humans highlight the deleterious impact of stress in periodontal disease, the complexity of the disease and the models used make it virtually impossible to study the SNS response alone. Thus, less complex models that still present an analogous response to bacterial infections, such as invertebrate models [Citation19], are ideal to reveal the impact of the adrenergic system (AS) on the host immune response and pathogen virulence separately .
Galleria mellonella, a lepidopteran insect, has been widely used to study pathogen/host interactions owing to its many advantages. In the larval stage, it can be maintained at 37 °C, a temperature ideal for most human pathogens; it is also easy to handle, allows for the controlled inoculation of microorganisms, and its immune system resembles that of rodents and humans. Previous studies have shown that G. mellonella is a useful model for studying P. gingivalis infection [Citation20–24]. Moreover, this insect produces octopamine (OCT), a hormone that structurally resembles NE [Citation25,Citation26].
Thus, to better understand the effects of the AS in the host immune response and the microbiota, specifically P. gingivalis, we investigated the systemic effects of isoproterenol (ISO) and NE at different concentrations (from low to high concentrations) in P. gingivalis infection outcomes using G. mellonella. Furthermore, we investigated the direct impact of ISO/NE on P. gingivalis virulence to verify the importance of SNS action on the bacteria versus that on the model’s (G. mellonella) immune system.
Results
ISO, NE, and OCT toxicity and cellular immune profiling
In Galleria mellonella, there was no to very limited toxicity across all tested concentrations, over a 5-day period, as compared with the PBS control group, for all three compounds (S1 appendix A – C).
Regarding circulating haemocytes, NE caused a decrease in haemocyte counts at a high concentration (1 µM), peaking at 30 min (). In contrary, ISO caused an increase in haemocyte count, with an inverse U-shaped concentration–response curve, peaking at 100 pM also at 30 min (). Interestingly, the results with 100 pM ISO were similar to the OCT endogenous hormone response (S2 appendix).
Figure 1. Z-Score-Normalized haemocyte counts at 30 (a), 90 (b), and 180 (c) minutes post-injection of several norepinephrine (NE) concentrations in G. mellonella larvae. Box plots represent z-normalized haemocyte counts for n = 20 larvae per concentration. NE decreases the number of circulating haemocytes at 1 µM (Kruskal–Wallis test, p < 0.0001) after 30 minutes. * Significantly different (p < 0.05) compared with the phosphate-buffered saline (PBS) group.
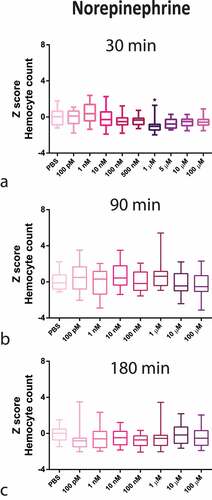
Figure 2. Z-Score-Normalized haemocyte counts at 30 (a), 90 (b), and 180 (c) minutes post-injection of a wide concentration range of isoproterenol (ISO) in G. mellonella larvae. Box plots represent z-normalized haemocyte counts for n = 20 larvae per concentration. ISO increases the number of haemocytes at 100 pM and 500 pM (Kruskal–Wallis test, p < 0.0001) after 30 min. * Significantly different (p < 0.05) compared with the phosphate-buffered saline (PBS) group.
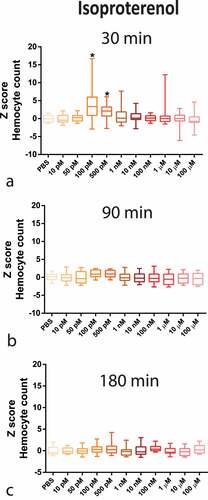
The complete time–concentration matrix for ISO and NE ( and highlights the transient time response (30 min peak) to adrenergic stimuli with a narrow window of concentration dependency, indicating a highly tunable response.
Systemic influence of adrenergic signalling (NE and ISO) in G. mellonella survival during P. gingivalis infection
ISO and NE influence on survival of G. mellonella during P. gingivalis infection
P. gingivalis injection killed 92.5% of larvae in 5 days, whereas co-injection with NE led to 100% larval death in only 2 days; this difference being statistically significant (). Opposite to NE response, there was a pro-survival effect of 100 pM ISO on the larvae co-injected with P. gingivalis, where the proportion of dead larvae was reduced to 45% by ISO after 5 days ().
Figure 3. (a) Survival curve over 5 days of concomitant injection of 1 µM NE and 108 CFU/mL P. gingivalis (n = 20 larvae per group, Kaplan–Meier plot, Log-rank [Mantel-cox] p = 0.0109). (b) Survival curve after concomitant administration of 100 pM ISO and P. gingivalis (n = 20 larvae per group, Kaplan–Meier plot, log-rank [Mantel-cox] p = 0.0099). Same lower-case letters close to the graph lines/bars represent absence of significant differences.
![Figure 3. (a) Survival curve over 5 days of concomitant injection of 1 µM NE and 108 CFU/mL P. gingivalis (n = 20 larvae per group, Kaplan–Meier plot, Log-rank [Mantel-cox] p = 0.0109). (b) Survival curve after concomitant administration of 100 pM ISO and P. gingivalis (n = 20 larvae per group, Kaplan–Meier plot, log-rank [Mantel-cox] p = 0.0099). Same lower-case letters close to the graph lines/bars represent absence of significant differences.](/cms/asset/bdfdbd35-4302-47e8-9b8c-a0c198db6370/kvir_a_2123302_f0003_oc.jpg)
P. gingivalis recovery from the haemolymph
To investigate if the phenotypes observed in the survival curve analysis were correlated with bacterial load in the insect haemolymph, we measured the CFU/mL of P. gingivalis 30 min post-infection. NE treatment did not influence P. gingivalis recovery from the larvae haemolymph, since the P. gingivalis + NE group presented similar bacterial counts to the P. gingivalis control group ().
Figure 4. P. gingivalis recovery from the haemolymph of larvae infected with P. gingivalis ±1 µM NE (n = 10 pools of three larvae per group, mean and standard deviation, t-test, p = 0.1306) measured as CFU/mL (a) and P. gingivalis ±100 pM ISO (n = 10 pools of three larvae per group, mean and standard deviation, t-test, p = 0.0357) (b).
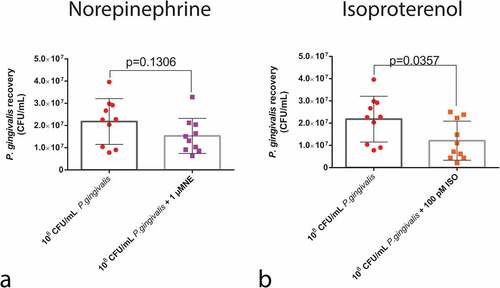
However, the larvae that received ISO + P. gingivalis had a reduction in the number of P. gingivalis cells recovered from the haemolymph (). These results highlight the fact that ISO treatment is capable of rapidly reducing the number of live bacteria post-infection.
Quantification of melanization and antimicrobial peptides
We colorimetrically measured the levels of larval melanization at 30 min and antimicrobial peptides (AMP) gene expression 24 hours post-injection to determine whether the changes in survival caused by NE/ISO were influenced by the humoral immune response. Regarding melanization, neither compound influenced it when compared with P. gingivalis alone group (). However, concerning AMP production, P. gingivalis infection increased the expression of cecropin and gloverin by 49 and 4.6-fold, respectively. Co-infection with both NE and ISO decreased the expression of cecropin when compared with the P. gingivalis group ().
Figure 5. Humoral response of larvae to P. gingivalis infection. Colorimetric measurement of melanization for (a) NE (n = 20 larvae per group, Box plot, Kruskal–Wallis test, p < 0.0001) and (c) ISO (n = 20 larvae per group, Box plot, Kruskal–Wallis test, p < 0.0001). qPCR analysis of gloverin and cecropin mRNA expression 24 h after infection and NE administration (b) (gloverin: n = 8 larvae for PBS group and n = 10 larvae for P. gingivalis +/- 1 µM NE; Kruskal–Wallis test, p < 0.0001; cecropin : n = 10 larvae for PBS and P. gingivalis and n = 9 for P. gingivalis + 1 µM NE, Kruskal–Wallis test, p = 0.0024). (d) P. gingivalis +/- ISO (gloverin: n = 8 for PBS group, and n = 10 for P. gingivalis and P. gingivalis+ 100 pM ISO groups, Box plot, Kruskal–Wallis test, p < 0.0001; cecropin: n = 10 larvae per group, Kruskal–Wallis test, p = 0.0024). Same lower-case letters close to the graph lines/bars represent an absence of significant differences. * Significantly different compared with the PBS group.
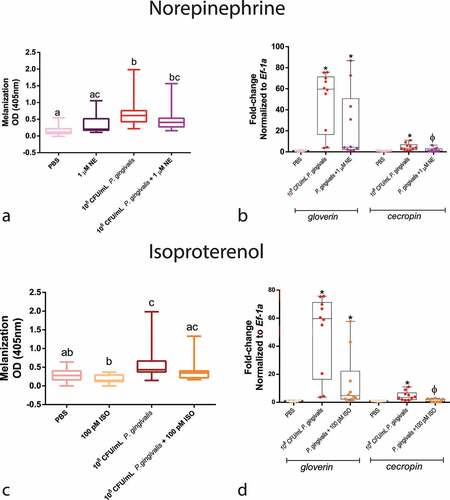
Haemocyte density
As shown earlier (), in the absence of infection, NE decreased the number of circulating haemocytes, which was also observed upon co-injection with P. gingivalis (), even though without reaching statistical significance from P. gingivalis alone group.
Figure 6. Haemocyte count. Upper panels: Z-score-normalized haemocyte counts measured after P. gingivalis ±1 µM NE compared to PBS group (n = 20 larvae per group, mean and standard deviation, ANOVA, p < 0.0001) (a) and ±100pM ISO (n = 20 larvae per group, mean and standard deviation, ANOVA, p < 0.0001) (b). Same lower case letters close to the graph lines/bars represent the absence of significant differences. Bottom panels: representative image of an H&E-stained sagittal section of PBS or ISO (100 pM) injected larvae. Image shows the sessile haemocytes adhering to the fat body (FB), muscle (M), and trachea (T) (yellow arrowhead) and circulating haemocytes in the haemolymph (H) (orange arrowhead) (scale bar: 100 µm in the upper images and 40 µm in the insets). (c) Z-score-normalized haemocyte counts from haemolymph and fat body/adjacent organs in larvae that received PBS or 100pM ISO ± propranolol [10 µM] (n = 20 larvae per group, heatmap, ANOVA, p < 0.0001). * Significantly different compared with the PBS group (on haemolymph). Φ Significantly different compared with the PBS group (on fat body/adjacent organs).
![Figure 6. Haemocyte count. Upper panels: Z-score-normalized haemocyte counts measured after P. gingivalis ±1 µM NE compared to PBS group (n = 20 larvae per group, mean and standard deviation, ANOVA, p < 0.0001) (a) and ±100pM ISO (n = 20 larvae per group, mean and standard deviation, ANOVA, p < 0.0001) (b). Same lower case letters close to the graph lines/bars represent the absence of significant differences. Bottom panels: representative image of an H&E-stained sagittal section of PBS or ISO (100 pM) injected larvae. Image shows the sessile haemocytes adhering to the fat body (FB), muscle (M), and trachea (T) (yellow arrowhead) and circulating haemocytes in the haemolymph (H) (orange arrowhead) (scale bar: 100 µm in the upper images and 40 µm in the insets). (c) Z-score-normalized haemocyte counts from haemolymph and fat body/adjacent organs in larvae that received PBS or 100pM ISO ± propranolol [10 µM] (n = 20 larvae per group, heatmap, ANOVA, p < 0.0001). * Significantly different compared with the PBS group (on haemolymph). Φ Significantly different compared with the PBS group (on fat body/adjacent organs).](/cms/asset/8c41cc3a-3f9b-4ba4-aaef-c8513a9fcab8/kvir_a_2123302_f0006_oc.jpg)
Differently, the larvae that received P. gingivalis together with ISO had a bacterial-induced decrease in haemocyte counts that was reversed to PBS levels (). Analysing circulating (in the haemolymph) and sessile haemocytes (from the fat body and adjacent organs – e.g. dorsal tube), from the same larvae, we demonstrated that the increase in circulating haemocytes corresponded to a comparable decrease in sessile haemocytes from the internal organs, clearly showing that ISO directly affects the release of sessile haemocytes (). This can also be seen in the histology sections (highlighted in the inserts of ) and has been quantified in the corresponding heatmap (). The β-specific release of haemocytes from internal organs was also observed for the endogenous hormone OCT (S3 appendix). We validated that the ISO/OCT-induced increase in circulating haemocytes was dependent upon the β-AR signalling by co-injecting the larvae with the β-AR-specific antagonist propranolol. As expected, propranolol completely blocked the ISO/OCT-induced increase in the circulating haemocyte count (, S3 appendix).
Nodule formation
As one of the hallmarks of the cellular response to bacteria is nodule formation, we performed a histological analysis of the infected larvae. NE did not lead to any difference in nodulation between the groups, in concordance with the P. gingivalis recovery assay (). On the other hand, ISO treatment decreased nodulation when compared with the P. gingivalis group ().
Figure 7. Effects of adrenergic signalling on nodulation. (Top) Representative figures of larvae injected with P. gingivalis after 30 min, showing nodules formed around the fat body, muscles, and trachea (H&E staining). GI = gastrointestinal tract, FB = Fat body, M = muscle, T = trachea, H = haemolymph. (Bottom) No differences observed between P. gingivalis and P. gingivalis +1 µm NE regarding number of nodules counted on histological sections (n = 10 larvae per group, three sections per larvae, mean and standard deviation, t-test, p = 0.0851) (a). Decrease in the number of nodules in the P. gingivalis +100 pM ISO (n = 10 larvae per group, 3 sections per animal, mean and standard deviation, t-test, p = 0.0021) (b).
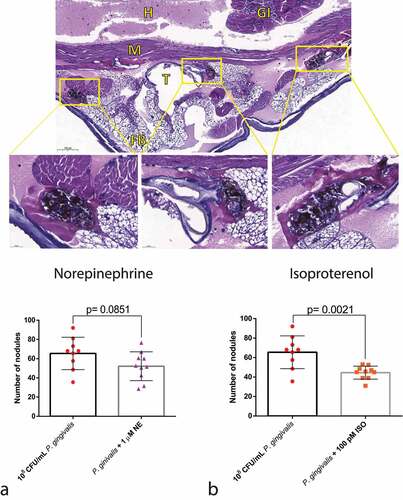
Direct influence of adrenergic signalling (NE and ISO) on P. gingivalis virulence and infection outcome in G. mellonella
Co-culture of P. gingivalis with NE (α-AR)
Although co-culture with NE increased the gene expression of gingipain (rgbp) and fimbriae (fimA) in vitro (), it did not change the infection outcome in vivo () leading to no difference in survival when compared to P. gingivalis.
Figure 8. Direct action of adrenergic signalling on P. gingivalis. rgpb and fimA gene expression after co-culturing P. gingivalis ±1 µM or 100 µM NE (rgbp, n = 6 biological replicates per group, ANOVA, p < 0.0001; fimA, n = 6 biological replicates per group, mean and standard-deviation, ANOVA, p < 0.0001) (a). Co-culture of P. gingivalis with 1 µM or 100 µM NE had no effect on larvae survival 24 h after infection (n = 10 larvae for PBS group, n = 45 larvae for P. gingivalis group, and n = 20 larvae for PgNE 1 µM and PgNE 100 µM groups, Kaplan–Meier plot, log-rank [Mantel-cox] p = 0.0005) (b). Same lower-case letters close to the graph bars/lines represent an absence of significant difference.
![Figure 8. Direct action of adrenergic signalling on P. gingivalis. rgpb and fimA gene expression after co-culturing P. gingivalis ±1 µM or 100 µM NE (rgbp, n = 6 biological replicates per group, ANOVA, p < 0.0001; fimA, n = 6 biological replicates per group, mean and standard-deviation, ANOVA, p < 0.0001) (a). Co-culture of P. gingivalis with 1 µM or 100 µM NE had no effect on larvae survival 24 h after infection (n = 10 larvae for PBS group, n = 45 larvae for P. gingivalis group, and n = 20 larvae for PgNE 1 µM and PgNE 100 µM groups, Kaplan–Meier plot, log-rank [Mantel-cox] p = 0.0005) (b). Same lower-case letters close to the graph bars/lines represent an absence of significant difference.](/cms/asset/874d8dc5-a921-4b88-b554-74163e29cd7e/kvir_a_2123302_f0008_oc.jpg)
Co-culture of P. gingivalis with ISO (β-AR)
ISO influenced the gene expression of gingipain (rgbp) and fimbriae (fimA) in vitro and changed the phenotype in vivo (). ISO-grown P. gingivalis (PgISO) was more toxic to the larvae than P. gingivalis at both concentrations tested in the first 24 h post-injection, starting to kill the larvae within the first few hours ().
Figure 9. Direct action of β-adrenergic signalling on P. gingivalis. A) rgbp and fimA gene expression after co-culturing P. gingivalis ±100pM or 100 µM ISO (rgbp, n = 6 biological replicates per group, mean and standard deviation, ANOVA, p < 0.0001; fimA, n = 6 biological replicates per group, ANOVA, p = 0.0131) (a). Co-culturing P. gingivalis with 100 pM and 100 µM ISO increased the mortality of the larvae 24 h after infection (n = 10 larvae for PBS group, n = 45 larvae for P. gingivalis group, n = 20 for PgISO 100 pM and PgISO 100 µm and 100 pM ISO + PgISO 100 pM groups, Kaplan–Meier plot, log-rank [Mantel-cox] p < 0.0001) (b). The systemic administration of 100 pM ISO could partially reverse the increased PgISO 100pM phenotype (c). Same lower-case letters close to the graph bars/lines represent an absence of significant difference.
![Figure 9. Direct action of β-adrenergic signalling on P. gingivalis. A) rgbp and fimA gene expression after co-culturing P. gingivalis ±100pM or 100 µM ISO (rgbp, n = 6 biological replicates per group, mean and standard deviation, ANOVA, p < 0.0001; fimA, n = 6 biological replicates per group, ANOVA, p = 0.0131) (a). Co-culturing P. gingivalis with 100 pM and 100 µM ISO increased the mortality of the larvae 24 h after infection (n = 10 larvae for PBS group, n = 45 larvae for P. gingivalis group, n = 20 for PgISO 100 pM and PgISO 100 µm and 100 pM ISO + PgISO 100 pM groups, Kaplan–Meier plot, log-rank [Mantel-cox] p < 0.0001) (b). The systemic administration of 100 pM ISO could partially reverse the increased PgISO 100pM phenotype (c). Same lower-case letters close to the graph bars/lines represent an absence of significant difference.](/cms/asset/1ccdc8c5-1717-49e2-bc53-8d2455265ccf/kvir_a_2123302_f0009_oc.jpg)
The interesting observation that ISO could behave differently if it was acting systemically on the larvae or directly on the bacterial environment prompted us to test whether the larvae pro-survival effects of circulating ISO could overcome the increased virulence of ISO-grown P. gingivalis. For this purpose, we co-injected G. mellonella with 100 pM ISO and 109 CFU/mL ISO (100 pM)-grown P. gingivalis and observed them hourly for 24 h. As shown in circulating ISO was indeed able to partially reverse the toxicity of the more virulent PgISO, indicating that the systemic effects of the β-adrenergic signalling are sufficient to counteract the toxicity of bacteria with higher virulence.
Discussion
In this study, we validated the adrenergic signalling response in G. mellonella haemocytes using known chemicals that bind to different ARs in higher organisms: ISO, a β-AR agonist, and NE, a preferential α-AR agonist. Haemocytes are insect cells analogous to neutrophils and macrophages that have been shown to express adrenergic-like receptors [Citation27]. We showed that these receptors are functional, as the administration of either ligand influenced the cellular response in the larvae in a concentration- and time-dependent manner. Interestingly, the effect was time-restricted, peaking at 30 min post-injection (similar to OCT [Citation28,Citation29], supplementary figure S2) and returning to basal levels within the first hour. Since this is the first study to analyse the effects of ISO and NE on G. mellonella haemocytes, we can only correlate the findings with OCT observations. Therefore, the time points were chosen based on the literature for invertebrates regarding OCT, the insect counterpart of NE. OCT is released 15 min to 1 hour after diverse stimuli before returning to basal levels [Citation28,Citation29]. In addition, OCT and its downstream pathways (e.g PKA) influence haemocyte number and activity 20–30 minutes post injection of compounds or microorganism challenge [Citation30–32]. Regarding concentration, some experiments showed increased haemocyte density at higher OCT concentrations (50–500 nM) and a decreased density at lower concentrations (5 nM) [Citation30]. In contrast, an injection with 1 mM OCT increased haemocyte count in crickets [Citation28], while OCT administration at ≤1000 pM mediated a transient upregulation of haemocyte count in shrimp (Litopenaeus vannamei) [Citation33]. Different concentrations have been used to explain the observed OCT-dependent effects on immune function. In this study, we observed that OCT acted more like a β-AR agonist at lower concentrations (ISO-like) and as an α-AR agonist at higher concentrations (NE-like). In other invertebrates, β-AR signalling (ISO) has also been linked to increased haemocyte count [Citation34,Citation35], while α-AR signalling (NE) to decreased haemocyte count and increased death rate [Citation36].
In invertebrates, an increase in circulating haemocytes has already been pointed out after stimulation and proposed to be due to the mobilization of these cells from the internal organs, especially the fat body, dorsal tube, and gastrointestinal tract [Citation37]. In vertebrates, catecholamines also increase the number of circulating NK cells and granulocytes in response to stressors [Citation38]. Herein, we show, for the first time, that the adrenergic response in G. mellonella is responsible for the swift (30 min) mobilization of haemocytes in a β-AR-specific manner. The specific intracellular pathways responsible for the mobilization and activation of haemocytes by β-AR are still under investigation. However, the protein kinase A and cyclic adenosine monophosphate pathways are known to be involved in haemocyte adhesion, one of the first steps in nodule formation [Citation31,Citation32,Citation39] and phagocytosis [Citation40–44].
Our results showed that, regarding P. gingivalis infection and virulence, the β-AR signalling was more prominent. G. mellonella was an excellent model to transition in vitro observations into in vivo scenarios, which aligns with the 3 R principles. Previous in vitro studies [Citation11,Citation16,Citation18] using high concentrations of NE showed increased P. gingivalis virulence, as observed by the expression of virulence factors in our study. However, we did not observe any difference in PgNE compared to P. gingivalis infection regarding larval survival. In contrast, PgISO infection caused higher larval mortality. These findings could be strain-specific, as Pseudomonas aeruginosa H103 treated with 10 µM epinephrine increased bacterial virulence and decreased the survival of G. mellonella [Citation45]. The increased mortality caused by the highly virulent ISO-treated P. gingivalis could be partially reversed by the systemic action of ISO on the immune response, thereby increasing the haemocyte density. This shows that the action of the AS on the immune response can overcome the higher bacterial virulence caused by the same agent.
We demonstrated that ISO action on survival was mainly related with the cellular response. On the humoral arm, we did not observe any influence of the ligands on melanization, as has already been shown for OCT [Citation30]. Regarding the influence of infection and NE/ISO treatment on the gene expression of cecropin and gloverin (the two main AMPs against gram-negative bacteria) NE and ISO had some reducing effects on the mRNA levels of both peptides. It has become clear that stress influences the immune system by simultaneously upregulating some functions while downregulating others [Citation41,Citation46]. This balance is needed at the organismal level because over- or under-activation of immune reactions can damage the host. The decrease in AMP observed for both ligands could be explained by different mechanisms.
On the one hand, for ISO, this could be due to the decrease in the bacterial load leading to AMP reduction. On the other hand, the decrease observed in the NE group suggests that other AMPs, or additional factors, might be more important in the survival of G. mellonella upon P. gingivalis infection. These delicate balances between all innate immunity components may constitute the key response to survival and infection resolution. Drosophila melanogaster and Sarcophaga peregrina are not killed by P. gingivalis, whereas G. mellonella and silkworms suffer from lethal host damage caused by this pathogen [Citation47], indicating that organismal differences in the innate immune system influence disease outcomes.
The G. mellonella model was limited by the inability for chronic exposure to bacteria and ligands, due to rapid adrenergic responses and lethal bacterial loads; therefore, we investigated the acute influence of α- and β-AR responses during P. gingivalis infection on innate immunity. The results clearly showed that the activation of β-AR during the initial phase of infection favours phagocytosis and bacterial clearing, preventing the death of the larvae.
Conclusions
Herein, we validated the use of the G. mellonella model to investigate the role of adrenergic signalling during bacterial infection. We showed that the ligands binding to different ARs had opposite effects during P. gingivalis infection, with α-AR (NE) negatively affecting the course of infection, and β-AR (ISO) being protective for the larvae. The protective effect of ISO was mainly through the mobilization of sessile haemocytes to the haemolymph, where they decreased the bacterial load, leading to a more controlled infection. Finally, the systemic action of the ISO surpassed the negative action of more virulent bacteria, suggesting that the immune system can modulate itself to various stimuli, ultimately dictating the outcome of the infection.
Materials and methods
Galleria mellonella
Fifth instar larvae, reared in the laboratory of Microbiology and Immunology at the Institute of Science and Technology (ICT/UNESP/Brazil), weighing 0.15–0.25 g, were selected from a pool of healthy individuals that showed no signs of cuticle pigmentation. The larvae were stored at 25 °C [Citation48] for one day before use when they were kept without food. There was no blinding of samples for the experiments and 20 larvae per group were used, unless otherwise stated.
Bacteria growth conditions
ATCC P. gingivalis 33277 (MicrobiologicsTM) were activated in Brucella agar as the basis for the blood agar (5% defibrinated sheep blood), supplemented with 1% haemin and 1% menadione. The plates were incubated in an anaerobiosis jar with the aid of an anaerobiosis generator at 37 °C for 5–7 days. The bacteria were used between passages 4 and 8 for all experiments. Characteristic black colonies were harvested from the agar plates and suspended in PBS to 108 CFU/mL (agar-grown bacteria). This suspension was used in the interaction experiments where P. gingivalis and ISO/NE were systemically injected in the last proleg of the larvae. For the experiments in which the bacteria were cultivated in the presence of the ligands (ISO/NE), colonies were transferred from agar to Brucella broth added to the ligands or PBS (control) and grown for 24 h in anaerobiosis (broth-grown bacteria). On the next day, samples were centrifuged at 5,000 rpm for 10 min and the pellet was resuspended in PBS to 109 CFU/mL. The final suspensions were used for injecting the larvae.
G. mellonella susceptibility to P. gingivalis
Bacterial suspensions (10 µL) were inoculated in the last left proleg of the G. mellonella larvae using micro syringes (Hamilton Inc., EUA) in different concentrations (107 and 108 CFU/mL for agar-grown bacteria; and 108 and 109 CFU/mL for broth-grown bacteria). After inoculation, the larvae were kept at 37 ºC, and survival was assessed daily for 5 days for agar-grown bacteria and hourly (up to 24 h) for broth-grown bacteria. At this point, the larvae were kept at 37 ºC because it is the ideal temperature for antimicrobial susceptibility testing of P. gingivalis [Citation49], and it is well tolerated by the G. mellonella larvae [Citation50,Citation51].
ISO, NE, and OCT toxicity and cellular immune profiling
To assess if the ligands were toxic for G. mellonella, a wide range of ISO (Isoproterenol hydrochloride, Sigma, I6504), NE (Noradrenaline tartrate, Sigma, N1100000), and OCT (Octopamine hydrochloride, Sigma, O0250) concentrations were injected into the last right proleg of the larvae. Concentrations were selected based on serum levels of NE in humans and OCT in insects during physiological, stress and pathological (pheochromocytoma) states [Citation16–18,Citation29,Citation30,Citation52,Citation53]. The larvae were inoculated with 10 µL of ISO/NE/OCT or PBS, which served as the negative control. After injection, the larvae were kept at 37 ºC, and the survival was noted every day for 5 days. In a separate set of larvae, after 30, 90 and 180 min incubation at 37 °C, the haemolymph was bled through an incision on the last left proleg, diluted 1:100 in IPS buffer (2% NaCl; 0.1 M glucose; 30 mM sodium citrate; 26 mM citric acid and 10 mM EDTA) and the haemocytes were counted in a Neubauer chamber. This procedure was performed to temporally assess the influence of treatments on haemocyte density.
ISO, NE, and OCT influence on the outcomes of P. gingivalis infection in G. mellonella
After concentration screening (see results section- ), we chose 100 pM for ISO and 1 µM for NE with a 108 CFU/mL of P. gingivalis for the subsequent experiments. Larvae were dived into six groups: PBS, P. gingivalis, 100 pM ISO, 1 µM NE, P. gingivalis +100 pM ISO, and P. gingivalis +1 µM NE. The insects were injected with 10 µL of PBS, ISO, or NE on the right last proleg, and immediately after they were injected 108 CFU/mL of P. gingivalis or PBS in the last left proleg. The larvae were incubated at 37 °C for 5 days and survival curve was determined daily. Other experimental readouts for the interaction studies were measured 30 min after the inoculation, except for the AMPs gene expression that was performed 24 h later. Additional two sets of larvae were bled to collect haemolymph for melanization and haemocyte density evaluation.
P. gingivalis recovery from the haemolymph
To analyse the possible influence of the ligands on bacterial load, the larvae were inoculated with 100 pM ISO, 1 µM NE, or PBS (control); and immediately infected with 108 CFU/mL of P. gingivalis. The insects were then incubated at 37 °C for 30 min. The time period chosen was based on the transient action of the ligands on the haemocyte count and previous experience of our group, wherein the amount of bacteria naturally decreased after 3 h of infection [Citation54]. Haemolymph from three larvae/group was collected by an incision in the last proleg and pooled, serially diluted to 1:10,000, 100 µL of the final dilution was plated on supplemented Brucella blood agar and incubated in anaerobiosis for 7 days. The characteristic black colonies were manually counted and expressed in CFU/mL. This procedure was performed with 10 pools of 3 larvae per group (total of 30 larvae per group).
Quantification of melanization
A 20 µL of haemolymph from each larva was collected into pre-chilled Eppendorf tubes (30 min at −20 °C) as described above and centrifuged at 12,000 rpm for 10 min at 4 ºC. A 10 µL of the supernatant was diluted in 40 µL of IPS in a 96-well plate. The plate was incubated at RT for 5 min, and then it was read at 405 nm using a spectrophotometer.
G. mellonella antimicrobial peptides (AMP) gene expression using RT-qPCR
Ten larvae per group were stored at −80 ºC, 24 h after injection of PBS, P. gingivalis, P. gingivalis +100 pM ISO and P. gingivalis +1 µM NE. The whole larvae were then macerated in liquid nitrogen using pestle and mortar. The powder was resuspended in 1 mL TRIzol® (ThermoFisher Scientific, 15,596,026), the RNA was isolated according to the manufacturer’s protocol and its concentration was measured using a NanoDrop 2000 Spectrophotometer. Only RNA samples with a 260/280 ratio of 1.8–2.0 and 230/260 ratio above 1.8 were used in reverse transcription. A 1,000 ng of the extracted RNA was treated with RQ1 RNase-Free DNase (Promega, M6101) and transcribed to cDNA using the GoScript™ Reverse Transcription Mix, Random Primers (Promega, A2801). Two reference genes for G. mellonella, β-actin, and Ef-1a, were run in all experimental groups. The obtained results were analysed at https://heartcure.com.au/, and the most stable gene was selected as the reference gene, which was Ef-1a. The primer sequences used are shown in . For the RT-qPCR reactions, GoTaq® qPCR Master Mix kit (Promega, A6002) was used. The reactions were performed in duplicate wells in a StepOnePlus™ Real-Time PCR System (Applied Biosystems, Foster, CA, USA), consisting of 5 μL GoTaq® qPCR Master, 0.3 μL CXR, 0.5 μM primers (final concentration), and target cDNA (2 µL of 1:10 diluted cDNA, final concentration-10 ng), supplemented with RNAse-free ddH2O to a final volume of 10 μL. The cycling parameters for the amplification reactions were 50 °C 20 s, 95 °C 3 min; 95 °C 20 s, 60 °C 1 min for 40 cycles; and 95 °C 15 s, 60 °C 1 min, 95 °C for 15 s. The level of gene expression was calculated by applying the 2 −ΔΔCT method.
Table 1. Genes investigating P. gingivalis virulence and G. mellonella immune defense.
Nodule quantification by histology
For histological analysis, 10 larvae from the groups P. gingivalis, P. gingivalis +100 pM ISO, and P. gingivalis +1 µM NE were used. Thirty minutes post-injection, the larvae were frozen at −20 °C for 10 min, injected with 100 µL of Bouin’s aqueous solution (Sigma, HT10132) in the last proleg for fixation, and kept at 4 °C for 72 hours. After that, the larvae were stored in 70% ethanol for 48 hours. For processing, the region between the last and second proleg was separated and hemisectioned, after which the pieces were routinely processed for paraffin embedding and haematoxylin & eosin staining. Three sections, 20 µm distant from each other per larvae, were evaluated in a brightfield microscope using a 20× objective. Structures containing more than 4 haemocytes arranged around a melanized area were considered a nodule. The number of nodules in the halves on the same histological plane was averaged and the values of the three sections were summed for statistical analysis.
Haemocyte density in the haemolymph and fat body/adjacent organs
Haemocytes can be free in the haemocoel in the haemolymph (circulating haemocytes) or in the surface of internal organs such as the fat body and dorsal tube (also called sessile haemocytes). To evaluate both haemocyte density, after collecting the haemolymph (for circulating cells), the fat body and adjacent organs were removed through the same incision to evaluate sessile cells. The fat body was collected in an Eppendorf tube containing 1 mL of chilled IPS, quickly vortexed, and centrifuged at 12,000 rpm, for 10 min at 4 ºC. The supernatant was removed from the tube, and the pellet was resuspended in 1 mL of cold IPS. The circulating and sessile haemocytes were counted using a Neubauer chamber. These experiments were performed using ISO (100 pM) and OCT (100 pM and 1 nM), and to validate the β-adrenergic origin of the responses elicited by them, we co-injected some larvae with ISO/OCT plus 10 µM of propranolol hydrochloride (Sigma, P8688), a β-blocker to try to blunt the responses [Citation58].
Direct influence of NE and ISO on P. gingivalis virulence and infection outcome
To assess the influence of ISO and NE directly on the virulence factors of P. gingivalis, bacteria were cultivated in broth with the presence of the ligands in the concentration that systemically influenced the haemocyte count in vivo (100 pM ISO, 1 µM NE) and with 100 µM (ISO and NE), a concentration that was shown to have activity in vitro [Citation16–18]. The larvae were injected with PBS, P. gingivalis grown in PBS, P. gingivalis grown in 100 pM ISO (PgISO 100 pM) or 100 µM ISO (PgISO 100 µM) supplemented broth, P. gingivalis grown in 1 µM NE (PgNE 1 µM) or 100 µM NE (PgNE 100 µM) supplemented broth. After assessing that ISO increased P. gingivalis virulence (see Results section, ), in order to verify if the systemic administration of the ISO could influence the outcome when PgISO was administered, one more group was added: immediately after the injection of 100 pM ISO, P. gingivalis grown in 100 pM ISO was also administered (100 pM ISO + PgISO 100 pM). The larvae were followed hourly during 24 h, for survival assessment.
To investigate which genes were involved in the enhanced virulence of P. gingivalis grown with ISO or NE, we performed RT-qPCR. After the incubation period (24 h) the bacteria were centrifuged at 5,000 rpm for 10 min and the pellet frozen down at −80 °C. Later, we performed RNA extraction using TRIzol® following manufacturing instructions and sample quality, DNase treatment, transcription, amplification, reference gene selection (16S), and gene expression calculation were as described in section 5.10. The primers for the genes analysed are presented in .
Statistical analysis
The experimental unit in this study was a single G. mellonella larvae, except for the P. gingivalis recovery assay in which a pool of three larvae was considered the experimental unit. Statistical analysis was carried out using the GraphPad Prism 6 program (GraphPad Software, LA Jolla California USA) or Orange [Citation59] for creating the heatmaps. For haemocyte count, data were normalized by Z score, using the PBS group of each experiment as the baseline. After assessing the distribution, data were submitted to ANOVA or Kruskal-Wallis, T-test, or Mann-Whitney. Significance was assigned if p < 0.05.
The study design described above is shown in .
Figure 10. Direct action of β-adrenergic signalling on P. gingivalis. A) rgbp and fimA gene expression after co-culturing P. gingivalis +100pM or 100 μM ISO (rgbp, n = 6 biological replicates per group, mean and standard deviation, ANOVA, p < 0.0001; fimA, n=6 biological replicates per group, ANOVA, p = 0.0131) (a). Co-culturing P. gingivalis with 100 pM and 100 μM ISO increased the mortality of the larvae 24 h after infection (n = 10 larvae for PBS group, n = 45 larvae for P. gingivalis group, n = 20 for PgISO 100 pM and PgISO 100 μM and 100 pM ISO + PgISO 100 pM groups, Kaplan–Meier plot, log-rank [Mantel-cox] p < 0.0001) (b). The systemic administration of 100 pM ISO could partially reverse the increased PgISO 100pM phenotype (c). Same lower-case letters close to the graph bars/lines represent an absence of significant difference.
![Figure 10. Direct action of β-adrenergic signalling on P. gingivalis. A) rgbp and fimA gene expression after co-culturing P. gingivalis +100pM or 100 μM ISO (rgbp, n = 6 biological replicates per group, mean and standard deviation, ANOVA, p < 0.0001; fimA, n=6 biological replicates per group, ANOVA, p = 0.0131) (a). Co-culturing P. gingivalis with 100 pM and 100 μM ISO increased the mortality of the larvae 24 h after infection (n = 10 larvae for PBS group, n = 45 larvae for P. gingivalis group, n = 20 for PgISO 100 pM and PgISO 100 μM and 100 pM ISO + PgISO 100 pM groups, Kaplan–Meier plot, log-rank [Mantel-cox] p < 0.0001) (b). The systemic administration of 100 pM ISO could partially reverse the increased PgISO 100pM phenotype (c). Same lower-case letters close to the graph bars/lines represent an absence of significant difference.](/cms/asset/d377e9ad-2732-4dc5-9717-49a9ca8cc6c6/kvir_a_2123302_f0010_oc.jpg)
Author contributions
RMM: Contributed to conception and funding acquisition, study design, data acquisition and interpretation, performing statistical analyses, and drafting and critically revising the manuscript.
MTG: Contributed to data acquisition and critically revising the manuscript.
FS: Contributed to study design, performing statistical analyses, drafting and critically revising the manuscript.
PPB: Contributed to data acquisition and critically revising the manuscript.
JCJ: Contributed to study design and critically revising the manuscript.
ALA: Contributed to conception and funding acquisition, study design, data analysis, drafting and critically revising the manuscript.
Data availability
The data that support the findings of this study are openly available in the institutional repository at https://repositorio.unesp.br/handle/11449/234896
Supplemental Material
Download Zip (2.7 MB)Disclosure statement
No potential conflict of interest was reported by the author(s).
Supplementary material
Supplemental data for this article can be accessed online at https://doi.org/10.1080/21505594.2022.2123302.
Additional information
Funding
References
- Coelho JMF, Miranda SS, da Cruz SS, et al. Is there association between stress and periodontitis? Clin Oral Investig. 2020;24(7):2285–2294. DOI:10.1007/s00784-019-03083-9
- Genco RJ, Ho AW, Grossi SG, et al. A relationship of stress, distress, and inadequate coping behaviors to periodontal disease. J Periodontol. 1999;70(7):711–723. doi:10.1902/jop.1999.70.7.711.
- Martins LG, Spreafico CS, Tanobe PG, et al. Influence of adrenergic neuromodulation during induction of periodontitis in rats. J Int Acad Periodontol. 2017;19(3):80–88.
- Scanzano A, Cosentino M. Adrenergic regulation of innate immunity: a review. Front Pharmacol. 2015;6:171. doi:10.3389/fphar.2015.00171.
- Lu H, Xu M, Wang F, et al. Chronic stress enhances progression of periodontitis via α1-adrenergic signaling: a potential target for periodontal disease therapy. Exp Mol Med. 2014;46(10):e118. doi:10.1038/emm.2014.65.
- Gruber R, Leimer M, Fischer MB, et al. Beta2-Adrenergic receptor agonists reduce proliferation but not protein synthesis of periodontal fibroblasts stimulated with platelet-derived growth factor-BB. Arch Oral Biol. 2013;58(12):1812–1817. doi:10.1016/j.archoralbio.2013.09.005.
- Breivik T, Gundersen Y, Opstad PK, et al. Chemical sympathectomy inhibits periodontal disease in Fischer 344 rats. J Periodontal Res. 2005;40(4):325–330. doi:10.1111/j.1600-0765.2005.00803.x.
- Okada Y, Hamada N, Kim Y, et al. Blockade of sympathetic β-receptors inhibits Porphyromonas gingivalis-induced alveolar bone loss in an experimental rat periodontitis model. Arch Oral Biol. 2010;55(7):502–508. doi:10.1016/j.archoralbio.2010.04.002.
- Rodrigues WF, Madeira MFM, Da Silva TA, et al. Low dose of propranolol down-modulates bone resorption by inhibiting inflammation and osteoclast differentiation. Br J Pharmacol. 2012;165(7):2140–2151. doi:10.1111/j.1476-5381.2011.01686.x.
- Belay T, Sonnenfeld G. Differential effects of catecholamines on in vitro growth of pathogenic bacteria. Life Sci. 2002;71(4):447–456. doi:10.1016/s0024-3205(02)01683-1.
- Belay T, Aviles H, Vance M, et al. Catecholamines and in vitro growth of pathogenic bacteria: enhancement of growth varies greatly among bacterial species. Life Sci. 2003;73(12):1527–1535. doi:10.1016/s0024-3205(03)00472-7.
- Roberts A, Matthews JB, Socransky SS, et al. Stress and the periodontal diseases: growth responses of periodontal bacteria to Escherichia coli stress-associated autoinducer and exogenous Fe. Oral Microbiol Immunol. 2005;20(3):147–153. doi:10.1111/j.1399-302X.2004.00196.x.
- Roberts A, Matthews JB, Socransky SS, et al. Stress and the periodontal diseases: effects of catecholamines on the growth of periodontal bacteria in vitro. Oral Microbiol Immunol. 2002;17(5):296–303. doi:10.1034/j.1399-302x.2002.170506.x.
- Weinstein LI, Revuelta A, Pando RH. Catecholamines and acetylcholine are key regulators of the interaction between microbes and the immune system. Ann N Y Acad Sci. 2015;1351(1):39–51. doi:10.1111/nyas.12792.
- Hajishengallis G. Immunomicrobial pathogenesis of periodontitis: keystones, pathobionts, and host response. Trends Immunol. 2014;35(1):3–11. doi:10.1016/j.it.2013.09.001.
- Graziano TS, Closs P, Poppi T, et al. Catecholamines promote the expression of virulence and oxidative stress genes in Porphyromonas gingivalis. J Periodontal Res. 2014;49(5):660–669. doi:10.1111/jre.12148.
- Boyanova L. Stress hormone epinephrine (adrenaline) and norepinephrine (noradrenaline) effects on the anaerobic bacteria. Anaerobe. 2017;44:13–19. doi:10.1016/j.anaerobe.2017.01.003.
- Saito T, Inagaki S, Sakurai K, et al. Exposure of P. gingivalis to noradrenaline reduces bacterial growth and elevates ArgX protease activity. Arch Oral Biol. 2011;56(3):244–250. doi:10.1016/j.archoralbio.2010.09.014.
- Pereira TC, deBarros PP, Fugisaki LRO, et al. Recent advances in the use of Galleria mellonella model to study immune responses against human pathogens. J Fungi. 2018;4(4):128. doi:10.3390/jof4040128.
- Gomes LAP, Figueiredo LMA, Palma ALR, et al. Punica granatum L. (Pomegranate) extract: in vivo study of antimicrobial activity against Porphyromonas gingivalis in Galleria mellonella model. Sci World J. 2016;2016:8626987. doi:10.1155/2016/8626987.
- dos Santos JD, de Alvarenga JA, Rossoni RD, et al. Immunomodulatory effect of photodynamic therapy in Galleria mellonella infected with Porphyromonas gingivalis. Microb Pathog. 2017;110:507–511. doi:10.1016/j.micpath.2017.07.045.
- Geraldo BMC, Batalha MN, Milhan NVM, et al. Heat-Killed Lactobacillus reuteri and cell-free culture supernatant have similar effects to viable probiotics during interaction with Porphyromonas gingivalis. J Periodontal Res. 2020;55(2):215–220. doi:10.1111/jre.12704.
- Moman R, O’Neill CA, Ledder RG, et al. Mitigation of the toxic effects of periodontal pathogens by candidate probiotics in oral keratinocytes, and in an invertebrate model. Front Microbiol. 2020;16(11):999. doi:10.3389/fmicb.2020.00999.
- Solbiati J, Duran-Pinedo A, Godoy Rocha F, et al. Virulence of the pathogen Porphyromonas gingivalis is controlled by the CRISPR-Cas protein Cas3. mSystems. 2020;5(5):20–e00852. doi:10.1128/mSystems.00852-20.
- Wartoń S, Dutkowski AB. Ultrastructure of the neurosecretory cells of the pars intercerebralis of Galleria mellonella (Lepidoptera) after noradrenaline administration. Gen Comp Endocrinol. 1977;33(2):179–186. doi:10.1016/0016-6480(77)90242-8.
- Adamo SA. Norepinephrine and octopamine: linking stress and immune function across phyla. Invertebr Surviv J. 2008;26(6):942–950. doi:10.1016/j.bbi.2012.04.007.
- Lange A, Beier S, Huson DH, et al. Genome sequence of Galleria mellonella (Greater Wax Moth). Genome Announc. 2018;6(2):17–e01220. doi:10.1128/genomeA.01220-17.
- Adamo SA. Why should an immune response activate the stress response? Insights from the insects (the cricket Gryllus texensis). Brain Behav Immun. 2010;24(2):194–200. doi:10.1016/j.bbi.2009.08.003.
- Davenport AP, Evans PD. Stress-Induced changes in the octopamine levels of insect haemolymph. Insect Biochem. 1984;14(2):135–143. doi:10.1016/0020-1790(84)90021-0.
- Dunphy GB, Downer RGH. Octopamine, a modulator of the haemocytic nodulation response of non-immune Galleria mellonella larvae. J Insect Physiol. 1994;40(3):267–272. doi:10.1016/0022-1910(94)90050-7.
- Brooks CL, Dunphy GB. Protein kinase a affects Galleria mellonella (Insecta: Lepidoptera) larval haemocyte non-self responses. Immunol Cell Biol. 2005;83(2):150–159. doi:10.1111/j.1440-1711.2005.01316.x.
- Cytryńska M, Zdybicka-Barabas A, Jakubowicz T. Protein kinase a activity and protein phosphorylation in the haemocytes of immune-challenged Galleria mellonella larvae. Comp Biochem Physiol B Biochem Mol Biol. 2007;148(1):74–83. doi:10.1016/j.cbpb.2007.04.020.
- Kuo HW, Lin DW, Cheng W. Transient enhancement of immune resistance functions in Litopenaeus vannamei through a low-dose octopamine injection. Fish Shellfish Immunol. 2019;84:532–540. doi:10.1016/j.fsi.2018.10.060.
- Kuo HW, Cheng W. Octopamine enhances the immune responses of freshwater giant prawn, Macrobrachium rosenbergii, via octopamine receptors. Dev Comp Immunol. 2018;81:19–32. doi:10.1016/j.dci.2017.11.002.
- Kong H, Yuan L, Dong C, et al. Immunological regulation by a β-adrenergic-like octopamine receptor gene in crowded larvae of the oriental Armyworm, Mythmina separata. Dev Comp Immunol. 2020;113. 113:103802. doi:10.1016/j.dci.2020.103802.
- Chang CC, Di HM, Cheng W. Norepinephrine depresses the immunity and disease-resistance ability via α1- and β1-adrenergic receptors of Macrobrachium rosenbergii. Dev Comp Immunol. 2011;35(6):685–691. doi:10.1016/j.dci.2011.01.020.
- King JG, Hillyer JF. Spatial and temporal in vivo analysis of circulating and sessile immune cells in mosquitoes: hemocyte mitosis following infection. BMC Biol. 2013;30(11):55. doi:10.1186/1741-7007-11-55.
- Bellinger DL, Lorton D. Autonomic regulation of cellular immune function. Auton Neurosci. 2014;182:15–41. doi:10.1016/j.autneu.2014.01.006.
- Marin D, Dunphy GB, Mandato CA. Cyclic AMP affects the haemocyte responses of larval Galleria mellonella to selected antigens. J Insect Physiol. 2005;51(5):575–586. doi:10.1016/j.jinsphys.2005.02.010.
- Baines D, DeSantis T, Downer RGH. Octopamine and 5-hydroxytryptamine enhance the phagocytic and nodule formation activities of cockroach (Periplaneta americana) haemocytes. J Insect Physiol. 1992;38(11):905–914. doi:10.1016/0022-1910(92)90102-J.
- Adamo SA. The stress response and immune system share, borrow, and reconfigure their physiological network elements: evidence from the insects. Horm Behav. 2017;88:25–30. doi:10.1016/j.yhbeh.2016.10.003.
- Geun SK, Nalini M, Kim Y, et al. Octopamine and 5-hydroxytryptamine mediate hemocytic phagocytosis and nodule formation via eicosanoids in the beet armyworm, Spodoptera exigua. Arch Insect Biochem Physiol. 2009;70(3):162–176. doi:10.1002/arch.20286.
- Huang J, Wu SF, Li XH, et al. The characterization of a concentration-sensitive α-adrenergic-like octopamine receptor found on insect immune cells and its possible role in mediating stress hormone effects on immune function. Brain Behav Immun. 2012;26(6):942–950. doi:10.1016/j.bbi.2012.04.007.
- Liu KF, Kuo HW, Chang CC, et al. The intracellular signaling pathway of octopamine upregulating immune resistance functions in Penaeus monodon. Fish Shellfish Immunol. 2019;92:188–195. doi:10.1016/j.fsi.2019.06.007.
- Cambronel M, Tortuel D, Biaggini K, et al. Epinephrine affects motility, and increases adhesion, biofilm and virulence of Pseudomonas aeruginosa H103. Sci Rep. 2019;9(1):20203. doi:10.1038/s41598-019-56666-7.
- Adamo SA. The effects of the stress response on immune function in invertebrates: an evolutionary perspective on an ancient connection. Horm Behav. 2012;62(3):324–330. doi:10.1016/j.yhbeh.2012.02.012.
- Ishii K, Hamamoto H, Imamura K, et al. Porphyromonas gingivalis peptidoglycans induce excessive activation of the innate immune system in silkworm larvae. J Biol Chem. 2010;285(43):33338–33347. doi:10.1074/jbc.M110.112987.
- Jorjão AL, Oliveira LD, Scorzoni L, et al. From moths to caterpillars: ideal conditions for Galleria mellonella rearing for in vivo microbiological studies. Virulence. 2018;9(1):383–389. doi:10.1080/21505594.2017.1397871.
- CLSI. Reference method for broth dilution antifungal susceptibility testing of yeasts. 4th ed. CLSI standard M27. Wayne, PA: Clinical and Laboratory Standards Institute;2017. Available from: www.clsi.org
- Tsai C-Y, Loh JMS, Proft T. Galleria mellonella infection models for the study of bacterial diseases and for antimicrobial drug testing. Virulence. 2016;7(3):214–229. doi:10.1080/21505594.2015.1135289.
- Ménard G, Rouillon A, Cattoir V, et al. Galleria mellonella as a suitable model of bacterial infection: past, present and future. Front Cell Infect Microbiol. 2021;11:782733. doi:10.3389/fcimb.2021.782733.
- Rocha MF, Tauzin-Fin P, Vasconcelos PL, et al. Assessment of serum catecholamine concentrations in patients with pheochromocytoma undergoing video laparoscopic adrenalectomy. Int Braz J Urol. 2005;31(4):299–307. doi:10.1590/s1677-55382005000400002.
- Adamo SA, Linn CE, Hoy RR. The role of neurohormonal octopamine during “fight or flight” behaviour in the field cricket Gryllus bimaculatus. J Exp Biol. 1995;198(8):1691–1700. doi:10.1242/jeb.198.8.1691.
- Santos TA, Scorzoni L, de C SA, et al. Galleria mellonella as an experimental model for studying periodontopathogens. J Indian Soc Periodontol. 2020 Nov-Dec;24(6):593–596. doi:10.4103/jisp.jisp_631_19.
- Azelmat J, Larente JF, Grenier D. The anthraquinone Rhein exhibits synergistic antibacterial activity in association with metronidazole or natural compounds and attenuates virulence gene expression in Porphyromonas gingivalis. Arch Oral Biol. 2015 Feb;60(2):342–346. doi:10.1016/j.archoralbio.2014.11.006.
- Rossoni RD, Fuchs BB, Barros PP, et al. Lactobacillus paracasei modulates the immune system of Galleria mellonella and protects against Candida albicans infection. PLoS One. 12(3) eCollection 2017:e0173332. 2017 Mar 7. doi:10.1371/journal.pone.0173332.
- Barros PP, Rossoni RD, Ribeiro FC, et al. Two sporulated Bacillus enhance immunity in Galleria mellonella protecting against Candida albicans. Microb Pathog. 2019 Jul;132:335–342 doi:10.1016/j.micpath.2019.05.023.
- Jiang Q, Zhou Z, Wang L, et al. Mutual modulation between norepinephrine and nitric oxide in haemocytes during the mollusc immune response. Sci Rep. 2014 Nov 7;4(1):6963. doi:10.1038/srep06963.
- Demšar J, Curk T, Erjavec A, et al. Orange: data mining toolbox in python. J Mach Learn Res. 2013;14:2349–2353. Available from. http://jmlr.org/papers/v14/demsar13a.html