ABSTRACT
Intracellular bacterial pathogens survive and replicate within specialized eukaryotic cell organelles. To establish their intracellular niches these pathogens have adopted sophisticated strategies to control intracellular membrane trafficking. Since Rab-family GTPases are critical regulators of endocytic and secretory membrane trafficking events, many intracellular pathogens have evolved specific mechanisms to modulate or hijack Rab GTPases dynamics and trafficking functions. One such strategy is the delivery of bacterial effectors through specialized machines to specifically target Rab GTPases. Some of these effectors functionally mimic host proteins that regulate the Rab GTP cycle, while others regulate Rabs proteins through their post-translation modifications or proteolysis. In this review, we examine how the localization and function of Rab-family GTPases are altered during infection with 3 well-studied intracellular bacterial pathogens, Mycobacterium tuberculosis, Salmonella enterica and Legionella pneumophila. We also discuss recent findings about specific mechanisms by which these intracellular pathogens target this protein family.
Introduction
Intracellular bacterial pathogens are able to survive and replicate in their host cells by establishing an intracellular niche. Although many of these pathogens are facultative intracellular pathogens, and therefore can replicate both outside and within host cells, it is clear that they gain an advantage from living within specialized membranous compartments in the cytoplasm of the eukaryotic cell. This advantage often derives from increased access to nutrients or avoidance of the immune system. In contrast to non-pathogenic bacteria that are internalized and efficiently killed by phagocytic cells, intracellular pathogens survive and often replicate after internalization into eukaryotic cells. Upon phagocytosis, non-pathogenic bacteria are internalized into a compartment, the early phagosome, which is originated through the invagination of the plasma membrane. This compartment undergoes rapid maturation and, through a series of membrane trafficking events, matures into a late phagosome and finally into a phagolysosome, where the internalized bacteria are destroyed.Citation1,2 As it will be discussed below, bacterial pathogens are able to escape this fate, in most cases by subverting the trafficking mechanisms controlling this maturation pathway.
Rab GTPases are the largest group of the Ras superfamily of small GTPases, with more than 60 members encoded within the human genome. They regulate different intracellular membrane trafficking events, including membrane fission from donor compartments, membrane cargo transport along the cytoskeleton, and membrane tethering and fusion to acceptor compartments.Citation3,4 Many intracellular bacterial pathogens evolved strategies to specifically target these proteins to modulate these different trafficking events. In this review we will focus on 3 bacterial pathogens – Mycobacterium tuberculosis, Salmonella enterica, Legionella pneumophila – that have been shown to disrupt Rab GTPase localization or function by different strategies.
Mycobacterium tuberculosis manipulation of the endocytic pathway
The genus Mycobacterium includes many important intracellular bacterial pathogens. Mycobacterium tuberculosis (M. tuberculosis) is the cause of tuberculosis, a bacterial air-borne infection that affects around 9 millions people worldwide. M. tuberculosis is grouped with other genetically related bacteria that cause similar disease in other animals, forming the Mycobacterium tuberculosis complex. Alveolar macrophages are among the first cell types encountered by these bacteria in the lungs and, therefore, subversion of macrophage function is critical for establishment of an infection. The interaction of M. tuberculosis and the other closely related pathogens, including the attenuated strain Mycobacterium bovis (M. bovis) BCG, with macrophages has been extensively investigated and no significant difference in the ability of the 2 pathogens to interact with trafficking pathways in these cells has been reported. Therefore, we will use the term Mycobacterium in this review to refer without distinction to either M. tuberculosis or M. bovis BCG. It is well established that after phagocytosis Mycobacterium can survive within macrophages,Citation5 and that intracellular survival depends on the pathogen's ability to inhibit phagosome fusion with lysosomal content ().Citation6,7 The Rab GTPases Rab5 and Rab7 sequentially control the formation and subsequent maturation of the phagosomes into phagolysosomes.Citation1 It has been shown that while Rab5 is detected on the Mycobacterium phagosome, Rab7 is not, suggesting that this pathogen blocks the progressions of the phagosome through the canonical phagocytic pathway, by blocking the Rab5 to Rab7 step of maturation (also known as Rab5-Rab7 conversion).Citation8-10 Consistent with this hypothesis, both mature lysosomal hydrolases and the vacuolar ATPase are not detected on the Mycobacterium-containing vacuole.Citation11,12 The specific mechanisms by which Mycobacterium prevents phagosomal maturation are incompletely understood but it is clear that unique lipids of its envelope, such as the mannose-capped lipoarabinomannan (man-LAM), play a central role in this process. It has been shown that in contrast to control latex beads, man-LAM coated beads prevent the recruitment of the Rab5 effector early endosome antigen-1 (EEA1) and delivery of lysosomal enzymes to the phagosome.Citation9,13 This block of phagosome maturation was suggested to be mediated by an inhibition of the phosphatidylinositol 3-phosphate (PI3P) production by the PI3Kinase hVps34.Citation13 More recently, somehow in contrast with previous findings, it has been reported that Mycobacterium does not necessarily inhibit the Rab7 recruitment to the vacuole but, rather, it appears to inhibit its function.Citation14 In fact, it was shown that Rab7 is present in a GDP-bound form in Mycobacterium-infected macrophages and that mycobacterial infection inhibits the recruitment of the Rab effector, Rab-interacting lysosomal protein (RILP). In support of the idea that Rab7 is present but inactive on the mycobacterial phagosome, it has been reported that rate of fluorescence recovery for Rab7 on mycobacterial phagosomes is lower than that on the phagosomes containing latex beads.Citation15 More recently, the secreted mycobacterial nucleoside diphosphate kinase (Ndk; ) was shown to act in vitro as a GTPase activating protein (GAP) for Rab5 and Rab7, suggesting a novel mechanism to prevents recruitment of RILP and EEA1 to the Mycobacterium phagosome.Citation16 However, the absence of Ndk has only a minor effect on the survival of Mycobacterium, suggesting that this bacterium may use additional strategies to prevent phagolysosome maturation.
Figure 1. Trafficking model of the Mycobacterium-containing vacuole. After phagocytosis the Mycobacterium-containing vacuole acquires early-phagocytic features and Rab GTPases (green circles). However, it does not interact with the late endocytic pathway and does not acquire lysosomal markers, such as lysosomal hydrolases, the vATPase and lysosomal glycoproteins.
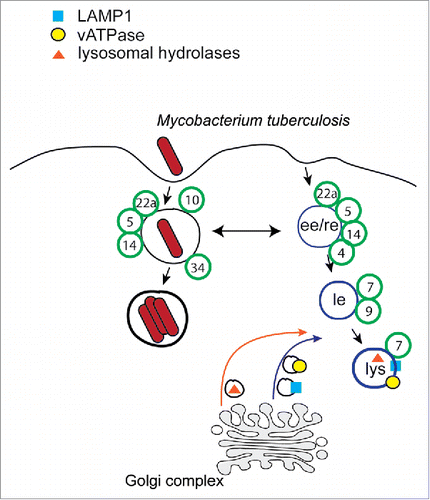
Table 1. Bacterial virulence factors, their biochemical activities, targets and effects on Rab GTPase function.
In addition to Rab7, mycobacteria also interact with other endosomal Rab GTPases. For example, Rab34 is upregulated in Mycobacterium-infected macrophagesCitation17 and Rab34 silencing or overexpression results in increased survival or killing of Mycobacterium.Citation18 Although the mechanisms by which Rab34 may limit Mycobacterium intracellular survival are not known, it is intriguing that RILP is also an effector for this GTPase. Furthermore, siRNA depletion of Rab34 impairs the fusion of phagosomes with late endosomes/lysosomes, while Rab34 overexpression promotes phagosomal maturation.Citation18
Rab10 was also detected on the mycobacterial phagosome at very early time points after infection, even before Rab5 recruitment.Citation19 RNAi-mediated Rab10 knockdown or overexpression of Rab10 dominant-negative mutant delayed maturation of phagosomes of IgG-opsonized latex beads or heat killed-mycobacteria. Moreover, overexpression of a constitutively active mutant of Rab10 partially rescued live-Mycobacterium-containing phagosomes maturation.Citation19 These results suggested that Rab10 acts upstream of Rab5 to modulate the phagosome formation and maturation. It is not clear how Rab10 may modulate Mycobacterium intracellular survival. In response to insulin stimulation, this GTPase controls the translocation to the plasma membrane of the GLUT4 glucose receptor. However, it is not known if there is a link between this activity and the ability of this GTPase to modulate the intracellular replication of Mycobacterium.
Rab14 and Rab22a are also recruited to the mycobacterial phagosome.Citation20,21 Disruption of the function of Rab14 or Rab22a either by depletion or the expression of dominant negative mutants disrupts the maturation of the Mycobacterium-containing phagosome leading to the acquisition of lysosomal markers.Citation20,21 In this sense, the function of these GTPases appears to be opposite to the role of the GTPases discussed above. However, the specific role of these GTPases in the maturation of the Mycobacterium-containing vacuole is not understood.
In conclusion, many Rab GTPases can modulate the ability of mycobacteria to survive in macrophages, indicating a complex regulation of the trafficking events underpinning the phagocytic process and the phagolysosome formation. However, the mechanisms underlying the ability of mycobacteria to hijack these Rab GTPases or the specific roles that the different Rab GTPases play in the establishment of the Mycobacterium intracellular niche remains mostly unknown.
Salmonella enterica interactions with endocytic and secretory Rabs
Salmonella enterica (Salmonella) is an intracellular bacterial pathogen species that comprises more than 2,000 serovars. They cause a variety of illnesses in vertebrate hosts, ranging from self-limited intestinal infections to life-threatening diseases.Citation22 Furthermore, while the majority of Salmonella serovars can infect a broad range of hosts (e.g., Salmonella Typhimurium), others are extremely host-adapated (e.g., Salmonella Typhi).Citation18 Despite their different pathogenic behavior and host range, all the Salmonella serovars share a core set of virulence factors that allow them to enter and replicate within host cells. These properties are strictly dependent on the delivery of a set of bacterial effectors through 2 type III secretion systems (TTSS) encoded within their pathogenicity islands 1 (SPI-1) and 2 (SPI-2).Citation23,24
Several studies have shown a close interaction between Salmonella and Rab-family GTPases. Immediately after Salmonella internalization, the Salmonella-containing vacuole (SCV) interacts with early endosomes and acquires Rab4, Rab5 and the Rab5 effector EEA1 ().Citation25,26 Rab5 recruitment to the SCV and its retention are modulated by the SPI-1 TTSS effector protein SopB, which through its a phosphatidylinositide phosphatase activity modulates the phosphoinositide composition of the SCV.Citation27-31 Specifically, how the phosphoinositide composition of the SCV affects the retention of Rab5 is not understood.
Figure 2. Trafficking model of the Salmonella-containing vacuole. (A) After phagocytosis the Salmonella-containing vacuole (SCV) acquires first early-endocytic features and later most of the lysosomal features. It also acquires sequentially early endocytic Rab GTPases and Rab7 (green circles). However, lysosomal hydrolases are not delivered to the SCV due to a SifA-mediated block of Rab9- and MPR-dependent transport pathway. Broad-host range Salmonella serovars, such as S. Typhimurium, target Rab32 and related Rab GTPases through GtgE and SopD2 and consequently inhibit the delivery of lysosome-related organelle (LRO) enzymes and antimicrobial factors to the LRO and SCV. (B) In contrast to the majority of other Salmonella serovars, the human-adapted S. Typhi does not deliver GtgE and SopD2 and, consequently, succumbs to the Rab32-dependent antimicrobial pathway in mice.
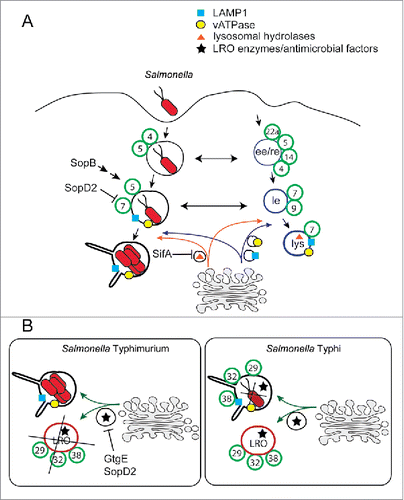
Within 1 hour from bacterial internalization, the SCV recruits Rab7, and with it the Rab7 effector RILP and the lysosomal glycoproteins, such as LAMP-1 and LAMP-2.Citation25,32,33 The SCV also acquires the vacuolar proton pump V-ATPase responsible for the acidification of this compartment.Citation25 Rab7 function is essential for the vacuolar acquisition of the lysosomal glycoprotein LAMP-1 and for Salmonella replication in epithelial cells, since its depletion or the expression of dominant-negative forms of this GTPase result in significant reduction in intracellular bacterial replication.Citation33,34 It has been shown that late endosomal/lysosomal content is transferred to the SCVs, early (30 min) after infection and continues for several hours, in a process that requires Rab7 and results in the acidification of the SCV.Citation35
Therefore, in contrast to mycobacteria that replicates in an intracellular compartment devoid of lysosomal markers, Salmonella replicates in an intracellular niche that acquires many features of a lysosome, including acidic pH. Indeed, acidification of the vacuolar environment is essential for Salmonella survival and replication,Citation36 because it is thought to be a very important cue for the induction of the SPI-2 T3SS, which is critical for Salmonella survival and replication.Citation37,38 Although sharing many features with a lysosome, the SCV is still devoid of many properties characteristic of this compartment. For example, it never acquires the mannose-6-phosphate receptor (MPR) and enzymes that are transported to lysosomes in an MPR-dependent manner, such as cathepsin D.Citation39,40 It has been shown that the SPI-2 T3SS effector SifA interferes with the Rab9-mediated delivery of MPR to the SCV by sequestering this Rab GTPase in a complex with kinesin-interacting protein (SKIP).Citation41
Recent work on the human-restricted pathogen S. Typhi has uncovered the existence of other Rab-dependent pathways leading from a post-Golgi compartment to the SCV. The Rab GTPases Rab32, Rab38 and Rab29, also known as Rab7L1, are recruited to the S. Typhi-containing vacuole, but not to the S. Typhimurium-containing vacuole ().Citation34,42 All these 3 phylogenetically related Rabs localize on the Golgi or post-Golgi compartments.Citation34,43,44 Rab32 and Rab38 are known to be required for the post-Golgi trafficking of melanocytic enzymes to maturing melanosomes in pigment cells and specialized cargo to maturing dense granules in platelets.Citation43,45 Rab29, which is associated with risk of Parkinson disease, is involved in post-Golgi trafficking events that lead to neuronal development,Citation46,47 and is required for the transport of typhoid toxin from the S. Typhi vacuoles to the plasma membrane.Citation34 The absence of Rab32, Rab38 and Rab29 from the S. Typhimurium-containing vacuole is due to the activity of the T3SS effector protein GtgE, which is not present in the genome of S. Typhi and Salmonella Paratyphi, another human-restricted Salmonella. GtgE, which belongs to the clan CA superfamily of cysteine proteases, is a specific protease for these 3 Rab GTPases.Citation42,48,49 Remarkably, expression of GtgE in S. Typhi allowed this human-specific pathogen to overcome host-restriction and replicate in mouse tissues.Citation42 Further dissection of this phenotype indicated that Rab32 is the relevant target for GtgE to overcome this host restriction mechanism since removal of this GTPase or its guanine nucleotide exchange factor BLOC-350 allowed S. Typhi survival in mouse macrophages and replication in mouse tissues.Citation42 These studies therefore identified a novel Rab32-dependent pathogen restriction pathway that prevents the replication of the human-adapted S. Typhi and S. Paratyphi in non-human hosts.
The importance of this novel cell-intrinsic pathogen-restriction pathway is highlighted by the finding that S. Typhimurium targets this pathway in a functionally redundant manner. Indeed, in addition to GtgE, Rab32 is targeted by the T3SS effector protein SopD2.Citation51 This effector is widely distributed across Salmonella serovars but it is a pseudogene in the human-adapted S. Typhi and Salmonella Paratyphi serovars. SopD2 exerts its inhibitory function of Rab32 by acting as a GAP and stimulates Rab32 intrinsic GTPase activity.Citation51 SopD2 GAP activity results in the Rab32 displacement from the surface of the vacuole.Citation51 Although deletion of either GtgE or SopD2 does not eliminate the ability of S. Typhimurium to prevent Rab32 recruitment to the its vacuole, removal of both effectors results in efficient Rab32 recruitment. A S. Typhimurium strain defective for both SopD2 and GtgE is drastically impaired for its ability to cause a systemic infection in mouse.Citation51 However, this mutant strain is as virulent as a wild-type strain in Rab32- or BLOC-3-deficient mice. This indicates that Salmonella evolved redundant strategies to neutralize a critical Rab32-dependent host-defense pathway and establish a systemic infection. In melanocytes and in platelets Rab32 is involved in a pathway that delivers specialized cargo from post-Golgi compartments to maturing lysosomal-related organelles.Citation45,52 The role of Rab32 in post-Golgi trafficking suggests that in macrophages, and possibly in other cell types dedicated to host-defense, Rab32 controls a trafficking pathway delivering specialized molecules that can kill S. Typhi or other intracellular pathogens unable to neutralize this host-defense pathway.Citation42,53,54 Interestingly, Rab32 appears to have quite an opposite role in the intracellular growth of L. pneumophila, an intracellular bacterial pathogen that, as discussed below, hijacks the early secretory pathway to establish a replicative vacuole. Indeed, Rab32 is required for efficient L. pneumophila replication in lung carcinoma epithelial cells.Citation55 These observations highlight the substantial difference of survival strategies implemented by different bacterial pathogens.
It has been suggested that SopD2 may have an additional activity that allows it to interfere with Rab7 function. Indeed, it has been shown that SopD2 blocks endocytic traffic to lysosomes by binding Rab7 and acting as an inhibitor of the Rab7 guanine nucleotide exchange reaction through a poorly understood mechanism.Citation56 It has been reported that this inhibitory activity is dependent on its N-terminal domain, indicating that, whatever its mechanism, this inhibitory function must be independent of its GAP activity, which requires an arginine in the C-terminal end of the protein. Therefore, SopD2 seems to have evolved 2 functions to facilitate Salmonella survival in an intracellular compartment. One to prevent Rab7-mediated lysosomal fusion, and the other to neutralize an antimicrobial Rab32-dependent trafficking pathway.
In addition to Rab32, Rab38 and Rab29, other Rabs GTPases appear to also be excluded from the SCV. For example, Rab8B, Rab13, Rab23, Rab35 are enriched on model phagosomes, but are absent from the S. Typhimurium-containing vacuole.Citation57 Exclusion of these Rabs from the SCV appears to be dependent on the SPI-1 TTSS effector SopB.Citation31 In fact, a S. Typhimurium ΔsopB mutant shows recruitment of these 4 Rabs on its SCV. It has been reported that the phosphoinositide phosphatase SopB prevents the localization of these 4 Rab GTPases by reducing the level of negative charged lipids on the surface of SCV.Citation31 In conclusion, Salmonella has evolved multiple mechanisms to modulate or antagonize Rab GTPase function to create a compartment where this pathogen can survive and replicate.
Legionella pneumophila subversion of secretory Rabs
Legionella pneumophila (L. pneumophila) uses multiple complex strategies to interact with Rab GTPases. The natural hosts of L. pneumophila are fresh water amoebas and it is believed that this bacterium only occasionally causes human infections as the result of inhalation of aerosolized water droplets contaminated with L. pneumophila. It is thought that through extensive co-evolution with its natural host, L. pneumophila has evolved very sophisticated and most often redundant mechanisms to survive and replicate within the intracellular environment of the amoeba. The conservation of many basic cellular biologic processes dictates that many of the strategies evolved by L. pneumophila to thrive in its natural unicellular host also allow it to replicate within human macrophages (reviewed in ref. Citation58). Once internalized by human macrophages, L. pneumophila resides within a specialized compartment known as the Legionella-containing vacuole (LCV). The LCV does not follow the classical phagocytic maturation route and therefore does not fuse with lysosomes (). Rather, through the activity of multiple effector proteins of a type IV protein secretion system (T4SS), Legionella modulates membrane trafficking to build a specific phagosomal compartment (reviewed in refs. Citation59-61). The LCV does not acquire Rab5 or Rab7 indicating that it deviates from the canonical endocytic pathway pretty early after its formation. The mechanism by which L. pneumophila targets the early endocytic machinery are not known but it has been suggested that the effector protein VipD may contribute to this activity by binding Rab5 and Rab22, thus preventing their interaction with their downstream effectors Rabaptin-5 and EEA1.Citation62 Recently, another L. pneumophila T4SS effector, Lgp0393, was reported to target Rab5, as well as Rab21 and Rab22.Citation63 Lgp0393 is remotely related to the Rab5 guanine nucleotide exchange factor Rabex-5 and has a low guanine nucleotide exchange factor activity on Rab5, Rab21 and Rab22. The functional role of the interaction of Lgp0393 with endosomal Rab GTPases and this enzymatic activity still remain to be clarified.
Figure 3. Trafficking model of the Legionella-containing vacuole. After phagocytosis the Legionella-containing vacuole (LCV) does not interact with the endocytic pathway and does not acquire any of endocytic Rab GTPases (green circles). However, it acquires the secretory Rab, Rab1, which is regulated and post-translationally modified by the Legionella T4SS effectors, DrrA, AnkX, SidD, Lem3, LepB and SidE.
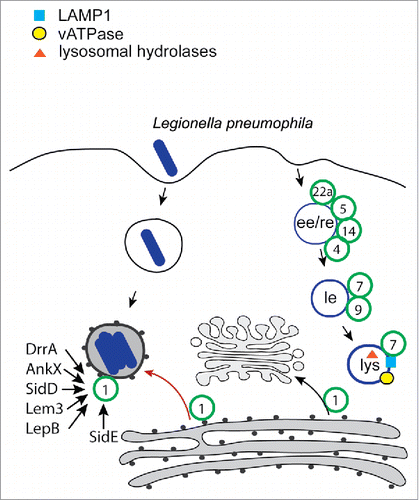
Remarkably, at least 6 of the L. pneumophila T4SS effectors target the Rab GTPases Rab1 (reviewed in ref. 59). Rab1, a critical regulator of trafficking between ER and the Golgi complex, is recruited to the Legionella-containing vacuole within the first hour of infection.Citation64,65 An effector called DrrA or SidM acts as a guanine exchange factor (GEF) for this GTPase, resulting in tethering and fusion of endoplasmic reticulum derived vesicles to the LCV.Citation66,67 DrrA has a PI4P binding domain that mediates its interaction with the membraneCitation68 and a GEF domain with high affinity for the GDP-bound form of Rab1.Citation69-71 Because of its high affinity for the GDP-bound form of Rab1 DrrA function both as a GEF and a Rab-guanine nucleotide displacement inhibitor (RabGDI) displacement factor. In addition, the amino-terminal region of DrrA act as a nucleotidyl transferase that covalently attaches an AMP moiety onto a conserved tyrosine residue of Rab1 using ATP as a substrate, a reaction known as AMPylation.Citation72,73 In addition, to DrrA, L. pneumophila has evolved other effectors that modify Rab1 to stabilize it on the LCV and preventing its inactivation, indicating that controlling Rab1 activity is critical for L. pneumophila intracellular survival and replication. Another L. pneumophila effector, called AnkX, also modifies Rab1 through the addition of a phosphocholine (PC) moiety to a serine residue using CDP-choline as substrate, a reaction called phosphocholination.Citation74,75 Both the tyrosine residue AMPylated by DrrA and the serine residue phosphocholinated by AnkX are located within the switch II loop of Rab1, and, consequently, the modified Rab1 protein has reduced affinity for GAPs and effectors.Citation72,74 Remarkably, the AMPylation and phosphocholination modification on Rab1 are reversed by the concerted action of the type IV effector proteins SidD and Lem3, which respectively deAMPylate and dephosphocholinate Rab1 (reviewed in ref. Citation76). The deAMPylase reaction is performed by the effector protein SidD, an enzyme with structural similarity to metal-dependent protein phosphatases.Citation76-80 The activity of Rab1 is also regulated by the type IV effector protein LepB, a Rab GAP that is found associated to the LCV only later during infection, when the LCV has acquired endoplasmic reticulum features.Citation81 Therefore, Rab1 activity appears to be tightly controlled, likely to ensure the proper spatial and temporal activation of Rab1 in the L. pneumophila-infected cell to facilitate the proper sculpting of the LCV and its removal from the endo-lysosomal pathway. In addition to Rab1, some of the effectors described above can also target other Rab GTPases although the functional consequences of these interactions are unclear.Citation74,82
L. pneumophila also targets Rab GTPases through ubiquitination mediated by a family of L. pneumophila effectors that use a novel mechanism of ubiquitination that does not require E2 or E3 ligases.Citation83 This family of effectors, which includes SidE, SdeA, SdeB, and SdeC, contains an aminoacid motif (R-S-ExE) found in mono-ADP ribosyltransferases.Citation83 This enzymatic domain mediates the ubiquitination of Rab33b, Rab1 and, to a lesser extent, other Rab GTPases associated with the endoplasmic reticulum and the Golgi complex, through a complex and unprecedented biochemical pathway, which involves the formation of a AMP-ubiquitin adduct and atypical direct transfer of ubiquitin.Citation83 Remarkably, Rab GTPases involved in pathogen internalization, such as Rab5, do not appear to be targets of this novel mechanism of ubiquitination. In summary, through mechanisms presumably evolved in the context of interaction with its natural unicellular host, L. pneumophila has adopted multiple mechanisms to modulate Rab GTPase function in macrophages and thus facilitate its intracellular survival and replication.
Conclusions
Intracellular bacterial pathogens have evolved multiple, often redundant mechanisms to target Rab GTPase proteins to modulate or antagonize their multiple and diverse functions. Remarkably, the 3 bacterial pathogens discussed in this review, Mycobacterium tuberculosis, Salmonella enterica, and Legionella pneumophila, display unique trafficking subversion strategies to avoid intracellular killing. The study of these mechanisms has not only generated very important understanding of pathogenic mechanisms but has also provided truly unique insight into Rab GTPase function. Furthermore, some of these studies have revealed novel post-translational modifications of key regulatory proteins thus opening new vistas into eukaryotic regulatory mechanism. Remarkable as these discoveries have been, the fact remains that most of the activities of bacterial effector proteins are unknown, a clear indication that the best is yet to come.
Disclosure of potential conflicts of interest
No potential conflicts of interest were disclosed.
Funding
Work in the Spanò laboratory is supported by the Wellcome Trust (grant no. 109680/Z/15/Z), the Royal Society (grant no. RG150386), and the BBSRC (grant no. BB/N017854/1). Work in the Galán laboratory is supported by the National Institute of Allergy and Infectious Diseases (grants AI055472 and AI079022 to J.E.G.).
References
- Fairn GD, Grinstein S. How nascent phagosomes mature to become phagolysosomes. Trends Immunol 2012; 33:397-405; PMID:22560866; https://doi.org/10.1016/j.it.2012.03.003
- Levin R, Grinstein S, Canton J. The life cycle of phagosomes: Formation, maturation, and resolution. Immunol Rev 2016; 273:156-79; PMID:27558334; https://doi.org/10.1111/imr.12439
- Pfeffer SR. Rab GTPase regulation of membrane identity. Curr Opin Cell Biol 2013; 25:414-9; PMID:23639309; https://doi.org/10.1016/j.ceb.2013.04.002
- Zhen Y, Stenmark H. Cellular functions of Rab GTPases at a glance. J Cell Sci 2015; 128:3171-6; PMID:26272922; https://doi.org/10.1242/jcs.166074
- Suter E. The multiplication of tubercle bacilli within normal phagocytes in tissue culture. J Exp Med 1952; 96:137-50; PMID:14955570; https://doi.org/10.1084/jem.96.2.137
- Armstrong JA, Hart PD. Phagosome-lysosome interactions in cultured macrophages infected with virulent tubercle bacilli. Reversal of the usual nonfusion pattern and observations on bacterial survival. J Exp Med 1975; 142:1-16; PMID:807671; https://doi.org/10.1084/jem.142.1.1
- Armstrong JA, Hart PD. Response of cultured macrophages to Mycobacterium tuberculosis, with observations on fusion of lysosomes with phagosomes. J Exp Med 1971; 134:713-40; PMID:15776571; https://doi.org/10.1084/jem.134.3.713
- Via LE, Deretic D, Ulmer RJ, Hibler NS, Huber LA, Deretic V. Arrest of mycobacterial phagosome maturation is caused by a block in vesicle fusion between stages controlled by rab5 and rab7. J Biol Chem 1997; 272:13326-31; PMID:9148954; https://doi.org/10.1074/jbc.272.20.13326
- Fratti RA, Backer JM, Gruenberg J, Corvera S, Deretic V. Role of phosphatidylinositol 3-kinase and Rab5 effectors in phagosomal biogenesis and mycobacterial phagosome maturation arrest. J Cell Biol 2001; 154:631-44; PMID:11489920; https://doi.org/10.1083/jcb.200106049
- Rink J, Ghigo E, Kalaidzidis Y, Zerial M. Rab conversion as a mechanism of progression from early to late endosomes. Cell 2005; 122:735-49; PMID:16143105; https://doi.org/10.1016/j.cell.2005.06.043
- Sturgill-Koszycki S, Schlesinger PH, Chakraborty P, Haddix PL, Collins HL, Fok AK, Allen RD, Gluck SL, Heuser J, Russell DG. Lack of acidification in Mycobacterium phagosomes produced by exclusion of the vesicular proton-ATPase. Science 1994; 263:678-81; PMID:8303277; https://doi.org/10.1126/science.8303277
- Sturgill-Koszycki S, Schaible UE, Russell DG. Mycobacterium-containing phagosomes are accessible to early endosomes and reflect a transitional state in normal phagosome biogenesis. EMBO J 1996; 15:6960-8; PMID:9003772
- Fratti RA, Chua J, Vergne I, Deretic V. Mycobacterium tuberculosis glycosylated phosphatidylinositol causes phagosome maturation arrest. Proc Natl Acad Sci U S A 2003; 100:5437-42; PMID:12702770; https://doi.org/10.1073/pnas.0737613100
- Sun J, Deghmane AE, Soualhine H, Hong T, Bucci C, Solodkin A, Hmama Z. Mycobacterium bovis BCG disrupts the interaction of Rab7 with RILP contributing to inhibition of phagosome maturation. J Leukoc Biol 2007; 82:1437-45; PMID:18040083; https://doi.org/10.1189/jlb.0507289
- Sugaya K, Seto S, Tsujimura K, Koide Y. Mobility of late endosomal and lysosomal markers on phagosomes analyzed by fluorescence recovery after photobleaching. Biochem Biophys Res Commun 2011; 410:371-5; PMID:21683685; https://doi.org/10.1016/j.bbrc.2011.06.023
- Sun J, Wang X, Lau A, Liao TY, Bucci C, Hmama Z. Mycobacterial nucleoside diphosphate kinase blocks phagosome maturation in murine RAW 264.7 macrophages. PLoS One 2010; 5:e8769; PMID:20098737; https://doi.org/10.1371/journal.pone.0008769
- Gutierrez MG, Mishra BB, Jordao L, Elliott E, Anes E, Griffiths G. NF-kappa B activation controls phagolysosome fusion-mediated killing of mycobacteria by macrophages. J Immunol 2008; 181:2651-63; PMID:18684956; https://doi.org/10.4049/jimmunol.181.4.2651
- Kasmapour B, Gronow A, Bleck CK, Hong W, Gutierrez MG. Size-dependent mechanism of cargo sorting during lysosome-phagosome fusion is controlled by Rab34. Proc Natl Acad Sci U S A 2012; 109:20485-90; PMID:23197834; https://doi.org/10.1073/pnas.1206811109
- Cardoso CM, Jordao L, Vieira OV. Rab10 regulates phagosome maturation and its overexpression rescues Mycobacterium-containing phagosomes maturation. Traffic 2010; 11:221-35; PMID:20028485; https://doi.org/10.1111/j.1600-0854.2009.01013.x
- Kyei GB, Vergne I, Chua J, Roberts E, Harris J, Junutula JR, Deretic V. Rab14 is critical for maintenance of Mycobacterium tuberculosis phagosome maturation arrest. EMBO J 2006; 25:5250-9; PMID:17082769; https://doi.org/10.1038/sj.emboj.7601407
- Roberts EA, Chua J, Kyei GB, Deretic V. Higher order Rab programming in phagolysosome biogenesis. J Cell Biol 2006; 174:923-9; PMID:16982798; https://doi.org/10.1083/jcb.200603026
- Dougan G, Baker S. Salmonella enterica serovar Typhi and the pathogenesis of typhoid fever. Annu Rev Microbiol 2014; 68:317-36; PMID:25208300; https://doi.org/10.1146/annurev-micro-091313-103739
- Figueira R, Holden DW. Functions of the Salmonella pathogenicity island 2 (SPI-2) type III secretion system effectors. Microbiology 2012; 158:1147-61; PMID:22422755; https://doi.org/10.1099/mic.0.058115-0
- Galán JE. Salmonella interactions with host cells: Type III secretion at work. Annu Rev Cell Dev Biol 2001; 17:53-86; PMID:11687484; https://doi.org/10.1146/annurev.cellbio.17.1.53
- Steele-Mortimer O, Meresse S, Gorvel JP, Toh BH, Finlay BB. Biogenesis of Salmonella typhimurium-containing vacuoles in epithelial cells involves interactions with the early endocytic pathway. Cell Microbiol 1999; 1:33-49; PMID:11207539; https://doi.org/10.1046/j.1462-5822.1999.00003.x
- Smith AC, Cirulis JT, Casanova JE, Scidmore MA, Brumell JH. Interaction of the Salmonella-containing vacuole with the endocytic recycling system. J Biol Chem 2005; 280:24634-41; PMID:15886200; https://doi.org/10.1074/jbc.M500358200
- Zhou D, Chen LM, Hernandez L, Shears SB, Galán JE. A Salmonella inositol polyphosphatase acts in conjunction with other bacterial effectors to promote host cell actin cytoskeleton rearrangements and bacterial internalization. Mol Microbiol 2001; 39:248-59; PMID:11136447; https://doi.org/10.1046/j.1365-2958.2001.02230.x
- Hernandez LD, Hueffer K, Wenk MR, Galán JE. Salmonella modulates vesicular traffic by altering phosphoinositide metabolism. Science 2004; 304:1805-7; PMID:15205533; https://doi.org/10.1126/science.1098188
- Patel JC, Hueffer K, Lam TT, Galán JE. Diversification of a Salmonella virulence protein function by ubiquitin-dependent differential localization. Cell 2009; 137:283-94; PMID:19379694; https://doi.org/10.1016/j.cell.2009.01.056
- Mallo GV, Espina M, Smith AC, Terebiznik MR, Aleman A, Finlay BB, Rameh LE, Grinstein S, Brumell JH. SopB promotes phosphatidylinositol 3-phosphate formation on Salmonella vacuoles by recruiting Rab5 and Vps34. J Cell Biol 2008; 182:741-52; PMID:18725540; https://doi.org/10.1083/jcb.200804131
- Bakowski MA, Braun V, Lam GY, Yeung T, Heo WD, Meyer T, Finlay BB, Grinstein S, Brumell JH. The phosphoinositide phosphatase SopB manipulates membrane surface charge and trafficking of the Salmonella-containing vacuole. Cell Host Microbe 2010; 7:453-62; PMID:20542249; https://doi.org/10.1016/j.chom.2010.05.011
- Brumell JH, Scidmore MA. Manipulation of rab GTPase function by intracellular bacterial pathogens. Microbiol Mol Biol Rev 2007; 71:636-52; PMID:18063721; https://doi.org/10.1128/MMBR.00023-07
- Meresse S, Steele-Mortimer O, Finlay BB, Gorvel JP. The rab7 GTPase controls the maturation of Salmonella typhimurium-containing vacuoles in HeLa cells. Embo J 1999; 18:4394-403; PMID:10449405; https://doi.org/10.1093/emboj/18.16.4394
- Spanò S, Liu X, Galán JE. Proteolytic targeting of Rab29 by an effector protein distinguishes the intracellular compartments of human-adapted and broad-host Salmonella. Proc Natl Acad Sci U S A 2011; 108:18418-23; PMID:22042847; https://doi.org/10.1073/pnas.1111959108
- Drecktrah D, Knodler LA, Howe D, Steele-Mortimer O. Salmonella trafficking is defined by continuous dynamic interactions with the endolysosomal system. Traffic 2007; 8:212-25; PMID:17233756; https://doi.org/10.1111/j.1600-0854.2006.00529.x
- Coombes BK, Brown NF, Valdez Y, Brumell JH, Finlay BB. Expression and secretion of Salmonella pathogenicity island-2 virulence genes in response to acidification exhibit differential requirements of a functional type III secretion apparatus and SsaL. J Biol Chem 2004; 279:49804-15; PMID:15383528; https://doi.org/10.1074/jbc.M404299200
- Lee AK, Detweiler CS, Falkow S. OmpR regulates the two-component system SsrA-ssrB in Salmonella pathogenicity island 2. J Bacteriol 2000; 182:771-81; PMID:10633113; https://doi.org/10.1128/JB.182.3.771-781.2000
- Beuzon CR, Banks G, Deiwick J, Hensel M, Holden DW. pH-dependent secretion of SseB, a product of the SPI-2 type III secretion system of Salmonella typhimurium. Mol Microbiol 1999; 33:806-16; PMID:10447889; https://doi.org/10.1046/j.1365-2958.1999.01527.x
- Garvis SG, Beuzon CR, Holden DW. A role for the PhoP/Q regulon in inhibition of fusion between lysosomes and Salmonella-containing vacuoles in macrophages. Cell Microbiol 2001; 3:731-44; PMID:11696033; https://doi.org/10.1046/j.1462-5822.2001.00153.x
- Garcia-del Portillo F, Finlay BB. Targeting of Salmonella typhimurium to vesicles containing lysosomal membrane glycoproteins bypasses compartments with mannose 6-phosphate receptors. J Cell Biol 1995; 129:81-97; PMID:7698996; https://doi.org/10.1083/jcb.129.1.81
- McGourty K, Thurston TL, Matthews SA, Pinaud L, Mota LJ, Holden DW. Salmonella inhibits retrograde trafficking of mannose-6-phosphate receptors and lysosome function. Science 2012; 338:963-7; PMID:23162002; https://doi.org/10.1126/science.1227037
- Spanò S, Galán JE. A Rab32-dependent pathway contributes to Salmonella typhi host restriction. Science 2012; 338:960-3; PMID:23162001; https://doi.org/10.1126/science.1229224
- Wasmeier C, Romao M, Plowright L, Bennett DC, Raposo G, Seabra MC. Rab38 and Rab32 control post-Golgi trafficking of melanogenic enzymes. J Cell Biol 2006; 175:271-81; PMID:17043139; https://doi.org/10.1083/jcb.200606050
- Helip-Wooley A, Thoene JG. Sucrose-induced vacuolation results in increased expression of cholesterol biosynthesis and lysosomal genes. Exp Cell Res 2004; 292:89-100; PMID:14720509; https://doi.org/10.1016/j.yexcr.2003.09.003
- Ambrosio AL, Boyle JA, Di Pietro SM. Mechanism of platelet dense granule biogenesis: Study of cargo transport and function of Rab32 and Rab38 in a model system. Blood 2012; 120:4072-81; PMID:22927249; https://doi.org/10.1182/blood-2012-04-420745
- MacLeod DA, Rhinn H, Kuwahara T, Zolin A, Di Paolo G, McCabe BD, Marder KS, Honig LS, Clark LN, Small SA, et al. RAB7L1 interacts with LRRK2 to modify intraneuronal protein sorting and Parkinson's disease risk. Neuron 2013; 77:425-39; PMID:23395371; https://doi.org/10.1016/j.neuron.2012.11.033
- Kuwahara T, Inoue K, D'Agati VD, Fujimoto T, Eguchi T, Saha S, Wolozin B, Iwatsubo T, Abeliovich A. LRRK2 and RAB7L1 coordinately regulate axonal morphology and lysosome integrity in diverse cellular contexts. Sci Rep 2016; 6:29945; PMID:27424887; https://doi.org/10.1038/srep29945
- Kohler AC, Spanò S, Galán JE, Stebbins CE. Structural and enzymatic characterization of a host-specificity determinant from Salmonella. Acta Crystallogr D Biol Crystallogr 2014; 70:384-91; PMID:24531472; https://doi.org/10.1107/S1399004713028393
- Xu C, Kozlov G, Wong K, Gehring K, Cygler M. Crystal structure of the Salmonella typhimurium effector GtgE. PLoS One 2016; 11:e0166643; PMID:27923041; https://doi.org/10.1371/journal.pone.0166643
- Gerondopoulos A, Langemeyer L, Liang JR, Linford A, Barr FA. BLOC-3 mutated in Hermansky-Pudlak syndrome is a Rab32/38 guanine nucleotide exchange factor. Curr Biol 2012; 22:2135-9; PMID:23084991; https://doi.org/10.1016/j.cub.2012.09.020
- Spanò S, Gao X, Hannemann S, Lara-Tejero M, Galán JE. A bacterial pathogen targets a host Rab-family GTPase defense pathway with a GAP. Cell Host Microbe 2016; 19:216-26; PMID:26867180; https://doi.org/10.1016/j.chom.2016.01.004
- Bultema JJ, Ambrosio AL, Burek CL, Di Pietro SM. BLOC-2, AP-3, and AP-1 proteins function in concert with Rab38 and Rab32 proteins to mediate protein trafficking to lysosome-related organelles. J Biol Chem 2012; 287:19550-63; PMID:22511774; https://doi.org/10.1074/jbc.M112.351908
- Solano-Collado V, Rofe A, Spanò S. Rab32 restriction of intracellular bacterial pathogens. Small GTPases 2016:1-8; PMID:27645564; https://doi.org/10.1080/21541248.2016.1219207
- Spanò S. Host restriction in Salmonella: Insights from Rab GTPases. Cell Microbiol 2014; 16:1321-8; PMID:24957519; https://doi.org/10.1111/cmi.12327
- Hoffmann C, Finsel I, Otto A, Pfaffinger G, Rothmeier E, Hecker M, Becher D, Hilbi H. Functional analysis of novel Rab GTPases identified in the proteome of purified Legionella-containing vacuoles from macrophages. Cell Microbiol 2014; 16:1034-52; PMID:24373249; https://doi.org/10.1111/cmi.12235
- D'Costa VM, Braun V, Landekic M, Shi R, Proteau A, McDonald L, Cygler M, Grinstein S, Brumell JH. Salmonella disrupts host endocytic trafficking by SopD2-mediated inhibition of Rab7. Cell Rep 2015; 12:1508-18; PMID:26299973; https://doi.org/10.1016/j.celrep.2015.07.063
- Smith AC, Heo WD, Braun V, Jiang X, Macrae C, Casanova JE, Scidmore MA, Grinstein S, Meyer T, Brumell JH. A network of Rab GTPases controls phagosome maturation and is modulated by Salmonella enterica serovar typhimurium. J Cell Biol 2007; 176:263-8; PMID:17261845; https://doi.org/10.1083/jcb.200611056
- Escoll P, Rolando M, Gomez-Valero L, Buchrieser C. From amoeba to macrophages: Exploring the molecular mechanisms of Legionella pneumophila infection in both hosts. Curr Top Microbiol Immunol 2013; 376:1-34; PMID:23949285; https://doi.org/10.1007/82_2013_351
- Sherwood RK, Roy CR. A Rab-centric perspective of bacterial pathogen-occupied vacuoles. Cell Host Microbe 2013; 14:256-68; PMID:24034612; https://doi.org/10.1016/j.chom.2013.08.010
- Isaac DT, Isberg R. Master manipulators: An update on Legionella pneumophila Icm/Dot translocated substrates and their host targets. Future Microbiol 2014; 9:343-59; PMID:24762308; https://doi.org/10.2217/fmb.13.162
- Goody RS, Itzen A. Modulation of small GTPases by Legionella. Curr Top Microbiol Immunol 2013; 376:117-33; PMID:23918171; https://doi.org/10.1007/82_2013_340
- Ku B, Lee KH, Park WS, Yang CS, Ge J, Lee SG, Cha SS, Shao F, Heo WD, Jung JU, et al. VipD of Legionella pneumophila targets activated Rab5 and Rab22 to interfere with endosomal trafficking in macrophages. PLoS Pathog 2012; 8:e1003082; PMID:23271971; https://doi.org/10.1371/journal.ppat.1003082
- Sohn YS, Shin HC, Park WS, Ge J, Kim CH, Lee BL, Heo WD, Jung JU, Rigden DJ, Oh BH. Lpg0393 of Legionella pneumophila is a guanine-nucleotide exchange factor for Rab5, Rab21 and Rab22. PLoS One 2015; 10:e0118683; PMID:25821953; https://doi.org/10.1371/journal.pone.0118683
- Derre I, Isberg RR. Legionella pneumophila replication vacuole formation involves rapid recruitment of proteins of the early secretory system. Infect Immun 2004; 72:3048-53; PMID:15102819; https://doi.org/10.1128/IAI.72.5.3048-3053.2004
- Kagan JC, Stein MP, Pypaert M, Roy CR. Legionella subvert the functions of Rab1 and Sec22b to create a replicative organelle. J Exp Med 2004; 199:1201-11; PMID:15117975; https://doi.org/10.1084/jem.20031706
- Machner MP, Isberg RR. Targeting of host Rab GTPase function by the intravacuolar pathogen Legionella pneumophila. Dev Cell 2006; 11:47-56; PMID:16824952; https://doi.org/10.1016/j.devcel.2006.05.013
- Murata T, Delprato A, Ingmundson A, Toomre DK, Lambright DG, Roy CR. The Legionella pneumophila effector protein DrrA is a Rab1 guanine nucleotide-exchange factor. Nat Cell Biol 2006; 8:971-7; PMID:16906144; https://doi.org/10.1038/ncb1463
- Brombacher E, Urwyler S, Ragaz C, Weber SS, Kami K, Overduin M, Hilbi H. Rab1 guanine nucleotide exchange factor SidM is a major phosphatidylinositol 4-phosphate-binding effector protein of Legionella pneumophila. J Biol Chem 2009; 284:4846-56; PMID:19095644; https://doi.org/10.1074/jbc.M807505200
- Schoebel S, Oesterlin LK, Blankenfeldt W, Goody RS, Itzen A. RabGDI displacement by DrrA from Legionella is a consequence of its guanine nucleotide exchange activity. Mol Cell 2009; 36:1060-72; PMID:20064470; https://doi.org/10.1016/j.molcel.2009.11.014
- Suh HY, Lee DW, Lee KH, Ku B, Choi SJ, Woo JS, Kim YG, Oh BH. Structural insights into the dual nucleotide exchange and GDI displacement activity of SidM/DrrA. EMBO J 2010; 29:496-504; PMID:19942850; https://doi.org/10.1038/emboj.2009.347
- Zhu Y, Hu L, Zhou Y, Yao Q, Liu L, Shao F. Structural mechanism of host Rab1 activation by the bifunctional Legionella type IV effector SidM/DrrA. Proc Natl Acad Sci U S A 2010; 107:4699-704; PMID:20176951; https://doi.org/10.1073/pnas.0914231107
- Muller MP, Peters H, Blumer J, Blankenfeldt W, Goody RS, Itzen A. The Legionella effector protein DrrA AMPylates the membrane traffic regulator Rab1b. Science 2010; 329:946-9; PMID:20651120; https://doi.org/10.1126/science.1192276
- Yarbrough ML, Li Y, Kinch LN, Grishin NV, Ball HL, Orth K. AMPylation of Rho GTPases by Vibrio VopS disrupts effector binding and downstream signaling. Science 2009; 323:269-72; PMID:19039103; https://doi.org/10.1126/science.1166382
- Mukherjee S, Liu X, Arasaki K, McDonough J, Galán JE, Roy CR. Modulation of Rab GTPase function by a protein phosphocholine transferase. Nature 2011; 477:103-6; PMID:21822290; https://doi.org/10.1038/nature10335
- Campanacci V, Mukherjee S, Roy CR, Cherfils J. Structure of the Legionella effector AnkX reveals the mechanism of phosphocholine transfer by the FIC domain. EMBO J 2013; 32:1469-77; PMID:23572077; https://doi.org/10.1038/emboj.2013.82
- Salomon D, Orth K. What pathogens have taught us about posttranslational modifications. Cell Host Microbe 2013; 14:269-79; PMID:24034613; https://doi.org/10.1016/j.chom.2013.07.008
- Neunuebel MR, Chen Y, Gaspar AH, Backlund PS, Jr, Yergey A, Machner MP. De-AMPylation of the small GTPase Rab1 by the pathogen Legionella pneumophila. Science 2011; 333:453-6; PMID:21680813; https://doi.org/10.1126/science.1207193
- Tan Y, Luo ZQ. Legionella pneumophila SidD is a deAMPylase that modifies Rab1. Nature 2011; 475:506-9; PMID:21734656; https://doi.org/10.1038/nature10307
- Chen Y, Tascon I, Neunuebel MR, Pallara C, Brady J, Kinch LN, Fernandez-Recio J, Rojas AL, Machner MP, Hierro A. Structural basis for Rab1 de-AMPylation by the Legionella pneumophila effector SidD. PLoS Pathog 2013; 9:e1003382; PMID:23696742; https://doi.org/10.1371/journal.ppat.1003382
- Tan Y, Arnold RJ, Luo ZQ. Legionella pneumophila regulates the small GTPase Rab1 activity by reversible phosphorylcholination. Proc Natl Acad Sci U S A 2011; 108:21212-7; PMID:22158903; https://doi.org/10.1073/pnas.1114023109
- Ingmundson A, Delprato A, Lambright DG, Roy CR. Legionella pneumophila proteins that regulate Rab1 membrane cycling. Nature 2007; 450:365-9; PMID:17952054; https://doi.org/10.1038/nature06336
- So EC, Schroeder GN, Carson D, Mattheis C, Mousnier A, Broncel M, Tate EW, Frankel G. The Rab-binding profiles of bacterial virulence factors during infection. J Biol Chem 2016; 291:5832-43; PMID:26755725; https://doi.org/10.1074/jbc.M115.700930
- Qiu J, Sheedlo MJ, Yu K, Tan Y, Nakayasu ES, Das C, Liu X, Luo ZQ. Ubiquitination independent of E1 and E2 enzymes by bacterial effectors. Nature 2016; 533:120-4; PMID:27049943; https://doi.org/10.1038/nature17657
- Ohlson MB, Huang Z, Alto NM, Blanc MP, Dixon JE, Chai J, Miller SI. Structure and function of Salmonella SifA indicate that its interactions with SKIP, SseJ, and RhoA family GTPases induce endosomal tubulation. Cell Host Microbe 2008; 4:434-46; PMID:18996344; https://doi.org/10.1016/j.chom.2008.08.012