ABSTRACT
Vibrio cholerae is a waterborne bacterium that primarily infects the human intestine and causes cholera fatality. Quorum sensing (QS) negatively regulates the expression of V. cholerae virulence gene. However, the primary associated mechanisms remain undetermined. This investigation identified a new QS regulator from the TetR family, LuxT, which increases V. cholerae virulence by directly inhibiting hapR expression. HapR is a master QS regulator that suppresses virulence cascade expression. The expression of luxT increased 4.8-fold in the small intestine of infant mice than in Luria-Bertani broth. ΔluxT mutant strain revealed a substantial defect in the colonizing ability of the small intestines. At low cell densities, the expression level of hapR was upregulated by luxT deletion, suggesting that LuxT can suppress hapR transcription. The electrophoretic mobility shift analysis revealed that LuxT directly binds to the hapR promoter region. Furthermore, luxT expression was upregulated by the two-component system ArcB/ArcA, which responses to changes in oxygen levels in response to the host’s small intestine’s anaerobic signals. In conclusion, this research reveals a novel cell density-mediated virulence regulation pathway and contributes to understanding the complex association between V. cholerae virulence and QS signals. This evidence furnishes new insights for future studies on cholerae’s pathogenic mechanisms.
Introduction
Vibrio cholerae is a gram-negative, facultative anaerobic bacterium that causes cholera. Cholera is responsible for enhanced mortality globally, causing 3–5 million new infections and > 100,000 deaths yearly [Citation1,Citation2]. Upon entrance into the small intestine, V. cholerae releases two critical virulence factors: the ctxAB-encoded cholera toxin (CT) on the lysogenic CTXΦ bacteriophage, directly causing diarrhea [Citation3] and the toxin-co-regulated pilus (TCP), essential for enterocytes attachment and intestinal colonization [Citation4]. CT and TCP are controlled by a tightly regulated signaling cascade [Citation5]. toxT transcription is a prerequisite for virulence factor expression and is controlled by the two membrane-localized complexes, ToxRS and TcpPH. ToxT directly stimulates the transcription of the ctx and tcp genes [Citation6,Citation7]. Several environmental stimuli can affect V. cholerae virulence, such as, pH and temperature regulate the expression of tcpP [Citation8,Citation9], bile salts regulate the expression of toxT [Citation10], and quorum sensing (QS) signals regulate the production of virulence factor [Citation11].
Quorum sensing is a cell density-dependent interaction among bacteria [Citation12], used by V. cholerae to modulate virulence factors release, biofilm generation, type VI release, metabolic modulation, and natural competence [Citation13–17]. QS is modulated via generating, releasing, and identifying signaling molecules called autoinducers (AIs), which control population-wide behavioral alterations. AIs signals control the activity of the membrane receptors CqsSR, LuxPQ, and VpsS. These membrane receptors, in turn, activate luxO transcription. LuxO stimulates small RNA at low cell densities, which represses hapR expression, whereas, at high cell densities, LuxO is suppressed, activating hapR transcription [Citation18,Citation19]. HapR, the master QS regulator, which represses V. cholerae virulence gene, is not expressed at this particular stage of infection. As a consequence, expression of virulence factors, including TCP and CT, are derepressed and timely produced [Citation11]. This investigation demonstrated another new regulator, LuxT, in the QS cascade that substantially regulates V. cholerae virulence.
LuxT is a transcriptional repression regulator of the TetR family that was initially discovered as a luxO start upstream codon region binding protein in V. harveyi [Citation20,Citation21]. Subsequently, Eickhoff et al. reported that LuxT represses qrr1 (a small regulatory RNA) transcription but not luxO transcription [Citation22]. Furthermore, LuxT modulates low cell density behaviors in V. harveyi, including type III release and production of siderophore and aerolysin [Citation23]. In V. vulnificus, LuxO stimulates luxT transcription, and LuxT suppresses the transcription of smcR (HapR homolog) [Citation24]. In V. alginolyticus, LuxT is associated with the QS cascade by positively regulating LuxO and LuxR (HapR homologs) [Citation25]. In V. parahaemolyticus, LuxT homolog SwrT positively modulates swarming motility by inhibiting SwrZ [Citation26]. However, the LuxT regulation in V. cholerae is undefined and poorly understood.
The redox-modulated anoxic binary system responds to oxygen as a signal in the environmental [Citation27]. ArcA, a global transcription factor, regulates hundreds of genes in different bacteria [Citation28,Citation29]. In V. cholerae, ArcA positively modulates toxT expression and enhances the V. cholerae virulence [Citation30]. ArcA regulates vpsT expression, associated with V. cholerae biofilm formation [Citation31]. Furthermore, it critically regulates flrA expression, thereby controlling V. cholerae motility [Citation32]. Further comprehensive analysis of ArcA-regulated genes will help better understand their pathogenic role in V. cholerae.
This investigation identified that LuxT, a previously unrecognized QS system regulator is essential for the virulence of V. cholerae and provided new insights on regulating the ArcA/ArcB two-component system.
Results
ArcA binds to LuxT promoter and positively regulates its expression
ArcA is a global modulator of various biological processes, with a regulon comprising hundreds of target genes in various bacteria [Citation28,Citation29]. However, its function in V. cholerae has been overlooked. Much research has been carried out on its conserved binding sites [Citation33,Citation34]. A putative ArcA-binding site was identified in V. cholerae E12382 (5′-AGTTCATTGT-3′; −111 to −120 from the VCA0917 translational start site) located in the promoter site of VCA0917 (Figure S1). This site was compared with the E. coli and Salmonella conserved binding sites, and a highly similar sequence was identified (Figure S2). To elucidate if VCA0917 was modulated directly by ArcA, ArcA with a 6× His-tagged fusion was isolated, and an electrophoretic mobility shift assay (EMSA) was carried out using the VCA0917 promoter region. Slow-migrating VCA0917 promoter bands were observed with increasing phosphorylated ArcA (ArcA-P) (). However, negative control 4.5s RNA revealed no migrating bands with increasing concentrations of ArcA-P (). Therefore, it was validated that ArcA directly interacts with the VCA0917 promoter.
Figure 1. ArcA positively regulates the expression of luxT by directly interacting with its promoter. (a) EMSA of ArcA-P protein and luxT promoter DNA. The luxT promoter DNA (50 ng) and ArcA-P protein (0 to 1.25 µM) were used in each reaction; 4.5s RNA was used as a negative control. (b) qRT-PCR elucidated luxT expression level in the WT, ΔarcA, and ΔarcA::ParcA strains in LB broth. Data were depicted as mean ± SD (n = 3); unpaired Student’s t-test was applied to assess p-values (*p < 0.05; **p < 0.01; ***p < 0.001; NS, not significant, p > 0.05).
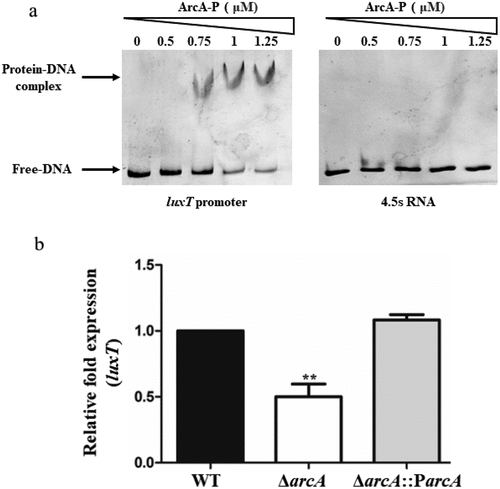
To investigate whether ArcA modulates VCA0917 expression, quantitative real-time PCR (qRT-PCR) was carried out to elucidate VCA0917 expression in the ΔarcA mutant and wild-type (WT) strains. About 2-fold reduction was in VCA0917 expression in the ΔarcA strain than the WT strain, which was reversed after the complementation of ΔarcA with arcA (). These findings indicate that ArcA directly positively regulated the expression of VCA0917.
The BlastP analysis revealed the sequence homology of VCA0917 with TetR family regulator LuxT, comprising the helix-turn-helix (HTH) domain for DNA binding. The luxT gene has also been observed in other Vibrio species [Citation20–26]. V. cholerae LuxT protein exhibited 90%, 92%, 93%, and 93% identity to those of V. alginolyticus (E1U688), V. vulnificus (CMCP6), V. harveyi (Q9ANS7), and V. parahaemolyticus (Q87J33), respectively, indicating that LuxT is conserved in Vibrio spp. We concluded that ArcA binds to luxT promoter and positively regulates its expression.
luxT expression is induced by a low oxygen signal in the small intestine via ArcA
In our study, it was found that ArcA directly positively regulated the expression of luxT. ArcA can sense changes in oxygen availability and regulate the expression of downstream genes [Citation28]. Therefore, we studied whether ArcA regulates luxT expression in response to the low oxygen concentration in the small intestine. We performed the qRT-PCR assays in vitro [Citation35]. The expression level of luxT in the WT strain increased approximately 5.8-fold in a low-oxygen state compared with that under high-oxygen conditions in LB broth (). However, luxT expression in the ΔarcA strain under low- and high-oxygen states was not markedly different (), indicating that low oxygen activates luxT expression via ArcA. In addition, qRT-PCR assay showed that luxT expression in V. cholerae infected infant mouse intestines increased approximately 4.8-fold compared with that in LB broth (), indicating that V. cholerae induces luxT expression in the small intestine. Furthermore, qRT-PCR assays showed that the expression of luxT was significantly decreased in the ΔarcA strain compared to WT in the small intestine (). Complementation of ΔarcA with arcA restored the expression level of luxT to the WT level in the small intestine (). These results indicate that V. cholerae induces luxT expression in the small intestine in response to the low oxygen conditions via ArcA.
Figure 2. luxT expression is induced by a low oxygen signal in the small intestine via ArcA. (a) qRT-PCR expression level of luxT in the WT and ΔarcA mutant strains in LB broth during anaerobic or aerobic conditions. (b) luxT expression in LB broth and in the small intestine. (c) qRT-PCR expression level of luxT in the WT, ΔarcA, and ΔarcA::ParcA strains in the small intestine. Data were depicted as mean ± SD (n = 3); unpaired Student’s t-test was applied to assess p-values (*p < 0.05; **p < 0.01; ***p < 0.001; NS, not significant, p > 0.05).
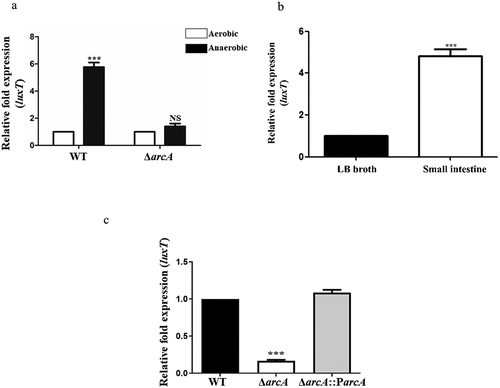
LuxT promotes V. cholerae colonization in infant mouse intestine
Once ingested by the host, V. cholerae colonizes intestinal epithelial cells’ surface and causes chronic diarrhea. A mouse intestinal colonization assay was carried out to investigate LuxT’s impact on the pathogenicity of V. cholerae. WT and ΔluxT mutant strains were administered intragastrically in 3-day-old CD-1 infant mice, and the bacteria recovered from the intestinal homogenates was quantified. It was revealed that mouse intestines infected with the ΔluxT mutant strain contained a relatively lower number of bacteria than that infected with the WT strain, which was reversed after the complementation of ΔluxT with luxT (). In addition, we performed an competition assay in vivo. The competitive assay in vivo showed that the intestinal colonization ability of the ΔluxT strain was significantly reduced compared with that of the WT strain by CI value at 0.23 (). Furthermore, the complementation of ΔluxT with luxT restored the colonization ability back to the WT level (). These findings indicate that LuxT positively influences intestinal colonization in V. cholerae.
Figure 3. LuxT promotes V. cholerae colonization in infant mouse intestine. (a) the capability of the WT, ΔluxT, and ΔluxT::PluxT strains to colonize the intestines of infant mice was assessed via a mouse intestinal colonization assay. Bacterial counts in the mouse intestine (CFU per milliliter) were determined 22–24 h after intragastric inoculation with V. cholerae E12382 strain. (b) competition assay comparing the colonizing ability of WT, ΔluxT, and ΔluxT::PluxT in infant mouse intestine. Each symbol represents the CI in an individual mouse, horizontal bars indicate the median. Unpaired Student’s t-test was applied to assess p-values (*p < 0.05; **p < 0.01; ***p < 0.001; NS, not significant, p > 0.05).
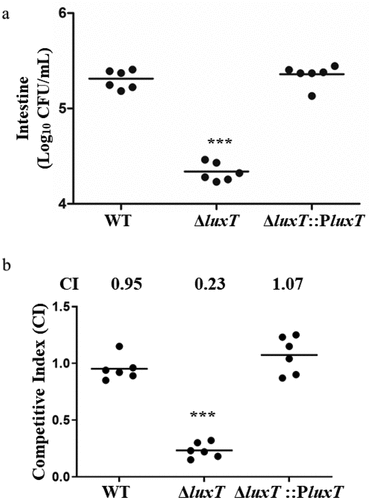
LuxT enhances V. cholerae virulence gene expression by directly repressing hapR expression
To assess the LuxT mechanism that regulates V. cholerae virulence, the expression of virulence gene in the ΔluxT mutant and WT strains were compared via qRT-PCR, revealing that the virulence gene expression was repressed in the ΔluxT mutant strain (). For example, tcpPH and toxT were inhibited in the ΔluxT mutant strain. TCP island genes were suppressed 1.5- to 3.6-fold, and ctxA and ctxB to 2-fold in the ΔluxT mutant strain (). In contrast, the expression of hapR, a master QS regulator, was ~ 5.2-fold higher in the ΔluxT strain than in the WT strain (). The literature reported that HapR inhibits the expression of virulence gene [Citation13,Citation36,Citation37], indicating that LuxT enhances V. cholerae virulence gene expression by repressing hapR expression.
Figure 4. LuxT enhances V. cholerae virulence gene expression by directly repressing hapR expression. (a) qRT-PCR elucidated virulence gene expression levels in the WT, ΔluxT, and ΔluxT::PluxT strains in LB broth. (b) qRT-PCR expression level of hapR in the WT, ΔluxT, and ΔluxT::PluxT strains in LB broth. Data were represented as the mean ± SD (n = 3); unpaired Student’s t-test was applied to assess p-values (*p < 0.05; **p < 0.01; ***p < 0.001; NS, not significant, p > 0.05). (c) EMSA of LuxT protein and hapR promoter DNA. The hapR promoter DNA (50 ng) and LuxT protein (0 to 2 µM) were used in each reaction; 4.5s RNA was used as a negative control. (d) LuxT binds to a motif in the hapR promoter region. Electropherograms indicated the hapR promoter region’s protection pattern after DNase I digestion and incubation with and without LuxT protein (2 μM). The protected region revealed a substantially decreased peak intensities pattern (red) than the control (blue). The bottom of the figure depicts elucidated LuxT-binding motif in a box.
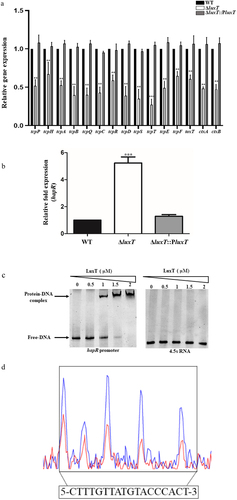
Furthermore, it was also elucidated if LuxT directly modulated hapR expression by EMSA, which indicated that LuxT-maltose-binding protein (MBP) directly bound to the promoter region of hapR, whereas LuxT-MBP protein lacked binding with the negative control 4.5s RNA (). With the help of dye-primer-based DNase I foot-printing assay, a distinct LuxT-bound sequence comprising 18 bp motif (5′-CTTTGTTATGTACCCACT-3′; −98 to −115 from hapR translational start site) was identified (). These findings indicate that LuxT enhances V. cholerae virulence gene expression by directly repressing hapR expression.
LuxT inhibits hapR transcription at a low cell density
The effects of cell density on LuxT-mediated regulation were investigated by monitoring hapR expression in the ΔluxT mutant and WT strains during the growth phase. It was noticed that at low cell densities (optical density at 600 nm [OD600] ≤0.6), hapR level were reduced in the WT strain than in the ΔluxT mutant strain (). However, at high cell densities (OD600 ≥1.5), its level was comparable in both strains (). They suggested that LuxT primarily inhibits hapR level at low cell densities.
Figure 5. LuxT inhibits hapR transcription at low cell densities. qRT-PCR assessed hapR expression level in the WT, ΔluxT, and ΔluxT::PluxT strains at the cell densities indicated. Data were represented as the mean ± SD (n = 3); unpaired Student’s t-test was applied to assess p-values (*p < 0.05; **p < 0.01; ***p < 0.001; NS, not significant, p > 0.05).
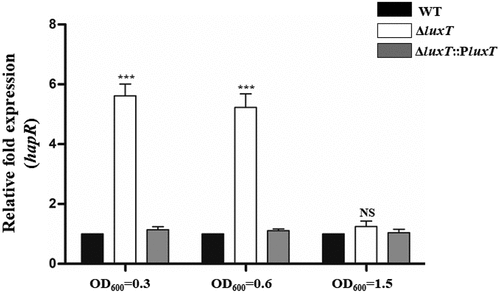
Discussion
Because V. cholerae is the cause of increasing deaths worldwide, it is essential to elucidate its virulence mechanisms and their regulation. This research identified a novel regulatory mechanism mediated by LuxT that is associated with the virulence of V. cholerae in a HapR-dependent manner, and cell density was demonstrated as a host cue for this contribution.
LuxT genetic analysis suggested that it is substantially conserved in Vibrio spp., with 85–93% sequence homology. However the roles of LuxT in regulating QS and the associated phenotypes seem to be elusive in various Vibrio spp. LuxT was first discovered in V. harveyi. LuxT could bind directly to the promoter region of luxO and could inhibit luxO transcription [Citation20,Citation21]. This conclusion was later overturned by Michaela J. Eickhoff et al., who found that LuxT did not inhibit the transcription of luxO in V. harveyi but inhibited the transcription of sRNA qrr1 [Citation22]. In a later study by Michaela J. Eickhoff et al., in V. harveyi, LuxT directly inhibits the transcription of a GntR family transcription factor SwrZ and controls of type III secretion, siderophore, and aerolysin [Citation23]. In V. parahaemolyticus, SwrT (homolog of LuxT) repressed the transcription of swrZ and SwrZ repressed the laf genes encoding the lateral flagellar [Citation26]. In V. vulnificus, LuxT inhibits elastase production by inhibiting smcR transcription, and LuxO directly positively regulates luxT transcription [Citation24]. In V. alginolyticus, LuxT positively regulates extracellular protease production, motility, and virulence. The transcription of luxT was cell density dependent and was positively regulated by LuxU. In addition, LuxT positively regulated both luxO at transcriptional level and luxR at post-transcriptional level, which is thoroughly different from the established QS regulation mode in V. harveyi and V. vulnificus [Citation25]. In our study, we found that LuxT promote V. cholerae virulence by inhibiting the expression of hapR at low cell density (). Consistent with our research, previous studies have shown QS induces virulence factor production by inhibiting the expression of hapR at low cell density [Citation38]. When V. cholerae cells initially entered inside the host small intestine, the population cell number is relatively low and the master QS regulator HapR is repressed. So the virulence factors, including TCP and CT, were produced to enables the pathogens to colonize inside the host small intestine and cause the watery diarrhea.
Figure 6. Diagram depicting the LuxT-mediated virulence regulatory networks in V. cholerae strain E12382. The expression of luxT in V. cholerae was induced in the small intestine in response to the low oxygen conditions via ArcA. Meanwhile, LuxT directly inhibited hapR translation at low cell density. As a consequence, the expression of virulence gene was derepressed and timely produced.

V. cholerae colonizes the surface of intestinal epithelial cells with low oxygen concentrations. Therefore, during infection, it must survive in an anaerobic environment and ArcB/ArcA two-component system is critical for this low-oxygen environment. The research indicates that ArcA positively modulates V. cholerae virulence by increasing toxT expression, and this effect is more pronounced during anaerobic growth [Citation30]. Here, it was revealed that in low-oxygen conditions, V. cholerae promotes luxT expression in the small intestine via ArcA, and LuxT then increases virulence (). It is highly likely that ArcA’s influence on V. cholerae virulence partially depends on regulating luxT expression. When the environment changes, ArcB, membrane sensor protein, is phosphorylated and transfers its phosphoryl group to ArcA. ArcA-P is then stimulated as a transcription factor, upregulating or downregulating many downstream genes [Citation39]. Further studies are required to confirm the redox signal in the small intestine for ArcB activation, which increases luxT expression.
In conclusion, this investigation elucidated the virulence and QS system modulatory activity of novel LuxT in V. cholerae. It was suggested that at low cell densities, LuxT enhances V. cholerae virulence by directly repressing hapR expression. Moreover, V. cholerae induces luxT expression under low-oxygen conditions in the small intestine via ArcB/ArcA ().
Materials and methods
Bacterial strains, plasmids, and growth conditions
The V. cholerae O1 El Tor biotype strain El2382 was isolated in 1994 and was acquired from the Shanghai Municipal Center for Disease Control and Prevention. Table S1 enlists the bacterial plasmids and strains utilized. Table S2 represents the primers applied. Bacteria were propagated in LB broth at 37 °C without shaking (anaerobic state) or with shaking (aerobic state) as per literature [Citation35]. Antibiotics were used as follows: polymyxin B (40 μg/mL), ampicillin (100 μg/mL), kanamycin (50 μg/mL), and chloramphenicol (25 μg/mL). All the chemicals utilized were acquired from Sigma (St. Louis, MO, USA).
Establishing mutant strains and complementation
Isogenic deletion mutant strains (ΔarcA and ΔluxT) were established with the help of suicide vector pRE112, as per literature [Citation40]. For complementation, first, PCR amplification of the gene was carried out; then, the gene was cloned into the vector pBAD33 [Citation41], and lastly, using electroporation, the vector was incorporated into V. cholerae. Table S2 represents all the primers utilized.
RNA isolation and quantitative real-time PCR
V. cholerae cells were propagated overnight in LB media at 37°C and subcultured aerobically or anaerobically at a 1:100 inoculation ratio. When the OD600 was 0.6, the cells were acquired via centrifugation for 5 min at 5000 rpm. Whole RNA was acquired with the help of TRIzol reagent (#15, 596–018; Invitrogen, Waltham, MA, USA) as directed on the kit. cDNA was prepared using the Prime Script RT Reagent Kit (Takara, Shiga, Japan). qRT-PCR was conducted in the Applied Biosystems 7500 sequence detection system with SYBR green fluorescence dye. For normalization, 16S rRNA was utilized [Citation42]. The 2–ΔΔCT method calculated fold change in the assessed gene expression [Citation43].
Electrophoretic mobility shift assay
The LuxT-MBP and 6×His-tagged ArcA were expressed and then purified as per the literature [Citation42]. Then, luxT and hapR promoter region’s DNA fragments (300 bp) were amplified via PCR. The luxT promoter (50 ng) DNA fragments were pre-incubated with an accelerating concentration of purified ArcA protein in a binding buffer (20 µL) (10 mM Tris – HCl [pH 8.0], 1 mM EDTA, 1 mM DTT, 1.5 mg/mL poly IC, 50 mM KCl, 50 μg/mL BSA, and 10% glycerol) augmented with acetyl phosphate (20 nM) for 30 min at ambient temperature (AT) [Citation31]. Similarly, hapR promoter (50 ng) DNA fragments and various concentrations of purified LuxT-MBP protein were incubated at AT for 30 min in a binding buffer (20 µL) (40 mM HEPES-KOH [pH 7.9], 400 mM KCl, 10 mM MgCl2, 2 mM DTT, 10% glycerol, and 1 μg of poly IC)) [Citation24]. 4.5s RNA was set as the negative control [Citation31]. For electrophoresis, the prepared samples were loaded on the 6% polyacrylamide gel in 0.5 × Tris-Borate EDTA (TBE); the gels were stained using Gel Red (1: 10000 dilutions in TBE) and visualized via a UV transilluminator (Tanon).
Dye primer-based DNase I footprinting assay
The 300 bp promoter of hapR was amplified using forward (with a 5’ end 6-FAM modification) and reverse primers. Different quantities of LuxT protein (0–2 μM) were added to 6-FAM-labeled hapR promoter (50 ng) in a binding buffer(10 mM Tris-HCl [pH 7.5], 0.2 mM DTT, 5 mM MgCl2, 10 mM KCl, and 10% glycerol) for 30 min at AT. With the help of 0.05 U DNase I (Thermo; EN0521), the protein-DNA mixtures were partially digested at 25 °C for 5 min and terminated by incubation with EDTA (250 mM) at 65 °C for 10 min. Using the QIAquick PCR Purification Kit (Qiagen; 28104), the DNA fragments were purified, eluted in distilled water (20 μL), and then assessed by MAP Biotech Co., Ltd. (Shanghai, China). The data were measured using Peak Scanner (v1.0).
Intestinal colonization assay
This assay was performed per the literature, with optimizations [Citation40]. CD-1 infant mice (age = 3 days) were acquired from the Beijing Vital River Laboratory Animal Technology (Beijing, China). Overnight V. cholerae cultures were subcultured in fresh LB broth with a 1:100 inoculation ratio. For OD600 of 1.5 V. cholerae (WT or mutant strains) (105 colony-forming units (CFU) in 10 µL PBS) was intragastrically administered to each mouse. After 22–24 h, their intestinal specimens were collected for CFU determination.
Competition assay for mouse intestinal colonization
In this study, the V. cholerae lacZ+ strains (both mutant and WT) and lacZ− strains (ΔlacZ) were grown overnight in LB broth under 37°C. Later, about 105 lacZ+ strains were blended with lacZ− cells at an equivalent amount, and then the resultant mixture was given into mice through intragastric administration. Small intestine was dissected after incubation for 22–24 h, the weight was measured, and the sample was put onto the LB agar plates supplemented with 5-bromo-4-chloro-3-indoyl-β-D galactopyranoside (X-gal) to enumerate those recovered bacteria and obtain the output ratios. The competitive index (CI) was calculated as lacZ+ to lacZ− cell output ratio divided by lacZ+ to lacZ− cell input ratio.
Statistical analyses
Statistical analysis was performed using SPSS (version 12.0; IBM Corp., Armonk, NY, USA). Origin 8.5 (Origin Lab Corporation) was utilized for acquiring figures. The data are depicted as mean ± standard deviation (SD) from three independent experiments. The Student’s t-test assessed the intergroup comparison. Differences were deemed significant at p < 0.05 (*, p < 0.05; **, p < 0.01; ***, p < 0.001; NS, not significant, p > 0.05).
Author contributions
Yuehua Li: conception and design, acquisition of data, analysis and interpretation of data, and drafting the article. Junxiang Yan: conception and design, analysis and interpretation of data, and drafting the article. Jinghao Li: conception and design, analysis and interpretation of data. Xinke Xue: analysis and interpretation of data. Wang Ying: conception and design, analysis and interpretation of data, and reviewing/revising it critically for important intellectual content. Boyang Cao: conception and design, analysis and interpretation of data, and drafting the article.
Supplemental Material
Download MS Word (178.4 KB)Disclosure statement
No potential conflict of interest was reported by the author(s).
Data availability statement
The authors confirm that the data supporting the findings of this study are available in the article and its supplementary materials.
Supplemental data
Supplemental data for this article can be accessed online at https://doi.org/10.1080/21505594.2023.2274640.
Additional information
Funding
References
- Weil AA, Becker RL, Harris JB. Vibrio cholerae at the intersection of immunity and the microbiome. mSphere. 2019;4(6):e00597–10. doi: 10.1128/mSphere.00597-19
- Ali M, Lopez AL, You YA, et al. The global burden of cholera. World Health Organ. 2012;90(3):209–218. doi: 10.2471/BLT.11.093427
- Waldor MK, Mekalanos JJ. Lysogenic conversion by a filamentous phage encoding cholera toxin. Science. 1996;272(5270):1910–1914. doi: 10.1126/science.272.5270.1910
- Yu RR, DiRita VJ. Regulation of gene expression in Vibrio cholerae by ToxT involves both antirepression and RNA polymerase stimulation. Mol Microbiol. 2002;43(1):119–134. doi: 10.1046/j.1365-2958.2002.02721.x
- Childers BM, Klose KE. Regulation of virulence in Vibrio cholerae: The ToxR regulon. Future Microbiol. 2007;2(3):335–344. doi: 10.2217/17460913.2.3.335
- Miller VL, Mekalanos JJ. Synthesis of cholera toxin is positively regulated at the transcriptional level by toxR. Proc Natl Acad Sci, USA. 1984;81(11):3471–3475. doi: 10.1073/pnas.81.11.3471
- DiRita VJ, Parsot C, Jander G, et al. Regulatory cascade controls virulence in Vibrio cholerae. Proc Natl Acad Sci, USA. 1991;88(12):5403–5407. doi: 10.1073/pnas.88.12.5403
- Skorupski K, Taylor RK. A new level in the Vibrio cholerae virulence cascade: AphA is required for transcriptional activation of the tcpPH operon. Mol Micro. 1999;31(3):763–771. doi: 10.1046/j.1365-2958.1999.01215.x
- Kovacikova G, Skorupski K. A Vibrio cholerae LysR homolog, AphB, cooperates with AphA at the tcpPH promoter to activate expression of the ToxR virulence cascade. J Bact. 1999;181(14):4250–4256. doi: 10.1128/JB.181.14.4250-4256.1999
- Hung DT, Mekalanos JJ. Bile acids induce cholera toxin expression in Vibrio cholerae in a ToxT-independent manner. Proc Natl Acad Sci U S A. 2005;102(8):3028–3033. doi: 10.1073/pnas.0409559102
- Zhu J, Miller MB, Vance RE, et al. Quorum-sensing regulators control virulence gene expression in Vibrio cholerae. Proc Natl Acad Sci U S A. 2002;99(5):3129–3134. doi: 10.1073/pnas.052694299
- Wu S, Liu J, Liu C, et al. Quorum sensing for population-level control of bacteria and potential therapeutic applications. Cell Mol Life Sci. 2020;77(7):1319–1343. doi: 10.1007/s00018-019-03326-8
- Suckow G, Seitz P, Blokesch M. Quorum sensing contributes to natural transformation of Vibrio cholerae in a species-specific manner. J Bacteriol. 2011;193(18):4914–4924. doi: 10.1128/JB.05396-11
- Hammer BK, Bassler BL. Quorum sensing controls biofilm formation in Vibrio cholerae. Mol Microbiol. 2003;50(1):101–104. doi: 10.1046/j.1365-2958.2003.03688.x
- Shao Y, Bassler BL. Quorum regulatory small RNAs repress type VI secretion in Vibrio cholerae. Mol Microbiol. 2014;92(5):921–930. doi: 10.1111/mmi.12599
- Hawver LA, Giulietti JM, Baleja JD, et al. Quorum sensing coordinates cooperative expression of pyruvate metabolism genes to maintain a sustainable environment for population stability. MBio. 2016;7(6):e01863–16. doi: 10.1128/mBio.01863-16
- Scrudato M, Blokesch M, Casadesús J. The regulatory network of natural competence and transformation of Vibrio cholerae. PLoS Genet. 2012;8(6):e1002778. doi: 10.1371/journal.pgen.1002778
- Higgins DA, Pomianek ME, Kraml CM, et al. The major Vibrio cholerae autoinducer and its role in virulence factor production. Nature. 2007;450(7171):883–886. doi: 10.1038/nature06284
- Watve S, Barrasso K, Jung SA, et al. Parallel quorum-sensing system in Vibrio cholerae prevents signal interference inside the host. PLOS Pathog. 2020;16(2):e1008313. doi: 10.1371/journal.ppat.1008313
- Lin YH, Miyamoto C, Meighen EA. Cloning and functional studies of a luxO regulator LuxT from Vibrio harveyi. Biochim Biophys Acta. 2000;1494(3):226–235. doi: 10.1016/S0167-4781(00)00236-0
- Lin YH, Miyamoto C, Meighen EA. Purification and characterization of a luxO promoter binding protein LuxT from Vibrio harveyi. Protein Expr Purif. 2000;20(1):87–94. doi: 10.1006/prep.2000.1285
- Eickhoff MJ, Fei C, Huang X, et al. LuxT controls specific quorum-sensing-regulated behaviors in vibrionaceae spp. Via repression of qrr1, encoding a small regulatory RNA. PLoS Genet. 2021;17(4):e1009336. doi: 10.1371/journal.pgen.1009336
- Eickhoff MJ, Fei C, Cong JP, et al. LuxT is a global regulator of low-cell-density behaviors, including type III secretion, siderophore production, and aerolysin production, in Vibrio harveyi. MBio. 2022;13(1):e0362121. doi: 10.1128/mbio.03621-21
- Roh JB, Lee MA, Lee HJ, et al. Transcriptional regulatory cascade for elastase production in Vibrio vulnificus: LuxO activates luxT expression and LuxT represses smcR expression. J Biol Chem. 2006;281(46):34775–34784. doi: 10.1074/jbc.M607844200
- Liu H, Gu D, Cao X, et al. Characterization of a new quorum sensing regulator luxT and its roles in the extracellular protease production, motility, and virulence in fish pathogen Vibrio alginolyticus. Arch Microbiol. 2012;194(6):439–452. doi: 10.1007/s00203-011-0774-x
- Jaques S, McCarter LL. Three new regulators of swarming in Vibrio parahaemolyticus. J Bacteriol. 2006;188(7):2625–2635. doi: 10.1128/JB.188.7.2625-2635.2006
- Gunsalus RP, Park SJ. Aerobic-anaerobic gene regulation in Escherichia coli: control by the ArcAB and Fnr regulons. Res Microbiol. 1994;145(5–6):437–450. doi: 10.1016/0923-2508(94)90092-2
- Salmon KA, Hung SP, Steffen NR, et al. Global gene expression profiling in Escherichia coli K12: effects of oxygen availability and ArcA. J Biol Chem. 2005;280(15):15084–15096. doi: 10.1074/jbc.M414030200
- Wang Z, Sun J, Xia T, et al. Proteomic delineation of the ArcA regulon in Salmonella Typhimurium during anaerobiosis. Mol & Cell Proteomics. 2018;17(10):1937–1947. doi: 10.1074/mcp.RA117.000563
- Sengupta N, Paul K, Chowdhury R. The global regulator ArcA modulates expression of virulence factors in Vibrio cholerae. Infect Immun. 2003;71(10):5583–5589. doi: 10.1128/IAI.71.10.5583-5589.2003
- Xi D, Yang S, Liu Q, et al. The response regulator ArcA enhances biofilm formation in the vpsT manner under the anaerobic condition in Vibrio cholerae. Microb Pathog. 2020;144:104197. doi: 10.1016/j.micpath.2020.104197
- Li Y, Yan J, Guo X, et al. The global regulators ArcA and CytR collaboratively modulate Vibrio cholerae motility. BMC Microbiol. 2022;22(1):22. doi: 10.1186/s12866-022-02435-y
- Lynch AS, Lin EC. Transcriptional control mediated by the ArcA two-component response regulator protein of Escherichia coli: characterization of DNA binding at target promoters. J Bacteriol. 1996;178(21):6238–6249. doi: 10.1128/jb.178.21.6238-6249.1996
- Morales EH, Calderón IL, Collao B, et al. Hypochlorous acid and hydrogen peroxide-induced negative regulation of Salmonella enterica serovar typhimurium ompW by the response regulator ArcA. BMC Microbiol. 2012;12(1):63. doi: 10.1186/1471-2180-12-63
- Fan F, Liu Z, Jabeen N, et al. Enhanced interaction of Vibrio cholerae virulence regulators TcpP and ToxR under oxygen-limiting conditions. Infect Immun. 2014;82(4):1676–1682. doi: 10.1128/IAI.01377-13
- Lin W, Kovacikova G, Skorupski K. Requirements for Vibrio cholerae HapR binding and transcriptional repression at the hapR promoter are distinct from those at the aphA promoter. J Bacteriol. 2005;187(9):3013–3019. doi: 10.1128/JB.187.9.3013-3019.2005
- Liu Z, Miyashiro T, Tsou A, et al. Mucosal penetration primes Vibrio cholerae for host colonization by repressing quorum sensing. Proc Natl Acad Sci U S A. 2008;105(28):9769–9774. doi: 10.1073/pnas.0802241105
- Jung SA, Hawver LA, Ng WL. Parallel quorum sensing signaling pathways in Vibrio cholerae. Curr Genet. 2016;62(2):255–260. doi: 10.1007/s00294-015-0532-8
- Teran-Melo JL, Peña-Sandoval GR, Silva-Jimenez H, et al. Routes of phosphoryl-group transfer during signal transmission and signal decay in the dimeric sensor histidine kinase ArcB. J Biol Chem. 2018;29(34):13214–13223. doi: 10.1074/jbc.RA118.003910
- Xu T, Cao H, Zhu W, et al. RNA-seq-based monitoring of gene expression changes of viable but non-culturable state of Vibrio cholerae induced by cold seawater. Environ Microbiol Rep. 2018;10(5):594–604. doi: 10.1111/1758-2229.12685
- Vettiger A, Basler M. Type VI secretion system substrates are transferred and reused among sister cells. Cell. 2016;167(1):99–110. doi: 10.1016/j.cell.2016.08.023
- Yang S, Xi D, Wang X, et al. Vibrio cholerae VC1741 (PsrA) enhances the colonization of the pathogen in infant mouse intestines in the presence of the long-chain fatty acid, oleic acid. Microb Pathog. 2020;147:104443. doi: 10.1016/j.micpath.2020.104443
- Livak KJ, Schmittgen TD. Analysis of relative gene expression data using real-time quantitative PCR and the 2− DDCt method. Methods. 2001;25(4):402–408. doi: 10.1006/meth.2001.1262