ABSTRACT
Metastatization is a complex multistep process requiring fine-tuned regulated cytoskeleton re-modeling, mediated by the cross-talk of actin with interacting partners, such as the Rho GTPases. Our expanding knowledge of invadopodia, small invasive membrane protrusions composed of a core of F-actin, actin regulators and actin-binding proteins, and hotspots for secretion of extracellular matrix (ECM) proteinases, contributes to clarify critical steps of the metastatic program. Growth factor receptors and their intermediate signaling molecules, along with matrix adhesion and rigidity, pH and hypoxia, act as drivers of cytoskeleton changes and invadopodia formation. We recently pro-posed a novel route map by which cancer cells regulates invadopodia dynamics supporting metastasis as response to the endothelin A receptor (ETAR), among the highly druggable G-protein coupled receptors in cancer. The metastatic behavior exhibited by ovarian cancer cells overe-xpressing ETAR is now explained by the interplay with β-arrestin1 (β-arr1), a scaffold protein acting as signal-integrating module of RhoC and cofilin signaling for specific invadopodia formation, accomplished by its interaction with a Rho guanine nucleotide exchange factor (GEF), PDZ-RhoGEF, in a G-protein independent manner. Here, we summarize this novel activation of the RhoC pathway from ETAR/β-arr1 signaling that may be exploited therapeutically and discuss new perspectives for future directions of investigations.
A growing body of evidence in vitro and in vivo, especially by using intravital imaging to visualize cancer cell movement in vivo, has demonstrated that during metastatization invasive cancerous cells possess the ability to extend membrane protrusions, referred as “invadopodia,” tailored for their penetration into the extracellular matrix (ECM), and acquisition of matrix-degrading capability.Citation1-4 To make them, cytoskeletal changes are expected to match with phenotypic alterations in cancer cells, thus augmenting the ability of these cells to migrate through the stroma, and to intravasate.Citation1,4,5 Invadopodia, showing both acto-adhesive and degradative functions, are composed of actin filaments and enriched in actin regulators, such as N-WASP, cortactin, Arp2/3, Tks5 and cofilin, as well as in proteinases, signaling and membrane remodeling proteins.Citation1,6 Although some cancer cells constitutively form invadopodia, emerging evidences highlight that inputs from growth factors and from the tumor microenvironment, such as stromal-cell interactions in an autocrine or paracrine fashion, ECM stiffness or composition, hypoxia and pH, and subsequently cell metabolism, function as drivers of invadopodia.Citation7 Growth factors, like platelet-derived growth factor, transforming growth factor-β and epidermal growth factor, which are produced by cancer cells but also by stromal cells, facilitating an invasive milieu for cancer cells, induce invadopodia formation and activity going through the stages of induction, initiation, assembly, and maturation.Citation7-9 Each of these steps are characterized by a specific signature pattern of protein activities, such as phosphorylation and/or activation, converging on common signaling hub, especially Src kinase, and Rho family GTPase, as well as fine regulation of cofilin activity.Citation8 Studies addressing on key regulators of invadopodia focused much attention around the involvement of Rho-family GTPases, identifying the activation and fine-tuned RhoGTPase activities, in particular that of RhoA, CdC42 and Rac1, as able to produce stable “invasive protrusions.”Citation10,11 More recently, it has been demonstrated that the contribution of different RhoGTPases in invadopodia formation is more complex and involves also the dynamics activity of RhoC in confining the actin polymerization in invadopodia.Citation12-15 A seminal work first revealed that, in metastatic mammary adenocarcinoma cells, RhoC activity is spatially confined to adhesion rings, areas surrounding the actin core of invadopodia, which in turn increases cofilin inactivation, and is crucial for the geometric confinement of active cofilin within the core of the invasive protrusion.Citation10 Accordingly, other studies further demonstrated that critical components of invadopodia, such as podoplanin, might control RhoC activity specifically at adhesion rings, allowing the inactivation of cofilin in this specific compartment of invadopodia.Citation15 In this context, we have recently proposed a picture at the molecular and cellular levels of the new protein complexes mediating actin and membrane dynamics, in which RhoC activity is dynamically regulated to the membrane of invasive ovarian cancer cells to form invadopodia-dependent ECM degradation.Citation16 Our study has revealed that endothelin-1 (ET-1) by activating the ETA receptor (ETAR), among the cancer-related G-protein coupled receptors (GPCR), is responsible for the cell ability to form active invadopodia, tightly controlling the intricate signaling and dynamic organization of cytoskeleton (). ET-1 activates two receptor subtypes known as ETAR and ETB receptor (ETBR), which are differently expressed on human cancers. Indeed, there are cancers that predominantly express ETAR, such as ovarian, breast, colon, renal, gastric, pancreatic thyroid, and nasopharyngeal cancers; cancers expressing ETBR, such as melanoma, glioblastoma and astrocytoma, and those cancers expressing both receptors, such as bladder, lung, vulvar cancers and Kaposi's sarcoma.Citation17 ET-1 initiates downstream signaling cascades, allowing precise control over multiple signaling pathways, to regulate cell motility and metastatic behavior, controlling also multiple and dynamic transitional states between the epithelial and mesenchymal phenotypes, and intravasation.Citation17-21 One of the most significant findings of this study is the knowledge that the molecular scaffold protein β-arrestin1 (β-arr1) regulates the spatial distribution of actin and actin regulators leading to invadopodia formation and activity through RhoC activity.Citation16 Our results revealed a previously unknown role for ETAR/β-arr1 representing a critical route by which the tumor cells form specialized invasive protrusions and acquire maximally pro-invasive capabilities, thus improving our understanding of the molecular specialized functions of this receptor to drive cancer invasion and metastasis (). Since most Rho GTPases are active and stimulate their downstream targets when bound to GTP, a process operated by guanine nucleotide exchange factors (GEFs),Citation22 we exploited putative GEFs implicated in ETAR-driven RhoC activation and identified ZO-1 homology (PDZ)-RhoGEF as primary RhoGEF implicated in the activation of RhoC. Interestingly, previously studies performed to coupling signaling of many GPCR to this class of proteins have been discovered that PDZ-RhoGEF represents one of the regulator for a specific control of the spatial distribution of RhoA activity acting downstream of GPCR through association with proteins Gα12 or Gα13 belonging to the G-protein family, implicated in cell migration.Citation23-27 Interestingly, for the first time we report that the link between GPCR and PDZ-RhoGEF is mediated by β-arr1, further highlighting the crucial importance of a β-arr1-dependent signaling in the tumor-promoting activity of ET-1. β-arrs promote signals through its common feature to form multi-protein signaling complexes, leading to a pleiotropic array of responses than originally thought.Citation28 A number of studies demonstrated that β-arrs can regulate cytoskeleton reorganization and chemotaxis induced by GPCR, and this regulation can occur either by scaffolding signaling proteins regulating GTPAses, such as RhoA or Ral, or by directly binding cytoskeleton proteins as those of cytoskeletal filaments, or cytoskeletal accessories and motor proteins, such as cofilin.Citation29-39 Recent evidences highlight the role of G-protein-dependent induction of invadopodia in cancer cells. Among the major ligands for GPCR, lysophosphatidic acid is implicated in the formation of invadopodia in various cancer cells, such as fibrosarcoma, melanoma, ovarian and prostate cancer cells in a G-protein-dependent manner, and involving Rac, CdC42 or RhoA.Citation40-43 Interestingly, in melanoma cells, ET-1 signaling is capable to mediate a rapid remodeling of invadopodia, acting through ETBR/Gi-dependent activation of Cdc42, accompanied by a rapid decrease in Rac activity.Citation40 Our findings fill an important gap in the understanding of how GPCR can dynamically control invadopodia formation and activity and depict a G-protein-independent molecular map in which we identify β-arr1 as platform in mediating through PDZ-RhoGEF/ RhoC/ROCK/LIMK pathway, restriction of cofilin activity and recruitment of matrix metalloproteases in these actin-rich structures, for efficient matrix degradation and thus activation of invadopodia, enabling ovarian cancer cells to move, invade and metastatize.Citation16 By investigating ETAR-dependent β-arr1 recruitment in driving the metastasis in vivo, our findings also demonstrate that pharmacological blockade of ET-1 receptors by the FDA approved small molecule macitentan, a dual ETAR and ETBR receptor antagonist, impairs β-arr1 ‘interactome’ driven by ET-1 axis in cell motility and metastasis formation. Interestingly, in vivo macitentan showed ability to control ovarian cancer metastasis formation, also through the inhibition of cofilin pathway, thus having important implication for cancer therapy because potential advantage of targeting not only cancer cells (which mainly express ETAR) but also microenvironment associated elements, such as vascular, lymphatic, and inflammatory cells and fibroblasts, which all express ETBR, that critically contribute to cancer metastasis. Although we defined a molecular mechanism for how cancer cells focus protrusions with efficient matrix degradation during invasion, highlighting ET-1/β-arr1 as critical node in the signaling network, many open questions remain to be evaluated. Since the ability of β-arr1 to directly interact with well-established components of invadopodia, such as N-WASP, or Arp2/3 complex, future studies should focus on determining whether β-arr1 might be a structural critical component within invasive protrusions. Moreover, because the adhesion rings surrounding the actin core of invadopodia, are composed of proteins known to be regulated by ET-1 signaling, such as β1 integrin, it is essential to understand whether β-arr1 might affect invadodopia formation and maturation by the specific segregation of β1 integrin into the adhesion ring/actin core.Citation9 Clearly, more advance in this field is likely to improve the knowledge of the molecular events between ET-1/β-arr1 signaling pathways and invadopodia, disclosing so far unexpected involvement of β-arr capable both of promoting actin assembly to form invadopodia and in facilitating gradient sensing and directional cytoskeletal reorganization, as well as by interacting with other GTPases regulating the traffic of specific proteinases through several subcellular compartments and their release at invadopodia.
Figure 1. Endothelin A receptor (ETAR) regulates formation of invadopodia by β-arrestin-1-dependent in cancer cells. Schematic view of an invadopodium that is actively degrading the extracellular matrix via signals induced by ET-1 and its receptor ETAR in ovarian cancer cells. The activation of ET-1 receptor initiates a G-protein-independent signaling through the recruitment of the scaffold protein β-arrestin1, which binds PDZ-RhoGEF favoring its tyrosine-phosphorylation, leading to RhoC activation and downstream RHO-associated coiled-coil containing protein kinase 1 (ROCK1), and LIMK activation, to control regulation of cofilin activity by Ser3 phosphorylation at adhesion ring. Indeed, ET-1/β-arrestin1 enhances the ability of tumor cells to form F-actin cores in membrane protrusions, followed by recruitment of invadopodia component proteins, like cortactin and Tks5, before activation of proteases (MT1-MMP, MMP-2, MMP-9) to complete invadopodia maturation, promoting invasion and metastasis.
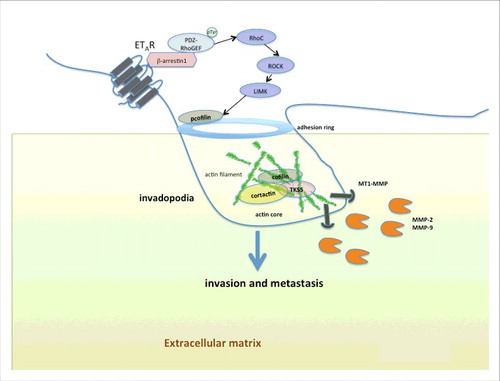
A better knowledge of the role of ET-1R/β-arr1 in the regulation of the invadopodia may suggest the possibility that targeting ET-1R-driven β-arr1 signaling pathways can represent a possible route of therapeutic intervention against cancer.Citation44
Disclosure of potential conflicts of interest
No potential conflicts of interest were disclosed.
Acknowledgments
We would acknowledge all authors of the original report.Citation16
Additional information
Funding
References
- Murphy DA, Courtneidge SA. The ‘ins’ and ‘outs’ of podosomes and invadopodia: characteristics, formation and function. Nat Rev Mol Cell Biol 2011; 12:413-26; PMID:21697900; https://doi.org/10.1038/nrm3141
- Eckert MA, Lwin TM, Chang AT, Kim J, Danis E, Ohno-Machado L, Yang J. Twist1-induced invadopodia formation promotes tumor metastasis. Cancer Cell 2011; 19:372-386; PMID:21397860; https://doi.org/10.1016/j.ccr.2011.01.036
- Lohmer LL, Kelley LC, Hagedorn EJ, Sherwood DR. Invadopodia and basement membrane invasion in vivo. Cell Adhes Migration 2014; 8:246-255; https://doi.org/10.4161/cam.28406
- Condeelis J, Segall JE. Intravital imaging of cell movement in tumours. Nat Rev Cancer 2003; 3:921-30; PMID:14737122; https://doi.org/10.1038/nrc1231
- Gligorijevic B, Wyckoff J, Yamaguchi H, Wang Y, Roussos ET, Condeelis J. N-WASP-mediated invadopodium formation is involved in intravasation and lung metastasis of mammary tumors. J Cell Sci 2012; 125:724-734; PMID:22389406; https://doi.org/10.1242/jcs.092726
- Destaing O, Petropoulos C, Albiges-Rizo C. Coupling between acto-adhesive machinery and ECM degradation in invadosomes. Cell Adh Migr 2014; 8:256-62; PMID:24727371; https://doi.org/10.4161/cam.28558
- Saltel F, Daubon T, Juin A, Ganuza I E, Veillat V, Génot E. Invadosomes: intriguing structures with promise. Eur J Cell Biol 2011; 90:100-107; PMID:20605056; https://doi.org/10.1016/j.ejcb.2010.05.011
- Saykali BA, El-Sibai M. Invadopodia, regulation, and assembly in cancer cell invasion. Cell Commun Adhes 2014; 21:207-12; PMID:24930891; https://doi.org/10.3109/15419061.2014.923845
- Artym VV, Swatkoski S, Matsumoto K, Campbell CB, Petrie RJ, Dimitriadis EK, Li X, Mueller SC, Bugge TH, Gucek M, Yamada KM. Dense fibrillar collagen is a potent inducer of invadopodia via a specific signaling network. J Cell Biol 2015; 208:331-50; PMID:25646088; https://doi.org/10.1083/jcb.201405099
- Spuul P, Ciufici P, Veillat V, Leclercq A, Daubon T, Kramer IJ, Génot E. Importance of RhoGTPases in formation, characteristics, and functions of invadosomes. Small GTPases 2014; 5:e28195; PMID:24967648; https://doi.org/10.4161/sgtp.28713
- Albiges-Rizo C, Destaing O, Fourcade B, Planus E, Block MR. Actin machinery and mechanosensitivity in invadopodia, podosomes and focal adhesions. J Cell Sci 2009; 122:3037-49; PMID:19692590; https://doi.org/10.1242/jcs.052704
- Bravo-Cordero JJ, Oser M, Chen X, Eddy R, Hodgson L, Condeelis J. A novel spatiotemporal RhoC activation pathway locally regulates cofilin activity at invadopodia. Curr Biol 2011; 21:635-44; PMID:21474314; https://doi.org/10.1016/j.cub.2011.03.039
- Pignatelli J, Tumbarello DA, Schmidt RP, Turner CE. Hic-5 promotes invadopodia formation and invasion during TGF-β-induced epithelial-mesenchymal transition. J Cell Biol 2012; 197:421-37; PMID:22529104; https://doi.org/10.1083/jcb.201108143
- Schlienger S, Campbell S, Claing A. ARF1 regulates the Rho/MLC pathway to control EGF-dependent breast cancer cell invasion. Mol Biol Cell 2014; 25:17-29; PMID:24196838; https://doi.org/10.1091/mbc.E13-06-0335
- Martín-Villar E, Borda-d'Agua B, Carrasco-Ramirez P, Renart J, Parsons M, Quintanilla M, Jones GE. Podoplanin mediates ECM degradation by squamous carcinoma cells through control of invadopodia stability. Oncogene 2015; 34:4531-44; https://doi.org/10.1038/onc.2014.388
- Semprucci E, Tocci P, Cianfrocca R, Sestito R, Caprara V, Veglione M, Castro VD, Spadaro F, Ferrandina G, Bagnato A, Rosanò L. Endothelin A receptor drives invadopodia function and cell motility through the β-arrestin/PDZ-RhoGEF pathway in ovarian carcinoma. Oncogene 2016; 35:3432-42; PMID:26522724; https://doi.org/10.1038/onc.2015.403
- Rosanò L, Spinella F, Bagnato A. Endothelin 1 in cancer: biological implications and therapeutic opportunities. Nat Rev Cancer 2013; 13:637-51; https://doi.org/10.1038/nrc3546
- Rosanò L, Bagnato A. Endothelin therapeutics in cancer: Where are we? Am J Physiol Regul Integr Comp Physiol 2016; 310:469-75; https://doi.org/10.1152/ajpregu.00532.2015
- Rosanò L, Cianfrocca R, Tocci P, Spinella F, Di Castro V, Spadaro F, Salvati E, Biroccio AM, Natali PG, Bagnato A. β-arrestin-1 is a nuclear transcriptional regulator of endothelin-1-induced β-catenin signaling. Oncogene 2013; 32:5066-77; https://doi.org/10.1038/onc.2012.527
- Rosanò L, Cianfrocca R, Masi S, Spinella F, Di Castro V, Biroccio A, Salvati E, Nicotra MR, Natali PG, Bagnato A. Beta-arrestin links endothelin A receptor to beta-catenin signalling to induce ovarian cancer cell invasion and metastasis. Proc Natl Acad Sci 2009; 106:2806-11; https://doi.org/10.1073/pnas.0807158106
- Rosanò L, Spinella F, Di Castro V, Nicotra MR, Dedhar S, de Herreros AG, Natali PG, Bagnato A. Endothelin-1 promotes epithelial-to-mesenchymal transition in human ovarian cancer cells. Cancer Res 2005; 65:11649-57; https://doi.org/10.1158/0008-5472.CAN-05-2123
- Hodge RG, Ridley AJ. Regulating Rho GTPases and their regulators. Nat Rev Mol Cell Biol 2016; 17:496–510; https://doi.org/ 10.1038/nrm.2016.67
- Fukuhara S, Murga C, Zohar M, Igishi T, Gutkind JS. A novel PDZ domain containing guanine nucleotide ex-change factor links heterotrimeric G proteins to Rho. J Biol Chem 1999; 274:5868-79; PMID:10026210; http://dx.doi.org/10.1074/jbc.274.9.5868
- Dunn HA, Ferguson SS. PDZ Protein Regulation of G Protein-Coupled Receptor Trafficking and Signaling Pathways. Mol Pharmacol 2015; 88:624-39; PMID:25808930; https://doi.org/10.1124/mol.115.098509
- Patel M, Kawano T, Suzuki N, Hamakubo T, Karginov AV, Kozasa T. Gα13/PDZ-RhoGEF/RhoA signaling is essential for gastrin-releasing peptide receptor-mediated colon cancer cell migration. Mol Pharmacol 2014; 86:252-62; PMID:24958816; https://doi.org/10.1124/mol.114.093914
- Purvanov V, Holst M, Khan J, Baarlink C, Grosse R. G-protein-coupled receptor signaling and polarized actin dynamics drive cell-in-cell invasion. Elife 2014; 3; https://doi.org/10.7554/eLife.02786
- Mikelis CM, Palmby TR, Simaan M, Li W, Szabo R, Lyons R, Martin D, Yagi H, Fukuhara S, Chikumi H, et al. PDZ-RhoGEF and LARG are essential for embryonic development and provide a link between thrombin and LPA receptors and Rho activation. J Biol Chem 2013; 288:12232-43; PMID:23467409; https://doi.org/10.1074/jbc.M112.428599
- Shukla AK, Xiao K, Lefkowitz RJ. Emerging paradigms of β-arrestin-dependent seven transmembrane receptor signaling. Trends Biochem Sci 2011; 36:57-69; https://doi.org/10.1016/j.tibs.2011.06.003
- Min J, Defea K. β-arrestin-dependent actin reorganization: bringing the right players together at the leading edge. Mol Pharmacol 2011; 80:760-8; PMID:21836019; https://doi.org/10.1124/mol.111.072470
- Parisis N, Metodieva G, Metodiev MV. Pseudopodial and β-arrestin-interacting proteomes from migrating breast cancer cells upon PAR2 activation. J Proteomics 2013; 80:91-106; PMID:23313222; https://doi.org/10.1016/j.jprot.2012.12.024
- Law J, Zhang G, Dragan M, Postovit LM, Bhattacharya M. Nodal signals via β-arrestins and RalGTPases to regulate trophoblast invasion. Cell Signal 2014; 26:1935-42; PMID:24863882; https://doi.org/10.1016/j.cellsig.2014.05.009
- Anthony DF, Sin YY, Vadrevu S, Advant N, Day JP, Byrne AM, Lynch MJ, Milligan G, Houslay MD, Baillie GS. β-Arrestin 1 inhibits the GTPase-activating protein function of ARHGAP21, promoting activation of RhoA following angiotensin II type 1A receptor stimulation. Mol Cell Biol 2011; 31:1066-75; PMID:21173159; https://doi.org/10.1128/MCB.00883-10
- Yun SP, Ryu JM, Jang MW, Han HJ. Interaction of profilin-1 and F-actin via a β-arrestin-1/JNK signaling pathway involved in prostaglandin E(2)-induced human mesenchymal stem cells migration and proliferation. J Cell Physiol 2011; 226:559-71; PMID:20717968; https://doi.org/10.1002/jcp.22366
- Xiao K, Sun J, Kim J, Rajagopal S, Zhai B, Villén J, Haas W, Kovacs JJ, Shukla AK, Hara MR, et al. Global phosphorylation analysis of beta-arrestin-mediated signaling downstream of a seven transmembrane receptor (7TMR). Proc Natl Acad Sci U S A 2010; 107:15299-304; PMID:20686112; https://doi.org/10.1073/pnas.1008461107
- Zoudilova M, Min J, Richards HL, Carter D, Huang T, DeFea KA. beta-Arrestins scaffold cofilin with chronophin to direct localized actin filament severing and membrane protrusions downstream of protease-activated receptor-2. J Biol Chem 2010; 285:14318-29; PMID:20207744; https://doi.org/10.1074/jbc.M109.055806
- Li TT, Alemayehu M, Aziziyeh AI, Pape C, Pampillo M, Postovit LM, Mills GB, Babwah AV, Bhattacharya M. Beta-arrestin/Ral signaling regulates lysophosphatidic acid-mediated migration and invasion of human breast tumor cells. Mol Cancer Res 2009; 7:1064-77; PMID:19609003; https://doi.org/10.1158/1541-7786.MCR-08-0578
- Scott MG, Pierotti V, Storez H, Lindberg E, Thuret A, Muntaner O, Labbé-Jullié C, Pitcher JA, Marullo S. Cooperative regulation of extracellular signal-regulated kinase activation and cell shape change by filamin A and beta-arrestins. Mol Cell Biol 2006; 26:3432-45; PMID:16611986; https://doi.org/10.1128/MCB.26.9.3432-3445.2006
- Barnes WG, Reiter E, Violin JD, Ren XR, Milligan G, Lefkowitz RJ. beta-Arrestin 1 and Galphaq/11 coordinately activate RhoA and stress fiber formation following receptor stimulation. J Biol Chem 2005; 280:8041-50; PMID:15611106; https://doi.org/10.1074/jbc.M412924200
- Bhattacharya M, Anborgh PH, Babwah AV, Dale LB, Dobransky T, Benovic JL, Feldman RD, Verdi JM, Rylett RJ, Ferguson SS. Beta-arrestins regulate a Ral-GDS Ral effector pathway that mediates cytoskeletal reorganization. Nat Cell Biol 2002; 4:547-55; PMID:12105416
- Kedziora KM, Leyton-Puig D, Argenzio E, Boumeester AJ, van Butselaar B, Yin T, et al. Rapid Remodeling of Invadosomes by Gi-coupled Receptors: dissecting the role of Rho GTPases. J Biol Chem 2016; 291:4323-33; PMID:26740622; https://doi.org/10.1074/jbc.M115.695940
- Harper K, Arsenault D, Boulay-Jean S, Lauzier A, Lucien F, Dubois CM. Autotaxin promotes cancer invasion via the lysophosphatidic acid receptor 4: participation of the cyclic AMP/EPAC/Rac1 signaling pathway in invadopodia formation. Cancer Res 2010; 70:4634-43; PMID:20484039; https://doi.org/10.1158/0008-5472.CAN-09-3813
- Ward JD, Ha JH, Jayaraman M, Dhanasekaran DN. LPA-mediated migration of ovarian cancer cells involves translocalization of Gαi2 to invadopodia and association with Src and β-pix. Cancer Lett 2015; 356:382-91; PMID:25451317; https://doi.org/10.1016/j.canlet.2014.09.030
- Hwang YS, Lee J, Zhang X, Lindholm PF. Lysophosphatidic acid activates the RhoA and NF-κB through Akt/IκBα signaling and promotes prostate cancer invasion and progression by enhancing functional invadopodia formation. Tumour Biol. 2016; 37:6775-85; PMID:26662305; https://doi.org/10.1007/s13277-015-4549-x
- Rosanò L, Bagnato A. β-arrestin1 at the cross-road of endothelin-1 signaling in cancer. J Exp Clin Cancer Res 2016; 35:121; https://doi.org/10.1186/s13046-016-0401-4