ABSTRACT
Metals are nutrients essential for almost all lifeforms. Bacteria have evolved several mechanisms to overcome the metal restrictions imposed by the host. Vibrio parahaemolyticus causes severe threats to public health and significant economic losses in shrimp aquaculture. Herein, we report that ZrgA contributes to zinc acquisition in this pathogen. The operon VP_RS01455 to VP_RS01475 of V. parahaemolyticus encodes the putative Zn transporter ZrgABCDE, whose homologs are widely distributed in Vibrionaceae. RNA sequencing analysis revealed that V. parahaemolyticus modulates the transcriptome in response to Zn limitation. Genes in the Zinc uptake regulator (Zur) regulon are upregulated during Zn limitation, including three genes annotated to encode Zn-binding proteins. Significant upregulation of these three genes during Zn limitation was also confirmed by quantitative real-time PCR (qRT-PCR) analysis. However, only the mutants containing a VP_RS01470 (zrgA) deletion exhibited impaired growth under Zn-deficient conditions, indicating that VP_RS01470 plays the predominant role in V. parahaemolyticus Zn acquisition. The VP_RS01470 deletion mutant displayed a false appearance of decreased swimming motility under Zn-deficient conditions, as revealed by the fact that the polar flagellar-related genes were not downregulated in the mutant. Moreover, VP_RS01470 deletion produced no noticeable impact on the swarming motility and virulence in mice. qRT-PCR analysis and β-galactosidase activity assays indicated that Zur negatively regulates VP_RS01470 expression in V. parahaemolyticus. Collectively, our findings suggest that ZrgA is required for Zn acquisition in V. parahaemolyticus and highlight the importance of detecting the expression of flagellar genes during analysis of motility of a mutant deficient in growth.
Introduction
Vibrio parahaemolyticus is a ubiquitous Gram-negative bacterium in a wide variety of environments, including oceans, coasts, and estuaries [Citation1]. Acute gastroenteritis can often be attributed to the ingestion of seafood contaminated by this bacterium [Citation2]. In addition, V. parahaemolyticus is occasionally associated with several other diseases, such as septicaemia, necrotizing fasciitis, and wound infections [Citation3]. In 1950, a foodborne disease caused by this pathogen was reported in Japan, which resulted in 272 illnesses with 20 deaths [Citation1]. Subsequently, this pathogen has been isolated worldwide in either sporadic cases or outbreaks of gastroenteritis [Citation4]. In China, V. parahaemolyticus represents the most prevalent foodborne pathogen in food commodities; while in America, it is the major cause of gastroenteritis associated with seafood [Citation5,Citation6]. Moreover, this bacterium causes acute hepatopancreatic necrosis disease in shrimp and thereby responsible for significant economic losses in shrimp aquaculture [Citation7,Citation8]. V. parahaemolyticus utilizes a large number of virulence factors during infection to cause cytotoxicity and enterotoxicity [Citation2]. Despite some progress, extensive research is still required to reveal the physiology and pathogenesis of this bacterium.
Metals are nutrients essential for almost all lifeforms. Many proteins need to interact with metal to maintain structural stability and/or exert their function [Citation9]. To defend against invading pathogens, the mammalian hosts have evolved a strategy termed nutritional immunity, i.e. sequestration and mobilization of metals, which renders them unavailable to the pathogens [Citation10]. For example, calprotectin, a host metal-binding protein released by neutrophils at sites of infection, can sequester manganese (Mn) and zinc (Zn), thus inhibiting bacterial proliferation [Citation11,Citation12]. However, bacterial pathogens have developed several mechanisms as countermeasures to combat the host-imposed nutritional immunity [Citation13]. For instance, Yersinia pestis produces yersiniabactin to repress the Zn-mediated nutritional immunity of the mammalian and insect hosts during infection [Citation14]. Despite their essential role, metals are toxic to cells when accumulated in excessive amounts [Citation15]. Moreover, the hosts exploit metal toxicity to control bacterial infection [Citation10]. To respond to restricted or excess metals, bacteria can regulate the expression of specific genes through metalloregulators [Citation13].
Metal acquisition and the impacts of metals on the physiology and pathogenesis of V. parahaemolyticus have been partly revealed. A previous study showed that iron (Fe) and Mn enhance V. parahaemolyticus virulence in mice [Citation16]. Calcium (Ca) and Fe have been reported to modulate type III secretion and swarming motility in V. parahaemolyticus [Citation17]. Nuclear magnetic resonance-based metabolomics revealed that ferric iron (Fe[III]) stimulation in V. parahaemolyticus upregulates 21 metabolites and downregulates nine [Citation18]. Interestingly, V. parahaemolyticus has adopted strategies to obtain Fe from host sources, including the utilization of siderophores, xenosiderophores, proteases, and iron-protein receptors [Citation19]. Zn ranks second in terms of the content of transition metals in living beings, behind only Fe; approximately 5% to 6% of the total proteins encoded in bacteria are Zn-binding proteins [Citation20]. A horizontally acquired ZnuA homolog, here referred to as ZnuAII, contributes to Zn transport and virulence in V. parahaemolyticus [Citation21]. However, little is known about the global transcriptome responses of V. parahaemolyticus to Zn limitation. Indeed, it remains unclear if additional Zn transporters exist in this bacterium.
In this study, the adaptive responses of V. parahaemolyticus to Zn limitation were explored by RNA sequencing analysis. VP_RS01470, a gene negatively regulated by the Zn uptake regulator (Zur), was found to play the predominant role in Zn acquisition in V. parahaemolyticus.
Materials and methods
Bacterial strains, plasmids, primers, and culture conditions
Bacterial strains and plasmids are listed in Table S1; primers are listed in Table S2. V. parahaemolyticus RIMD2210633 [Citation22] was used as the wild type (WT) strain. Unless otherwise specified, all V. parahaemolyticus and Escherichia coli strains were grown at 37°C in lysogeny broth (LB) with constant shaking at 220 rpm or on LB agar. The Zn chelator N,N,N’,N’-Tetrakis (2-pyridylmethyl) ethylenediamine (TPEN, dissolved in DMSO; Sigma-Aldrich) was used to prepare Zn-deficient medium [Citation23]. When required, either carbenicillin (50 μg/mL), chloramphenicol (25 μg/mL), or isopropyl β-D-1-thiogalactopyranoside (IPTG; 1 mM) was added.
RNA extraction
The RIMD2210633 strain was grown to an OD600 of 0.7 (early-exponential phase). Three aliquots (1 mL) were removed and centrifuged to collect bacterial cells. The three bacterial pellets were resuspended with 1 mL LB pretreated with either DMSO, 35 μM TPEN, or 35 μM TPEN along with 500 μM Zn, respectively. After incubating at 37°C for 15 min, the cultures were centrifuged to collect the cells. Total RNA was extracted using the Eastep Super Total RNA Isolation Kit (Promega). Three biological replicates, obtained from three independent experiments, grown in each of the conditions of interest were used for RNA extraction. After evaluating RNA integrity and RNA concentrations, the RNA samples were used for RNA sequencing and quantitative real-time PCR (qRT-PCR) analysis.
In another experiment, the WT, Δ1470, and CΔ1470 strains were grown to an OD600 of 0.7. Then, bacterial cells from 1 mL of each culture were collected and resuspended with 1 mL LB pretreated with 35 μM TPEN. After incubating at 37°C for 15 min, bacterial cells were collected for RNA extraction. Three biological replicates, obtained from three independent experiments, grown in each of the conditions of interest were used.
Additionally, the WT, Δzur, and CΔzur strains were grown to an OD600 of 0.7. Subsequently, bacterial cells from 1 mL of each culture were collected for RNA extraction. Three biological replicates, obtained from three independent experiments, grown in each of the conditions of interest were used.
RNA sequencing analysis
Ribosomal RNA depletion, cDNA library preparation, and sequencing were performed at Sangon Biotech Co. Ltd., as previously described [Citation24]. The clean reads were mapped to V. parahaemolyticus RIMD2210633 genome using Bowtie2. Gene expression was estimated by TPM (transcripts per million) using featureCounts. The genes with a fold change greater than 2 and an adjusted P value (q value) less than 0.05 were defined as the differentially expressed genes (DEGs). KEGG pathway enrichment analysis was performed using KOBAS-i [Citation25]; the pathways with a q value less than 0.05 were considered significantly enriched. RNA sequencing data have been submitted to Gene Expression Omnibus (GEO) under accession no. GSE200927.
Reverse transcription-PCR (RT-PCR) analysis
Total RNA extracted from the RIMD2210633 strain was used to synthesize cDNA as previously described [Citation24]. The resulting cDNA was amplified using primers 1455-F and 1475-R. PCR amplification of the genomic DNA (gDNA) from RIMD2210633 and cDNA- (cDNA reaction without reverse transcriptase) were used as positive and negative controls, respectively.
qRT-PCR analysis
qRT-PCR analysis was performed as previously described [Citation24]. The PCR efficiency for each primer pair was assessed by qRT-PCR analysis of serially diluted gDNA (1:10 dilution) as previously described [Citation26]. Only the primer pairs with an efficiency of 90% − 110% were used. The 2−ΔΔCT method [Citation27] was used to calculate gene expression levels, with gyrB as the internal standard.
Construction of mutant and complementation strains
Gene deletion was generated via homologous recombination using the pDM4 plasmid [Citation28]. The target gene upstream and downstream regions (700–850 bp) were amplified. Overlap extension PCR was implemented to fuse the two DNA fragments together. After performing a restriction digest with the appropriate enzymes, the DNA fragment was ligated into pDM4. The resulting plasmid was transformed into E. coli S17–1 λpir and next conjugated into RIMD2210633. LB agar containing carbenicillin and chloramphenicol was used for selection of single crossover recombination strain. Counterselection using LB agar containing 15% sucrose was performed to obtain double crossover recombination mutants, which were then verified by PCR and DNA sequencing. The double and triple mutants were constructed using a similar procedure, except that the recombinant plasmid was transferred into a mutant or a double mutant.
Thereafter, pMMB207, a wide-host-range low-copy-number vector [Citation29] was used to construct the complementation strain. A DNA fragment composed of a target gene and an additional ribosome-binding site was amplified from the RIMD2210633 genome. After enzymes digestion, the DNA fragment was ligated into pMMB207. The resulting plasmid was transformed into E. coli S17–1 λpir and next conjugated into Δ1470. The complementation strain was selected on agar plates containing carbenicillin and chloramphenicol and verified by PCR analysis.
Growth under zn-deficient conditions
The V. parahaemolyticus strains were grown to an OD600 of 2 and diluted 1:500 in LB pretreated with DMSO (i.e. normal conditions), varying concentrations of TPEN (30 μM, 35 μM, or 40 μM), or 35 μM TPEN along with 20 μM Zn, respectively. The cultures were transferred into 96-well plates containing 200 μL per well and three wells for each culture. The cultures were incubated at 37°C and 120 rpm. The OD595 values were measured hourly with a microplate reader.
Bacterial motility assay
The swimming and swarming motility assays were performed as previously described with some modifications [Citation30,Citation31]. To analyse the swimming motility, the colonies of each strain were pierced into the semi-solid agar plates (i.e. LB containing 0.3% agar) supplemented with DMSO, 35 μM TPEN, or 35 μM TPEN along with 20 μM Zn, respectively. The plates were photographically documented after incubating at 37°C for 18 h. To evaluate swarming, the V. parahaemolyticus strains were grown to an OD600 of 0.7; bacterial suspensions of each strain (1 μL) were spotted onto the agar plates (i.e. brain heart infusion (BHI) containing 1.5% agar, 50 μM 2,2´-Bipyridyl and 4 mM CaCl2) supplemented with 35 μM TPEN. The plates were photographically recorded after incubating at 24°C for 16 h.
Mouse infection experiment
The animal experiments were approved by the Animal Welfare and Ethics Committees of Yangzhou University and performed as previously described [Citation21].
40 female C57BL/6 mice (8–12 weeks old) were randomly divided into four groups (10 mice per group). The WT, Δ1470, and Δ1470Δ4160Δ21425 were cultured in LB broth for 12 h at 30°C and adjusted to 1 × 109 CFU/mL in PBS, respectively. For each group, the mice were intraperitoneally infected with either PBS or 100 μL of the strains. Mouse survival was recorded twice daily for seven days.
β-galactosidase activity assays
The promoter of the operon VP_RS01455 to VP_RS01475 was cloned into pDM8, a plasmid containing the promoterless lacZ gene [Citation32]. The resulting plasmid pDM8-P1475–1455 was transformed into E. coli S17 λpir and then conjugated into the WT strain and Δzur, respectively. The WT strain and Δzur containing the empty pDM8 plasmid were implemented as the control strains.
β-galactosidase activity assays were performed with some modifications to the previously described method [Citation33,Citation34]. The reporter strains were grown in LB pretreated with DMSO or 35 μM TPEN to an OD600 of 0.7. Afterwards, 1 mL of each culture was removed, centrifuged, and resuspended in 1 mL PM buffer (60 mM Na2HPO4, 40 mM NaH2PO4, 10 mM KCl, and 1 mM MgSO4, 50 mM β-mercaptoethanol, pH 7.0). The bacterial suspensions were measured for the A600 values and 200 μL of each suspension was added to 800 μL of PM buffer alongside 100 μL of chloroform and 50 μL of 0.1% SDS. After a vigorous vortex to lyse bacterial cells, the reaction was started by adding 200 μL of o-nitrophenyl-β-galactopyranoside (ONPG, 4 mg/mL in PM buffer). When the reaction mixture turned yellowish, 1 M Na2CO3 (500 μL) was added to stop the reaction. The supernatant of the mixture was then measured for A420. The β-galactosidase activity was calculated using the following formula: A420 ×1000 × min−1 × ml−1 × A600−1.
Bioinformatic and statistical analysis
The regulon of Zur was predicted by RegPrecise [Citation35]. Statistical analyses were performed using GraphPad Prism 5 (San Diego, USA). qRT-PCR data and β-galactosidase activity assays were analysed by one-way analysis of variance (ANOVA) with a Bonferroni’s post-test. Mouse survival was analysed by the log-rank test.
Results
Regulon of zur is partly conserved in vibrionaceae
Bacteria in Streptococcaceae use a Zn-binding repressor termed AdcR/ZitR to modulate Zn acquisition, while other bacteria utilize Zur, a regulator belonging to the Ferric uptake regulator (Fur) superfamily [Citation36]. RegPrecise, a resource for genome-scale exploration of transcription factor regulons [Citation35], predicted that the Zur regulon is partly conserved in Vibrionaceae (Fig. S1). In Vibrio cholerae, dual Zn transporter systems (i.e. ZnuABC and ZrgABCDE) that are regulated by Zur have been characterized [Citation23]. The ZnuABC system, composed of ZnuA – a periplasmic Zn-binding protein, ZnuB – a transmembrane permease, and ZnuC – a cytoplasmic ATPase, is a widespread high-affinity Zn uptake transporter [Citation37,Citation38]. While ZnuABC has been described in many bacterial species, ZrgABCDE has been rarely reported [Citation23,Citation39–43]. Among the V. cholerae ZrgABCDE system, ZrgABC is a putative ABC transporter similar to ZnuABC, while ZrgD and ZrgE represent two proteins with unknown functions [Citation23]. In V. parahaemolyticus, the loci VP_RS01455 to VP_RS01475 form an operon as confirmed by reverse transcription-PCR analysis (Fig. S2). This operon encodes a homolog of the V. cholerae ZrgABCDE system. BlastP analysis revealed that ZrgA, ZrgB, ZrgC, ZrgD, and ZrgE in V. parahaemolyticus share 49.38%, 82.82%, 61.34%, 40.96%, and 66.67% amino acid identity to their homologs in V. cholerae, respectively. The ZrgABCDE-like systems are widely distributed in Vibrionaceae (). Most species of Vibrionaceae encode five proteins in this system, whereas Vibrio fischeri and Photobacterium profundum lack the ZrgD encoding gene (). Multiple sequence alignment of the ZrgA proteins from these species showed that ZrgA possesses several conserved motifs potentially involved in Zn binding (Fig. S3). These results suggest the ZrgABCDE-like system might serve as a common Zn acquisition mechanism in Vibrionaceae. In addition to ZnuABC and ZrgABCDE, V. parahaemolyticus, Vibrio vulnificus, and Vibrio campbellii possess a gene cluster that encodes a Zn-regulated TonB-dependent outer membrane receptor, a second ZnuA (designated ZnuA2), and a second ZnuB (designated ZnuB2) (Fig. S1).
Figure 1. The genetic structures of ZrgABCDE-like systems in Vibrionaceae. The structures were based on the following genomes: V. parahaemolyticus RIMD2210633, NC_004603.1; V. cholerae N16961, NC_002505.1; V. vulnificus CMCP6, NC_004459.3; V. campbellii ATCC BAA-1116, NC_009783.1; V. shilonii AK1 1,103,207,001,947, NZ_ABCH01000030.1; V. atlanticus LGP32, NC_011753.2; V. fischeri ES114, CP000020.2; A. salmonicida LFI1238, NC_011312.1; and P. profundum SS9, NC_006370.1.
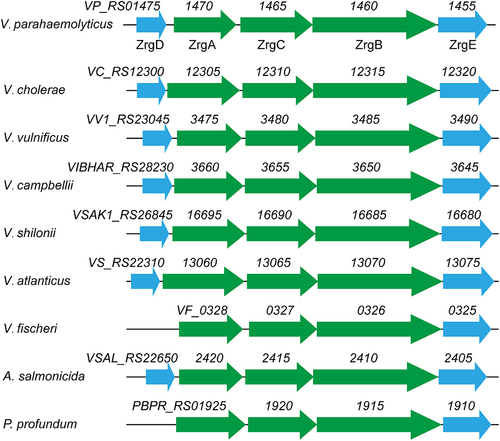
Transcriptional responses of V. parahaemolyticus to Zn limitation
Initially, RNA sequencing was used to reveal the molecular mechanisms of the V. parahaemolyticus response to Zn limitation. We compared the transcriptome of V. parahaemolyticus RIMD2210633 grown under Zn-deficient (LB pretreated with 35 μM TPEN) and Zn-replete conditions (LB pretreated with 35 μM TPEN along with 500 μM Zn). A total of 402 genes (8.5% of the genome) were differentially expressed during Zn limitation, with 244 genes upregulated and 158 genes downregulated (). The DEGs can be enriched into 70 KEGG pathways, and the top 20 enriched pathways are shown in . Among these, six pathways were significantly enriched, including citrate cycle, oxidative phosphorylation, butanoate metabolism, microbial metabolism in diverse environments, two-component system, and sulphur metabolism ( and Table S3). These results indicate that V. parahaemolyticus modulates the transcriptome in response to Zn limitation.
Figure 2. The transcriptome of V. parahaemolyticus is altered in response to Zn limitation. (a) the transcriptome change under Zn-deficient conditions compared to Zn-replete conditions. DEGs, up, and down represent the differentially expressed genes, upregulated genes, and downregulated genes, respectively. (b) the top 20 enriched KEGG pathways of the DEGs. The KEGG pathways with a q value <0.05 were considered significantly enriched. (c) Heatmap showing the expression of genes in the Zur regulon based on the TPM (transcripts per million) values. Zn-deficient_rep1–3 and Zn-replete 1–3 represent the three biological replications of Zn-deficient and Zn-replete conditions, respectively.
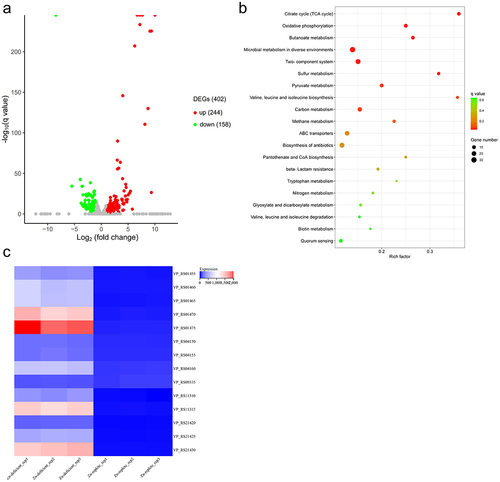
Regulon of V. parahaemolyticus Zur is significantly upregulated under zn limitation conditions
RNA sequencing analysis revealed that the Zur regulon was significantly upregulated under Zn limitation conditions ( and ). The operon VP_RS21420 to VP_RS21430, which encodes a known Zn transporter in V. parahaemolyticus [Citation21], was among the most highly upregulated genes (). The genes VP_RS04150 to VP_RS04160, which encode the conserved Zn transporter ZnuABC, were upregulated approximately 8- to 16-fold (). Notably, the operon VP_RS01455 to VP_RS01475, which encodes the ZrgABCDE system in V. parahaemolyticus, was also highly upregulated (). qRT-PCR data showed that the expression of VP_RS01470, VP_RS04160, and VP_RS21425, which are annotated to encode Zn-binding proteins, was upregulated approximately 288-, 33-, and 170-fold, respectively, under Zn-deficient conditions, and returned to the baseline levels under Zn-replete conditions (). These data are consistent with the RNA sequencing results, and collectively, indicate that the Zur regulon is important for V. parahaemolyticus response to Zn limitation.
Figure 3. V. parahaemolyticus upregulates the expression of genes encoding Zn-binding proteins in response to Zn deficiency. Bar plots representing the mean ± standard deviations of the expression levels of these genes in RIMD2210633 grown in the conditions of interest obtained through quantitative PCR. The data were obtained from three independent experiments. ***, P <0.0001.
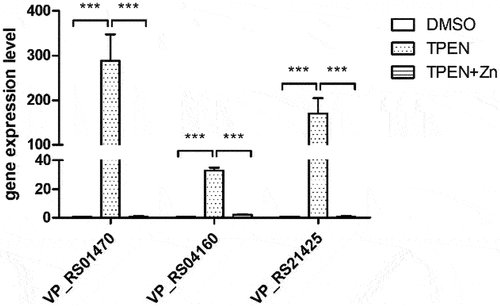
Table 1. Expression of Zur regulon under Zn-deficient conditions compared to Zn-replete conditions.
Deletion of VP_RS01470 impairs V. parahaemolyticus growth under zn-deficient conditions
To determine whether VP_RS01470 involves in Zn acquisition alongside revealing the interactions of the three Zn-binding proteins, we constructed mutants for these Zn-binding proteins in V. parahaemolyticus RIMD2210633. These included the single mutants (Δ1470, Δ4160, and Δ21425), the double mutants (Δ1470Δ4160, Δ1470Δ21425, and Δ4160Δ21425), and the triple mutant (Δ1470Δ4160Δ21425). All these mutants were confirmed by PCR (Fig. S4) and DNA sequencing.
The growth of RIMD2210633 and its derived mutants were evaluated under Zn-deficient and Zn-replete conditions. All the strains grew well and exhibited almost identical growth curves when cultured in LB pretreated with DMSO (). However, when grown in LB pretreated with TPEN, the mutants lacking either VP_RS01470 alone or VP_RS01470 together with other single or multiple genes, exhibited distinctly impaired growth (). Notably, when cultured in LB pretreated with 30 μM TPEN, Δ1470 grew better than the double and triple mutants containing a VP_RS01470 deletion (). Comparatively, the other three mutants showed similar growths compared to the WT strain (). Furthermore, adding 20 μM Zn to TPEN (35 μM)-treated LB restored the growth of the mutants lacking VP_RS01470 ().
Figure 4. VP_RS01470 is required for V. parahaemolyticus growth under Zn-deficient conditions. (a-d) the strains were grown in LB pretreated with DMSO (a), 30 μM (b), 35 μM (c), or 40 μM TPEN (d). (e) the strains were grown in TPEN (35 μM)-treated LB supplemented with 20 μM Zn. At least three independent experiments were performed for each condition. The data represent the mean ± standard deviations from three wells in an independent experiment.
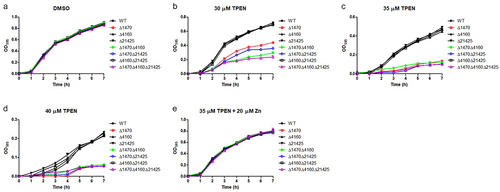
To demonstrate that the growth defect of Δ1470 is not due to polar effects or undetected mutations outside the gene, we complemented the mutant using plasmid pMMB207-1470, and repeated the growth experiments. As seen in , CΔ1470 exhibited growth curves similar to the WT strain under all tested conditions.
Figure 5. Growth curves of the V. parahaemolyticus strains under Zn-deficient and Zn-replete conditions. (a-d) the strains were grown in LB pretreated with DMSO (a), 30 μM (b), 35 μM (c), or 40 μM TPEN (d). (e) the strains were grown in TPEN (35 μM)-treated LB supplemented with 20 μM Zn. At least three independent experiments were performed for each condition. The data represent the mean ± standard deviations from three wells in an independent experiment.
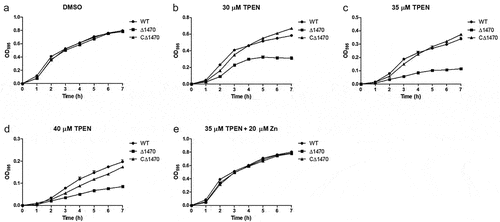
Overall, our results indicate that in V. parahaemolyticus, VP_RS01470 plays the predominant role in Zn acquisition, while VP_RS04160 and VP_RS21425 play a minor role in Zn acquisition in the case of VP_RS01470 deletion.
The reduced swimming motility of Δ1470 under zn-deficient conditions appears to be due to the growth defect of this mutant
To determine whether VP_RS01470 involves in the motility of V. parahaemolyticus, the WT, Δ1470, and CΔ1470 strains were either pierced into the semi-solid agar or spotted onto the BHI agar supplemented with DMSO, 35 μM TPEN, or 35 μM TPEN along with 20 μM Zn, respectively. The three strains exhibited similar swimming motility on the semi-solid agar plates supplemented with DMSO (). However, when the plates were supplemented with 35 μM TPEN, Δ1470 exhibited notably reduced swimming motility compared to the WT and CΔ1470 strains (). Furthermore, the swimming motility of Δ1470 was restored on the semi-solid agar plates supplemented with 35 μM TPEN along with 20 μM Zn (). Nonetheless, no marked difference in swarming motility was observed between the WT and Δ1470 strains on the BHI −2,2´-Bipyridyl−CaCl2 agar supplemented with 35 μM TPEN (Fig. S5).
Figure 6. Reduced swimming motility of VP_RS01470 deletion mutant under Zn-deficient conditions. The strains were grown on LB agar supplemented with DMSO (a), 35 μM TPEN (b), or 35 μM TPEN along with 20 μM Zn (c). The images are representative of at least three independent experiments.
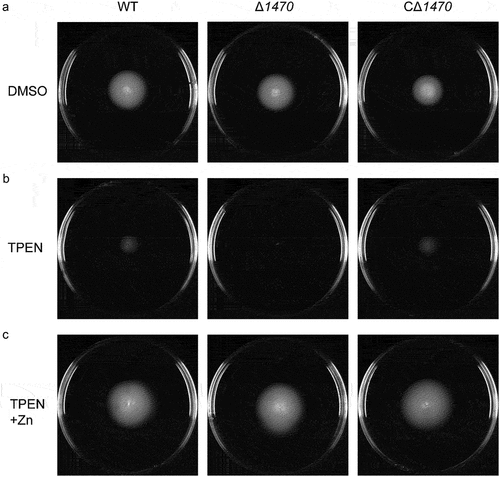
As the growth of Δ1470 is severely inhibited under Zn-deficient conditions, the reduced swimming motility of Δ1470 might be due to the attenuated growth of this mutant. In V. parahaemolyticus, the polar flagellum is involved in swimming [Citation44,Citation45]. Therefore, the expression of 12 polar flagellar genes in the WT and Δ1470 strains grown in LB pretreated with 35 μM TPEN were determined by qRT-PCR analysis. As seen in , these genes were not downregulated in Δ1470 compared to the WT strain.
Figure 7. The expression of the polar flagellar-related genes in the WT and Δ1470 strains grown under Zn-deficient conditions. The first gene in each operon was chosen for quantitative PCR analysis. Bar plots representing the mean ± standard deviations of the expression levels of these genes in the strains of interest. The data were obtained from three independent experiments. *, P <0.05; **, P <0.01; ***, P <0.001.
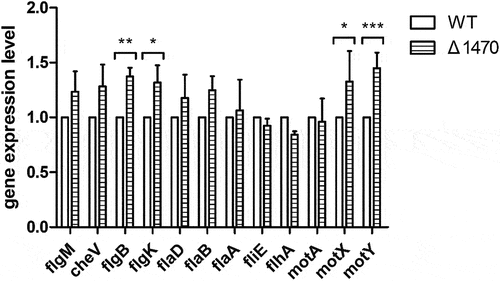
Taken together, these results suggest that the reduced swimming motility phenotype of Δ1470 under Zn-deficient conditions seems to be due to the growth defect of this mutant.
Deletion of VP_RS01470 does not affect V. parahaemolyticus virulence in mice
A mouse model was employed to evaluate the impact of VP_RS01470 deletion on V. parahaemolyticus virulence. All mice injected with PBS survived over the course of the experiment. As seen in , 40% of the WT-infected mice died within 12 h, while 30% of the mice infected with Δ1470 died within this period. The final survival rates were 40% and 20% for the WT- and Δ1470-infected mice, respectively (). Furthermore, the mice infected with the triple mutant Δ1470Δ4160Δ21425 showed a final survival rate of 30%. There was no significant difference in the survival rates of mice between the different groups. These results indicate that in this model, VP_RS01470 plays no apparent role in V. parahaemolyticus virulence.
VP_RS01470 is negatively regulated by Zur in V. parahaemolyticus
To confirm the regulation of VP_RS01470 by Zur, a zur deletion mutant (Δzur) and its complementation strain (CΔzur) were constructed (Fig. S4). qRT-PCR data showed that when cultured in LB, VP_RS01470 expression was significantly upregulated in Δzur, and downregulated in CΔzur (). To further explore the Zur-mediated regulation of VP_RS01470 in the V. parahaemolyticus response to Zn limitation, we constructed LacZ reporter strains and performed β-galactosidase activity assays. When cultured in LB pretreated with DMSO, the β-galactosidase activity of Δzur harbouring the pDM8-P1475–1455 plasmid was 2-fold higher than the WT strain harbouring the same plasmid (). Furthermore, the β-galactosidase activity of the WT strain harbouring pDM8-P1475–1455 grown in LB pretreated with 35 μM TPEN was approximately 2-fold higher than in LB pretreated with DMSO (). Conversely, the WT strain and Δzur harbouring the empty pDM8 plasmid exhibited only basal levels of β-galactosidase activity, irrespective of the LB treatment (). Altogether, these results reveal that VP_RS01470 is repressed by Zur in V. parahaemolyticus and is derepressed under Zn limitation conditions.
Figure 9. Zur Negatively regulates VP_RS01470 in V. parahaemolyticus. (a) Bar plots representing the mean ± standard deviations of the expression levels of VP_RS01470 in the strains of interest obtained through quantitative PCR. The data were obtained from three independent experiments. (b) β-galactosidase activity of the WT strain or Δzur harbouring either pDM8 or pDM8 containing the promoter of the operon VP_RS01475 to VP_RS01455. The data represent the mean ± standard deviations from three biological replicates.
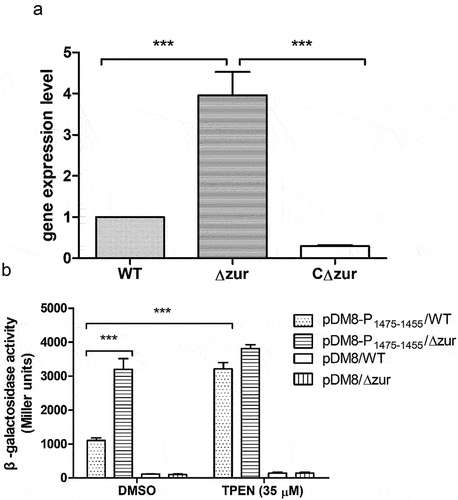
Discussion
Despite metals playing a crucial role in bacterial physiology and pathogenesis, the mechanisms of metal acquisition in V. parahaemolyticus are not fully understood. Here, the transcriptome change of V. parahaemolyticus under Zn-deficient conditions was explored in comparison with Zn-replete conditions. Ultimately, a Zn acquisition-associated protein, referred to as ZrgA, was identified in this bacterium. We demonstrated that ZrgA plays a predominant role in Zn acquisition in V. parahaemolyticus.
Microbes have evolved complex and diverse mechanisms to respond to metal restriction, including metal acquisition, mobilization, sparing, and recycling [Citation46]. Among these, metal acquisition using high-affinity uptake systems plays a dominant role [Citation13]. Thus, it was unsurprising that the expression of a number of genes was altered under Zn-deficient conditions compared to Zn-replete conditions. Further, the genes encoding Zn transporter systems were among the top upregulated genes. Our results revealed that ZrgA plays the principal role in Zn acquisition in V. parahaemolyticus, since only the mutants lacking VP_RS01470, rather than VP_RS04160 and VP_RS21425, exhibited a growth defect under the Zn limitation conditions. Moreover, when cultured in LB pretreated with 30 μM TPEN, Δ1470 grew better than the double mutants lacking VP_RS01470 and the triple mutant, indicating that during VP_RS01470 deletion, VP_RS04160 and VP_RS21425 can partly complement the function of this gene. Analysis of the WT and various mutants’ growth characteristics demonstrated that VP_RS01470 plays the predominant role in Zn acquisition in V. parahaemolyticus. However, to demonstrate that the growth defect of Δ1470 is not due to polar effects or undetected mutations outside VP_RS01470, we generated a complementation strain for Δ1470. Expectedly, the WT and complementation strains exhibited similar growth curves under Zn-deficient conditions.
Gram-positive bacteria generally utilize the AdcABC and AdcAII systems to acquire Zn. These two systems have been demonstrated as essential for Zn acquisition and virulence in Enterococcus faecalis and several streptococcal species [Citation11,Citation24,Citation47–52]. In contrast, the contribution of the ZnuABC system to Zn uptake and Zn-dependent physiological processes has been well established in Gram-negative bacteria. For example, ZnuABC is critical for biofilm formation and cell adhesion in enterotoxigenic E. coli and contributes to virulence in Chromobacterium violaceum [Citation39,Citation41]. V. cholerae possesses two Zn uptake systems, namely ZnuABC and ZrgABCDE, where ZnuABC plays the predominant role [Citation23]. Unlike the findings in V. cholerae, our results revealed that ZrgA plays the primary role in V. parahaemolyticus. Moreover, the ZrgABCDE-like systems are present in various species of Vibrionaceae, revealing that Vibrionaceae may implement this system as a secondary mechanism for Zn uptake. The product of VP_RS21425 has been characterized as a Zn transporter acquired by V. parahaemolyticus through horizontal gene transfer [Citation21]. However, no growth inhibition was observed when the VP_RS21425 gene deletion mutant was grown under Zn limitation conditions. Liu et al. used the clinical isolate VP3218 as the WT strain [Citation21], while the RIMD2210633 isolate was used in this study. One hypothesis is that the primary Zn uptake mechanism differs between V. parahaemolyticus isolates. In support of this hypothesis, the environmental and virulent Francisella species use different Zn acquisition mechanisms to adapt to Zn limitation [Citation53]. Additionally, we could not exclude the possibility that the other two Zn transporters may exert their functions under alternative circumstances.
To adapt to complex and changing environments, V. parahaemolyticus has evolved two different types of flagellar systems, i.e. the polar flagellum that is essential for swimming and the lateral flagella that are involved in swarming [Citation44,Citation45]. The regulation of swarming by Ca and Fe has been described previously in V. parahaemolyticus [Citation17]. In Proteus mirabilis, Zn acquisition contributes to swimming and swarming motility [Citation54]. The ΔznuABC mutant of Salmonella enterica serovar Typhimurium and C. violaceum exhibited decreased swimming motility under Zn limitation conditions [Citation41,Citation55]. In S. enterica serovar Typhimurium, a reduction of Zn importation downregulates the expression of flagella, thus leading to reduced motility [Citation55]. Compared to the WT and complementation strains, Δ1470 exhibited observably reduced swimming rather than swarming motility under Zn limitation conditions. However, the polar flagellar genes were not downregulated in the mutant; thus, the reduced swimming motility observed for Δ1470 could probably be an artefact of growth defect.
The contribution of Zn acquisition to the pathogenesis has been well established in bacteria [Citation14,Citation21,Citation24,Citation39,Citation41,Citation49,Citation52]. However, ZnuABC is not essential for the virulence of Y. pestis, despite that it is the predominant Zn importer during in vitro growth [Citation56]. Here, we used a mouse model to examine the impacts of ZrgA deletion on V. parahaemolyticus virulence. The results showed that ZrgA plays no noticeable role in the virulence of this bacterium in mice, as the mice infected with either Δ1470 or the triple mutant exhibited similar survival rates compared to the WT-infected mice. Given that Zn is relatively abundant at various host sites in mice [Citation57], we speculate that V. parahaemolyticus might not need the Zn transporters for the process of infection. Alternatively, additional Zn acquisition mechanisms might play a role during the infection of mice. It should be noted that the expression of VP_RS01470 in V. parahaemolyticus isolated from the caecal fluid of infant rabbits is approximately 16-fold higher than from LB cultures [Citation58]. The role of ZrgA in V. parahaemolyticus virulence can be investigated in future research using an infant rabbit model.
In V. cholerae, Zur negatively regulates the operon zrgABCDE in response to limited Zn [Citation23]. Consistent with the observations in V. cholerae, the zrgA expression was significantly upregulated in Δzur of V. parahaemolyticus. Moreover, the β-galactosidase activity suggests that zrgA is repressed by Zur under normal conditions and derepressed in Δzur or under Zn-deficient conditions. The mechanism of Zur regulation has been well illustrated in other bacteria, whereby under Zn-replete conditions, Zur binds to DNA and represses the expression of genes encoding Zn transporters. Alternatively, under Zn-deficient conditions, Zur loses its DNA-binding affinity, causing derepression of the target genes [Citation59,Citation60]. Analysis with RegPrecise identified of a putative Zur-binding site―TGAGTGTTATATTATAACACCCG in the upstream of the zrgABCDE operon. Consequently, it is likely that Zur regulates the zrgABCDE operon via a similar mechanism in V. parahaemolyticus.
In conclusion, V. parahaemolyticus upregulates the expression of Zur regulon in response to Zn limitation. Moreover, this bacterium prefers ZrgA to uptake Zn under Zn-deficient conditions.
Author contributions
CZ, XZ, and XJ conceived and designed the experiments. CZ, JQ, YZ, and MW performed the experiments. CZ, JQ, YZ, and MW analysed the data. CZ wrote the paper. XZ, and XJ revised the manuscript.
Supplemental Material
Download MS Word (2 MB)Disclosure statement
No potential conflict of interest was reported by the author(s).
Data availability statement
The authors confirm that the data supporting the findings of this study are available within the article and its supplementary materials
Supplementary Material
Supplemental data for this article can be accessed online at https://doi.org/10.1080/21505594.2022.2156196.
Additional information
Funding
References
- Letchumanan V, Chan KG, Lee LH. Vibrio parahaemolyticus: a review on the pathogenesis, prevalence, and advance molecular identification techniques. Front Microbiol. 2014;5:705.
- Zhang L, Orth K. Virulence determinants for Vibrio parahaemolyticus infection. Curr Opin Microbiol. 2013;16(1):70–14.
- Ghenem L, Elhadi N, Alzahrani F, et al. Vibrio Parahaemolyticus: a review on distribution, pathogenesis, virulence determinants and epidemiology. Saudi J Med Med Sci. 2017;5(2):93–103. DOI:10.4103/sjmms.sjmms_30_17
- Matsuda S, Hiyoshi H, Tandhavanant S, et al. Advances on Vibrio parahaemolyticus research in the postgenomic era. Microbiol Immunol. 2020;64(3):167–181. DOI:10.1111/1348-0421.12767
- Paudyal N, Pan H, Liao XY, et al. A meta-analysis of major foodborne pathogens in Chinese food commodities between 2006 and 2016. Foodborne Pathog Dis. 2018;15(4):187–197. DOI:10.1089/fpd.2017.2417
- Klein SL, West CKG, Mejia DM, et al. Genes similar to the Vibrio parahaemolyticus virulence-related genes tdh, tlh, and vscC2 occur in other vibrionaceae species isolated from a pristine estuary. Appl Environ Microb. 2014;80(2):595–602. DOI:10.1128/AEM.02895-13
- Lee CT, Chen IT, Yang YT, et al. The opportunistic marine pathogen Vibrio parahaemolyticus becomes virulent by acquiring a plasmid that expresses a deadly toxin. Proc Natl Acad Sci, USA. 2015;112(34):10798–10803. DOI:10.1073/pnas.1503129112
- Prachumwat A, Taengchaiyaphum S, Mungkongwongsiri N, et al. Update on early mortality syndrome/acute hepatopancreatic necrosis disease by April 2018. J World Aquacult Soc. 2019;50(1):5–17. DOI:10.1111/jwas.12559
- Begg SL. The role of metal ions in the virulence and viability of bacterial pathogens. Biochem Soc Trans. 2019;47(1):77–87.
- Hood MI, Skaar EP. Nutritional immunity: transition metals at the pathogen-host interface. Nat Rev Microbiol. 2012;10(8):525–537.
- Burcham LR, Le Breton Y, Radin JN, et al. Identification of zinc-dependent mechanisms used by group B streptococcus to overcome calprotectin-mediated stress. MBio. 2020;11(6):e02302–20. DOI:10.1128/mBio.02302-20
- Makthal N, Nguyen K, Do H, et al. A critical role of zinc importer AdcABC in group a Streptococcus-host interactions during infection and its Implications for vaccine development. EBioMedicine. 2017;21:131–141.
- Chandrangsu P, Rensing C, Helmann JD. Metal homeostasis and resistance in bacteria. Nat Rev Microbiol. 2017;15(6):338–350.
- Price SL, Vadyvaloo V, DeMarco JK, et al. Yersiniabactin contributes to overcoming zinc restriction during Yersinia pestis infection of mammalian and insect hosts. Proc Natl Acad Sci, USA. 2021;118(44):e2104073118. DOI:10.1073/pnas.2104073118
- Honsa ES, Johnson MD, Rosch JW. The roles of transition metals in the physiology and pathogenesis of Streptococcus pneumoniae. Front Cell Infect Microbiol. 2013;3:92.
- Karunasagar I, Joseph SW, Twedt RM, et al. Enhancement of Vibrio parahaemolyticus virulence by lysed erythrocyte factor and iron. Infect Immun. 1984;46(1):141–144. DOI:10.1128/iai.46.1.141-144.1984
- Gode-Potratz CJ, Chodur DM, McCarter LL. Calcium and iron regulate swarming and type III secretion in Vibrio parahaemolyticus. J Bacteriol. 2010;192(22):6025–6038.
- Zhou J, Lu C, Zhang D, et al. NMR-based metabolomics reveals the metabolite profiles of Vibrio parahaemolyticus under ferric iron stimulation. J Microbiol. 2017;55(8):628–634. DOI:10.1007/s12275-017-6551-z
- León-Sicairos N, Angulo-Zamudio UA, de la Garza M, et al. Strategies of Vibrio parahaemolyticus to acquire nutritional iron during host colonization. Front Microbiol. 2015;6:702.
- Andreini C, Banci L, Bertini I, et al. Zinc through the three domains of life. J Proteome Res. 2006;5(11):3173–3178. DOI:10.1021/pr0603699
- Liu M, Yan M, Liu L, et al. Characterization of a novel zinc transporter ZnuA acquired by Vibrio parahaemolyticus through horizontal gene transfer. Front Cell Infect Microbiol. 2013;3:61.
- Makino K, Oshima K, Kurokawa K, et al. Genome sequence of Vibrio parahaemolyticus: a pathogenic mechanism distinct from that of V cholerae. Lancet. 2003;361(9359):743–749. DOI:10.1016/S0140-6736(03)12659-1
- Sheng Y, Fan F, Jensen O, et al. Dual zinc transporter systems in Vibrio cholerae promote competitive advantages over gut microbiome. Infect Immun. 2015;83(10):3902–3908. DOI:10.1128/IAI.00447-15
- Zheng C, Qiu J, Zhao X, et al. The AdcR-regulated AdcA and AdcAII contribute additively to zinc acquisition and virulence in Streptococcus suis. Vet Microbiol. 2022;269:109418.
- Bu D, Luo H, Huo P, et al. KOBAS-i: intelligent prioritization and exploratory visualization of biological functions for gene enrichment analysis. Nucleic Acids Res. 2021;49(W1):W317–25. DOI:10.1093/nar/gkab447
- Zhao F, Maren NA, Kosentka PZ, et al. An optimized protocol for stepwise optimization of real-time RT-PCR analysis. Hortic Res. 2021;8(1):179. DOI:10.1038/s41438-021-00616-w
- Livak KJ, Schmittgen TD. Analysis of relative gene expression data using real-time quantitative PCR and the 2(T)(-delta delta C) method. Methods. 2001;25(4):402–408.
- Milton DL, O’Toole R, Horstedt P, et al. Flagellin a is essential for the virulence of Vibrio anguillarum. J Bacteriol. 1996;178(5):1310–1319. DOI:10.1128/jb.178.5.1310-1319.1996
- Morales VM, Bäckman A, Bagdasarian M. A series of wide-host-range low-copy-number vectors that allow direct screening for recombinants. Gene. 1991;97(1):39–47.
- Whitaker WB, Richards GP, Boyd EF. Loss of sigma factor RpoN increases intestinal colonization of Vibrio parahaemolyticus in an adult mouse model. Infect Immun. 2014;82(2):544–556.
- Heering J, Alvarado A, Ringgaard S. Induction of cellular differentiation and single cell imaging of Vibrio parahaemolyticus swimmer and swarmer cells. J Visualized Exp. 2017;123:55842. DOI:10.3791/55842
- Croxatto A, Chalker VJ, Lauritz J, et al. VanT, a homologue of Vibrio harveyi LuxR, regulates serine, metalloprotease, pigment, and biofilm production in Vibrio anguillarum. J Bacteriol. 2002;184(6):1617–1629. DOI:10.1128/JB.184.6.1617-1629.2002
- Gu D, Zhang Y, Wang Q, et al. S-nitrosylation-mediated activation of a histidine kinase represses the type 3 secretion system and promotes virulence of an enteric pathogen. Nat Commun. 2020;11(1):5777. DOI:10.1038/s41467-020-19506-1
- Xiao Y, Chen H, Nie L, et al. Identification of c-di-GMP/FleQ-regulated new target genes, including cyaA, encoding adenylate cyclase, in Pseudomonas putida. mSystems. 2021;6(3):e00295–21. DOI:10.1128/mSystems.00295-21
- Novichkov PS, Kazakov AE, Ravcheev DA, et al. RegPrecise 3.0 – a resource for genome-scale exploration of transcriptional regulation in bacteria. BMC Genomics. 2013;14(1):745. DOI:10.1186/1471-2164-14-745
- Varela PF, Velours C, Aumont-Nicaise M, et al. Biophysical and structural characterization of a zinc-responsive repressor of the MarR superfamily. PLoS ONE. 2019;14(2):e0210123. DOI:10.1371/journal.pone.0210123
- Wątły J, Potocki S, Rowińska-Żyrek M. Zinc homeostasis at the bacteria/host interface-from coordination chemistry to nutritional immunity. Chemistry. 2016;22(45):15992–10.
- Capdevila DA, Wang J, Giedroc DP. Bacterial strategies to maintain zinc metallostasis at the host-pathogen interface. J Biol Chem. 2016;291(40):20858–20868.
- Quan GM, Xia PP, Lian SQ, et al. Zinc uptake system ZnuACB is essential for maintaining pathogenic phenotype of F4ac(+) enterotoxigenic E. coli (ETEC) under a zinc restricted environment. Vet Res. 2020;51(1):127. DOI:10.1186/s13567-020-00854-1
- Goethe E, Gieseke A, Laarmann K, et al. Identification and characterization of Mycobacterium smegmatis and Mycobacterium avium subsp. paratuberculosis zinc transporters. J Bacteriol. 2021;203(11):e00049–21. DOI:10.1128/JB.00049-21
- Santos RERS, da Silva WP, Harrison S, et al. The zinc transporter ZnuABC is critical for the virulence of Chromobacterium violaceum and contributes to diverse zinc-dependent physiological processes. Infect Immun. 2021;89(11):e00311–21. DOI:10.1128/IAI.00311-21
- Goethe E, Laarmann K, Luhrs J, et al. Critical role of Zur and SmtB in zinc homeostasis of Mycobacterium smegmatis. mSystems. 2020;5(2):e00880–19. DOI:10.1128/mSystems.00880-19
- Bobrov AG, Kirillina O, Fosso MY, et al. Zinc transporters YbtX and ZnuABC are required for the virulence of Yersinia pestis in bubonic and pneumonic plague in mice. Metallomics. 2017;9(6):757–772. DOI:10.1039/C7MT00126F
- Broberg CA, Calder TJ, Orth K. Vibrio parahaemolyticus cell biology and pathogenicity determinants. Microbes Infect. 2011;13(12–13):992–01.
- Wang R, Zhong Y, Gu X, et al. The pathogenesis, detection, and prevention of Vibrio parahaemolyticus. Front Microbiol. 2015;6:144.
- Merchant SS, Helmann JD. Elemental economy: microbial strategies for optimizing growth in the face of nutrient limitation. Adv Microb Physiol. 2012;60:91–210.
- Lam LN, Brunson DN, Molina JJ, et al. The AdcACB/AdcAII system is essential for zinc homeostasis and an important contributor of Enterococcus faecalis virulence. Virulence. 2022;13(1):592–08. DOI:10.1080/21505594.2022.2056965
- Plumptre CD, Eijkelkamp BA, Morey JR, et al. AdcA and AdcAII employ distinct zinc acquisition mechanisms and contribute additively to zinc homeostasis in Streptococcus pneumoniae. Mol Microbiol. 2014;91(4):834–851. DOI:10.1111/mmi.12504
- Bayle L, Chimalapati S, Schoehn G, et al. Zinc uptake by Streptococcus pneumoniae depends on both AdcA and AdcAII and is essential for normal bacterial morphology and virulence. Mol Microbiol. 2011;82(4):904–916. DOI:10.1111/j.1365-2958.2011.07862.x
- Pan Y, Chen Y, Chen J, et al. The adc regulon mediates zinc homeostasis in Streptococcus mutans. Mol Oral Microbiol. 2021;36(5):278–290. DOI:10.1111/omi.12350
- Makthal N, Do H, Wendel BM, et al. Group a Streptococcus AdcR regulon participates in bacterial defense against host-mediated zinc sequestration and contributes to virulence. Infect Immun. 2020;88(8):e00097–20. DOI:10.1128/IAI.00097-20
- Ganguly T, Peterson AM, Kajfasz JK, et al. Zinc import mediated by AdcABC is critical for colonization of the dental biofilm by Streptococcus mutans in an animal model. Mol Oral Microbiol. 2021;36(3):214–224. DOI:10.1111/omi.12337
- Moreau GB, Qin A, Mann BJ. Zinc acquisition mechanisms differ between environmental and virulent Francisella species. J Bacteriol. 2018;200(4):e00587–17.
- Nielubowicz GR, Smith SN, Mobley HL. Zinc uptake contributes to motility and provides a competitive advantage to Proteus mirabilis during experimental urinary tract infection. Infect Immun. 2010;78(6):2823–2833.
- Ammendola S, D’Amico Y, Chirullo B, et al. Zinc is required to ensure the expression of flagella and the ability to form biofilms in. Salmonella enterica sv Typhimurium. Metallomics. 2016;8(10):1131–1140. DOI:10.1039/C6MT00108D
- Desrosiers DC, Bearden SW, Mier I, et al. Znu is the predominant zinc importer in Yersinia pestis during in vitro growth but is not essential for virulence. Infect Immun. 2010;78(12):5163–5177. DOI:10.1128/IAI.00732-10
- McDevitt CA, Ogunniyi AD, Valkov E, et al. A molecular mechanism for bacterial susceptibility to zinc. PLOS Pathogens. 2011;7(11):e1002357. DOI:10.1371/journal.ppat.1002357
- Livny J, Zhou XH, Mandlik A, et al. Comparative RNA-Seq based dissection of the regulatory networks and environmental stimuli underlying Vibrio parahaemolyticus gene expression during infection. Nucleic Acids Res. 2014;42(19):12212–12223. DOI:10.1093/nar/gku891
- Choi S, Bird AJ. Zinc’ing sensibly: controlling zinc homeostasis at the transcriptional level. Metallomics. 2014;6(7):1198–15.
- Kandari D, Joshi H, Bhatnagar R. Zur: zinc-sensing transcriptional regulator in a diverse set of bacterial species. Pathogens. 2021;10(3):344.