Abstract
Exosomes in plasma of glioma patients hold promise as biomarkers of prognosis. We aimed to determine whether changes in total exosomal protein and mRNA expression levels could serve as surrogate markers of immunological and clinical responses in glioma patients receiving antitumor vaccines. Exosomes were isolated from pre/post-vaccine plasma specimens in 20/22 patients enrolled in a phase I/II trial with the antitumor vaccine. Exosomal protein content was analyzed and mRNA expression levels for 24 genes were simultaneously assessed by qRT-PCR. Pre- to post-vaccination changes in exosomal protein and ΔCt values were correlated with immunological and clinical responses and survival using Spearman rank statistics and hazard ratios (HR). Exosomal protein levels positively correlated (p < 0.0043) with the WHO tumor grade at diagnosis. Protein levels were lower in post- vs. pre-vaccination exosome fractions. Post-therapy increases in tumor size were associated with elevations in exosome proteins in glioblastoma but not always in anaplastic astrocytoma (AA). Only exosomal ΔCt values for IL-8, TIMP-1, TGF-β and ZAP70 were significant (p < 0.04 to p < 0.001). The ΔCt for IL-8 and TGF-β mRNA positively correlated with post-vaccine immunologic responses to glioma antigens, while ΔCt for TIMP-1 mRNA was negatively correlated to ΔCt for IL-8 and TGF-β. Only ΔCt for IL-8 weakly correlated with OS and time to progression (TTP). In post-vaccine exosomes of the longest surviving patient with AA, mRNA for PD-1 was persistently elevated. Protein and mRNA expression levels for immune-related genes in plasma exosomes were useful in evaluating glioma patients' response to vaccination therapy.
Abbreviations:
- AA, anaplastic astrocytoma
- AO, anaplastic oligodendroglioma
- ATP, adenosine triphosphates
- EV, extracellular vesicles
- GAA, glioma associated antigens
- GBM, glioblastoma multiforme
- MRI, magnetic resonance imaging
- NC, normal controls
- OS, overall survival
- PD-1, programmed death-1
- PD-L1, programmed death ligand 1
- TEM, transmission electron microscopy
- TEX, tumor-derived exosomes
- TTP, time to progression
Introduction
Intercellular communication is a critically important function in multicellular organisms, and it can be mediated through direct cell-to-cell contact or transfer of secreted molecules. Recently, an additional mechanism of intercellular communication has been discovered, which involves transfer of extracellular vesicles (EVs) carrying membrane-tethered molecules between different cells.Citation1 These EVs vary in size, content and biologic activity, and this diversity has been a major problem in defining in vivo physiological significance of EVs.Citation2-4. Exosomes are a type of EVs ranging in size from 30 to 100 nm, which originate from fusion of the multivesicular endosome with the plasma membrane and are released by cells via an ATP-dependent mechanism.Citation5 Characteristic properties of exosomes, such as size, morphology, buoyant density and surface protein profiles, distinguish exosomes from other EVs.Citation5,6 Their vesicular content, including nucleic acids, cytokines, enzymes and soluble factors, reflects the cytoplasmic content of the mother cell.Citation7 Exosomes are present in all body fluids, and they are secreted by cells maintained in culture.Citation8 While all cells can produce exosomes, tumor cells, including glioma cells, secrete large quantities of exosomes and, consequently, exosomal fractions obtained from plasma of cancer patients are considerably larger than those obtained from normal donor plasma.Citation8,9 Also, tumor-derived exosomes, referred to as tumor-derived exosomes (TEX), appear to have properties distinct from exosomes secreted by normal cells and are strongly immunosuppressive.Citation9,10 TEX can deliver intercellular signals or transfer bioactive materials to recipient cells thus altering cellular characteristics of these cells.Citation9-11 Because of these properties, TEX have been getting increasing attention as potential clinical biomarkers.
In body fluids of cancer patients, isolated exosome fractions contain tumor-derived as well as normal cell-derived vesicles. Nevertheless, the size, protein content and molecular composition of the exosome fractions obtained from cancer patients' plasma have been reported to reflect the disease status and to be prognostically useful.Citation11 Specifically, Skog and colleagues showed that exosomes carrying glioma-associated proteins and angiogenic factors accumulated in the plasma of patients with glioma and proposed that exosomes could be used as disease biomarkers.Citation12 More recent studies also suggest that in glioma, where few prognostic markers exist, the exosomal cargo determined at the time of diagnosis may be useful in predicting outcome.Citation13
We have optimized a method for the isolation of morphologically and functionally intact exosomes from plasma of patients with cancer.Citation14 Here, we have applied this method to isolate and analyze exosomes from plasma of patients with recurrent malignant glioma who were enrolled in a phase I/II clinical trial. The trial was performed at the University of Pittsburgh Cancer InstituteCitation15 testing safety and efficacy of a novel vaccine which contained α-type1 polarized dendritic cells (αDC1) loaded with synthetic peptides specific for glioma-associated antigen (GAA) epitopes in combination with poly(I:C) and poly(ICLC).Citation15 The trial has demonstrated immunogenicity and clinical activity of this DC-based vaccine.Citation15 Serially-collected, banked plasma samples from the vaccinated patients were available for exosome isolation. The objective of this study was to determine whether identifiable changes in total protein and mRNA expression levels could be observed in plasma-derived exosomes obtained from the patients prior to and after the first vaccination. The hypothesis tested was that changes in the levels of total exosomal protein and in mRNA expression levels for the selected number of immune-related genes in exosomes isolated from patients' plasma samples before and after vaccination could serve as additional biomarkers of response to immunotherapy and of outcome.
Results
Characterization of isolated exosomes
Exosomes were successfully isolated from plasma specimens of all patients or controls. They were visualized by TEM as nanovesicles ranging in size from 20 to less than 100 nm (Fig. S1A). Examination by NanoSight (Fig. S1B) of a representative patient sample gave the mean particle diameter as 111 nm (mean 109 ± 8 nm, n = 6) and the exosome concentration of ca. 3 × 1011/mL of plasma (mean 13 ± 17 × 1011/mL, n = 6).
Total exosomal protein in plasma vs. disease grade
For initial protein determinations, exosomes were isolated from plasma of 10 NC and of 34 glioma patients phlebotomized at the time of diagnosis. The patient cohort included 20 vaccine participants and 14 randomly selected glioma patients. As shown in , total protein levels in the isolated exosome fractions in normal controls (NC) were significantly lower (mean = 9.9 ug/mL plasma) than those measured in exosome fractions isolated from patients with glioma (mean = 76.1 ug/mL plasma). Notably, the protein levels in patients' exosomes varied broadly from near normal levels to up to 175 ug/mL plasma. Further, the total exosome protein levels in glioma patients correlated with the WHO tumor grade: the mean protein value (31.4 ug/mL plasma) for exosomes from patients with WHO grade III tumors (n = 5) was significantly lower than that (104.2 ug/mL plasma) for exosomes from patients with WHO grade IV tumors (n = 6) ().
Figure 1. Total protein in exosomes isolated from plasma samples of normal controls (NC) or patients with glioma. (A) Significantly lower protein levels in exosomes of NC vs. exosomes isolated from plasma of patients with glioma obtained at diagnosis. (B) The WHO grade-dependent increase in protein levels of exosomes isolated from plasma collected prior to vaccination (at baseline) from 11 patients enrolled in the clinical trial. Data for 9 patients with prior tumor recurrence were omitted. (C) Protein concentrations in plasma exosomes from glioma patients before (baseline) and after the first vaccination (week 8). The p value for decreased post-vaccination protein levels was 0.059. In (A), (B), and (C), All protein levels were normalized per 1 mL of plasma. The bars indicate mean values. The data were analyzed by a paired Wilcoxon signed rank test with continuity correction (V = 156).
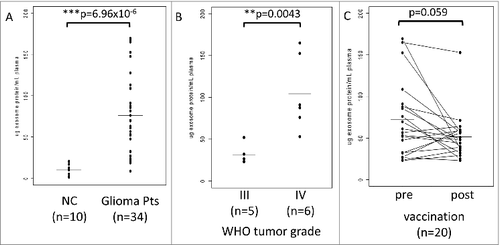
Based on these promising preliminary results, the possibility was considered that vaccinated glioma patients, especially those responding to the vaccine, might have lower exosomal protein levels after vaccination than patients whose disease progressed after vaccination. Total protein levels were measured in exosomes isolated from pre- and post-vaccination plasma specimens derived from 20 patients who participated in the trial. As shown in , the mean protein values were lower after than before vaccination, and in 8/20 patients, protein levels in isolated exosomes showed a dramatic drop to near normal levels after vaccination. However, the difference in mean values (baseline = 72.0 ug/mL plasma; week 8 = 51.4 ug/mL plasma) only had borderline significance (p < 0.059), probably because of limited patient numbers. Notably, regression of the mean for the post values reflected the greatest decreases in high protein values and lowest decreases in low protein values ().
Effects of vaccination on the tumor size vs. exosome protein levels
In 4/19 vaccinated patients (#3, #6, #7 and #18), MRI-detected increases in the tumor size were evident at eight weeks after vaccination (). In the two patients with the highest increase in tumor size, the total exosomal protein levels were increased, although patient #6 had a decrease in the exosomal protein level. The majority of patients showed no change in tumor burden after vaccination, but in two cases, a slight decrease in tumor size was detected (patients #14 and #16) and was associated with a decrease in exosome protein levels (). Overall, no consistent association between tumor burden and exosomal protein levels could be established in this small patient cohort.
Figure 2. The waterfall plot of percent tumor size change in 20 vaccinated glioma patients (from baseline to week 8). Four patients presented with enlarged tumors, and in two, a small decrease in size was observed. Tumor sizes were determined by magnetic resonance imaging (MRI) scans with contrast enhancement as previously reported.Citation12 Patient #13 had no image data available for week 8 (patient survival was 2 months). The numbers indicate increases (+) or decreases (−) in total exosomal protein levels.
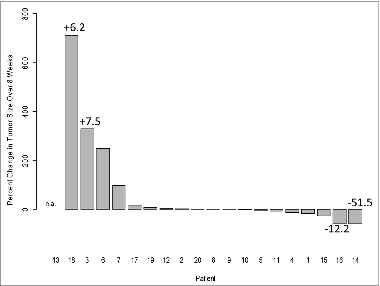
Effects of vaccination on exosomal mRNA content
In addition to total protein levels in exosomes, we also monitored vaccine-induced changes in exosomal mRNA expression levels. In initial experiments, mRNA expression levels for 24 genes potentially relevant to glioma progression were evaluated in exosomes isolated from plasma of three arbitrarily selected non-responders and three responders to the vaccine. Although initially we used a small quantity of exosomes (total protein = 100 ug/mL plasma) and the recovered mRNA levels were low, detectable differences (ΔCt) were seen in mRNA expression levels of five genes (Angiogenin, VEGF, PD-L1, TIMP-1 and IL-8) between the responder and non-responder groups (Fig. S2). These preliminary results encouraged us to further evaluate mRNA expression levels in exosomes isolated from pre- vs. post-vaccination plasma using a greater quantity (150 ug/mL plasma) of exosomes. The pre- to post-vaccine changes in mRNA expression levels (ΔCt) for 24 genes in exosomes isolated from the four patients whose tumor size had increased after vaccination as compared to the two patients whose tumor had decreased in size (), did not reveal any consistent pattern (data not shown). However, the analysis of ΔCt values for all 20 patients indicated substantial decreases in expression levels of TIMP-1, IL-8, TGF-β and ZAP70 genes in the pre- to post-vaccination samples (). These genes have been reported to be related to angiogenesis and to clinical outcome in gliomas.Citation12 Since ACTB expression levels were not altered by vaccination (), the data set was not normalized to ACTB. shows significant increases in ΔCt values for each of the four genes following vaccination. Supplemental Table 1 lists ΔCt values for these genes from pre to post vaccination for all patients.
Figure 3. Changes in mRNA expression levels for selected genes in exosomes isolated from plasma at baseline and eight weeks after vaccination therapy. Significant changes in ΔCt values were seen for IL-8, TIMP-1, TGF-β and ZAP70, but not for actin B (ACTB) as also illustrated in Supplemental Table 1 for ΔCt in each patient's exosomes.
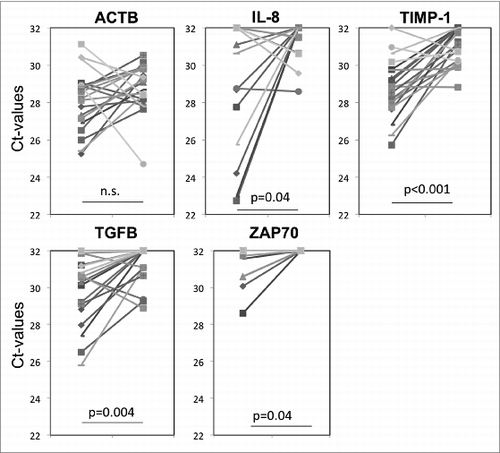
The overall mRNA expression levels for all 24 genes tested at baseline and after vaccination at week 8 in exosomes isolated from plasma of 19 patients are shown as heat maps in . The #15 patient's data are omitted (see the legend to ). The upper heat map shows that at baseline, mRNA expression levels were low, except for TIMP-1, IL-8, TGF-β and ZAP70. The ΔCt values for these four genes increased after vaccination (), which translates into decreased mRNA expression levels. The lower heat map in which profiles gene expression levels in exosomes isolated from plasma of patients at eight weeks post vaccination shows that expression levels of mRNA coding for TIMP-1, IL-8 and ZAP-70, which had the highest expression at baseline, were strongly downregulated (see the lower heat map in ).
Figure 4. Heat maps at baseline (an upper panel) and at 8 weeks post vaccination (a lower panel) for mRNA expression levels for 24 immune-related genes in 19/20 glioma patients. Patient #15 was omitted, since very high expression levels for the PD-1 gene in exosomes were causing a considerable shift in color coding. The boxes on the right show the color key and the distribution of the gene expression is indicated by a solid blue line.
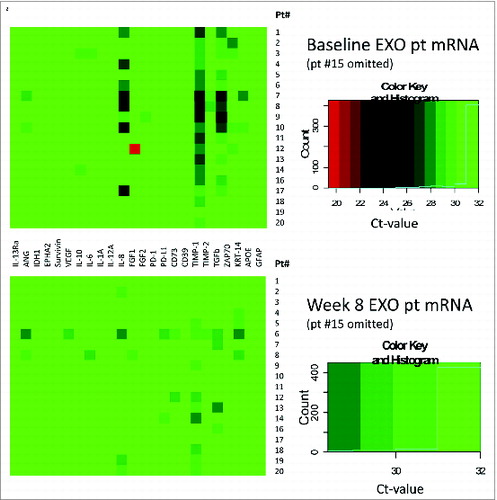
Vaccine-induced changes in mRNA expression levels and disease progression
shows the Kaplan–Meier survival curve for the 20 vaccination patients whose exosomes were isolated and tested in this study. These patients have been followed for a median of 64 months; the minimum follow-up was 25 months. To date, only three patients (#4, #15 and #16) are alive, and only one patient (#15) remains progression-free at 54 months post vaccination. Two of three had AA and one of three (#16) had GBM. All had primary disease. All three had positive immune responses to the vaccine,Citation12 noticeable but variable post-vaccine changes in total exosomal protein and mRNA expression levels, specifically in TIMP-1, IL-8, TGF-β and PD-1 genes ().
Figure 5. Overall survival of the 20 glioma patients presented as a Kaplan–Meier plot. Each number indicates an individual patient. The probability of surviving one year was 0.55 (95% CI = 0.37 – 0.82). The dotted lines represent the 95% confidence interval.
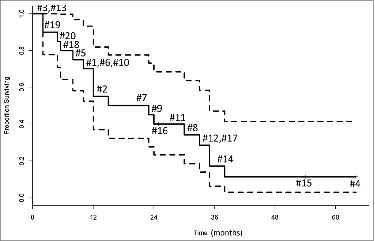
Table 1. Hazard ratios for the four selected genesFootnote
To explore potential correlations between the pre/post vaccine changes (ΔCt values) in expression of the four genes in exosomes and clinical outcome (i.e., TTP [time to progression] and OS [overall survival]), the HR were calculated (). Only the ΔCt for IL-8 mRNA expression weakly correlated with the OS (the HR ratio of 1.56), whereas the same parameter showed a similar association for TTP (the HR of 1.48). For the other mRNA changes, no significant correlations were evident.
mRNA expression levels in exosomes and patients' immunological responses
Within the four genes mentioned above, only ΔCt values for IL-8 and TGF-β genes in exosomes showed a clear decrease, and this decrease correlated with the immunological response, as measured by the positive ELISPOT assaysCitation15 in patients' T cells responding to the GAA GP100 and IL-13R ( but not YKL40) (Fig. S3). Further, the ΔCt value for TIMP-1 mRNA expression after vaccination correlated negatively with ΔCt values for TGF-β and IL-8 in patients' exosomes (Fig. S3).
Comments on patient #15
This patient is a 28-year old man with a primary, recurrent AA of the brainstem. At 54 weeks after vaccine therapy, the patient demonstrated partial response. The lesion measurement in the MRI scan (T2 flair) was 838 mm2 and gradually decreased to become 95 mm2 (11% of the baseline) after 2 years. Interestingly, his total exosomal protein levels increased from 32 ug/mL plasma at baseline to 56.1 ug/mL at week 8. Interestingly, in contrast to all other vaccinated patients, patient #15 showed a striking up-regulation of mRNA expression level for PD-1 in post-vaccination exosomes (13.8 vs. 32.0 at baseline with ΔCt = –18.2). As exosomes carrying mRNA for PD-1 could originate from the tumor or activated immune cells, we phenotyped peripheral blood mononuclear cells of this patient (banked pre- and post-vaccine) for PD-1 protein expression T cells and other potentially relevant immune markers. As indicated in Supplemental Table 2, CD4+ and CD8+ effector T cells with surface expression of PD-1 and CTLA-4 (inhibitory receptors) dramatically declined in frequency after vaccination relative to the baseline values and remained low in T cells collected 2 years later. This suggests a loss of the inhibitory receptors or mRNA coding for these receptors from activated T cells, perhaps via release in exosomes. In aggregate, these data suggest that CD4+ and CD8+ T cells rather than tumor could be a source of post-vaccine exosomes enriched in PD-1 mRNA. Also, the frequency of circulating CD4+FOXP3+CD25high Treg or Treg which express PD-1 decreased after vaccination and remained low over time (Table S1), confirming the post-vaccine immune recovery of patient #15.
Discussion
The potential of exosomes as markers of prognosis or response to therapy in patients with solid tumors, including brain tumors, is being actively investigated.Citation13,16-18 The initial studies in mice suggested that murine brain tumor exosomes had unique features that differentiated them from other exosomes, including expression of the mutated tumor antigen EGFRvIII and of immunosuppressive cytokine TGF-β. Further, these exosomes had immunomodulating properties and influenced tumor growth.Citation19,20 Subsequent studies of exosomes isolated from sera of patients with brain tumors have led to the conclusion that circulating exosomes by virtue of their content, which includes coding and non-coding RNAs in addition to DNA, protein and other cargo, can be used to analyze primary tumor mutations and to predict responses to therapy as well as outcome.Citation13,21-23 Based on these reports and taking advantage of the recently optimized method of exosome isolation from human plasma,Citation14 we obtained pre- and post-therapy exosomes from plasma of glioma patients who participated in a recently completed phase I/II vaccination trial.Citation15 As clinical and immunological responses were seen in this trial, there emerged a possibility of testing whether the total protein or mRNA content of isolated exosomes could be related to clinicopathologic and immunologic parameters in vaccinated patients. Specimens available to us for this study were collected from 20/22 patients immediately prior to and eight weeks after the delivery of the first vaccine. Thus, the study is limited by a small number of patients and a short post-therapy time period. Therefore, only the most robust and early effects evident in harvested exosomes were detected. Another caveat is that total exosomal fractions, and not tumor-derived exosomes, were evaluated. Despite these limitations, analyses of both the total protein and mRNA expression data have yielded information that confirms the usefulness of circulating exosomes as predictors of response to vaccination therapy and of immunologic activity in patients with advanced glioma.
The predictive value of total exosomal protein levels has been described for several human solid and hematologic malignancies.Citation11,24-27 In this study, total protein levels in isolated exosomes discriminated between NC and glioma patients or between glioma patients with grade III and IV tumors. In addition, these protein levels rapidly decreased within an eight week period after vaccination in a large proportion of our patients. It should be noted that the post-vaccine down-regulation in exosomal protein levels did not always correlate with decreases in tumor burden. Especially in some patients with AA, we noted that exosomal proteins increased in values despite the MRI-confirmed tumor shrinkage. As three of four patients with AA in this trial had immunologic responses to the vaccine (the fifth AA patient was not tested), this observation could be explained by the fact that vaccine-activated immune cells may be an as good or even better source of exosomes than tumor cells. It may be that immunologically-responsive AA patients have a more favorable prognosis and accumulate immune cell-derived exosomes in their plasma. In this respect, plasma exosomes could serve as an indirect measure of post-vaccination immune response.
It appears that the mRNA content of plasma-derived exosomes further confirms their potential importance in evaluating glioma response to vaccination therapy. Spearman rank analyses demonstrated positive correlations between immunologic responses to the vaccine defined by ELISPOT and changes in exosomal mRNA coding for IL-8 and TGF-β, cytokines known to be critically important in glioma growth and metastasis.Citation28,29 These results suggest that the exosomal mRNA content reflects vaccine-induced changes in the patients' immune responses. In addition, negative correlations between vaccine-induced changes in exosomal mRNA expression levels for TIMP-1 with changes in IL-8 and TGF-β gene expression could be taken as further evidence that exosome content informs about ongoing cellular responses, whether they take place in the tumor or immune cells after vaccination.Citation30-33 Although these data are sparse, they indicate the potential of exosomal mRNA and protein cargos for monitoring responses to glioma immunotherapy. It is likely that using proteomics in place of total protein levels and/or a broader panel of genes for mRNA analyses in tumor-derived vs. immune cell-derived exosomes and a longer follow-up post-therapy period, exosomes might prove to be an excellent metric for measuring response to glioma therapy as also suggested by Shao et al.Citation21
Among the three glioma patients with longest survival, patient #15 stood out because of the unique exosomal mRNA profile which was enriched in PD-1 and an inverse relationship between total exosomal protein and tumor shrinkage. As this patient was an immunologic and clinical responder to the vaccine, we suspected that activated CD8+ and CD4+ T cells were the source of exosomes carrying mRNA for PD-1. Phenotyping of PD-1+ and CTLA-4+ T lymphocyte subsets in the peripheral circulation of patient #15 showed a drastically decreased frequency of CD8+ and CD4+ effector T cells expressing the inhibitory receptors after therapy vs. baseline. At the same time, the CD4+/CD8+ T-cell ratio stabilized and the frequency of CD4+CD25highFOXP3+ Treg substantially decreased after vaccination. These data are consistent with immunologic post-vaccine recovery that is accompanied by enrichment in plasma exosomes carrying mRNA for PD-1. The source of these exosomes may be activated T cells, which recover their immunologic function after successful vaccination.
In this study, evaluations of the protein and mRNA content of exosomes isolated from plasma of glioma patients receiving immune-based therapy appear to be informative and to provide additional evidence for the role of vaccine-induced immunological responses in clinical outcome.
Patients and Methods
Patients, normal donors and plasma specimens
Twenty-two patients (13 with glioblastoma multiformae [GBM], 5 with AA, 3 with anaplastic oligodendroglioma [AO]) and one with anaplastic oligoasrocytoma (AOA) were enrolled in a phase I/II vaccination trial as previously reported.Citation15 The patients received at least one vaccination, and 19 patients received at least four vaccinations at two αDC1 dose levels (1× or 3 × 10e×7/dose at two week intervals intranodally). Patients also received twice weekly intramuscular injections of 20 μg/kg poly-ICLC. T-lymphocyte responses against GAA epitopes were assessed by ELISA and HLA-tetramer assays. Plasma samples were collected prior to vaccination and after the first vaccine (week 8 of therapy) and were banked. Samples were available for this study from 20/22 enrolled patients. Specimens for 1/13 GBM patients and 1/3 AO patients were not available. Among 20 patients, 12 were male and 8 were female. The age range was 21–71 years, with a mean of 47 years. The OS for these patients was 22 months and TTP was 15 months. Eight out of twenty patients had immune response after the first vaccine as measured by tetramers and ELISPOT assays.Citation12 Plasma samples obtained at diagnosis were also banked from all patients on the protocol and from additional glioma patients not enrolled in the vaccination protocol (n = 14) as well as from 10 normal age- and sex-matched volunteers (NC). The NC cohort included 6 males and 4 females. The 14 glioma patients not enrolled in the protocol were randomly selected from patients seen by the Neurosurgery Clinic at the UPCI. Venous blood specimens were collected, processed and banked from these subjects under the IRB-approved protocols (IRB #960279 and IRB# 0403105, respectively). Informed consent before blood draws was obtained from all subjects.
Exosome isolation
Exosomes were isolated from human plasma specimens as previously described.Citation14 Briefly, 2 mL aliquots of plasma were differentially centrifuged, ultrafiltrated (0.22 μm filter, Millipore, Billicera, MA, USA) and individually separated by size exclusion chromatography on Sepharose 2B-packed A50m columns (Bio-Rad Laboratories, Hercules, Ca, USA). Exosomes were isolated by ultracentrifugation of the collected exclusion-volume fractions. The total protein content of isolated exosome preparations was measured in a BCA protein assay (Thermo Scientific Rockford, IL, USA). All protein values were normalized to 1 mL of normal or patient plasma.
NanoSight measurements
Isolated exosomes were diluted in PBS to obtain a uniform particle distribution, and were evaluated in a NanoSight Instrument (Malvern Instruments Ltd, Malvern, UK) as previously described.Citation14 The settings were as previously reported, and at least 900 particles per sample were counted.
Transmission electron microscopy (TEM)
TEM was performed in the Center for Biologic Imaging at the University of Pittsburgh as described by us.Citation14 Isolated exosomes were placed on a copper grid coated with 0.125% Formvar in chloroform. After staining with 1% v/v uranyl acetate in ddH2O, the samples were visualized using a JEOL 1011 transmission electron microscope.
Flow cytometry
Banked peripheral blood mononuclear cells of one patient (#15) were thawed and used for multiparameter flow cytometry. Cells were incubated in the dark for 20 min at room temperature with a panel of mAbs as follows: anti-CD4-eFluor 450 (48-0048-42, eBioscience, CA, USA), CD8-ECD (6604728, Beckman Coulter, Brea, CA, USA), PD-1-PE (12-2799-42, BioLegend), CD25-APC/Cy7 (302613, BioLegend, San Diego, CA, USA) and Foxp3-APC (17-4776-41, eBioscience). Isotype controls were used for each experiment. Cells were again washed, re-suspended in flow buffer and analyzed using Gallios flow cytometer (Beckman Coulter). At least 5 × 104 events were collected, and the data were analyzed using Kaluza software (Beckman Coulter).
mRNA extraction from exosomes
mRNA was extracted from isolated exosomes, reverse transcribed and analyzed in 96-well aRT-PCR assays for expression of 24 immune response-related genes and glioma progression-related genes: ACTB, IL13-Ra, ANG, IDH1, EPHA2, Survivin, VEGF, IL-10, IL-6, IL1a, IL-12a, IL-8, FGF-1, FGF-2, PD-1, PD-L1, CD73, CD39, TIMP-1, TIMP-2, TGF-β, ZAP70, KRT-14, APOE and GFAP. Supplemental Table 3 lists the primer sequences used. Isolated exosomes were dispensed into individual wells of a filter plate based on their protein content. Exosomes were lysed and the lysates were transferred to an oligo (dT)-immobilized microplate for capture of poly (A)+RNA. Next, cDNA was synthesized using target gene-specific antisense primers cocktail on the plate. As previously described.Citation34,35 In this system, cDNA primed by specific primers comes to solution, while oligo (dT)-primed cDNA remains immobilized on the plate. Reverse transcription PCR of the solubilized specifically primed cDNA follows. In the absence of reverse transcriptase, no amplification takes place in this system.Citation34,35 For PCR, the initial temperature cycle of 95°C for 5 min was followed by 45 cycles of 95°C for 30 s, 65°C for 1 min in a final volume of 5 μL of 1x SYBR green PCR master mix (Bio-Rad, Hercules, CA, USA) in a 384-well plate. The cycle threshold (Ct) was determined by the analytical software (SDS, Applied Biosystems). A Ct of 32 was used as a baseline. All samples were analyzed in triplicate and all samples for a single experiment were tested simultaneously. Results are expressed as ΔCt values. The sensitivity and reproducibility of this method was previously described.Citation34,35
Statistics
The values for total exosomal protein determined for samples of off-protocol glioma patients, on- protocol patients at diagnosis and NC were compared using the two-tailed Wilcoxon test. Paired (pre-post therapy) clinical trial specimens were plotted by subject, and the pre and post values were compared using the two tailed signed rank test. The Spearman correlation coefficient was estimated for assessing association changes in total protein, gene-specific mRNA expression or tumor response. OS for glioma patients participating in this trial was estimated by the Kaplan–Meier method. Computations were conducted with the statistical package R (R Development Core Team (2008). R: A language and environment for statistical computing. R Foundation for Statistical Computing, Vienna, Austria. ISBN 3-900051-07-0, http://www.R-project.org).
Disclosure of Potential Conflicts of Interest
No potential conflicts of interest were disclosed.
Supplemental Material
Supplemental data for this article can be accessed on the publisher's website.
1008347_Supplementary_Materials.zip
Download Zip (649.7 KB)Funding
This study was supported in part by NIH grants R0-1 CA168628 to TLW and 2P0-1 NS40923 and 1R21 CA117152 to H.O. Dr. Muller was supported by the Swiss National Foundation grant #PBSK3_140119/1.
References
- Corrado C, Raimondo S, Chiesi A, Ciccia F, De Leo G, Alessandro R. Exosomes as intercellular signaling organelles involved in health and disease: basic science and clinical applications. Int J Mol Sci 2013; 14:5338-66; PMID:23466882; http://dx.doi.org/10.3390/ijms14035338
- Cocucci E, Racchetti G, Meldolesi J. Shedding microvesicles: artefacts no more. Trends Cell Biol 2008; 19:43-51; http://dx.doi.org/10.1016/j.tcb.2008.11.003
- Gyorgy B, Szabo TG, Pasztoi M, Pal Z, Misják P, Aradi B, László V, Pállinger E, Pap E, Kittel A et al. Membrane vesicles current state-of-the-art: emerging role of extracellular vesicles. Cell Mol Life Sci 2011; 68:2667-88; PMID:21560073; http://dx.doi.org/10.1007/s00018-011-0689-3
- Van der Pol E, Boing AN, Harrison P, Sturk A, Nieuwland R. Classification, functions, and clinical relevance of extracellular vesicles. Pharmacol Rev 2012; 64:676-705; PMID:22722893; http://dx.doi.org/10.1124/pr.112.005983
- Raposo G, Stoorvogel W. Extracellular vesicles: exosomes, microvesicles, and friends. J Cell Biol 2013; 200:373-83; PMID:23420871; http://dx.doi.org/10.1083/jcb.201211138
- Chaput N, Théry C. Exosomes: immune properties and potential clinical implementations. Semin Immunopathol 2011; 33:419-40; PMID:21174094; http://dx.doi.org/10.1007/s00281-010-0233-9
- Record M, Subra C, Silvente-Poirot S, Poirot M. Exosomes as intercellular signalosomes and pharmacological effectors. Biochem Pharmacol 2011; 81:1171-82; PMID:21371441; http://dx.doi.org/10.1016/j.bcp.2011.02.011
- Théry C, Amigorena S, Raposo G, Clayton A. Isolation and characterization of exosomes from cell culture supernatants and biological fluids. Curr Protoc Cell Biol 2006; Chapter 3:Unit 3.22; PMID:18228490; http://dx.doi.org/10.1002/0471143030.cb0322s30
- D'Asti E, Garnier D, Lee TH, Montermini L, Meehan B, Rak J. Oncogenic extracellular vesicles in brain tumor progression. Front Physiol 2012; 3:294; PMID:22934045; http://dx.doi.org/10.3389/fphys.2012.00294
- Whiteside TL. Immune modulation of T-cell and NK (natural killer) cell activities by TEXs (tumour-derived exosomes). Biochem Soc Trans 2013; 41:245-51; PMID:23356291; http://dx.doi.org/10.1042/BST20120265
- Peinado H, Alečković M, Lavotshkin S, Matei I, Costa-Silva B, Moreno-Bueno G, Hergueta-Redondo M, Williams C, García-Santos G, Ghajar C et al. Melanoma exosomes educate bone marrow progenitor cells toward a pro-metastatic phenotype through MET. Nat Med 2012; 18:883-91; PMID:22635005; http://dx.doi.org/10.1038/nm.2753
- Skog J, Würdinger T, van Rijn S, Meijer DH, Gainche L, Sena-Esteves M, Curry WT Jr, Carter BS, Krichevsky AM, Breakefield XO. Glioblastoma microvesicles transport RNA and proteins that promote tumour growth and provide diagnostic biomarkers. Nat Cell Biol 2008; 10:1470-6; PMID:19011622; http://dx.doi.org/10.1038/ncb1800
- Redzic JS, Ung TH, Graner MW. Glioblastoma extracellular vesicles: reservoirs of potential biomarkers. Pharmgenomics Pers Med 2014; 7:65-77; PMID:24634586; http://dx.doi.org/10.2147/PGPM.S30768
- Muller L, Hong CS, Stolz DB, Watkins SC, Whiteside TL. Isolation of biologically-active exosomes from human plasma. J Immunol Methods 2014; 411:55-65; PMID:24952243; http://dx.doi.org/10.1016/j.jim.2014.06.007
- Okada H, Kalinski P, Ueda R, Hoji A, Kohanbash G, Donegan TE, Mintz AH, Engh JA, Bartlett DL, Brown CK et al. Induction of CD8+ T-cell responses against novel glioma-associated antigen peptides and clinical activity by vaccinations with {alpha}-type 1 polarized dendritic cells and polyinosinic-polycytidylic acid stabilized by lysine and carboxymethylcellulose in patients with recurrent malignant glioma. J Clin Oncol 2011; 29(3):330-6; PMID:21149657; http://dx.doi.org/10.1200/JCO.2010.30.7744
- Taylor DD, Gercel-Taylor C. The origin, function, and diagnostic potential of RNA within extracellular vesicles present in human biological fluids. Front Genet 2013; 4:142; PMID:23908664; http://dx.doi.org/10.3389/fgene.2013.00142
- Chistiakov DA, Chekhonin VP. Extracellular vesicles shed by glioma cells: pathogenic role and clinical value. Tumour Bio 2014; 35:8425-38; PMID:24969563; http://dx.doi.org/10.1007/s13277-014-2262-9
- Manterola L, Guruceaga E, Gállego Pérez-Larraya J, González-Huarriz M, Jauregui P, Tejada S, Diez-Valle R, Segura V, Samprón N, Barrena C et al. A small noncoding RNA signature found in exosomes of GBM patient serum as a diagnostic tool. Neuro Oncol 2014;16:520-7; PMID:24435880; http://dx.doi.org/10.1093/neuonc/not218
- Graner MW, Alzate O, Dechkovskaia AM, Keene JD, Sampson JH, Mitchell DA, Bigner DD. Proteomic and immunologic analyses of brain tumor exosomes. FASEB J 2009; 23:1541-57; PMID:19109410; http://dx.doi.org/10.1096/fj.08-122184
- Kucharzewska P, Christianson HC, Welch JE, Svensson KJ, Fredlund E, Ringnér M, Mörgelin M, Bourseau-Guilmain E, Bengzon J, Belting M. Exosomes reflect the hypoxic status of glioma cells and mediate hypoxia-dependent activation of vascular cells during tumor development. Proc Natl Acad Sci U S A 2013; 110:7312-7; PMID:23589885; http://dx.doi.org/10.1073/pnas.1220998110
- Shao H, Chung J, Balaj L, Charest A, Bigner DD, Carter BS, Hochberg FH, Breakefield XO, Weissleder R, Lee H. Protein typing of circulating microvesicles allows real-time monitoring of glioblastoma therapy. Nat Med 2012; 18:1835-40; PMID:23142818; http://dx.doi.org/10.1038/nm.2994
- Li CC, Eaton SA, Young PE, Lee M, Shuttleworth R, Humphreys DT, Grau GE, Combes V, Bebawy M, Gong J et al. Glioma microvesicles carry selectively packaged coding and non-coding RNAs which alter gene expression in recipient cells. RNA Biol 2013; 10:1333-44; PMID:23807490; http://dx.doi.org/10.4161/rna.25281
- Noerholm M, Balaj L, Limperg T, Salehi A, Zhu LD, Hochberg FH, Breakefield XO, Carter BS, Skog J. RNA expression patterns in serum microvesicles from patients with glioblastoma multiforme and controls. BMC Cancer 2012; 12:22; PMID:22251860; http://dx.doi.org/10.1186/1471-2407-12-22
- Szczepanski MJ, Szajnik M, Welsh A, Whiteside TL, Boyiadzis M. Blast-derived microvesicles in sera from patients with acute myeloid leukemia suppress natural killer cell function via membrane-associated transforming growth factor-beta1. Haematologica 2011; 96:1302-9; PMID:21606166; http://dx.doi.org/10.3324/haematol.2010.039743
- Hong CS, Muller L, Whiteside TL, Boyiadzis M. Plasma exosomes as markers of therapeutic response in patients with acute myeloid leukemia. Front Immunol 2014; 5:160; PMID:24782865; http://dx.doi.org/10.3389/fimmu.2014.00160
- Khan S, Jutzy JM, Valenzuela MM, Turay D, Aspe JR, Ashok A, Mirshahidi S, Mercola D, Lilly MB, Wall NR. Plasma-derived exosomal survivin, a plausible biomarker for early detection of prostate cancer. PLoS One 2012; 7:e46737; PMID:23091600; http://dx.doi.org/10.1371/journal.pone.0046737
- Chiorean R, Berindan-Neagoe I, Braicu C, Florian IS, Leucuta D, Crisan D, Cernea V. Quantitative expression of serum biomarkers involved in angiogenesis and inflammation, in patients with glioblastoma multiforme: correlations with clinical data. Cancer Biomark 2014; 14:185-94; PMID:24878820; http://dx.doi.org/10.3233/CBM-130310
- Roy LO, Poirier MB, Fortin D. Transforming growth factor-beta and its implication in the malignancy of gliomas. Target Ocol 2015; 10:1-14; Published online, March 5, 2014; PMID:24590691
- Sun J, Liu SZ, Lin Y, Cao XP, Liu JM. TGF-β promotes glioma cell growth via activating Nodal expression through Smad and ERK1/2 pathways. Biochem Biophys Res Commun 2014; 443:1066-72; PMID:24370825; http://dx.doi.org/10.1016/j.bbrc.2013.12.097
- Van Meir E, Ceska M, Effenberger F, Walz A, Grouzmann E, Desbaillets I, Frei K, Fontana A, de Tribolet N. Interleukin-8 is produced in neoplastic and infectious diseases of the human central nervous system. Cancer Res 1992; 52:4297-305; PMID:1643627
- Brat DJ, Bellail AC, Van Meir EG. The role of interleukin-8 and its receptors in gliomagenesis and tumoral angiogenesis. Neuro Oncol 2005; 7(2):122-33; PMID:15831231; http://dx.doi.org/10.1215/S1152851704001061
- Aaberg-Jessen C, Christensen K, Offenberg H, Bartels A, Dreehsen T, Hansen S, Schrøder HD, Brünner N, Kristensen BW. Low expression of tissue inhibitor of metalloproteinases-1 (TIMP-1) in glioblastoma predicts longer patient survival. J Neurooncol 2009; 95:117-28; PMID:19430729; http://dx.doi.org/10.1007/s11060-009-9910-8
- Crocker M, Ashley S, Giddings I, Petrik V, Hardcastle A, Aherne W, Pearson A, Bell BA, Zacharoulis S, Papadopoulos MC. Serum angiogenic profile of patients with glioblastoma identifies distinct tumor subtypes and shows that TIMP-1 is a prognostic factor. Neuro Oncol 2011; 13:99-108; PMID:21163810; http://dx.doi.org/10.1093/neuonc/noq170
- Mitsuhashi M. Ex vivo simulation of leukocyte function: stimulation of specific subset of leukocytes in whole blood followed by the measurement of function-associated mRNAs. J Immunol Methods 2010; 363(1):95-100; PMID:20951704; http://dx.doi.org/10.1016/j.jim.2010.10.002
- Aoki J, Ohashi K, Mitsuhashi M, Murakami T, Oakes M, Kobayashi T, Doki N, Kakihana K, Sakamaki H. Post-transplantation bone marrow assessment by quantifying hematopoietic cell-derived mRNAs in plasma exosomes/microvesicles. Clin Chem 2014; 60:675-82; PMID:24452836; http://dx.doi.org/10.1373/clinchem.2013.213850