ABSTRACT
Despite their homologous structure and central nervous system(CNS) expression patterns, the GRF1 and GRF2 guanine nucleotide exchange factors(GEF) appear to play distinct, non-overlapping functions in cellular excitability, synaptic plasticity or neuromodulation.
We recently uncovered a new functional role of GRF2 controlling nuclear migration in cone photoreceptors during postnatal neuroepithelial differentiation of the mouse retina. Analyzing GRF2-KO mice, we detected the specific accumulation of abnormally located, “ectopic” cone photoreceptor nuclei in the photoreceptor segment(PS) layer of their retinas. This alteration was accompanied by defective electroretinograms(ERG) indicative of impaired cone-mediated visual function, and accumulation around the “ectopic” nuclei of signaling molecules known to be functionally relevant for intracellular organelle migration, cytoskeletal reorganization or cell polarity establishment including PAR3, PAR6, and the phosphorylated proteins pPAK, pMLC2 and pVASP.
We propose a mechanism whereby the absence of a productive functional interaction between GRF2 and its downstream target CDC42 leads to altered formation/structure of PAR-containing, polarity-related macromolecular complexes and abnormal activation of downstream signaling mediated by activated, phosphorylated forms of PAK, VASP and MLC2. As cone photoreceptors are responsible for color vision and visual acuity, these observations are potentially relevant for degenerative diseases of the human retina, harboring almost double number of cones than mice.
The RASGRF family of mammalian guanine nucleotide exchange factors (GEFs) encompasses 2 highly homologous members (GRF1 and GRF2) that are preferentially expressed in the central nervous system (CNS) and are able to activate different members of the Ras or Rho/Rac families of small GTPasesCitation1 in different cellular contexts. Because of their similar protein structure and overlapping expression patterns, the specific functional roles played by these by 2 GEFs are still imprecise, although a number of non-overlapping functions have been demonstrated for them in CNS processes such as synaptic excitability and plasticity. Previous reports suggested that GRF1 regulates long term depression (LTD) whereas GRF2 modulates long-term potentiation (LTP) in the hippocampus through modulation of the NMDA receptors.Citation2 GRF1 KO mice show also altered retinal electrophysiological responses associated with changes in the expression pattern of several genes known for their association with various retinal pathologies (CRB1, Myo7a, Folh1 or Pttg1),Citation3 suggesting important, relevant functions for this family of proteins in the retina.
In a recent report, we have further evaluated the cellular roles of GRF proteins by means of parallel, functional and phenotypical analyses of the retinas of WT, GRF1-KO, GRF2-KO and GRF1/2-dKO mice sharing the same genetic background. Our data identified defective cone nuclear migration as a novel phenotype in GRF2-depleted mouse retinas and uncovered a critical role of GRF2 in control of the nuclear migration processes required for proper postnatal development and function of retinal cone photoreceptors.Citation4
Proper nuclear positioning is of critical importance for correct development and function of a great variety of cellular types and tissues in eukaryotic organisms. In a process called interkinetic nuclear migration (INM), the cellular precursors of vertebrate neuroepithelia are known to undergo apico-basal movements of their nuclei that are synchronized with the mechanisms regulating their exit of the cell cycle and the determination of their cell fate. Although the exact mechanisms are not yet fully defined, nuclear apical movement during INM appears to be controlled by microtubules and actin, probably in a 2-step process.Citation5
In the development of the vertebrate retina, the mechanisms responsible for the so-called late migratory phase are still poorly understood. This process occurs soon after birth, between postnatal days 11 and 15 (P11-P15), when all retinal progenitor cells have already differentiated, and involves apical movement of the nuclei of cone photoreceptors toward the outer limiting membrane (OLM), reaching their final location in areas proximally underlying this barrier.Citation6
Our observations showed that this nuclear migration process was significantly altered in GRF2-KO and GRF1/2-DKO retinas, whose cone nuclei ended up located much closer to the OLM than in WT or GRF1-KO retinas, in some cases even disrupting the OLM and protruding (“ectopic” nuclei) into the photoreceptor segments (PS) area (). These phenotypic changes were also accompanied by altered electroretinogram recordings (EGR) in response to different light stimuli. Interestingly the ERG defects observed in GRF2-depleted retinas affected specifically the cone-mediated photoreception responses, and were completely different from the alterations previously observed in GRF1-KO retinas, evidencing the different functionality roles that these 2 GEF proteins seem to play in this visual organ.Citation4
Figure 1. Abnormal presence of “ectopic” cone photoreceptor nuclei in the Photoreceptor Segments (PS) area of adult, GRF2-KO mouse retinas. (A) During the late migratory phase of development of wild-type retinas, the nuclei of cone photoreceptors migrate toward the outer limiting membrane (OLM) and are finally located in areas proximal to that physiological barrier (tailed arrows) within the scleral part of the retina (ONL). (B) In GRF2-KO retinas, the nuclei of cone cells end up located at positions much closer to the OLM (tailed arrows) and in some cases even trespass this limiting membrane (“ectopic” nucleus) and protrude into the photoreceptor segments (PS) area (arrowheads). In these pictures, the cone cells were labeled in green with anti-NSE (neuron specific enolase) antibodies, the OLM was labeled in red with β-catenin, and the nuclei were labeled with Hoechst. OS, outer segments; IS, inner segments; ONL, outer nuclear layer; OPL, outer plexiform layer. Scale bar: 25µm.
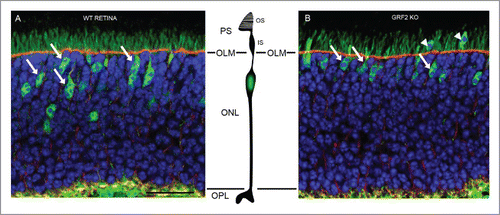
Little is known about the mechanistic details of the cellular processes occurring in cone photoreceptors during the late migratory phase of retinal development. Various reports from different biological systems including different neuronal cell types or muscle myotubes have identified macromolecular complexes containing PAR3, PAR6, CDC42 and atypical protein kinase C (PKCζ) as key regulatory elements triggering the activation of the downstream signaling events that regulate the cytoskeletal reorganization processes required for establishment of apico-basal cell polarity, migration, and associated organelle and nuclear movements during those development programs.Citation7-10
Consistent with these known developmental models, our analysis of GRF2-depleted retinas uncovered a significantly altered cellular distribution, and accumulation, in areas surrounding the ectopic nuclei, of high levels of several signaling molecules including PAR3, PAR6, pPAK, pVASP and pMLC2. The acknowledged roles of PAR3 and PAR6 as regulators of cell polarity,Citation7 of pPAK as direct downstream target of the small GTPase CDC42,Citation11 and of pVASP (an actin binding protein)Citation12 and pMLC2 (the activated form of the myosin light chain)Citation13 as cytoskeletal regulators, strongly suggest that the alterations that those signaling molecules undergo in GRF2-depleted retinas are mechanistically linked to the generation of the new, altered retinal phenotype observed in this particular neuroepithelial tissue in the absence of GRF2.
All these considerations suggest a mechanistic model () whereby GRF2 acts as an essential key regulator of the signaling events modulating cone photoreceptor nuclear migration during the retinal late migratory phase. As CDC42 is a recognized target for physical interaction with and negative regulation by GRF2,Citation13 our observations support the notion that, in GRF2-devoid retinas, the absence of productive, functional interactions between GRF2 and its downstream target CDC42 results in misreregulation (excess activation) of the PAR-containing macromolecular complexes and subsequent over-activation of downstream signaling events mediated by activated pPAK, pVASP and pMLC2, thus leading to faulty cone nuclear migration ().
Figure 2. Proposed mechanistic model for GRF2-dependent regulation of cone photoreceptor nuclear migration in postnatal adult retinas. Schematic representation of the cone nuclear movements (upper areas) and the underlying molecular mechanisms (lower areas) involved in the process of nuclear migration occurring in cone photoreceptors during the late migratory phase of development in wild type retinas (WT, left panel) and GRF2-depleted retinas (GRF2-KO, right panel). The cone nuclear migration process occurs in mice between P11 and P15 and involves the scleral displacement of the nuclei toward the outer limiting membrane (OLM). In WT retinas (left panel) the nuclei always stop their migration once they reach areas proximal to the OLM, whereas in GRF2-KO retinas (right panel) the final location of the nuclei is much closer to the OLM and in some cases they even trespass this physiological barrier and get ultimately located within the photoreceptor segments (PS) area (“ectopic” nuclei, marked in red). Mechanistically, the nuclear migration process involves initial formation of macromolecular complexes involving the small GTPase CDC42, the PAR3 and PAR6 polarity proteins, and atypical protein kinase C (PKCζ). The productive interactions among components within those complexes would then result in triggering of downstream signals responsible for the regulated activation through phosphorylation of various signaling molecules including pPAK (direct downstream target of CDC42) and the cytoskeletal regulators pVASP (actin binding protein) and pMLC2 (activated myosin light chain). In WT retinas, GRF2 (which is known to directly bind to, and downregulate, CDC42 activation) is proposed to act as a key physiological modulator responsible for generating the right balance of downstream signals leading to a correct migration and positioning of the cone nuclei. In contrast, in GRF2-KO retinas, the absence of GRF2 would entail reduced downregulation of CDC42 activity and concomitant over-activation (including increased phosphorylation of VASP and MLC2) of the downstream signals driving nuclear movement, thus resulting in exacerbated migration of the cone nuclei and generation of “ectopic” nuclei.
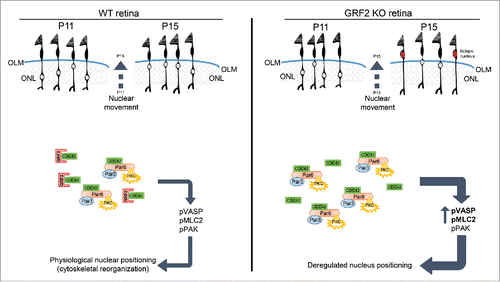
Although this mechanistic model was drawn to account for the regulation of nuclear migration in retinal cone photoreceptors, other mammalian developmental systems involving nuclear migration may be regulated by similar GRF2-dependent mechanisms. This could possibly be the case for processes involved in development of the neuronal layers of the cerebellum, an organ whose development involves important steps of neuronal migration. Interestingly, in the mouse, the migration of cerebellar granule neurons from the external granule layer to the internal granule layer has been shown to be regulated by a CDC42-Par3-Par6 complex and occurs precisely between postnatal day 8 and 15.Citation14,15 As this process occurs in a temporal window exactly coincident with the period of cone nuclear migration occurring during the retinal late migratory phase, it will be interesting in future to analyze cerebella of mice of the same age to search for possible nuclear mislocalizations in that particular cerebellar layer.
Our observations in the retinal cones of GRF2-depleted mice may have translational implications for diseases of human vision as they may provide relevant insights for potential biomarkers or therapy targets useful in the management of some of those pathologies. It should be mentioned that, in contrast to most known retinal pathologies involving defective photoreceptor nuclear movements, where the cellular alterations are usually accompanied by photoreceptor cell death,Citation12,16,17 the cones of murine GRF2-depleted retinas presented normal morphology and their survival was not compromised (at least until our analysis at 5 months of age). In any case, the significant mechanistic proximity between GRF2 and the control of cone nuclear movements still justifies further future studies to determine if the reduced ERG responses recorded in our KO mice are due to alterations in the synaptogenesisCitation6 of the retinal cone cells lacking GRF2. Based on all these considerations, it will not be unreasonable to search for GRF2-related defects of vision in humans, as retinal cones are responsible for color vision and cone loss is the main cause of legal blindness. Interestingly, in human retinas the relative number of cones is almost double (5%) than in mice (2,8%),Citation18,19 and this particular cell type is concentrated in the macula, which is a cone-only area responsible for visual acuity.
For future studies, the experimental challenges posed by the scarcity of retinal cone cells may be overcome with approaches involving the analysis of purified cone preparations isolated by means of flow cytometry cell sorting assays,Citation20,21 or by using genetically modified mouse strains allowing specific, conditional targeting to cone photoreceptors (where expression of CRE recombinase is driven by a cell type-specific opsin promoterCitation22,23) of molecules of interest such as, for example, null mutant forms of GRF2, RAC1 or CDC42. It will also be important to try and determine whether or not a direct mechanistic link exists between the cone nuclear mislocalization and the defective ERG recordings observed in GRF2-depleted retinas, or whether those are 2 independent phenotypes resulting from GRF2 absence. Furthermore, as the GRF proteins are known to modulate synaptic plasticity in the hippocampus through interaction with NR2A or NR2B subunits of NMDA receptors,Citation2 and cone cells use glutamate as neurotransmitter,Citation24 the possible contribution of GRF2 to modulation of NMDA receptors in the retina merits further study.
Altered nuclear positioning is likely to cause additional pathophysiological consequences besides the retinal defects described above. Indeed, reports on various biological systems have documented the relevance of this type of alterations with regards to pathological conditions such as myopathies,Citation25 neurological diseases,Citation26,27 agingCitation19,28 or even cancer.Citation29-31 Thus, the presence of ectopic nuclei in the retina has been shown to increase significantly under various pathological conditions such as age-related macular degeneration, senile cataract, diabetes mellitus, systemic infections or exposure to high light intensities.Citation28,32 Furthermore, the appearance of ectopic nuclei in the PS area has also been associated with aging in different species.Citation19,28,32 Finally, alterations of nuclear positioning or of proteins involved in nuclear movement have also been reported in association with various human adenomas and carcinomas.Citation29-31
Disclosure of potential conflicts of interest
No potential conflicts of interest were disclosed.
Funding
Work supported by grants FIS PI13/02846 and RTICC RD12/0036/0001 from ISCIII (MINECO); grants SA181U13 and BIO/SA03/14 from JCyL, Spain.
References
- Fernández-Medarde A, Santos E. The RasGrf family of mammalian guanine nucleotide exchange factors. Biochim Biophys Acta 2011; 1815:170-88.
- Li S, Tian X, Hartley DM, Feig LA. Distinct roles for Ras-guanine nucleotide-releasing factor 1 (Ras-GRF1) and Ras-GRF2 in the induction of long-term potentiation and long-term depression. J Neurosci 2006; 26:1721-9; PMID:16467520; http://dx.doi.org/10.1523/JNEUROSCI.3990-05.2006
- Fernández-Medarde A, Barhoum R, Riquelme R, Porteros A, Núñez A, de Luis A, de Las Rivas J, de la Villa P, Varela-Nieto I, Santos E. RasGRF1 disruption causes retinal photoreception defects and associated transcriptomic alterations. J Neurochem 2009; 110:641-52; http://dx.doi.org/10.1111/j.1471-4159.2009.06162.x
- Jimeno D, Gomez C, Calzada N, de la Villa P, Lillo C, Santos E. RASGRF2 controls nuclear migration in postnatal retinal cone photoreceptors. J Cell Sci 2016; 129:729-42; PMID:26743081; http://dx.doi.org/10.1242/jcs.180919
- Spear PC, Erickson CA. Interkinetic nuclear migration: A mysterious process in search of a function. Dev Growth Differ 2012; 54:306-16; PMID:22524603; http://dx.doi.org/10.1111/j.1440-169X.2012.01342.x
- Rich KA, Zhan Y, Blanks JC. Migration and synaptogenesis of cone photoreceptors in the developing mouse retina. J Comp Neurol 1997; 388:47-63; PMID:9364238; http://dx.doi.org/10.1002/(SICI)1096-9861(19971110)388:1%3c47::AID-CNE4%3e3.0.CO;2-O
- Etienne-Manneville S. Cdc42 - the centre of polarity. J Cell Sci 2004; 117:1291-300; PMID:15020669; http://dx.doi.org/10.1242/jcs.01115
- Solecki DJ, Model L, Gaetz J, Kapoor TM, Hatten ME. Par6alpha signaling controls glial-guided neuronal migration. Nat Neurosci 2004; 7:1195-203; PMID:15475953; http://dx.doi.org/10.1038/nn1332
- Cadot B, Gache V, Vasyutina E, Falcone S, Birchmeier C, Gomes ER. Nuclear movement during myotube formation is microtubule and dynein dependent and is regulated by Cdc42, Par6 and Par3. EMBO Rep 2012; 13:741-9; PMID:22732842; http://dx.doi.org/10.1038/embor.2012.89
- Dupin I, Etienne-Manneville S. Nuclear positioning: mechanisms and functions. Int J Biochem Cell Biol 2011; 43:1698-707; PMID:21959251; http://dx.doi.org/10.1016/j.biocel.2011.09.004
- Zhang S, Han J, Ann M, Chernoffʈ J, Knaus UG, Ulevitch RJ, Bokoch GM. Rho family GTPases regulate p38 mitogen-activated protein kinase through the downstream mediator pak1. Biochemistry 1995; 10:23934-6
- Trifunović D, Dengler K, Michalakis S, Zrenner E, Wissinger B, Paquet-Durand F. CGMP-dependent cone photoreceptor degeneration in the cpfl1 mouse retina. J Comp Neurol 2010; 518:3604-17; PMID:20593360; http://dx.doi.org/10.1002/cne.22416
- Calvo F, Sanz-Moreno V, Agudo-Ibáñez L, Wallberg F, Sahai E, Marshall CJ, Crespo P. RasGRF suppresses Cdc42-mediated tumour cell movement, cytoskeletal dynamics and transformation. Nat Cell Biol 2011; 13:819-26; PMID:21685891; http://dx.doi.org/10.1038/ncb2271
- Nguyen L, Hippenmeyer S, Reiner O, Sapir T, Nguyen L, Hippenmeyer S. Cellular and Molecular Control of Neuronal Migration. Dordrecht: Springer Netherlands; 2014
- Ramahi JS, Solecki DJ. The PAR polarity complex and cerebellar granule neuron migration. Adv Exp Med Biol 2014; 800:113-31; PMID:24243103; http://dx.doi.org/10.1007/978-94-007-7687-6_7
- Pow D V, Sullivan RKP. Nuclear kinesis, neurite sprouting and abnormal axonal projections of cone photoreceptors in the aged and AMD-afflicted human retina. Exp Eye Res 2007; 84:850-7; PMID:17343852; http://dx.doi.org/10.1016/j.exer.2007.01.005
- Song H, Bush RA, Vijayasarathy C, Fariss RN, Kjellstrom S, Sieving PA. Transgenic Expression of Constitutively Active RAC1 Disrupts Mouse Rod Morphogenesis. Invest Ophthalmol Vis Sci 2014; 55:2659-68; PMID:24651551; http://dx.doi.org/10.1167/iovs.13-13649
- Carter-Dawson L, LaVail MM. Rods and Cones in the Mouse Retina. J Comp Neurol 1979; 188:263-72; PMID:500859; http://dx.doi.org/10.1002/cne.901880205
- Curcio CA, Sloan KR, Kalina RE, Hendrickson AE. Human photoreceptor topography. J Comp Neurol 1990; 292:497-523; PMID:2324310; http://dx.doi.org/10.1002/cne.902920402
- Trimarchi JM, Stadler MB, Roska B, Billings N, Sun B, Bartch B, Cepko CL. Molecular heterogeneity of developing retinal ganglion and amacrine cells revealed through single cell gene expression profiling. J Comp Neurol 2007; 502:1047-65; PMID:17444492; http://dx.doi.org/10.1002/cne.21368
- Feodorova Y, Koch M, Bultman S, Michalakis S, Solovei I. Quick and reliable method for retina dissociation and separation of rod photoreceptor perikarya from adult mice. MethodsX 2015; 2:39-46; PMID:26150970; http://dx.doi.org/10.1016/j.mex.2015.01.002
- Le Y, Zheng L, Zheng W, Ash JD, Agbaga M, Zhu M, Anderson RE. Mouse opsin promoter-directed Cre recombinase expression in transgenic mice. Mol Vis 2006; 12:389-98; PMID:16636658
- Le Y, Ash JD, Al-Ubaidi MR, Chen Y, Ma J, Anderson RE. Targeted expression of Cre recombinase to cone photoreceptors in transgenic mice. Mol Vis 2004; 10:1011-8; PMID:15635292
- Nelson R, Kolb H. Synaptic patterns and response properties of bipolar and ganglion cells in the cat retina. Vision Res 1983; 23:1183-95; PMID:6649437; http://dx.doi.org/10.1016/0042-6989(83)90032-9
- Metzger T, Gache V, Xu M, Cadot B, Folker ES, Richardson BE, Gomes ER, Baylies MK. MAP and kinesin-dependent nuclear positioning is required for skeletal muscle function. Nature 2012; 484:120-4; PMID:22425998; http://dx.doi.org/10.1038/nature10914
- Reiner O, Carrozzo R, Shen Y, Wehnert M, Faustinella F, Dobyns WB, Caskey CT, Ledbetter DH. Isolation of a Miller-Dieker lissencephaly gene containing G protein beta-subunit-like repeats. Nature 1993; 364:717-21; PMID:8355785; http://dx.doi.org/10.1038/364717a0
- Gros-Louis F, Dupré N, Dion P, Fox MA, Laurent S, Verreault S, Sanes JR, Bouchard J-P, Rouleau GA. Mutations in SYNE1 lead to a newly discovered form of autosomal recessive cerebellar ataxia. Nat Genet 2007; 39:80-5; PMID:17159980; http://dx.doi.org/10.1038/ng1927
- Lai Y, Jacoby RO, Jonas AM. Age-related and light-associated retinal changes in Fischer rats. 1978; 634-8.
- Konishi F, Morson BC. Pathology of colorectal adenomas: a colonoscopic survey. J Clin Pathol 1982; 35:830-41; PMID:7107955; http://dx.doi.org/10.1136/jcp.35.8.830
- Marmé A, Zimmermann HP, Moldenhauer G, Schorpp-Kistner M, Müller C, Keberlein O, Giersch A, Kretschmer J, Seib B, Spiess E, et al. Loss of Drop1 expression already at early tumor stages in a wide range of human carcinomas. Int J Cancer 2008; 123:2048-56; http://dx.doi.org/10.1002/ijc.23763
- Sjöblom T, Jones S, Wood LD, Parsons DW, Lin J, Barber TD, Mandelker D, Leary RJ, Ptak J, Silliman N, et al. The consensus coding sequences of human breast and colorectal cancers. Science 2006; 314:268-74; PMID:16959974; http://dx.doi.org/10.1126/science.1133427
- Lai Y-L, Masuda K, Mangum MD, Lug R, Macrae DW, Fletcher G, Liu YP. Subretinal displacement of photoreceptor nuclei in human retina. Exp Eye Res 1982; 34:219-28; PMID:7060649; http://dx.doi.org/10.1016/0014-4835(82)90056-2