Abstract
In a phase IV trial, 84 patients (age 18–79) with acute myeloid leukemia (AML) in first complete remission (CR) received cycles of immunotherapy with histamine dihydrochloride (HDC) and low-dose human recombinant interleukin 2 (IL-2) for 18 months to prevent leukemic relapse. During cycles, the treatment resulted in expansion of CD56bright (CD3−/16−/56bright) and CD16+ (CD3−/16+/56+) natural killer (NK) cells in the blood along with increased NK cell expression of the natural cytotoxicity receptors (NCRs) NKp30 and NKp46. Multivariate analyses correcting for age and risk group demonstrated that high CD56bright NK cell counts and high expression of NKp30 or NKp46 on CD16+ NK cells independently predicted leukemia-free survival (LFS) and overall survival (OS). Our results suggest that the dynamics of NK cell subsets and their NCR expression may determine the efficiency of relapse-preventive immunotherapy in AML.
Introduction
AML is characterized by the rapid accumulation of abnormal myeloid cells in the bone marrow and other organs. In the United States, AML is the most common form of acute leukemia in adults with 20,000 estimated new cases in 2015.Citation1 The current therapy comprises induction chemotherapy aiming at reducing the burden of the leukemia to microscopically undetectable levels (CR) and consolidation chemotherapy aiming at eradicating residual malignant cells. Younger AML patients with high-risk disease may also be subjected to allogeneic stem cell transplantation, which is usually performed in the post-consolidation phase. Despite advances in AML therapy in recent decades, the 5-year survival rate is in the range of 25–40%, which is significantly explained by a high incidence of leukemic relapse.Citation2-5 Novel therapies, including strategies to prevent relapse in the post-chemotherapy phase, are highly warranted.
Myeloid leukemic cells are frequently susceptible to the cytotoxicity of NK cells. The results of clinical studies imply that a deficient NK cell-mediated cytotoxicity and reduced NK cell counts in the blood are common in AML and that NK cell functions may determine the risk of relapse and survival.Citation6-14 The claim that NK cell function is relevant to the course of AML is further bolstered by reports of a low incidence of relapse in allo-transplanted patients with donor/recipient mismatches of killer immunoglobulin-like receptors (KIR) and HLA, whose leukemic cells are incapable of inhibiting alloreactive NK cells via KIR/HLA interactionsCitation15,16 (reviewed in Citationref. 17).
Human NK cells comprise two main cellular phenotypes that differ in function and in their expression of the cell surface markers CD16 and CD56.Citation18 In healthy subjects, 90–95% of blood NK cells are CD16+/56+ (here referred to as CD16+ NK cells) and 5–10% are CD16−/56bright cells (CD56bright NK cells). CD16+ NK cells are cytotoxic to several histiotypes of malignant cells, including myeloid leukemic cells, whereas CD56bright NK cells are only weakly cytotoxic and are widely accepted to be precursors to the cytotoxic CD16+ NK cells.Citation19-22 NK cell cytotoxicity is regulated by activating and inhibitory NK cell receptors and their cognate ligands on leukemic cells.Citation23-26 The main inhibitory receptors encompass the family of KIRs and the NKG2A – CD94 heterodimer, whereas the main activating receptors comprise the NCRs (NKp46, NKp30, and NKp44) and NKG2D.Citation23-26
The insufficiency of NK cells in AML and the purported prognostic role of NK cells have inspired the design of NK cell-based immunotherapies, including the use of NK cell-activating cytokines and the adoptive transfer of NK cells, for relapse prevention.Citation13,27 In a phase III trial, immunotherapy with histamine dihydrochloride and low-dose interleukin-2 (HDC/IL-2) was shown to prevent relapse in patients with AML who had achieved CR and completed the phase of consolidation chemotherapy.Citation28 The IL-2 component of this regimen aims at promoting antitumor functions of NK cells, whereas the HDC component aims at reducing the production of immunosuppressive reactive oxygen species (ROS) from malignant and non-malignant myeloid cells, and thus at rescuing NK cells from ROS-induced inactivation and apoptosis.Citation29-32 In vitro and in vivo studies imply that NK cells are essential for the anti-neoplastic efficacy of HDC, used as a single agent or in conjunction with IL-2Citation31,33,34; however, a systematic analysis of the immunomodulatory properties of treatment with HDC/IL-2 within the framework of a clinical trial has not been carried out.
Here we report the results of a phase IV trial (Re:Mission trial; NCT01347996) in patients with AML who received immunotherapy with HDC/IL-2 for relapse control. Our results suggest that subsets of NK cells and their NCR expression are induced during immunotherapy and that these aspects of NK cell biology herald favorable outcome in terms of relapse risk and survival.
Results
Immunotherapy with HDC/IL-2 triggers accumulation of NK cell subsets in the blood
AML patients in first CR received 10 consecutive 3-week cycles of HDC/IL-2 in the post-consolidation phase. Peripheral blood was collected before and after treatment cycles 1 and 3, and analyzed for NK cell content and phenotype. The treatment schema is outlined in . Treatment with HDC/IL-2 induced a 3-fold increase in the absolute number of blood NK cells during cycle 1. An increment of NK cell counts was observed in 46/47 evaluable patients. The expanded NK cells comprised CD56bright and CD16+ NK cells (, with gating strategies shown in Fig. S1). NK cell numbers in the blood declined between the end of treatment cycle 1 and the start of cycle 3. CD56bright cell counts typically returned to pre-treatment levels, whereas CD16+ NK cell counts remained modestly elevated at the onset of cycle 3 as compared with levels at the onset of cycle 1 (p < 0.01, paired t-test; n = 46). A renewed accumulation of CD56bright and CD16+ NK cells in the blood was observed during treatment cycle 3 ().
Figure 1. Overview of the Re:Mission phase IV trial. AML patients in first complete remission (CR) were evaluated for eligibility after induction and consolidation chemotherapy. Eligible patients received 3-week cycles of HDC/IL-2 over 18 months. Peripheral blood mononuclear cells (PBMC) were collected before and after cycles 1 and 3. The protocol specified additional follow-up for 6 months after completing the last treatment cycle.
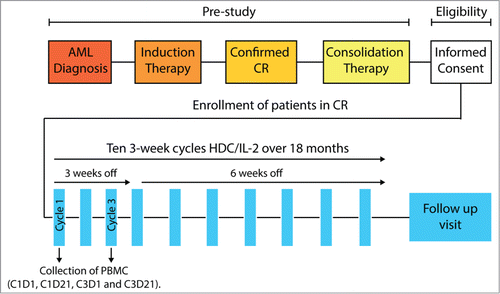
Figure 2. Induction of NK cell subsets in the blood during immunotherapy with HDC/IL-2. (A) shows representative dot plots of the expression intensity of CD16 and CD56 on CD3− cells in samples obtained at the onset of cycle 1 (C1D1), at the end of cycle 1 (C1D21), at the onset of cycle 3 (C3D1) and at the end of cycle 3 (C3D21). Figures indicate the percentage of CD56bright and CD16+ NK cells of CD3- lymphocytes. (B and C) show blood counts of CD56bright and CD16+ NK cells before and after cycles 1 and 3. Treatment with HDC/IL-2 increased blood counts of CD56bright cells and CD16+ NK cells during cycle 1 (n = 47) and cycle 3 (n = 46; Student's paired t-test).
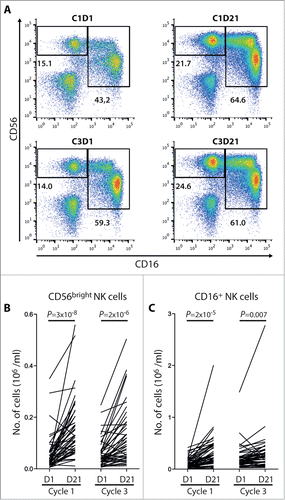
Induction of NCR expression on NK cells
During cycle 1 the median fluorescence intensity (MFI) of NKp30 and NKp46 expressed by CD16+ NK cells increased by 30% and 50%, respectively (). The MFI of NCRs on CD16+ NK cells increased during cycle 1 in 50/56 evaluable patients for NKp30 and in 43/56 patients for NKp46. For CD56bright cells, the results were discordant with a pronounced induction of NKp30 and no induction of NKp46 during cycle 1 (). As was observed for NK cell counts, the expression intensity of these NCRs declined during the resting period between cycles. However, for CD56bright cells the NKp30 expression remained significantly elevated at the onset of cycle 3 compared with levels at the onset of cycle 1 and was significantly higher at the end of cycle 3 than at the end of cycle 1 (P < 0.05, paired t-test; n = 42). The NKp30 expression on CD56bright cells, as well as the NCR expression on CD16+ NK cells, increased during cycle 3 to an extent similar to that recorded during cycle 1 ().
Figure 3. Induction of NCRs during immunotherapy with HDC/IL-2. The box plots show the median fluorescence intensity (MFI) of NKp30 and NKp46 expression on CD56bright (A and C) and CD16+ NK cells (B and D) at indicated time points (D1 = day 1). NKp30 was induced in both NK cell subsets during cycle 1 (n = 56) and during cycle 3 (n = 48). NKp46 was significantly up-regulated on CD16+ NK cells during both cycles, while no significant induction of NKp46 was observed on CD56bright cells (Student's paired t-test).
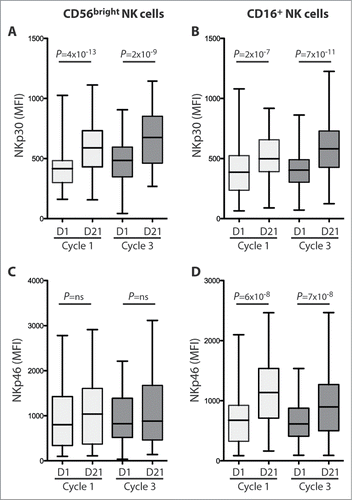
Impact of NK cell counts and NCR expression on LFS and OS
In analyses of the impact of NK cell markers on clinical outcome (reflected by leukemia-free survival, LFS, and overall survival, OS), patients were dichotomized at the median with respect to NK cell counts in the blood or NCR expression (MFI) on NK cells and analyzed for LFS and OS using the logrank test. Hazard ratios were determined by univariate Cox regression analyses and multivariate Cox analyses adjusted for age and risk groups. There was a strong correlation between relapse and death – one patient (59 years old) died without a preceding relapse (from myocardial infarction at 19 months after the onset of treatment) – emphasizing that relapse is a major risk factor for death for AML patients in CR.Citation35,36
At the onset of the first cycle of therapy, high counts of CD56bright NK cells predicted LFS, which translated into an improved OS ( with multivariate analysis shown in ). In contrast, CD16+ NK cell blood counts at onset of cycle 1 did not influence outcome (). Also, LFS and OS were not significantly impacted by the magnitude of the increment of CD56bright or CD16+ NK cell counts during the first cycle of therapy (data not shown) or by the absolute counts of these NK cell populations on day 21 of cycle 1 ().
Figure 4. Impact of NK cell subsets on LFS and OS in AML patients receiving HDC/IL-2. Patients were dichotomized based on above (red) or below (black) median blood cell counts of CD56bright (A, B and D, E) or CD16+ (C and F) NK cells before or after one cycle of treatment and analyzed with regard to LFS (A, C, D and F) and OS (B and E). LFS and OS were analyzed using the logrank test.
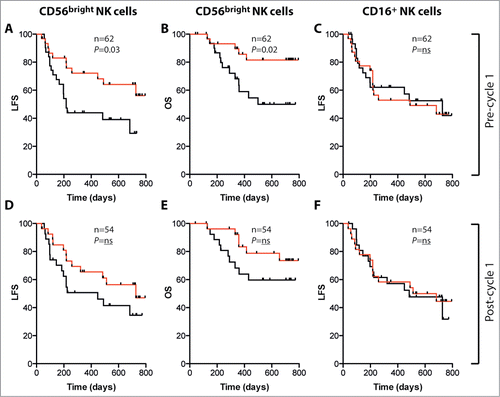
Table 1. Multivariate analysis of LFS and OS. LFS or OS in patients with above or below median values of each variable as determined by univariate and multivariate Cox regression analyses. In the multivariate analyses, hazard ratios were corrected for age and risk group classification.
A high (above median) expression of NKp46 on CD16+ NK cells at the onset of cycle 1 was associated with a favorable outcome ( and ). The magnitude of NCR induction during cycle 1 did not predict LFS or OS. However, patients with high NKp46 MFI on CD16+ NK cells on day 21 of cycle 1 showed a favorable outcome ( and ). A similar trend was observed for patients with high NKp30 expression before and after cycle 1 (). There were also trends toward a favorable outcome in patients with high NKp30 and NKp46 expression on CD56bright NK cells (Fig. S2).
Figure 5. Impact of NCR expression on LFS and OS in AML patients receiving HDC/IL-2. Patients were dichotomized based on above (red) or below (black) median expression (MFI) of NKp30 (A and D) or NKp46 (B, C, E and F) on CD16+ NK cells before or after first treatment cycle. LFS and OS were analyzed using the logrank test.
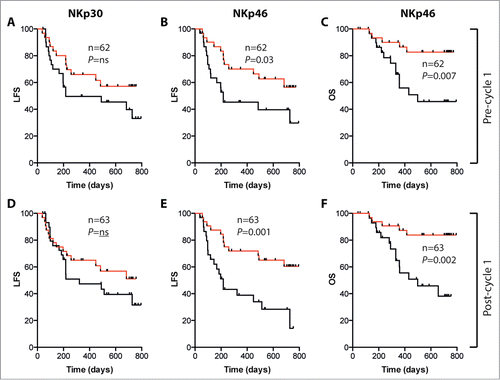
Treatment-induced accumulation of CD16+ NK cells predicts outcome
At the onset of therapy, there was a skewed ratio of CD56bright cells to CD16+ cells in favor of CD56bright cells (median ratio = 0.58 on cycle 1, day 1 vs. <0.1 in healthy subjects), which is in agreement with previous studies.Citation37,38 The increase of CD16+ NK cell counts between the onset of treatment in cycle 1 and the start of cycle 3 resulted in a shift of the distribution of NK cell subtypes in blood toward a normalized ratio of CD56bright to CD16+ NK cells between cycle 1, day 1 and cycle 3, day 1 (0.58 vs. 0.28, median ratios, p = 0.001, signed rank test, n = 46). When assessing the induction of CD16+ NK cells in relapsing and non-relapsing patients followed for at least 18 months, a significant induction was observed only in patients who remained in remission (). In line with this finding, there was a trend toward a favorable LFS and OS in patients with a high maintained level of CD16+ NK cells at the onset of cycle 3 ( and ).
Figure 6. Impact of sustained expansion of CD16+ NK cells on LFS and OS. (A) shows the absolute blood counts of CD16+ NK cells on day 1 of cycles 1 and 3 (C1D1 and C3D1) in relapsing (n = 16) and non-relapsing (n = 16) patients followed for more than 18 months. In (B) and (C), patients were dichotomized based on above (red) or below (black) the median induction of CD16+ NK cells between C1D1 and C3D1. LFS (B) and OS (C) were analyzed using the logrank test. Panels (D)–(F) show post-hoc results from a phase III AML trial (0201), where patients in CR were randomized to receive HDC/IL-2 or no treatment (control). (D) shows lymphocyte counts at the onset of treatment and at the onset of cycle 3 (C1D1 and C3D1) in HDC/IL-2-treated patients and controls, analyzed using the Student's paired t-test. In (E) and (F), HDC/IL-2-treated patients were dichotomized based on above (red) or below (black) median induction of lymphocytes between C1D1 and C3D1. LFS (E) and OS (F) were analyzed using the logrank test.
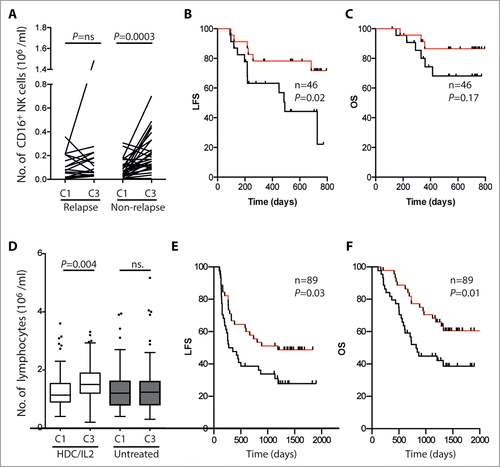
Post-hoc analyses of lymphocyte increment vs. LFS in a phase III trial
In an attempt to confirm that CD16+ NK cells accumulated in the blood between the onset of cycles 1 and 3, we performed post-hoc analyses of the results of a phase III trial (M0201 trial) that compared outcomes in AML patients in CR who were randomly assigned to receive HDC/IL-2 (n = 160) or standard-of-care (n = 160).Citation28 Lymphocyte counts in the blood, but not lymphocyte phenotypes, were captured before and during treatment cycles. In the phase III trial, the absolute lymphocyte counts increased between onset of treatment (C1D1) and the start of cycle 3 in HDC/IL-2-treated patients (p = 0.004), but not in untreated control patients ().Citation39 The increment of lymphocyte counts between treatment cycles in this phase III trial likely reflected an accumulation of CD16+ NK cells, since CD16+ NK cells accounted for the majority (median 53%) of the lymphocyte increment between the onset of cycles 1 and 3 in the current phase IV Re:Mission trial using the identical treatment regimen. In agreement with the results in the Re:Mission trial, the lymphocyte induction between the onset of treatment and the start of cycle 3 in HDC/IL-2 treated patients in the phase III trial was predictive of LFS and OS (). No such correlations were observed in the corresponding control patients (p > 0.5, data not shown).
Discussion
The purported role of NK cells for the outcome of AML has inspired attempts at pharmacologically enhancing NK cell functions to improve clearance of leukemic cells and thus preventing relapse. Such immunotherapy seems intuitively attractive for patients in CR who have completed consolidation chemotherapy and thus carry a minimal burden of leukemia. However, most previous attempts to boost the functions of endogenous NK cells for relapse prevention in AML have been unsuccessful. For example, monotherapy with IL-2, a prototypic NK cell-activating cytokine, in the post-consolidation phase of AML did not significantly prevent relapse or prolong the duration of CR in any of six randomized trials.Citation40-45 The inefficiency of IL-2 monotherapy to prevent relapse is supported by the results of meta-analyses comprising a total of >1,400 IL 2-treated patients.Citation36,46
HDC is assumed to improve the anti-leukemic efficiency of NK cell activators by targeting immunosuppressive ROS.Citation29-32 These toxic oxygen derivatives, which are formed by the NADPH oxidase (NOX2) of several subsets of myeloid cells, are pivotal effector molecules in anti-microbial defense but have also been ascribed a role as negative regulators of cellular immunity by inducing dysfunction and apoptosis in adjacent NK cells and T cells.Citation30,31,47 HDC blocks the activity of NOX2 by targeting H2-type histamine receptors. The resulting reduction of extracellular release of ROS from myeloid cells rescues NK cells from inhibition and apoptosis and thus promotes activation of anti-leukemic properties of IL-2 and other NK cell-activating compounds.Citation29-31 The synergy between IL-2 and HDC in activating NK cells in vitro Citation31,48 and in reducing the growth of NK cell-sensitive tumors in murine models in vivo Citation33 formed the basis for the evaluation of the clinical efficacy of HDC/IL-2 in several forms of cancer.Citation49,50 A phase III trial with 320 non-transplanted AML patients in CR in the post-consolidation phase showed a significantly improved LFS and a reduced incidence of relapse among patients randomly assigned to receive HDC/IL-2 vs. standard-of-care, in particular among patients in their first CR.Citation28 This regimen has not yet been evaluated in patients who have not achieved CR.
A main finding in the present study was that the administration of HDC/IL-2 to patients with AML pronouncedly augmented NK cell counts in the blood. The induction of NK cell counts was noted during each evaluated 21-d cycle and comprised NK cells of the CD16+ and CD56bright phenotypes. Attempts to define the impact of NK cell phenotypes in the blood on LFS and OS revealed that above-median counts of CD56bright NK cells at onset, but not at later time points, predicted a favorable outcome as did treatment-induced accumulation of CD16+ NK cells between the start of cycle 1 and start of cycle 3. The validity of the latter finding was supported by post-hoc analyses of the results of a previous phase III trial, where induction of lymphocytes between cycles 1 and 3 was significantly associated with LFS and OS in patients randomized to receive HDC/IL-2 but not in untreated control patients.Citation39 Since CD56bright NK cells are assumed to be the immediate precursors of CD16+ NK cells,Citation19-22 we hypothesize from these findings that the anti-leukemic efficacy of HDC/IL-2 immunotherapy results, at least in part, from initial activation and expansion of CD56bright NK cells that subsequently differentiate into cytotoxic CD16+ cells. The finding of a significantly increased ratio of CD16+ to CD56bright NK cell counts between the first days of cycles 1 and 3 supports that CD56bright cells may have differentiated into cytotoxic CD16+ cells during immunotherapy.
The expression of NKp30 on subsets of NK cells during treatment cycles largely paralleled the induction of NK cell counts in blood. Thus, the MFI of NKp30 was markedly increased during cycles 1 and 3 on CD16+ NK cells as well as on CD56bright cells, and the NKp30 expression remained modestly elevated on CD56bright NK cells at the onset of cycle 3 when compared with the level of expression at the start of therapy. NKp46 expression was strongly induced on CD16+ cells but not on CD56bright cells and no between-cycles induction of NKp46 was observed in any of the NK cell subtypes. Despite these differences in the dynamics of NCR expression during immunotherapy, a high expression of NKp30 or NK46 on CD16+ NK cells at onset and/or after the first cycle of therapy was positively and significantly associated with LFS and OS. The validity of these findings is bolstered by previous studies showing that newly diagnosed AML patients frequently show deficient expression of NCRs with negative impact on OS.Citation7
In our study, we did not observe significant correlations between outcome and in-cycle induction of NCR expression when analyzing the entire study population, and it thus remains uncertain whether the favorable outcome in patients with high NCR expression was related to the immunotherapy or a reflection of variable baseline NCR expression among patients. However, post-hoc analyses of patients with low NCR expression at onset of therapy supported that treatment-induced NCR expression was associated with a favorable outcome. In this subgroup, induction of NKp46 expression on CD56bright cells during the first treatment cycle was significantly associated with a favorable outcome (Fig. S3).
We propose that the dynamics of NK cell subsets and their NCR expression may contribute to the anti-leukemic efficiency of immunotherapy with HDC/IL-2 in AML. While the exploratory nature of these findings should be emphasized, it is conceivable that AML patients with an intact NCR expression may benefit from relapse-preventive immunotherapy with HDC/IL-2, or other strategies aimed at boosting NK cell function and, also, that NCR expression may be a valid biomarker in future trials evaluating immunotherapy in AML.
Patients and Methods
Patients
Eighty-four patients aged 18 years or older (age 18–79, median 61) with de novo or secondary AML in first CR were eligible for enrollment. In this multicenter study, induction and consolidation courses were given as per each participating center. For all patients, the induction courses included cytarabine, either as continuous infusion 7 d or in intermediate doses (2000–3000 mg bid) for 3–5 d. Anthracyclines used in the induction courses were daunorubicin (n = 77), whereas in 7 cases idarubicin and fludarabine were used. Ten patients received cytarabine with other additional chemotherapy including etoposide (n = 7), amsacrine (n = 1) and ozogamizin (n = 1). Twenty patients achieved CR after two induction courses.
The number of post-remission courses were 1 (n = 15), 2 (n = 20), 3 (n = 37), 4 (n = 5), or 5 (n = 1).Typically, consolidation courses included cytarabine and an anthracycline; daunorubicin (n = 45), idarubicin (n = 10) or mitoxantrone (n = 2). Four patients received cytarabine and other drugs (etoposide,ozogamizin, methotrexate). In 15 patients the consolidation courses comprised high or intermediate doses of cytarabine as the single drug. Six patients did not receive any post-remission therapy, of these 5 had received 2 induction courses to achieve CR. Precise data on consolidation were missing in 2 patients.
The baseline characteristics of participating patients and their impact on LFS are shown in Table S1. Inclusion criteria included adequate renal, cardiac, and pulmonary functions along with a performance status (according to Eastern Cooperative Oncology Group [ECOG] criteria) of 0 to 1. Any previous induction or consolidation therapy, including autologous bone marrow transplantation, was allowed with the exception of allogeneic transplantation (performed or planned). Other exclusion criteria included FAB-M3 AML, class III or class IV cardiac disease, other active malignancies and severe hypersensitivity reactions. Elapsed time from dates of CR and the completion of consolidation chemotherapy were not to exceed 6 and 3 months respectively. The mean time from CR to study entry was 121 d.
Study design and objective
This single-armed multicenter phase IV study (Re:Mission, NCT01347996) enrolled 84 patients at 20 European centers between September 2009 and August 2012. The primary endpoint included assessment of the quantitative and qualitative pharmacodynamic effects of HDC/IL-2 on the immune responses of T and NK cells, including NCR expression. An interim analysis report based on data available on May 21st 2013 was submitted by the study sponsor to the European Medicines Agency (EMA). The analyses of NK cell markers vs. outcome are based on data for LFS available at this date. The trial was approved by the Ethics Committees of each participating institution, and was conducted in accordance with the Helsinki Declaration. All patients gave written informed consent before enrollment.
Treatment and dosing
Patients received 10 consecutive 3-week cycles of HDC/IL-2 with 3 weeks off treatment in cycles 1 to 3, and 6 weeks off treatment in cycles 4 to 10. The treatment continued for a total of 18 months or until the patients relapsed, died, discontinued therapy because of adverse events, withdrew consent, or became lost to follow-up. In each cycle, patients received HDC (0.5 mg; EpiCept Corporation) subcutaneously bid and human recombinant IL-2 (aldesleukin; 16,400 U/kg; Chiron Corporation) subcutaneously bid (). The scheduled total follow-up time was 2 years, and all patients included for the present analyses were followed until the closing date for this analysis.
Definitions and response criteria
Relapse was defined as at least 5% blast cells in the bone marrow or extramedullary leukemia. LFS was defined as the time in days from the first day of treatment with HDC/IL-2 to relapse or death from any cause. OS was defined as corresponding time to death regardless of cause. Risk groups were classified according to recommendations by the European LeukemiaNet.Citation51
Sampling of peripheral blood and flow cytometry
Peripheral blood was collected before and after treatment cycles 1 and 3, i.e. on day 1 and day 21 of cycle 1 (C1D1 and C1D21), and on day 1 and day 21 of cycle 3 (C3D1 and C3D21). The patient blood was collected into BD Vacutainer CPT tubes with sodium citrate (BectonDickinson, Stockholm, Sweden), and within two hours PBMCs were isolated by density centrifugation. The prepared PBMCs were cryopreserved at local sites in CryoMaxx S cryopreservation media (PAA), and shipped on dry ice to the central laboratory for immunological assessment (at the Sahlgrenska Cancer Center, University of Gothenburg, Sweden). The central laboratory operates under Good Clinical Practice (GCP) conditions and passed a GCP-inspection by the EMA in August 2013 (2013/014). In addition it forms part of the Good Laboratory Practice (GLP) accredited laboratories at the Department of Clinical Virology, which is reviewed by the Swedish Board for Accreditation and Conformity Assessment. Samples were available from 79 out of 84 participating patients.
Samples were analyzed flow cytometrically within 30 months of collection, with a median time until analysis of 15 months. The frozen PBMC samples were thawed quickly and washed in warm medium. Samples were first stained with LIVE/DEAD Fixable Yellow Stain (Invitrogen) in PBS. After washing, cells were stained with a cocktail of antibodies in PBS containing 0.5% BSA and 0.1% EDTA. The following anti-human monoclonal antibodies were purchased from BD Biosciences (Stockholm, Sweden): anti-CD3-FITC (clone: HIT3a), CD4-APCH7 (RPA-T4), CD8-PerCpCy5.5 (SK1), CD16-V450 (3G8) CD56-APC (B159). The antibodies to NCRs NKp30-PE (AF29-4D12), NKp46-APC (9E2) were purchased from Miltenyi Biotec. Samples were collected via a 4-laser BD LSRFortessa SORP (405, 488, 532, and 640 nm; BD Biosciences). Data were analyzed with FACSDiva Version 6 software (BD Biosciences) or FlowJo Version 8.4 software (TreeStar). All available samples were analyzed. If an analysis failed according to pre-defined criteria (experimental failure, few cells, below 25% cellular viability), a second sample was thawed for re-analysis. In 18 cases for C1D1 samples and in 12 cases for C1D21 samples, also the second attempt failed to generate data, and these samples were excluded from analysis. Differential counts of whole blood were performed at local sites and were utilized to calculate absolute counts of blood NK cells.
Analyses of lymphocyte counts vs. outcome in a phase III trial
In an open-label, randomized phase III study (NCT00003991), 320 AML patients were enrolled after induction/consolidation therapies and randomly assigned to an HDC/IL-2 arm (with treatment schedule and dosing identical to patients in the Re:Mission Trial) or a control arm (no treatment).Citation28 Lymphocyte counts were captured before treatment as well as before and after treatment cycles. For the present study, the prognostic impact of an increment in lymphocyte counts during therapy was determined by dichotomizing patients by high or low (by the median) lymphocyte counts followed by analysis of LFS and OS in these groups by the logrank test.
Statistics
As specified in the statistical plan, paired t-test was used for single comparisons of NK cell phenotypes. In exploratory analyses the impact of NK cell-related markers on outcome were determined by dichotomizing patients by high or low (by the median) NK cell counts or NCR expression intensity (median fluorescence intensity, MFI) followed by analysis of LFS and OS in these groups by the logrank test. Parameters that significantly predicted LFS and/or OS using the logrank test were further analyzed by univariate and multivariate Cox regression analysis. In the multivariate analyses, hazard ratios were corrected for age and risk group classification (). For practical reasons, the first day of treatment was set to day 0 in all survival analyses, also in those that evaluated immunological status on day 1 in cycle 3. Since the time between first day of treatment and C3D1 is constant for all patients (84 d), this has no consequence for the conducted analyses. All indicated p values are 2-sided.
Disclosure of Potential Conflicts of Interest
Authors KH and MB are past or present consultants to the study sponsor (Meda Pharma). Author KH holds patents protecting the use of histamine dihydrochloride in cancer immunotherapy. Authors AM, RF, and FBT have received honoraria and/or travel grants from the study sponsor. The other authors declare no conflict of interest.
1041701_Supplemental_Material.zip
Download Zip (515.4 KB)Acknowledgments
The authors are indebted to all participants in the Re:Mission Study Group.
Funding
This work was supported by Meda Pharma, Bad Homburg, Germany (Study Sponsor), the Swedish Research Council (2012–2047, 2012–3205, 2011–3003), the Swedish Society for Medical Research (SSMF), the Swedish Society of Medicine (SLS-406151), the Swedish Cancer Society (CAN 212/595, CAN 213/550), the Swedish state via the ALF agreement (ALFGBG-151441, ALFGBG-292701), the Erna and Victor Hasselblad foundation, the Torsten and Ragnar Söderberg Foundation, The IngaBritt and Arne Lundberg Foundation, BioCARE – a National Strategic Research Program at University of Gothenburg, and the Sahlgrenska Academy at University of Gothenburg.
References
- Siegel RL, Miller KD, Jemal A. Cancer statistics, 2015. CA Cancer J Clin 2015; 65:5-29; PMID:25559415; http://dx.doi.org/10.3322/caac.21254
- Breems DA, Van Putten WL, Huijgens PC, Ossenkoppele GJ, Verhoef GE, Verdonck LF, Vellenga E, De Greef GE, Jacky E, Van der Lelie J et al. Prognostic index for adult patients with acute myeloid leukemia in first relapse. J Clin Oncol 2005; 23:1969-78; PMID:15632409; http://dx.doi.org/10.1200/JCO.2005.06.027
- Burnett AK, Goldstone A, Hills RK, Milligan D, Prentice A, Yin J, Wheatley K, Hunter A, Russell N. Curability of patients with acute myeloid leukemia who did not undergo transplantation in first remission. J Clin Oncol 2013; 31:1293-301; PMID:23439754; http://dx.doi.org/10.1200/JCO.2011.40.5977
- Tallman MS, Gilliland DG, Rowe JM. Drug therapy for acute myeloid leukemia. Blood 2005; 106:1154-63; PMID:15870183; http://dx.doi.org/10.1182/blood-2005-01-0178
- Juliusson G, Lazarevic V, Horstedt AS, Hagberg O, Hoglund M, Swedish Acute Leukemia Registry G. Acute myeloid leukemia in the real world: why population-based registries are needed. Blood 2012; 119:3890-9; PMID:22383796; http://dx.doi.org/10.1182/blood-2011-12-379008
- Costello RT, Sivori S, Marcenaro E, Lafage-Pochitaloff M, Mozziconacci MJ, Reviron D, Gastaut JA, Pende D, Olive D, Moretta A. Defective expression and function of natural killer cell-triggering receptors in patients with acute myeloid leukemia. Blood 2002; 99:3661-7; PMID:11986221; http://dx.doi.org/10.1182/blood.V99.10.3661
- Fauriat C, Just-Landi S, Mallet F, Arnoulet C, Sainty D, Olive D, Costello RT. Deficient expression of NCR in NK cells from acute myeloid leukemia: Evolution during leukemia treatment and impact of leukemia cells in NCRdull phenotype induction. Blood 2007; 109:323-30; PMID:16940427; http://dx.doi.org/10.1182/blood-2005-08-027979
- Lauria F, Raspadori D, Rondelli D, Ventura MA, Foa R. In vitro susceptibility of acute leukemia cells to the cytotoxic activity of allogeneic and autologous lymphokine activated killer (LAK) effectors: correlation with the rate and duration of complete remission and with survival. Leukemia 1994; 8:724-8; PMID:8182932
- Orleans-Lindsay JK, Barber LD, Prentice HG, Lowdell MW. Acute myeloid leukaemia cells secrete a soluble factor that inhibits T and NK cell proliferation but not cytolytic function–implications for the adoptive immunotherapy of leukaemia. Clin Exp Immunol 2001; 126:403-11; PMID:11737054; http://dx.doi.org/10.1046/j.1365-2249.2001.01692.x
- Panoskaltsis N, Reid CD, Knight SC. Quantification and cytokine production of circulating lymphoid and myeloid cells in acute myelogenous leukaemia. Leukemia 2003; 17:716-30; PMID:12682629; http://dx.doi.org/10.1038/sj.leu.2402835
- Tajima F, Kawatani T, Endo A, Kawasaki H. Natural killer cell activity and cytokine production as prognostic factors in adult acute leukemia. Leukemia 1996; 10:478-82; PMID:8642865
- Lion E, Willemen Y, Berneman ZN, Van Tendeloo VF, Smits EL. Natural killer cell immune escape in acute myeloid leukemia. Leukemia 2012; 26:2019-26; PMID:22446501; http://dx.doi.org/10.1038/leu.2012.87
- Martner A, Thoren FB, Aurelius J, Hellstrand K. Immunotherapeutic strategies for relapse control in acute myeloid leukemia. Blood Rev 2013; 27:209-16; PMID:23871358; http://dx.doi.org/10.1016/j.blre.2013.06.006
- Sanchez-Correa B, Morgado S, Gayoso I, Bergua JM, Casado JG, Arcos MJ, Bengochea ML, Duran E, Solana R, Tarazona R. Human NK cells in acute myeloid leukaemia patients: analysis of NK cell-activating receptors and their ligands. Cancer Immunol Immunother 2011; 60:1195-205; PMID:21644031; http://dx.doi.org/10.1007/s00262-011-1050-2
- Ruggeri L, Capanni M, Urbani E, Perruccio K, Shlomchik WD, Tosti A, Posati S, Rogaia D, Frassoni F, Aversa F et al. Effectiveness of donor natural killer cell alloreactivity in mismatched hematopoietic transplants. Science 2002; 295:2097-100; PMID:11896281; http://dx.doi.org/10.1126/science.1068440
- Hsu KC, Keever-Taylor CA, Wilton A, Pinto C, Heller G, Arkun K, O'Reilly RJ, Horowitz MM, Dupont B. Improved outcome in HLA-identical sibling hematopoietic stem-cell transplantation for acute myelogenous leukemia predicted by KIR and HLA genotypes. Blood 2005; 105:4878-84; PMID:15731175; http://dx.doi.org/10.1182/blood-2004-12-4825
- Moretta L, Locatelli F, Pende D, Marcenaro E, Mingari MC, Moretta A. Killer Ig-like receptor-mediated control of natural killer cell alloreactivity in haploidentical hematopoietic stem cell transplantation. Blood 2011; 117:764-71; PMID:20889923; http://dx.doi.org/10.1182/blood-2010-08-264085
- Nagler A, Lanier LL, Cwirla S, Phillips JH. Comparative studies of human FcRIII-positive and negative natural killer cells. J Immunol 1989; 143:3183-91; PMID:2530273
- Caligiuri MA. Human natural killer cells. Blood 2008; 112:461-9; PMID:18650461; http://dx.doi.org/10.1182/blood-2007-09-077438
- Chan A, Hong DL, Atzberger A, Kollnberger S, Filer AD, Buckley CD, McMichael A, Enver T, Bowness P. CD56bright human NK cells differentiate into CD56dim cells: role of contact with peripheral fibroblasts. J Immunol 2007; 179:89-94; PMID:17579025; http://dx.doi.org/10.4049/jimmunol.179.1.89
- Poli A, Michel T, Theresine M, Andres E, Hentges F, Zimmer J. CD56bright natural killer (NK) cells: an important NK cell subset. Immunology 2009; 126:458-65; PMID:19278419; http://dx.doi.org/10.1111/j.1365-2567.2008.03027.x
- Romagnani C, Juelke K, Falco M, Morandi B, D'Agostino A, Costa R, Ratto G, Forte G, Carrega P, Lui G et al. CD56brightCD16- killer Ig-like receptor- NK cells display longer telomeres and acquire features of CD56dim NK cells upon activation. J Immunol 2007; 178:4947-55; PMID:17404276; http://dx.doi.org/10.4049/jimmunol.178.8.4947
- Babor F, Fischer JC, Uhrberg M. The role of KIR genes and ligands in leukemia surveillance. Frontiers in immunology 2013; 4:27; PMID:23404428; http://dx.doi.org/10.3389/fimmu.2013.00027
- Lanier LL. NK cell recognition. Annu Rev Immunol 2005; 23:225-74; PMID:15771571; http://dx.doi.org/10.1146/annurev.immunol.23.021704.115526
- Long EO, Kim HS, Liu D, Peterson ME, Rajagopalan S. Controlling natural killer cell responses: integration of signals for activation and inhibition. Annu Rev Immunol 2013; 31:227-58; PMID:23516982; http://dx.doi.org/10.1146/annurev-immunol-020711-075005
- Moretta A, Bottino C, Vitale M, Pende D, Cantoni C, Mingari MC, Biassoni R, Moretta L. Activating receptors and coreceptors involved in human natural killer cell-mediated cytolysis. Annu Rev Immunol 2001; 19:197-223; PMID:11244035; http://dx.doi.org/10.1146/annurev.immunol.19.1.197
- Geller MA, Miller JS. Use of allogeneic NK cells for cancer immunotherapy. Immunotherapy 2011; 3:1445-59; PMID:22091681; http://dx.doi.org/10.2217/imt.11.131
- Brune M, Castaigne S, Catalano J, Gehlsen K, Ho AD, Hofmann WK, Hogge DE, Nilsson B, Or R, Romero AI et al. Improved leukemia-free survival after postconsolidation immunotherapy with histamine dihydrochloride and interleukin-2 in acute myeloid leukemia: results of a randomized phase 3 trial. Blood 2006; 108:88-96; PMID:16556892; http://dx.doi.org/10.1182/blood-2005-10-4073
- Aurelius J, Martner A, Brune M, Palmqvist L, Hansson M, Hellstrand K, Thoren FB. Remission maintenance in acute myeloid leukemia: impact of functional histamine H2 receptors expressed by leukemic cells. Haematologica 2012; 97:1904-8; PMID:22689678; http://dx.doi.org/10.3324/haematol.2012.066399
- Aurelius J, Thoren FB, Akhiani AA, Brune M, Palmqvist L, Hansson M, Hellstrand K, Martner A. Monocytic AML cells inactivate antileukemic lymphocytes: role of NADPH oxidase/gp91(phox) expression and the PARP-1/PAR pathway of apoptosis. Blood 2012; 119:5832-7; PMID:22550344; http://dx.doi.org/10.1182/blood-2011-11-391722
- Hellstrand K, Asea A, Dahlgren C, Hermodsson S. Histaminergic regulation of NK cells. Role of monocyte-derived reactive oxygen metabolites. J Immunol 1994; 153:4940-7; PMID:7963557
- Hellstrand K, Hermodsson S. Histamine H2-receptor-mediated regulation of human natural killer cell activity. J Immunol 1986; 137:656-60; PMID:3722819
- Hellstrand K, Asea A, Hermodsson S. Role of histamine in natural killer cell-mediated resistance against tumor cells. J Immunol 1990; 145:4365-70; PMID:2147942
- Thoren FB, Aurelius J, Martner A. Antitumor properties of histamine in vivo. Nature medicine 2011; 17:537; PMID:21546964; http://dx.doi.org/10.1038/nm0511-537a
- Buyse M, Squifflet P, Lucchesi KJ, Brune ML, Castaigne S, Rowe JM. Assessment of the consistency and robustness of results from a multicenter trial of remission maintenance therapy for acute myeloid leukemia. Trials 2011; 12:86; PMID:21429214; http://dx.doi.org/10.1186/1745-6215-12-86
- Berry SM, Broglio KR, Berry DA. Addressing the incremental benefit of histamine dihydrochloride when added to interleukin-2 in treating acute myeloid leukemia: a Bayesian meta-analysis. Cancer Invest 2011; 29:293-9; PMID:21469978; http://dx.doi.org/10.3109/07357907.2011.568563
- Dauguet N, Recher C, Demur C, Fournie JJ, Poupot M, Poupot R. Pre-eminence and persistence of immature natural killer cells in acute myeloid leukemia patients in first complete remission. Am J Hematol 2011; 86:209-13; PMID:21264910; http://dx.doi.org/10.1002/ajh.21906
- Lichtenegger FS, Lorenz R, Gellhaus K, Hiddemann W, Beck B, Subklewe M. Impaired NK cells and increased T regulatory cell numbers during cytotoxic maintenance therapy in AML. Leuk Res 2014; 38:964-9; PMID:24957413; http://dx.doi.org/10.1016/j.leukres.2014.05.014
- Hallner A, Aurelius J, Thoren FB, Sander FE, Brune M, Hellstrand K, Martner A. Immunotherapy with histamine dihydrochloride and low-dose interleukin-2 favors sustained lymphocyte recovery in acute myeloid leukemia. Eur J Haematol 2015; 94:279-80; PMID:25256820; http://dx.doi.org/10.1111/ejh.12454
- Baer MR, George SL, Caligiuri MA, Sanford BL, Bothun SM, Mrozek K, Kolitz JE, Powell BL, Moore JO, Stone RM et al. Low-dose interleukin-2 immunotherapy does not improve outcome of patients age 60 years and older with acute myeloid leukemia in first complete remission: Cancer and Leukemia Group B Study 9720. J Clin Oncol 2008; 26:4934-9; PMID:18591543; http://dx.doi.org/10.1200/JCO.2008.17.0472
- Blaise D, Attal M, Reiffers J, Michallet M, Bellanger C, Pico JL, Stoppa AM, Payen C, Marit G, Bouabdallah R et al. Randomized study of recombinant interleukin-2 after autologous bone marrow transplantation for acute leukemia in first complete remission. Eur Cytokine Netw 2000; 11:91-8; PMID:10705305
- Kolitz JE, George SL, Benson DM, Jr., Maharry K, Marcucci G, Vij R, et al. Recombinant interleukin-2 in patients aged younger than 60 years with acute myeloid leukemia in first complete remission: results from Cancer and Leukemia Group B 19808. Cancer 2014; 120:1010-7. PMID:24382782; http://dx.doi.org/10.1002/cncr.28516
- Lange BJ, Smith FO, Feusner J, Barnard DR, Dinndorf P, Feig S, Heerema NA, Arndt C, Arceci RJ, Seibel N et al. Outcomes in CCG-2961, a children's oncology group phase 3 trial for untreated pediatric acute myeloid leukemia: a report from the children's oncology group. Blood 2008; 111:1044-53; PMID:18000167; http://dx.doi.org/10.1182/blood-2007-04-084293
- Lotzova E, Savary CA, Herberman RB. Induction of NK cell activity against fresh human leukemia in culture with interleukin 2. J Immunol 1987; 138:2718-27; PMID:3494084
- Pautas C, Merabet F, Thomas X, Raffoux E, Gardin C, Corm S, Bourhis JH, Reman O, Turlure P, Contentin N et al. Randomized study of intensified anthracycline doses for induction and recombinant interleukin-2 for maintenance in patients with acute myeloid leukemia age 50 to 70 years: results of the ALFA-9801 study. J Clin Oncol 2010; 28:808-14; PMID:20048183; http://dx.doi.org/10.1200/JCO.2009.23.2652
- Buchner T, Urbanitz D, Hiddemann W, Ruhl H, Ludwig WD, Fischer J, Aul HC, Vaupel HA, Kuse R, Zeile G. Intensified induction and consolidation with or without maintenance chemotherapy for acute myeloid leukemia (AML): two multicenter studies of the German AML Cooperative Group. J Clin Oncol 1985; 3:1583-9; PMID:3906048
- Gabrilovich DI, Nagaraj S. Myeloid-derived suppressor cells as regulators of the immune system. Nat Rev Immunol 2009; 9:162-74; PMID:19197294; http://dx.doi.org/10.1038/nri2506
- Hellstrand K, Hermodsson S. Synergistic activation of human natural killer cell cytotoxicity by histamine and interleukin-2. Int Arch Allergy Appl Immunol 1990; 92:379-89; PMID:2150668; http://dx.doi.org/10.1159/000235169
- Donskov F, Middleton M, Fode K, Meldgaard P, Mansoor W, Lawrance J, Thatcher N, Nellemann H, von der Maase H. Two randomised phase II trials of subcutaneous interleukin-2 and histamine dihydrochloride in patients with metastatic renal cell carcinoma. Br J Cancer 2005; 93:757-62; PMID:16136045; http://dx.doi.org/10.1038/sj.bjc.6602768
- Agarwala SS, Glaspy J, O'Day SJ, Mitchell M, Gutheil J, Whitman E, Gonzalez R, Hersh E, Feun L, Belt R et al. Results from a randomized phase III study comparing combined treatment with histamine dihydrochloride plus interleukin-2 versus interleukin-2 alone in patients with metastatic melanoma. J Clin Oncol 2002; 20:125-33; PMID:11773161; http://dx.doi.org/10.1200/JCO.20.1.125
- Dohner H, Estey EH, Amadori S, Appelbaum FR, Buchner T, Burnett AK, Dombret H, Fenaux P, Grimwade D, Larson RA et al. Diagnosis and management of acute myeloid leukemia in adults: recommendations from an international expert panel, on behalf of the European LeukemiaNet. Blood 2010; 115:453-74; PMID:19880497; http://dx.doi.org/10.1182/blood-2009-07-235358