ABSTRACT
Polydnaviruses (PDVs), obligatory symbionts with parasitoid wasps, function as host immune suppressors and growth and development regulator. PDVs can induce host haemocyte apoptosis, but the underlying mechanism remains largely unknown. Here, we provided evidence that, during the early stages of parasitism, the activated Cotesia vestalis bracovirus (CvBV) reduced the overall number of host haemocytes by inducing apoptosis. We found that one haemocyte-highly expressed CvBV gene, CvBV-26-4, could induce haemocyte apoptosis. Further analyses showed that CvBV-26-4 has four homologs from other Cotesia bracoviruses and BV from wasps in the genus Glyptapanteles, and all four of them possessed a similar structure containing 3 copies of a well-conserved motif (Gly-Tyr-Pro-Tyr, GYPY). Mass spectrometry analysis revealed that CvBV-26-4 was secreted into plasma by haemocytes and then degraded into peptides that induced the apoptosis of haemocytes. Moreover, ectopic expression of CvBV-26-4 caused fly haemocyte apoptosis and increased the susceptibility of flies to bacteria. Based on this research, a new family of bracovirus genes, Bracovirus apoptosis-inducing proteins (BAPs), was proposed. Furthermore, it was discovered that the development of wasp larvae was affected when the function of CvBV BAP was obstructed in the parasitized hosts. The results of our study indicate that the BAP gene family from the bracoviruses group is crucial for immunosuppression during the early stages of parasitism.
Introduction
The endoparasitoid wasps have adapted various methods to adjust their host’s physiology and development to guarantee the survival of wasp offspring [Citation1,Citation2]. Haemocytes play important roles in the immune responses of insects against parasites [Citation3,Citation4]. Therefore, parasitoid wasps induce a series of changes in host haemocytes to avoid haemocyte-mediated immune responses by polydnavirus (PDV) and venom, which are injected into host larvae along with parasitoid eggs during oviposition. For example, Cotesia vestalis parasitization inhibits recognition and encapsulation of the host haemocytes [Citation5]; Diadegma semiclausum parasitization affects haematopoietic regulation and haemocyte-mediated immune responses in host larvae [Citation6]; Cotesia kariyai parasitization reduces the total haemocyte count (THC) of the hosts [Citation7]; The parasitization of Pimpla turionellae and C. vestalis alter the haemocyte population of their hosts and even the functions of the host haemocytes [Citation8,Citation9]. Among the changes of haemocyte-mediated immune responses post parasitization, apoptosis of haemocytes plays an important role, which was induced by PDVs [Citation10–12].
PDV virion particles contain multiple segments of double-stranded, superhelical DNAs and form a mutually beneficial relationship with parasitoid wasps [Citation13]. PDVs were classified into two genera: bracoviruses (BVs) and ichnoviruses (IVs) [Citation14]. PDV virions infect nearly all host tissues but the haemocytes are the most heavily infected cells [Citation15–17]. The DNAs of PDV are integrated chromosomally into host haemocytes [Citation18–20]. Their viral genes are then transcribed in the affected host cells [Citation15,Citation21], resulting in the production of virulence proteins [Citation22,Citation23] or microRNAs [Citation24].
The genome of eight BVs [Citation25–32] and five IVs [Citation29,Citation33–36] have been fully sequenced and several PDV gene families were identified [Citation37]. Gene families such as protein tyrosine phosphatases (PTPs), ankyrin-motif proteins, cys-motif proteins, and BEN-domain proteins were conserved among BVs [Citation38]. PTPs act by dephosphorylating target proteins [Citation2]. Ankyrins, which resemble Drosophila melanogaster NF-κb transcription factor inhibitors, block host immune responses, induce or inhibit the apoptosis of insect cells, and disrupt ecdysone biosynthesis [Citation39–41]. BEN-domain proteins play a crucial role in suppressing haemocyte nodule formation [Citation42]. In addition to the conserved gene families, BV genomes contain many genes that code for hypothetical proteins that lack the conserved domains to predict the functions [Citation38]. Thus, the functions of many PDV genes remain unknown.
This paper aims to reveal the functional PDV gene(s) responsible for host haemocyte apoptosis. Herein, it is demonstrated that C. vestalis reduced the host THC by apoptosis induced by C. vestalis BV (CvBV). The global transcriptome statistics of CvBV genes in host haemocytes showed that CvBV-26-4 is secreted by haemocytes and degraded into functional peptides that induced haemocyte apoptosis. Further analysis displayed that CvBV-26-4 and its analogous proteins from other BVs all contain 3 well-conserved motifs, Gly-Tyr-Pro-Tyr and function in inducing apoptosis. Hence, a new PDV gene family, Bracovirus Apoptosis-inducing Protein (BAP), was suggested for these proteins. Ectopic expression of CvBV BAP induced apoptosis of fly haemocytes and increased susceptibility of male flies to Staphylococcus aureus. Knockdown of BAP had a deleterious impact on the development of C. vestalis progeny. Thus, we inferred that the BV BAP family is important for host immunosuppression during parasitization.
Materials and methods
Insects
P. xylostella and C. vestalis were maintained in our lab as described in a previous study [Citation43]. Drosophila melanogaster stocks were raised on standard cornmeal/yeast/agar medium at 18°C. In this study, W1118 was used as the wild-type stock and Bloomington stock Hml-GAL4 (BS#8700) was also used.
Haemocyte viability and counts
About 30 parasitized or non-parasitized P. xylostella haemocytes were collected. Cell viability and number counts were determined by trypan blue staining using Cell Counter (Countstar, Shanghai, China). Hemocyte counting was done 4 h post parasitization (pp). Cell viability was determined at 6 h, 2 h, and 4 h pp. 16 repeats (collections of 30 P. xylostella haemocytes) of each group were determined.
Virus and venom collection
From the female wasps, CvBV virions were collected and incapacitated using UV light as described in a previous study [Citation44]. C. vestalis venom gland was dissected and the stored venom protein was extracted. We defined the quantity of CvBV or venom from one female as one female equivalent (FE).
Injection
The third instar larvae of P. xylostella (2 h post-ecdysis) and male flies (7 days post-eclosion) were used for injection of different fluids as previously described [Citation44].
Apoptosis detection
Haemocytes collected from 30 P. xylostella after different treatments or Drosophila larvae were suspended in 00 μL phosphate buffered saline (PBS, pH 7.4) and plated into the Lab-Tek II Chambered Cover glass (Thermo Fisher, MA, USA) for 30 min at room temperature. Apoptotic haemocytes were visualized using a terminal deoxynucleotidyl transferase dUTP nick end labelling (TUNEL) assay kit (Vazyme, Nanjing, China) according to guidelines. And 4, 6-diamidino-2-phenylindole (DAPI) (Sigma-Aldrich) was used for nuclei counterstaining. The images were captured using a confocal microscope, Zeiss LSM 800. To quantify the percentage of apoptotic haemocytes from P. xylostella and D. melanogaster, the labelled nuclei of > 3 images were counted using the Image J software.
Caspase activity assay
After 4 h post treatment, the haemocytes were collected from P. xylostella. And the Caspase-Glo® 3/7 assay Kit (Promega, USA) was used to perform the Caspase activity assay. Each group contains 15 biological replicates.
RNA extraction
To analyse gene expression at different times pp, we extracted the total RNA of P. xylostella at 0.5 h, 1 h, 2 h, 4 h, 8 h, 6 h, 4 h, 8 h, 2 h, 6 h, and 20 h pp. P. xylostella were dissected in PBS (pH 7.4) at 4 h pp and their tissues were collected to analyse gene expression in different tissues: silk gland, epidermis, midgut, fat body, central nervous system (CNS), haemocytes, testis, and malpighian tubule (MT). Total RNA was isolated using the Trizol method (Invitrogen, Carlsbad, CA, USA).
mRNA sequencing and data analysis
The haemocytes of P. xylostella after being parasitized for 6 h, 2 h, and 4 h were collected and subject to transcriptome sequencing. In detail, each group contained 3 biological replicates in the form of 200 parasitized P. xylostella. For each library preparation, a total of 1 μg RNA was used as input material. And the VAHTS mRNA-seq v2 Library Prep Kit (Vazyme, Nanjing, China) was used for library generation. Clustering of the index-coded samples was performed on a cBot Cluster Generation System (Illumina, USA) according to guidelines. Then, the sequencing was performed on an Illumina HiSeq X Ten platform using the 150 bp paired-end module.
Paired-end clean reads were mapped to the CvBV genome [Citation26] with TopHat (v2.1.1) [Citation45] using the CvBV genome index created by Bowtie (v2.1.0) [Citation46]. Raw sequencing data are available in the SRA database of NCBI (SRR10863199-SRR10863201). Cuffdiff (v2.2.1) [Citation47] was used to calculate fragments per kilobase of exon per million fragments mapped (FPKMs) for coding genes in each group.
Quantitative PCR
Quantitative PCR (qPCR) was used to validate the transcriptome data and study the expression profiles of selected genes in varying developmental stages and tissues. qPCR was performed as previously described [Citation48]. β-actin gene (GenBank accession No. AB282645) and β-tubulin gene (GenBank accession No. EU127912) of P. xylostella were used as internal controls. The relative expression levels were calculated using the 2−ΔΔCt method [Citation48].
Sequence analysis
A homolog search was carried out against other species using BLASTP (http://www.ncbi.nlm.nih.gov/), with CvBV-26-4 as the seed sequence. The signal peptide of CvBV-26-4 was predicted by SIGNALP 6.0 (http://www.cbs.dtu.dk/services/SignalP/). MEGA 7.0 software was used to perform the alignment analyses. The figures of the sequence analysis were created using Jalview [Citation49].
RNA silencing
The T7 RiboMAXTM Express kit (Promega, USA) was used to synthesize double-stranded RNA (dsRNA) of the CvBV-26-4 gene and GFP gene. Primers used for dsRNA synthesis are displayed in Table S7. Before parasitization, 500 ng of dsCvBV-26-4 was injected into the middle 3rd instar larvae of P. xylostella, and dsGFP was used as the negative control. The efficacy of the RNA interference was determined by qPCR at 4 h pp.
Recombinant baculovirus concentration
The vector, pFASTBAC-HTb (Invitrogen, CA, USA), was used for baculovirus expression in P. xylostella haemocytes. We modified pFASTBAC-HTb by inserting the open reading frames (ORFs) of GFP, CvBV-2-1, CvBV-3-5, and CvBV-26-4. Whole cDNA sequences of CCQ71098.1 from CcBV and CCQ19291.1 from C. sesamiae Kitale bracovirus (CsKBV) were synthesized by a simplified gene synthesis method. CCQ71098.1 and CCQ19291.1 were also inserted into the pFASTBAC-HTb. The production and concentration of recombinant baculovirus were performed as previously described [Citation21,Citation50].
Western blot
A polyclonal antiserum against CvBV-26-4 was generated by the Shanghai Sangon Biotechnology Company using a peptide antigen for antiserum development. Epitopes are predicted by the GenScript Optimum Antigen design tool. The peptide sequence synthesized for the CvBV-26-4 antiserum development was YPYPSSDGSSGYFS.
Haemocytes and plasma of P. xylostella were separated by centrifugation. After adding SDS PAGE Loading Buffer, the samples were boiled for 0 min to denature the proteins. The western blot was performed as previously described [Citation21]. In this study, polyclonal antiserum against CvBV BAP (1:500) and actin (1:3000), and secondary antibody against rabbit IgG (1:3000) were used. The ECL western blotting substrate (Promega, USA) was used to visualize the bands.
Mass spectrometry of plasma peptides
To confirm whether CvBV-26-4 protein was secreted outside haemocytes and degraded into peptides, we collected peptides from the plasma of P. xylostella.~0 μl of haemolymph from 4 h pp P. xylostella larvae was added to 00 μl anticoagulant buffer [Citation44]. The diluted haemolymph was centrifuged at 000 g at 4 ℃ for 5 min to obtain the plasma in the supernatant. The peptides from the plasma were isolated with a 3 kDa Millipore ultrafiltration device. The peptide mixtures were subjected to mass spectrometry analysis using a Thermo Scientific Easy nanoLC 1000 (Thermo Fisher Scientific, MA, USA). Peptide-spectrum matches (PSMs) were set to 10.
Ectopic expression of CvBV-26-4 in drosophila melanogaster
TheORF sequence of CvBV-26-4 was cloned into the pUAST-attb vector [Citation51] to obtain the CvBV BAP transgenic lines. Integrase-mediated insertion by phiC31 into the attP2 landing-site locus on the 3rd chromosome was used to obtain the transgenic Drosophila line carrying the UAS-BAP gene.
The survival assay was conducted as described [Citation52]. Briefly, each male fly at 7 days post-eclosion was injected with 40 nL of the Staphylococcus aureus resuspension using an Eppendorf Femtojet (Eppendorf, Germany). The death was recorded every 2 h; flies that died within the first 6 h were removed. Flies were kept at 25°C and were transferred to new food every day.
CvBV-26-4 peptide rescue experiments
Each middle 3rd P. xylostella larva was injected with 0.1 μg of CvBV-26-4 antiserum and 0.1 μg of CvBV-26-4 peptide, and then parasitized by C. vestalis. Haemocytes collected from 30 P. xylostella at 4 h pp were used for apoptosis detection, as mentioned in the apoptosis detection section.
Determination of C. vestalis development
To detect the effects of CvBV-26-4 on C. vestalis offspring, 0.1 μg each of CvBV BAP antiserum and rabbit IgG (negative control) were injected into the middle 3rd instar P. xylostella, which were then parasitized by C. vestalis. The body length of wasp pupae was measured one day after pupation. The rate of wasp pupa formation was recorded. The eclosion of C. vestalis was also noted following wasp larva pupation.
Statistical analysis
All statistical analyses were performed using SPSS 20.0 software. Data were presented as means ± SD and were examined by the One-way ANOVA and Tukey’s test, with a p-value of 0.05 as the significance threshold. Different survival curves were compared using log rank tests. Chi-squared tests were used to assess the rate of wasp pupa formation and eclosion.
Results
Parasitization reduced the total count of host haemocytes through apoptosis
We analysed the total number of haemocytes from parasitized host larvae at 4 h pp by trypan blue staining using Cell Counter. The results showed that the overall number of haemocytes of parasitized host larvae was less than that of the control group (). As the number of haemocytes decreased within 4 h pp, the survival ratio of haemocytes should be affected earlier after parasitization. As expected, the survival ratio of haemocytes started to be affected at 2 h pp (). The survival ratio of haemocytes from parasitized host larvae at 4 h pp was below 70% (). Additionally, the detection of apoptosis at 4 h pp by the TUNEL assay revealed many positive haemocytes from parasitized host larvae, whereas most haemocytes from non-parasitized host larvae were negative ( and S1a). Furthermore, we detected the caspase activity of haemocytes from P. xylostella at 4 h pp and the result showed that parasitization induced the caspase activity of host haemocytes (Figure S2a).
Figure 1. Parasitization reduced the total count of P. xylostella haemocytes via apoptosis. (a) the total haemocyte count (THC) of P. xylostella at 4 h pp by C. vestalis. (b) the survival ratio of P. xylostella haemocytes at 6 h, 2 h, and 4 h pp. Error bars indicate ± SD. Differences among samples were tested with tukey-test (ns: no significance; *** p<0.001). (c) TUNELlabeling to observe changes of P. xylostella apoptotic haemocytes at 4 h pp. Hemocytes were dissected and isolated, nuclei were stained with DAPI (blue), and apoptotic cells were labelled by TUNEL (red) (scale bar = 0 μm).
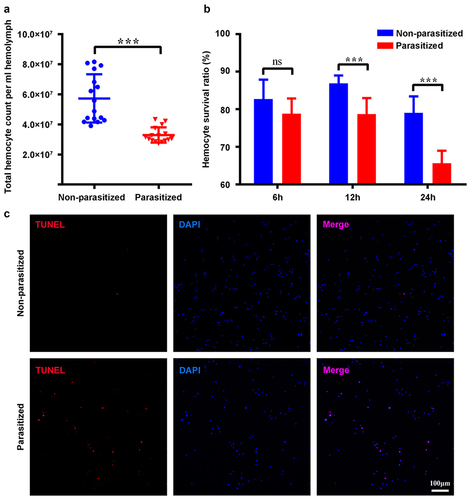
The activated CvBV-induced apoptosis of host haemocytes
The injection of CvBV or venom into host larvae during wasp oviposition might induce apoptosis of host haemocytes. To evaluate the effects of CvBV and venom on the apoptosis of host haemocytes pp, we detected the apoptotic haemocytes of P. xylostella following CvBV and venom injection by a TUNEL assay. The results showed that 0.05 FE CvBV, a dosage close to that administered during real parasitism [Citation44], induced apoptosis of haemocytes while 0.05 FE venom or PBS could not ( and S1b). Further analyses indicated that apoptosis of host haemocytes was not induced by 0.05 FE inactivated CvBV ( and S1b), which suggested that apoptosis was induced by virulent CvBV instead of its capsid proteins.
Figure 2. The activated CvBV induced apoptosis of host haemocytes. TUNEL apoptotic labelling to observe changes of apoptotic haemocytes of P. xylostella at 4 h post-injection of PBS, venom, inactivated and activated CvBV. The dosages of CvBV and venom were 0.05 FE. CvBV virions were put under UV-light for 2 hours to incapacitate the virus. Hemocytes were dissected and isolated, nuclei were stained with DAPI (blue), and apoptotic cells were labelled by TUNEL (red) (scale bar = 0 μm).
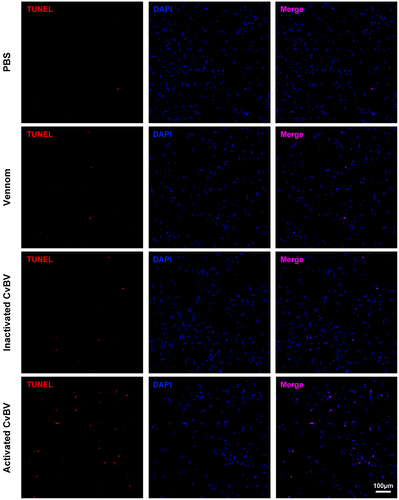
Global transcriptome analysis of CvBV genes in P. xylostella haemocytes
RNA-seq analyses of host haemocytes were performed to elucidate genes or gene families of CvBV that induce apoptosis of host haemocytes. The target gene or genes should be abundantly expressed before 2 h pp, at which time the survival ratio of haemocytes is affected (). The Illumina HiSeq X Ten platform was used to sequence the mRNA of haemocytes from host larvae at 6 h, 2 h, and 4 h pp to obtain the gene expression profiles. A total of 537,693,406 clean reads were obtained from 9 cDNA libraries (Table S8). The lowest number of clean reads obtained from sample 6 h-3 nearly reached 55 million, and the highest number of reads obtained from sample 4 h-1 was more than 67 million. A total of 16,810,694 reads (3.13%) corresponding to the CvBV genome were obtained from 9 libraries (Table S1).
The dynamic changes of CvBV gene expression profiles at different times pp were analysed. Among 157 predicted CvBV genes [Citation26], 140 were detected in haemocytes at 6 h, 2 h, and 4 h pp. Their FPKMs are shown in Tables S9, S10, and S11. Global transcriptome statistics of CvBV gene transcripts showed that the relative expression levels of various CvBV genes differed at different times pp (). Based on the differences between gene expression levels in haemocytes, CvBV genes were classified into 4 categories, high (FPKM≥1000), medium (1000>FPKM≥100), low (100>FPKM≥10), and marginal (10>FPKM>0). To validate the results analysed from transcriptomes, 16 CvBV genes were randomly selected across the observed range of expression for qPCR analysis of the cDNA samples from haemocytes used for sequencing. These qPCR analyses confirmed the trends observed from transcriptome analyses (). The specific number of CvBV genes expressed in host haemocytes at 6 h, 2 h, and 4 h pp was 129, 124, and 133, respectively (Figure S3a). There were 14 CvBV genes expressed in haemocytes at a high level within the observed 4 h pp (Figure S3b).
Figure 3. Expression levels of CvBV genes in P. xylostella haemocytes. CvBV gene expression levels in P. xylostella haemocytes at 6 h (a), 2 h (b), and 4 h (c) pp by C. vestalis. The transcripts were classified according to their location in the genome. 35 non-redundant circular CvBV genome was represented as linear molecules to visualize size and scale bar is in bps. For each circle, grey lines represent intergenic regions and different colours indicate the FPKMs of CvBV genes (see the list in S9, S10, and S11 Tables). Relative expression levels of CvBV genes in P. xylostella haemocytes were analysed using qPCR at 6 h (d), 2 h (e), and 4 h (f) pp. The right y-axis represents their FPKMs (green line) based on transcriptome data and the left ordinate represents relative expression levels (yellow columns). Error bars indicate ± SD.
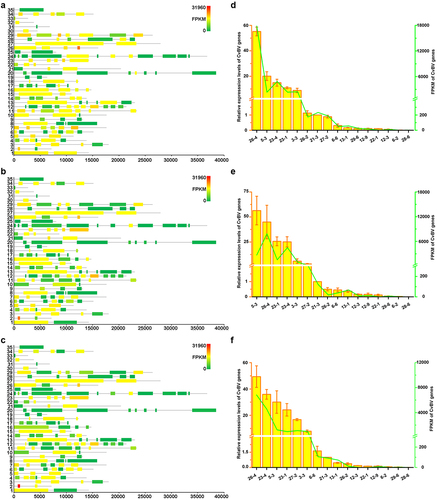
CvBV-26-4 induced apoptosis of P. xylostella haemocytes
Among the top 10 CvBV genes highly expressed in host haemocytes at 6 h pp, CvBV-2-1, CvBV-3-5, and CvBV-26-4 were the three most highly expressed within 4 h pp (Figure S4), indicating that these three genes might be involved in the apoptosis of host haemocytes. To figure out which gene was involved in inducing apoptosis of host haemocytes, CvBV-2-1 (GenBank accession No. AEE09453.1), CvBV-3-5 (GenBank accession No. GU299780.1), and CvBV-26-4 (GenBank accession No. AEE09579.1) were firstly cloned from parasitized host larvae, and 104 pfu baculoviruses inserted with GFP, CvBV-2-1, CvBV-3-5, and CvBV-26-4 were then injected into middle 3rd instar P. xylostella, respectively. Host haemocytes at 4 h post-injection (pi) were used for apoptosis detection. The results showed that only CvBV-26-4 was involved in the apoptosis of host haemocytes ( and S1c).
Figure 4. Overexpression of CvBV-26-4 induced apoptosis of P. xylostella haemocytes. The TUNEL apoptotic labelling to observe changes of haemocytes infected by modified baculoviruses (Bac-GFP, Bac-CvBV-2-1, Bac-CvBV-3-,5 and Bac-CvBV-26-4). Hemocytes were dissected and isolated, nuclei were stained with DAPI (blue), and apoptotic cells were labelled by TUNEL (red) (scale bar = 0 μm).
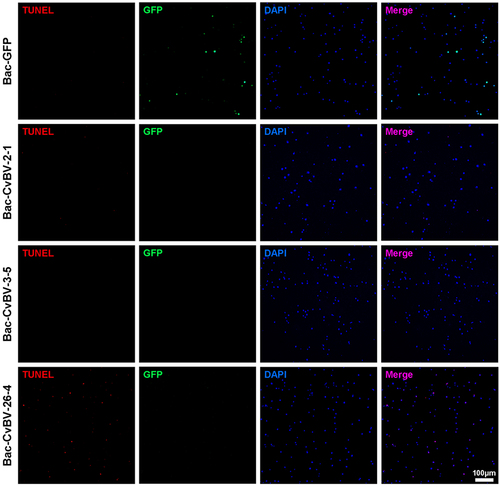
Furthermore, the expression levels of CvBV-26-4 in parasitized host larvae at different times were determined with the qPCR analysis. Our analyses showed that CvBV-26-4 was expressed abundantly at 4 h pp and rapidly decreased by 8 h pp (). However, six more sample time points within 4 h pp were refined to determine the transcript profiles of CvBV-26-4 at the earlier stage. CvBV-26-4 expression level increased rapidly at 4 h pp (). qPCR analysis showed that the transcript level of CvBV-26-4 was much higher in the haemocytes at 4 h pp than that in other tissues (), suggesting that CvBV-26-4 plays an important role in the apoptosis of host haemocytes during the early stages of parasitism.
Figure 5. Expression profile of CvBV-26-4 in P. xylostella. (a) CvBV-26-4 expression profile in P. xylostella at 4 h, 8 h, 2 h, 6 h, and 20 h pp. (b) CvBV-26-4 expression profile in P. xylostella at 0.5 h, 1 h, 2 h, 4 h, 8 h, and 6 h pp. (c) the expression profile of CvBV-26-4 was measured by qPCR in 8 tissues of P. xylostella at 4 h pp including the epidermis, silk gland, fat body, midgut, haemocytes, central nervous system (CNS), malpighian tubules (MT), and testis. Error bars indicate ± SD (n = 3). Samples were compared with the tukey-test (**: indicates samples with a significant difference p<0.01).
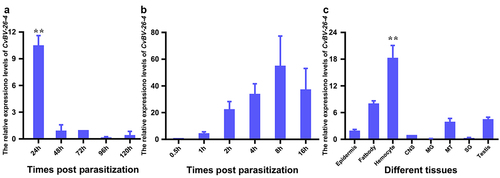
To further test whether the apoptosis was caused by CvBV-26-4, gene silencing was conducted via dsRNA injection, while it was discovered that neither the transcript level of CvBV-26-4 nor its protein content was affected (and S5). Then, prior to parasitization, we interfered with CvBV-26-4 via injecting the CvBV-26-4 antiserum, an injection of rabbit IgG that served as a negative control. We detected fewer TUNEL positive haemocytes after injection of the CvBV-26-4 antiserum compared with negative control (), which suggests that CvBV-26-4 indeed caused the apoptosis of host haemocytes.
Figure 6. CvBV-26-4 is involved in the apoptosis of P. xylostella haemocytes. (a) the expression levels of CvBV-26-4 were measured by qPCR 4 h after the interference of CvBV-26-4 was introduced. Error bars indicate ± SD (n = 3). (b) Western blot of CvBV-26-4 in host haemolymph 4 h after the interference of CvBV-26-4. (c) TUNEL labelling of apoptotic haemocytes from P. xylostella at 4 h pi with rabbit IgG or CvBV-26-4 antiserum. Hemocytes were dissected and isolated, nuclei were stained with DAPI (blue), and apoptotic cells were labelled by TUNEL (red) (scale bar = 0 μm). (d) the percentage of apoptotic haemocytes from P. xylostella at 4 h post-injection of normal rabbit IgG or the CvBV-26-4 antiserum (n = 10). Differences among samples were tested with tukey-test (***: statistically significant p<0.001).
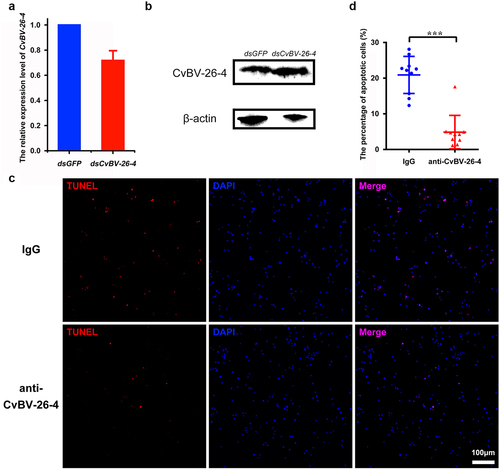
CvBV-26-4 homologs induced apoptosis of P. xylostella haemocytes
When using BLASTP to conduct the homolog search, four homologs of CvBV-26-4 were identified: two homologs from Cotesia BV (GenBank accession No. CCQ19291.1 and CCQ71098.1) and two homologs from the genus Glyptapanteles (GenBank accession No. ACE75128.1 and ABK57046.1), BV-carrying wasps. We aligned the amino acid sequences of these homologs with CvBV-26-4 to clarify whether there are common structural features. The alignment showed that the selected homologs possessed the same signal peptide and 3 well-conserved Gly-Tyr-Pro-Tyr (GYPY) motifs (). To assess whether the two homologous proteins from Cotesia BV have a similar function to CvBV-26-4, 104 pfu baculoviruses modified with the insertion of GFP, CvBV-26-4, CcBV_CCQ71098.1, and CsKBV_CCQ19291.1 were injected into middle 3rd instar P. xylostella, respectively. The expression of CvBV-26-4 was confirmed by western blotting (Figure S6). Host haemocytes at 4 h pi were observed for apoptosis detection and the results showed that CvBV-26-4, CcBV_CCQ71098.1, and CsKBV_CCQ19291.1 can induce apoptosis of haemocytes while GFP cannot ( and S1c). With common structural features and a similar function, these proteins were therefore named Bracovirus Apoptosis-inducing Proteins (BAPs).
Figure 7. CvBV-26-4 homologs induced apoptosis of P. xylostella haemocytes. (a) amino acid sequence alignments of CvBV-26-4 and its homologs in Cotesia and glyptapanteles. The red box indicates the signal peptides. GYPY motifs conserved in CvBV-26-4 and its homologs are marked by red **** above the sequences. The numbers on the right are the position of the final amino acid. (b) the TUNEL apoptotic labelling of haemocytes at 4 h post-infection by modified baculoviruses (Bac-GFP, Bac-CcBV_CCQ71098.1, and Bac-CsKBV_CCQ19291.1). Hemocytes were dissected and isolated, nuclei were stained with DAPI (blue), and apoptotic cells were labelled by TUNEL (red) (scale bar = 0 μm).
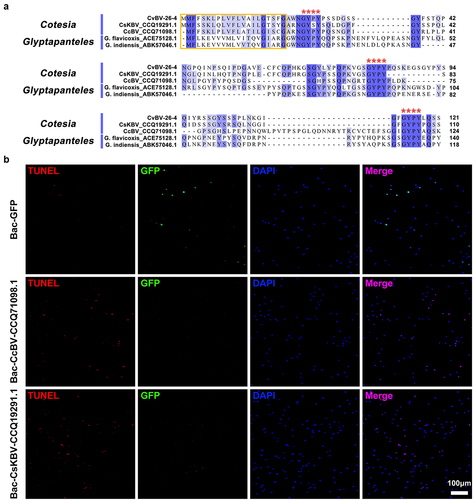
CvBV BAP peptide induced apoptosis of P. xylostella haemocytes
As CvBV BAP (CvBV-26-4) contains a signal peptide, it was hypothesized that CvBV BAP may be secreted and function outside of haemocytes. In the host haemocytes and plasma, CvBV BAP protein content was detected at 6 h, 2 h, and 4 h pp. The CvBV BAP proteins in the host haemocytes and plasma were detected at 6 h pp, accumulated rapidly by 2 h pp, and were maintained at a high level at 4 h pp ( and S7). We wonder if the CvBV BAP proteins could be degraded into peptides in host plasma but not in host haemocytes. We collected peptides of host plasma at 4 h pp for mass spectrometry. One peptide of CvBV BAP (peptide-spectrum matches, PSMs = 14) was identified (). The CvBV BAP peptide contained 18 amino acids, SSGYSSSPLNKGIGFGYP, which is at the C terminus of the CvBV BAP protein. Apoptosis detection of host haemocytes at 4 h pi of BAP peptide showed that CvBV BAP peptide can induce apoptosis of haemocytes ( and S1d). And the caspase activity of P. xylostella also increased post injection of the BAP peptide (Figure S2b). Injection of the CvBV BAP antiserum can rescue the apoptosis haemocytes from parasitized P. xylostella (), but injection of CvBV BAP antiserum can not rescue the apoptosis of haemocytes from parasitized P. xylostella when co-injected with the BAP short active peptide ( and S1d).
Figure 8. CvBV-26-4 peptide induced apoptosis of P. xylostella haemocytes. (a) amount of CvBV-26-4 protein in the haemocytes (top) and plasma (bottom) of P. xylostella at 6 h, 2 h, and 4 h pp. A band in the size range of 13 kDa that corresponds to the predicted CvBV-26-4 protein. Western blot are reported in supplementary information (figure S7). (b) the locations of CvBV 26–4 peptide (red) identified in the plasma of P. xylostella at 4 h pp by mass spectrometry. PSMs = 14. The conserved GYPY motifs were marked by underline. (c) the TUNEL apoptotic labelling of apoptotic haemocytes of P. xylostella at 4 h post-injection of the CvBV-26-4 peptide. (d) TUNEL labelling of apoptotic haemocytes in parasitized P. xylostella 4 h post-injection with CvBV-26-4 antibody and CvBV-26-4 peptide. Hemocytes were dissected and isolated, nuclei were stained with DAPI (blue), and apoptotic cells were labelled by TUNEL (red) (scale bar = 0 μm).
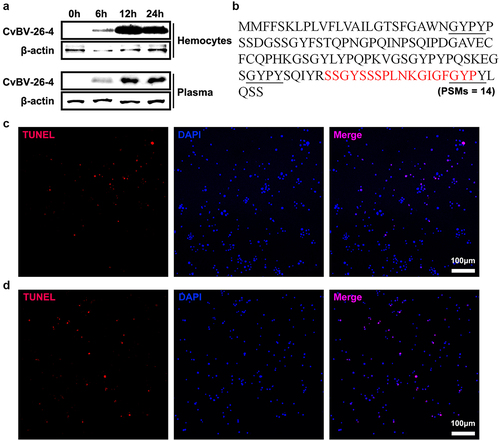
CvBV BAP induced apoptosis of D. melanogaster haemocytes
To confirm the function of CvBV BAP proteins in non-adapted hosts, D. melanogaster, we used the GAL4/UAS binary expression system [Citation53]. A D. melanogaster transgenic cell line carrying a UAS transgene encoding the CvBV BAP protein was produced. Then, we used a haemocyte specific expression driver, Hml-GAL4, to express UAS-BAP in D. melanogaster haemocytes [Citation54]. Morphological observation showed that haemocytes from the 3rd instar Hml>BAP larvae rarely keep normal forms (). The aberrant morphology phenotype was caused by apoptosis (). In addition, a survival assay was used to determine the effect of CvBV BAP on D. melanogaster haemocytes. The susceptibility to S. aureus of Hml>BAP flies was increased compared to controls ( and S1e).
Figure 9. CvBV-26-4 induced apoptosis of D. melanogaster haemocytes. (a) morphologies of 3rd instar fly haemocytes that ectopically expressed CvBV-26-4 (scale bar = 0 μm). (b)apoptotic labelling of Drosophila larvae blood cells that ectopically expressed CvBV-26-4. Hemocytes were dissected and isolated, nuclei were stained with DAPI (blue), and apoptotic cells were labelled by TUNEL (red) (scale bar = 0 μm). (c) representative survival graphs of adult male Drosophila ectopically expressing CvBV-26-4 after injection of S. aureus (OD600 0.4). n = 20–25 flies. Experiments were performed in triplicate. (d) apoptotic staining of haemocytes from 3rd instar Drosophila larvae (W1118) at 4 h post-injection of 0.1 μg CvBV BAP peptide (injection of PBS was used as a negative control). Hemocytes were dissected out and nuclei were stained with DAPI (blue) and apoptotic cells were labelled by TUNEL (red) (scale bar = 0 μm).
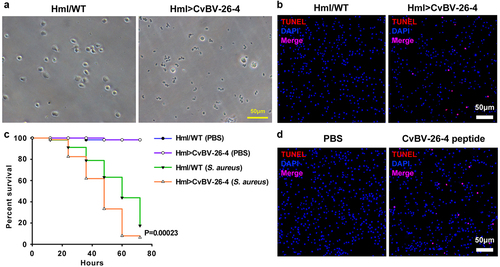
The TUNEL signal was detected in haemocytes from 3rd instar D. melanogaster larvae 4 h post CvBV BAP (0.1 μg) injection showing that CvBV BAP can induce apoptosis of D. melanogaster haemocytes ( and S1f).
Effects of CvBV BAP on parasitization
For detecting the effect of CvBV BAP on parasitization, the CvBV BAP antiserum was injected into the middle 3rd instar larvae of P. xylostella which were then parasitized by C. vestalis. The body lengths of the wasp pupae, the wasp pupa formation, and the eclosion of C. vestalis were analysed to evaluate the wasp development. The results showed that the body lengths of wasp pupae were significantly decreased post-injection of BAP antiserum compared to the control group because of the blocked function of CvBV BAPs (). Injection of CvBV BAP antiserum reduced the percentage of wasp pupa formation () as well as the eclosion rate of C. vestalis ().
Figure 10. Interference of CvBV-26-4 on wasp development. 3rd instar P. xylostella larvae were injected with CvBV-26-4 antibody or rabbit IgG before parasitization. (a) body lengths of 1-day wasp pupae (µm). Error bars indicate ± SD. Differences among samples were tested with tukey-test (**: significant difference between samples p<0.01). Rate of wasp pupa formation (b) and eclosion rate of C. vestalis (c) in 3rd instar P. xylostella larvae injected with CvBV-26-4 antibody or rabbit IgG before parasitization. Differences among samples were tested with the chi-squared test. (*: significant difference between samples p <.
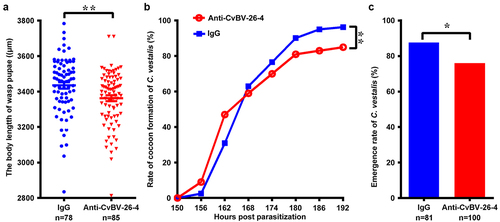
Discussion
The total number of haemocytes including granular cells and plasmatocytes decreased significantly in 4th instar host larvae parasitized by C. vestalis [Citation9]. This study found that parasitization affected the THC of P. xylostella much earlier in development. The total number of haemocytes from the late 3rd host larvae at 4 h pp decreased and the survival ratio of haemocytes was affected at 2 h pp. It was similar to the reports indicating that the number of host haemocytes decreased during the early stages of parasitism by C. melanoscela [Citation55], Campoletis sonorensis [Citation56], C. kariyai [Citation7], and Pimpla turionellae [Citation8]. The THC of P. xylostella larvae was reduced because of apoptosis caused by parasitization, which was consistent with that found in Pseudoplusia includes [Citation11] and Spodoptera litura [Citation12]. Inducing apoptosis of host haemocytes is an important strategy for immunosuppression.
C. vestalis has three important wasp-associated factors which influence the physiology and development of host larvae, including CvBV, venom, and teratocytes [Citation57]. Teratocytes are released into the host when the wasp egg hatches [Citation58], while venom and PDV function earlier. Our results of different injections showed that the activated CvBV induced apoptosis of host haemocytes. In the CvBV genome, 157 ORFs were identified [Citation26]. RNA-seq analyses of the host haemocyte transcriptomes showed that CvBV-26-4 might be involved in the induction of apoptosis of host haemocytes. It is not easy to silence CvBV-26-4 via injection of dsRNA, so we injected its antiserum to block functional CvBV-26-4. As expected, apoptotic haemocytes were reduced by nearly 75% after injection of the CvBV antiserum, which indicated that CvBV-26-4 was one of the key genes inducing apoptosis during the early stage of parasitism.
CvBV-26-4 homologs were found in two Cotesia BVs and two Glyptapanteles wasps. The homologs from CcBV and CsKBV also induced apoptosis of P. xylostella haemocytes. Therefore, we named these proteins as Bracovirus Apoptosis-inducing Proteins (BAPs). These BAP genes may belong to a new gene family of BVs. It is not surprising that BAPs were found only in Glyptapanteles and Cotesia wasps because Glyptapanteles is the sister genus to Cotesia (Figure S8) [Citation59], which suggests that other variants may exist given the species diversity in these two genera. We proposed that BAPs might have originated between 54 Mya and 17 Mya based on the phylogeny of the microgsterid lineage (Figure S8).
The transcript level of CvBV BAP in the haemocytes of parasitized P. xylostella at 6 h pp was higher than that at 2 h pp, but BAP protein content in the parasitized host haemocytes was most abundant at 2 h pp when the survival ratio of haemocytes started to be affected, which suggests that BAP proteins were accumulated. An unexpected finding was that CvBV BAP degraded into a functional peptide in host plasma. PDV proteins secreted by haemocytes into plasma could affect a greater number of haemocytes and possibly other tissues. For example, CvBV BAP peptides may be a ligand for some receptors, which can induce apoptosis. Since CvBV BAP peptide was detected in the plasma (), but not in the haemocytes (mass spectrometry analysis, data not shown), and the precursor of BAP has a predicted signal peptide, we hypothesized that the precursor of BAP was secreted into plasma and degraded into the active forms. The antiserum made against a different part of the BAP protein may bind BAP secreted into the haemolymph to prevent the processing of BAP into the active forms, which would explain why the antiserum is able to inhibit the protein’s function (). In our study, the level of baculovirus infection is not high, but Bac-CvBV-26-4-induced apoptosis was observed in a large number of host haemocytes, which confirms our hypothesis. It is worth noting that a fraction of CvBV BAP may get into plasma due to cell lysis for more apoptotic haemocytes post parasitization. Further work is required to confirm that the degradation happens in the haemolymph and is necessary for function, to determine which proteases cleave CvBV BAP, and to identify the receptor of the CvBV BAP peptide.
CvBV BAP can also induce apoptosis of Drosophila haemocytes, which suggests that CvBV BAP could affect some conserved factors in the apoptosis pathway. The development of wasp larvae in the host was affected when the function of CvBV BAP was blocked. The host immunity response increased at the early stage of parasitism for the blocking of CvBV BAP, which results in shorter body lengths of wasp pupae, a lower percentage of wasp pupation, and a reduced rate of pupa to adult eclosion.
In summary, a new gene family from BVs was identified: the BAP family. Genes from the BAP family encoded proteins containing GYPY motifs, as well as proteins that induced apoptosis of P. xylostella haemocytes. CvBV BAP was secreted into host haemocoel and degraded into functional peptides to induce apoptosis. The BAP gene family is important for the successful parasitization of Cotesia wasps for its function in immunosuppression.
Author contributions
Conception and design of study, X-xC and J-hH. Acquisition and analysis of data, Z-hW, X-tW, and X-qY; Drafting the manuscript, Z-hW, X-tW and Z-zW. All authors have read and agreed to the published version of the manuscript.
Supplemental Material
Download MS Word (1.7 MB)Acknowledgments
We thank Drs. Mike Strand and Jean-Michel Drezen for discussion and suggestions during the course of this study.
Disclosure statement
No potential conflict of interest was reported by the authors.
Data availability statement
The raw data for mRNA sequencing of P. xylostella haemocytes are deposited at SRA database of NCBI at https://www.ncbi.nlm.nih.gov, with accession number SRR10863199~SRR10863201.
Supplementary material
Supplemental data for this article can be accessed online at https://doi.org/10.1080/21505594.2023.2171691
Additional information
Funding
References
- Pennacchio F, Strand MR. Evolution of developmental strategies in parasitic hymenoptera. Annu Rev Entomol. 2006;51(1):233–16.
- Ye XQ, Shi M, Huang JH, et al. Parasitoid polydnaviruses and immune interaction with secondary hosts. Dev Comp Immunol. 2018;83:124–129.
- Strand MR, Pech LL. Immunological basis for compatibility in parasitoid-host relationships. Annu Rev Entomol. 1995;40(1):31–56.
- Lavine MD, Strand MR. Insect hemocytes and their role in immunity. Insect Biochem Mol Biol. 2002;32(10):1295–1309.
- Bai S, Li X, Tang L, et al. Immunosuppressive factors carried by eggs of the parasitoid wasp Cotesia vestalis (Hymenoptera: braconidae). Acta Entomologica Sinica. 2009;52(5):487–494.
- Huang F, Shi M, Yang YY, et al. Changes in hemocytes of Plutella xylostella after parasitism by Diadegma semiclausum. Arch Insect Biochem Physiol. 2009;70(3):177–187.
- Teramoto T, Tanaka T Mechanism of reduction in the number of the circulating hemocytes in the Pseudaletia separata host parasitized by Cotesia kariyai. J Insect Physiol. 2004;50(12):1103–1111.
- Er A, Uçkan F, Rivers DB, et al. Effects of parasitization and envenomation by the endoparasitic wasp Pimpla turionellae (Hymenoptera: ichneumonidae) on hemocyte numbers, morphology, and viability of its host Galleria mellonella (Lepidoptera: pyralidae). Ann Entomol Soc Am. 2010;103(2):273–282.
- Ibrahim AMA, Kim Y. Parasitism by Cotesia plutellae alters the hemocyte population and immunological function of the diamondback moth plutella xylostella. J Insect Physiol. 2006;52(9):943–950.
- Suderman RJ, Pruijssers AJ, Strand MR. Protein tyrosine phosphatase-H2 from a polydnavirus induces apoptosis of insect cells. J Gen Virol. 2001;89(6):1411–1420.
- Strand MR, Pech LL. Microplitis demolitor polydnavirus induces apoptosis of a specific haemocyte morphotype in Pseudoplusia includens. J Gen Virol. 1995;76(2):283–291.
- Luo KJ, Pang Y. Spodoptera litura multicapsid nucleopolyhedrovirus inhibits Microplitis bicoloratus polydnavirus-induced host granulocytes apoptosis. J Insect Physiol. 2006 Aug;52(8):795–806.
- Strand MR, Burke GR. Polydnaviruses: from discovery to current insights. Virology. 2015;479-480:393–402. DOI:10.1016/j.virol.2015.01.018
- Francki RIB, Fauquet CM, Knudson DL, et al. Classification and nomenclature of viruses: fifth report of the international committee on taxonomy of viruses for virology division of the international union of microbiological societies. Springer-Verlag. 1991; Berlin.
- Bitra K, Zhang S, Strand MR. Transcriptomic profiling of Microplitis demolitor bracovirus reveals host, tissue and stage-specific patterns of activity. J Gen Virol. 2011;92(9):2060–2071.
- Beck MH, Inman RB, Strand MR Microplitis demolitor bracovirus genome segments vary in abundance and are individually packaged in virions. Virology. 2007;359(1):179–189. DOI:10.1016/j.virol.2006.09.002
- Strand MR. Microplitis demolitor polydnavirus infects and expresses in specific morphotypes of Pseudoplusia includens hemocytes. J Gen Virol. 1994;75:3007–3020.
- Chevignon G, Periquet G, Gyapay G, et al. Cotesia congregata bracovirus circles encoding PTP and Ankyrin genes integrate into the DNA of parasitized Manduca sexta hemocytes. J Virol. 2018;92(15): e00438-18.
- Wang ZH, Zhou YN, Yang J, et al. Genome-wide profiling of Diadegma semiclausum ichnovirus integration in parasitized Plutella xylostella hemocytes identifies host integration motifs and insertion sites. Front Microbiol. 2021;11:608346.
- Wang ZH, Ye XQ, Zhou YN, et al. Bracoviruses recruit host integrases for their integration into caterpillar’s genome. PLoS Genet. 2021;17(9):e1009751.
- Wang ZH, Zhou YN, Ye XQ, et al. CLP gene family, a new gene family of Cotesia vestalis bracovirus inhibits melanization of Plutella xylostella hemolymph. Insect Sci. 2021;28(6):1567–1581.
- Eum JH, Bottjen RC, Pruijssers AJ, et al. Characterization and kinetic analysis of protein tyrosine phosphatase-H2 from Microplitis demolitor bracovirus. Insect Biochem Mol Biol. 2010;40(9):690–698.
- Beck MH, Strand MR. A novel polydnavirus protein inhibits the insect prophenoloxidase activation pathway. Proc Natl Acad Sci U S A. 2007;104(49):19267–192672.
- Wang ZZ, Ye XQ, Shi M, et al. Parasitic insect-derived miRnas modulate host development. Nat Commun. 2018; 9(1):2205.
- Wyder S, Tschannen A, Hochuli A, et al. Characterization of Chelonus inanitus polydnavirus segments: sequences and analysis, excision site and demonstration of clustering. J Gen Virol. 2002;83(1):247–256.
- Chen YF, Gao F, Ye XQ, et al. Deep sequencing of Cotesia vestalis bracovirus reveals the complexity of a polydnavirus genome. Virology. 2011;414(1):42–50.
- Espagne E, Dupuy C, Huguet E, et al. Genome sequence of a polydnavirus: insights into symbiotic virus evolution. Science. 2004;306(5694):286–289. DOI:10.1126/science.1103066
- Desjardins CA, Gundersen-Rindal DE, Hostetler JB, et al. Comparative genomics of mutualistic viruses of Glyptapanteles parasitic wasps. Genome Biol. 2008;9(12):1–17.
- Webb BA, Strand MR, Dickey SE, et al. Polydnavirus genomes reflect their dual roles as mutualists and pathogens. Virology. 2006;347(1):160–174. DOI:10.1016/j.virol.2005.11.010.
- Jancek S, Bezier A, Gayral P, et al. Adaptive selection on bracovirus genomes drives the specialization of Cotesia parasitoid wasps. PLoS ONE. 2013;8(5):e64432. DOI:10.1371/journal.pone.0064432.
- Choi JY, Roh JY, Kang JN, et al. Genomic segments cloning and analysis of Cotesia plutellae polydnavirus using plasmid capture system. Biochem Biophys Res Commun. 2005;332(2):487–493.
- Yu DS, Chen YB, Li M, et al. A polydnaviral genome of Microplitis bicoloratus bracovirus and molecular interactions between the host and virus involved in NF-kappa B signaling. Arch Virol. 2016;161(11):3095–3124.
- Djoumad A, Stoltz D, Béliveau C, et al. Ultrastructural and genomic characterization of a second banchine polydnavirus confirms the existence of shared features within this ichnovirus lineage. J Gen Virol. 2013;94(8):1888–1895.
- Lapointe R, Tanaka K, Barney WE, et al. Genomic and morphological features of a banchine polydnavirus: comparison with bracoviruses and ichnoviruses. J Virol. 2007;81(12): 6491–6501.
- Tanaka K, Lapointe R, Barney WE, et al. Shared and species-specific features among ichnovirus genomes. Virology. 2007;363(1):26–35. DOI:10.1016/j.virol.2006.11.034
- Doremus T, Cousserans F, Gyapay G, et al. Extensive transcription analysis of the Hyposoter didymator ichnovirus genome in permissive and non-permissive lepidopteran host species. PLoS ONE. 2014; 9(8):e104072. DOI:10.1371/journal.pone.0104072
- Strand MR, Burke GR Polydnaviruses: nature’s genetic engineers. Annu Rev Virol. 2014;1(1):333–354.
- Gundersen-Rindal D, Dupuy C, Huguet E, et al. Parasitoid polydnaviruses: evolution, pathology and applications. Biocontrol Sci Technol. 2013;23(1):1–61.
- Bitra K, Suderman RJ, Strand MR Polydnavirus ank proteins bind NF-kappa B homodimers and inhibit processing of relish. PLOS Pathog. 2012;8(5):e1002722.
- Kroemer JA, Webb BA Divergences in protein activity and cellular localization within the Campoletis sonorensis ichnovirus vankyrin family. J Virol. 2006;80(24):12219–12228.
- Ignesti M, Ferrara R, Romani P, et al. A polydnavirus-encoded ANK protein has a negative impact on steroidogenesis and development. Insect Biochem Mol Biol. 2018;95:26–32.
- Ramjan Ali M, Kim Y. A novel polydnaviral gene family, BEN, and its immunosuppressive function in larvae of Plutella xylostella parasitized by Cotesia plutellae. J Invertebr Pathol. 2012;110(3):389–397.
- Chen Y, Shi M, Liu P, et al. Characterization of an I kappa B-like gene in Cotesia vestalis polydnavirus. Arch Insect Biochem Physiol 2000;68(2):71–78.
- Wang ZH, Hu RM, Ye XQ, et al. Laccase 1 gene from Plutella xylostella (PxLac1) and its functions in humoral immune response. J Insect Physiol. 2018;107:197–203.
- Langmead B, Salzberg SL. Fast gapped-read alignment with bowtie 2. Nat Methods. 2012;9(4): 357-U54.
- Kim D, Pertea G, Trapnell C, et al. TopHat2: accurate alignment of transcriptomes in the presence of insertions, deletions and gene fusions. Genome Biol. 2013;14(4):R36.
- Trapnell C, Hendrickson DG, Sauvageau M, et al. Differential analysis of gene regulation at transcript resolution with RNA-seq. Nat Biotechnol. 2013;31(1):46–53.
- Livak KJ, Schmittgen TD Analysis of relative gene expression data using real-time quantitative PCR and the 2(-delta delta (CT)) method. Methods. 2001;25(4):402–408.
- Waterhouse AM, Procter JB, Martin DMA, et al. Jalview version 2—a multiple sequence alignment editor and analysis workbench. Bioinformatics. 2009;25(9):1189–1191.
- Philipps B, Rotmann D, Wicki M, et al. Time reduction and process optimization of the baculovirus expression system for more efficient recombinant protein production in insect cells. Protein Expr Purif. 2005;42(1):211–218.
- Bischof J, Maeda RK, Hediger M, et al. An optimized transgenesis system for Drosophila using germ-line-specific phiC31 integrases. Proc Natl Acad Sci U S A. 2007;104(9):3312–3317.
- Qi YX, Jia H, Li MQ, et al. Serotonin modulates insect hemocyte phagocytosis via two different serotonin receptors. Elife. 2016;5:e12241.
- Brand AH, Perrimon N Targeted gene-expression as a means of altering cell fates and generating dominant phenotypes. Develop. 1993;118(2):401–415.
- Sinenko SA, Kim EK, Wynn R, et al. Yantar, a conserved arginine-rich protein is involved in Drosophila hemocyte development. Dev Biol. 2004 Sep 1;273(1):48–62.
- Guzo D, Stoltz DB Observations on cellular-immunity and parasitism in the tussock moth. J Insect Physiol. 1987;33(1):19–31.
- Davies DH, Strand MR, Vinson SB Changes in differential hemocyte count and in vitro behavior of plasmatocytes from host Heliothis virescens caused by Campoletis sonorensis polydnavirus. J Insect Physiol. 1987; 33(3):143–153.
- Bai S, Chen X, Cheng J, et al. Effects of wasp-associated factors of Cotesia plutellae on growth and development of Plutella xylostella larvae. Acta Phytophylacica Sinica. 2005;32(3):235–240.
- Strand MR Teratocytes and their functions in parasitoids. Curr Opin Insect Sci. 2014;6:68–73.
- Murphy N, Banks JC, Whitfield JB, et al. Phylogeny of the parasitic microgastroid subfamilies (Hymenoptera: braconidae) based on sequence data from seven genes, with an improved time estimate of the origin of the lineage. Mol Phylogenet Evol. 2008;47(1):378–395.