ABSTRACT
Malignant melanoma is characterized by a rapid progression, metastasis to distant organs and resistance to chemo and radiotherapy. Although melanoma is capable of eliciting an immune response, the disease progresses and the overall results of immunotherapeutic clinical studies are not satisfactory. Recently, we have developed a novel genetic platform for improving an induction of peptide-specific CD8+ T cells by dendritic cell (DC) based on membrane-anchored β2-microglobulin (β2m) linked to a selected antigenic peptide at the N-terminus and to the cytosolic domain of TLR4 at the C-terminus. In vitro transcribed mRNA transfection of antigen-presenting cells (APCs) resulted in an efficient coupling of peptide presentation and cell activation. In this research, we utilize the chimeric platform to induce an immune response in ret transgenic mice that spontaneously develop malignant skin melanoma and to examine its effect on the overall survival of tumor-bearing mice. Following immunization with chimeric construct system, we observe a significantly prolonged survival of tumor-bearing mice as compared to the control group. Moreover, we see elevations in the frequency of CD62LhiCD44hi central and CD62LloCD44hi effector memory CD8+ T-cell subsets. Importantly, we do not observe any changes in frequencies of regulatory T cells (Tregs) and myeloid-derived suppressor cells (MDSCs) in the vaccinated groups. Our data suggest that this novel vaccination approach could be efficiently applied for the immunotherapy of malignant melanoma.
Introduction
The incidence of melanoma cases is increasing worldwide and despite early detection, appropriate surgical resection and adjuvant therapy, the number of patients dying from metastatic disease continues to rise.Citation1-3 The prognosis of advanced melanoma remains to be poor and the treatment options for metastatic disease are limited.Citation1-3 After the standard therapy of stage III and IV melanoma, clinically disease-free patients have a 50–90% risk of recurrence. Despite recent therapeutic advances in metastatic melanoma dealing with the application of inhibitors targeting the mutated signaling molecule BRAF as well as antibodies blocking negative checkpoint CTLA-4 and PD-1,Citation4-6 many treated patients do not respond to these treatments indicating that new approaches for melanoma immunotherapy are urgently needed.
It is well established that spontaneous anti-melanoma immune responses are directed in part against melanocyte differentiation antigens (e.g., gp100, tyrosinase (Tyr), tyrosinase-related protein-1 (TRP-1), TRP-2, MelanA).Citation7 There are several therapeutic strategies aiming at enhancing the cellular antitumor immunity against these specific melanoma-associated antigens (MAAs). One such strategy is to use autologous DCs loaded with MAAs.Citation8
DCs form a network of APCs that shape immune responses by linking innate and adaptive immunity.Citation9 Proliferation, activation and differentiation of specific T-cell subsets are influenced by DCs. This makes DCs an attractive instrument for cancer immunotherapies.Citation10,11 Antitumor therapeutic vaccines should be able to prime naive T cells, but most importantly, to induce the transition of existing memory T cells from non-protective to potent effector CD8+ T cells.Citation11
However, the clinical impact of DC immunotherapy has been limited so far despite the induction of tumor-specific T-cell responses in many patients and occasional tumor regressions. Many reasons may explain this lack of success with DC vaccines. DC-based immunotherapies require optimization at several levels: (i) the maturation stimuli in use; (ii) the type and form of antigen to be loaded on DCs; (iii) the origin, subset and the number of DCs to inject; (iv) the amount, frequency, route and the site of injections.Citation12,13
In addition to the limitations of DCs immunotherapy, numerous immunosuppressive cells and factors strongly contribute to the impairment of the antitumor immune response leading to the tumor progression. Among them are MDSCs, which represent a heterogeneous population of immature myeloid cells that could severely impair antitumor activities of T cells.Citation14-16 Tregs are considered to be pivotal mediators of peripheral tolerance and immune suppression. However, they are highly enriched in the tumor microenvironment and are considered to be important for limiting antitumor T-cell-mediated immune responses.Citation17,18
We have earlier developed a novel genetic platform for the induction of antigen-specific effector CD8+ T cells by DCs vaccination using the MHC-I light chain, β2m.Citation9 To this end, we have converted β2m into an integral membrane protein by linking antigenic peptides to its N-terminus and the intracellular toll-like receptor (TLR4) signaling domain to its C-terminus. We showed that efficient peptide presentation can be coupled to constitutive TLR4 signaling through the polypeptide product of a single gene, and that this dual effect can be achieved by virtue of mRNA electroporation.Citation19 We also showed that these constructs can mediate an effective antitumor activity in the transplantable B16 melanoma model.Citation20
In this study, we utilize this chimeric mRNA construct system to examine whether DCs immunization will affect melanoma progression in a spontaneous ret transgenic mouse melanoma model that closely resembles human melanoma regarding histopathology and clinical development.Citation21,22 In addition, primary skin melanomas and metastases in lymph nodes (LNs) and distant organs of transgenic mice express similar MAAs to those expressed in human melanoma and B16 tumors (such as gp100, Tyr, TRP-1 and TRP-2).Citation22,23
Our data suggest that immunotherapy with DCs-based antitumor vaccine can significantly improve the survival of tumor-bearing ret transgenic mice. Analyzing the mechanism of such effect, we observed elevated frequencies of central and effector memory T-cell subsets in vaccinated mice. Interestingly, we found a significant survival improvement only in the group of mice, which was immunized with a vaccine composed of mRNA mixture of the two MAAs, gp100 and TRP-2, indicating that an aggressive disease may require more vaccine combinations. Moreover, in order to enhance the vaccine efficacy, we should consider applying Tregs and MDSCs inhibitors in the treatment protocol.
Materials and methods
Mice
C57BL/6 mice expressing human ret transgene in melanocytes under the control of mouse metallothionein-I promoter–enhancerCitation21 were provided by Dr I. Nakashima (Chubu University, Aichi, Japan). Animals were crossed and kept under specific pathogen-free conditions in the animal facility of German Cancer Research Center (Heidelberg, Germany). Experiments were performed in accordance with government and institutional guidelines and regulations.
Antibodies
The anti-mouse APC-CD11b, APC-CD62L, APC-CD25, PE-CD44, FITC-CD45RB, FITC-CD3, Pe-Cy7-Gr1, Pe-Cy7-CD4, PerCp-Cy5.5-CD45.2 and PerCp-Cy5.5-CD3 were purchased from BD Biosciences (San Jose, CA). The anti-mouse PE-F4/80, PE-FoxP3 and FITC-MHC-II were purchased from eBioscience (San Diego, CA). The anti-mouse APC-Cy7-CD11c and APC-Cy7-CD8 and the PE-conjugated anti-human β2 m antibody were purchased from Biolegend (San Diego, CA).
Generation of DCs from murine bone-marrow cells
Murine bone marrow derived DCs (BMDCs) were performed as described by Lutz et al.Citation24 with minor modifications. Briefly, bone-marrow (BM) cells from femurs and tibiae of 4–6 weeks old C57BL/6, female mice were cultured in DC medium containing RPMI (GibcoBRL, Grand Island, NY), supplemented with 10% FBS (Hyclone, Logan, UT), 10 mM HEPES, 2 mM L-glutamine, 50 mM 2-mercaptoethanol (2-ME), gentamicin and 200 U/mL recombinant mouse (rm)GM-CSF (Prospec, Rehovot, Israel). On day 8, non-adherent cells were harvested and further cultured in fresh medium containing 100 U/mL rmGM-CSF for 24 h. Non-adherent cells were analyzed by flow cytometry for the expression of CD11c, CD80, CD86, Gr1, F4/80, CD11b and MHC class II, followed by mRNA electroporation procedure.
mRNA constructs assembly and expression
The β2m-TLR4 and β2m-Kb backbones were covalently linked to the tumor-associated peptides as previously described.Citation19 Human gp10025–33 or mouse TRP-2180–188 peptides were used.
In vitro mRNA transcription
Template DNA cloned in the pGEM4Z-A64 vector was prepared with the EndoFree Plasmid Maxi Kit (Qiagen, Valencia, CA) and linearized via the SpeI restriction site positioned at the 3′ end of the poly (A) tract of the vector. 1 μg of linear plasmid was used for in vitro mRNA transcription with AmpliCap-Max T7 High Yield Message Maker Kit (CellScript, Biotechnologies, Madison, WI). The concentration and quality of the mRNA was assessed by spectrophotometry.
mRNA electroporation
Procedure was performed as previously described by Cafri et al.Citation20 Shortly, BMDCs cultured for 8 d, were washed twice with OptiMEM medium (GibcoBRL) and resuspended in 150 μL OptiMEM medium containing 20 μg transcribed mRNA and electroporated using BTX ECM 830 electroporator (BTX, Holliston, MA) 400 V, 0.9 ms, one pulse, using a 2 mm cuvette. Cells were resuspended in 5 mL growth medium and transferred into tissue culture plates for further incubation. Constructs expression was assessed by flow cytometry 6 h after electroporation.
Immunizations
BMDCs were electroporated with 10–20 μg of transcribed mRNA (5 μg of each construct according to the vaccinated group) followed by 6 h incubations at 37°C. Cells were washed three times and resuspended to 2.5 × 106/mL in PBS. In all experiments, mice were vaccinated i.p. with 200 μL cell suspension (0.5 × 106 cells per mouse) three times weekly.
Flow cytometry analysis
Mice were sacrificed 10 d after the last vaccination. Cell suspensions from spleens, BM, LNs and skin tumor were prepared and adjusted to 1 × 106 cells/100 μL in staining buffer (PBS with 0.5% BSA and 0.1% sodium azide). Fluorochrome-conjugated antibodies were added for 30 min at 4°C in the dark. Acquisition was performed by six-color flow cytometry using FACSCanto II with FACSDiva software (both from BD Biosciences). The compensation control was performed with BD CompBeads set (BD Biosciences) using the manufacturer's instruction. FlowJo software (Tree Star, Ashland, OR) was used to analyze at least 100,000 events. Data were expressed as dot plots.
Statistical analysis
Statistical analysis of cell surface markers and Tregs was conducted with one-way ANOVA. Significance of the differences was assessed by Dunnett's multiple comparison posttest. Survival curves were generated using the product limit (Kaplan–Meier) method and comparisons were conducted using the log-rank (Mentel–Cox) test. A value of p < 0.05 was considered statistically significant.
Results
We have recently described the development and assessment of recombinant bi-functional β2m-based polypeptides, which couple MHC-I presentation to constitutive TLR4 activation.Citation19 To test whether these polypeptides confer antitumor activity in a spontaneous melanoma model, we have used ret transgenic mice. We have applied both the Kb and TLR4 anchors covalently linked to either hgp10025–33 or TRP-2180–188 antigens. The use of both anchors, and not only one, was due to our previous data showing that although the Kb anchored peptides are more stable on the cell surface, the TLR4 anchor is important for transmitting DC maturation signals.Citation20 We utilize the recombinant bi-functional β2m-based polypeptides system for the immunization of melanoma-bearing mice. The vaccination by a single peptide (derived from gp100 or TRP-2) with both anchors (Kb and TLR4) is designated as a double construct. Composition of the different combinations used in the experiment and their designations are shown in .
Figure 1. Scheme of the β2m-based bi-functional constructs. (A) Genetic design. The sites of promoter (pr), leader peptide (lead), antigenic peptide (p), linker peptide (li) and bridge (br) are shown. (B) Anticipated configuration of the polypeptide products with a linked antigenic peptide in the context of an MHC-I heavy (α) chain. (C) Designations of the different constructs generated and used for immunizations in this study.
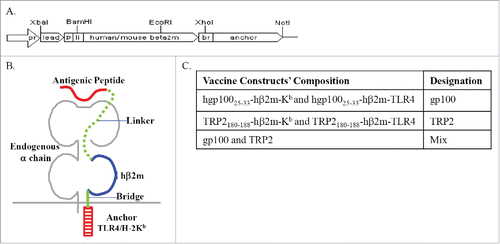
For every type of construct, 5 μg of mRNA were electroporated into BMDCs, resulting in total of 10 μg mRNA in the gp100 and TRP-2 groups and 20 μg in the quadrate constructs (Mix) group. Although a trend for improved survival was observed in the group treated with gp100 containing double constructs, immunization with a double construct of a single peptide did not significantly increase the survival of mice (). However, the use of the constructs mixture containing gp100-Kb, gp100-TRL4, TRP-2-Kb and TRP-2-TLR4 (quadrate constructs) in the Mix group significantly improved survival and prolonged life expectancy of mice up to day 55 (p <0.05; ).
Figure 2. Prolonged survival in mice vaccinated with Mix mRNA constructs. Melanoma-bearing ret transgenic mice were vaccinated three times at weekly intervals with BMDCs electroporated with 10–20 μg transcribed mRNA of gp100 (n = 8), TRP-2 (n = 8), Mix constructs combinations (n = 20) or empty construct (Control group, n = 20). Survival was monitored up to day 55. Presented are results obtained from two consecutive experiments. *p = 0.0454 Mix vs. control group.
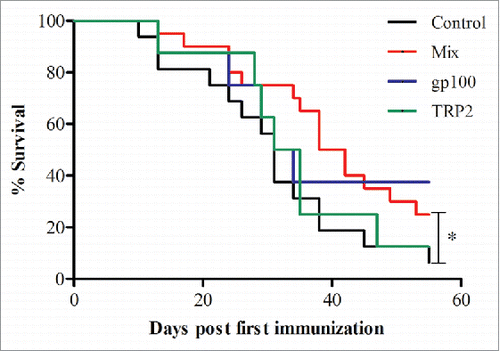
Next, we investigated the changes in the immune cells that could be involved in the survival benefit of melanoma-bearing mice upon the vaccination. We have collected tissues of BM, metastatic LNs, spleen and skin tumors of treated groups 10 d after the last vaccination and performed a phenotypic analysis of T lymphocytes. As shown in , the frequency of CD8+CD62LhiCD44hi central memory T cells in the BM and spleens from mice of the Mix group was significantly increased as compared to the control group (p <0.05). Moreover, a significant increase in the frequency of CD8+CD62LloCD44hi effector memory T cells was demonstrated in the BM from mice of the Mix group as well as in primary skin tumors and metastatic LN from mice treated with the gp100 construct as compared to the control group (p <0.05; ).
Figure 3. Phenotypic analysis of CD8 memory T cell subsets following vaccination with mRNA electroporated DCs. Melanoma-bearing ret transgenic mice were vaccinated three times at weekly intervals with BMDCs electroporated with 10–20 μg transcribed mRNA of gp100 (n = 5), TRP-2 (n = 6), Mix construct combinations (n = 12) or empty construct (Control group, n = 12). 10 d after the last vaccination melanoma lesions (skin tumors and metastatic LN), BM, and spleens were analyzed by flow cytometry. CD8+ CD62L+CD44hi central memory (A) and CD8+ CD62L-CD44hi effector memory (B) T cells were shown as the percentage of respective cells among total CD8+ T cells. *p <0.05, **p <0.01, ***p <0.001.
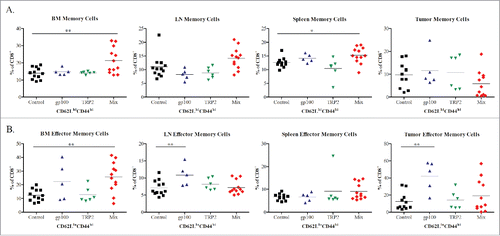
To characterize central and effector memory CD4+ T-cell subsets, we have used CD44 and CD62L markers similar to CD8+ T cells.Citation25 We have found significantly elevated frequencies of CD4+CD62LhiCD44hi central memory T cells in the LN of the control compared to the gp100 group (p <0.05; ). In the spleen, we detected significantly elevated levels of CD4+ memory cells in the control group as compared to all other groups. Analyzing CD4+CD62LloCD44hi effector memory T cells, we observed their enhanced frequencies in the tumor from mice treated with gp100 construct as well as in the BM from mice of Mix group as compared to the control group (p <0.05; ).
Figure 4. Phenotypic analysis of CD4+ memory T cell subsets following vaccination with mRNA electroporated DCs. Melanoma-bearing ret transgenic mice were vaccinated three times at weekly intervals with BMDCs electroporated with 10–20 μg transcribed mRNA of gp100 (n = 5), TRP-2 (n = 6), Mix construct combinations (n = 12) or empty construct (Control group, n = 12). 10 d after the last vaccination melanoma lesions (skin tumors and metastatic LN), BM, and spleens were analyzed by flow cytometry. CD4+ CD62L+CD44hi central memory (A) and CD4+ CD62L-CD44hi effector memory (B) T cells were shown as the percentage of respective cells among total CD4+ T cells. *p <0.05, **p <0.01, ***p <0.001.
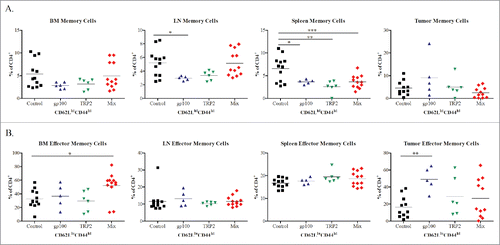
Next, we investigated the effect of vaccination with different constructs on the frequency of two major immunosuppressive cell populations, i.e., CD4+CD25+FoxP3+ Tregs and CD11b+Gr1+ MDSCs. Studying Tregs, we demonstrated a tendency to the decrease in the BM from Mix group as compared to that in control group (). A significant downregulation of Treg frequencies was observed in metastatic LN from the TRP-2 group (p <0.05), whereas skin tumors of these mice displayed no statistically significant changes in Tregs as compared to the control group (). Furthermore, the vaccination did not induce any changes in MDSC in melanoma lesions and lymphoid organs as compared to non-treated mice ().
Figure 5. Phenotypic analysis of Tregs and MDSCs populations following vaccination with mRNA electroporated DCs. Melanoma-bearing ret transgenic mice were vaccinated three times at weekly intervals with BMDCs electroporated with 10–20 μg transcribed mRNA of gp100 (n = 5), TRP-2 (n = 6), Mix construct combinations (n = 12) or empty construct (control group, n = 12). 10 d after the last vaccination melanoma lesions (skin tumors and metastatic LN), BM, and spleens were analyzed by flow cytometry. CD4+CD25+FoxP3+ Tregs (A) and CD11b+Gr1+ MDSCs (B) were shown as the percentage of CD4+ and total monocytes respectively. *p <0.05, **p <0.01, ***p < 0.001.
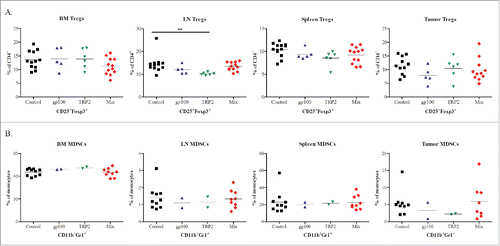
Discussion
We have previously shown in the transplantable B16 melanoma model that the peptide-hβ2m-TLR4 constructs were expressed at the cell surface of BMDCs and prolonged effective peptide presentation up to at least 3 d post-mRNA electroporation.Citation20 Moreover, we observed an increased antitumor immune reactivity indicated by an elevated cytokine production by effector CD8+ T cells and their enhanced cytotoxic activity in in vivo killing assay as compared to peptide-loaded mature BMDCs.Citation20 This system allows continuous peptide presentation and improves the prospects of peptide-bearing DCs to enter the LNs and encounter peptide-specific naive T cells. Moreover, by using mRNA rather than cDNA as gene carrier, we can prevent the development of long-term toxic effects arising from continuous TLR4 activation.Citation19,20
However, the ability of a transplantable model system to adequately predict therapeutic responses in human patients is limited. In this study, we attempt to utilize our mRNA constructs system in ret transgenic mice that develop spontaneously skin tumors and metastases in the BM, lungs, liver and brain.Citation21,22 Moreover, melanoma lesions expressed several MAAs such as tyrosinase, gp100, TRP-1 and TRP-2. It has been also reported that ret transgenic mice could develop specific T-cell responses against TRP-2 upon vaccination.Citation21,22
Here, ret transgenic mice with clinically visible tumors were vaccinated with DCs electroporated with mRNAs constructs for gp100 and TRP-2. We demonstrated that an immunotherapy with a double mix anchor (Kb and TLR4), for a single antigen (gp100 or TRP-2) induced some increase in mouse survival. However, the application of mRNA containing a double mix anchor and both antigens, quadrate constructs (Mix), significantly improved survival of tumor-bearing mice.
We have previously characterized the different constructs used in this study and performed phenotypic analysis of healthy immunized naive C57BL/6 mice.Citation20 We tested the activation status of T lymphocytes, by the expression of CD44 and CD62L on cell surface. We have shown that vaccination with quadrate constructs Mix induced elevation in the effector-memory CD8+ T-cell population and decrease in the total number of memory cells. We have also analyzed Tregs frequency cells where no statistically significant differences were seen among all treatments.Citation20
Here, we analyzed the effect of vaccination on different immune cell subsets in melanoma lesions (skin tumors and metastatic LN) as well as lymphatic organs (spleen and BM) from ret transgenic mice. We observed an elevated frequency of CD8+CD62LhiCD44hi central memory and CD8+CD62LloCD44hi effector memory T cells in the Mix group as compared to the control group. CD8+ effector memory T cells were also accumulated in mice vaccinated with gp100 construct as compared to the control. These data are consistent with our previous results, showing that the Mix treatment, expressing the two antigens, induced the most effective CD8+ response in a B16 transplantable melanoma model owing to its dual functionality, which allowed long-lasting peptide presentation through Kb in conjunction with DCs maturation driven by TLR4.Citation20
Studying CD4+ memory T cells, we observed elevated levels of CD4+CD62LhiCD44hi central memory cells in the control group as compared to the gp100 group in the LNs and elevated levels of these cells in the control group as compared to all other groups in the spleen. This might be explained by the fact that the contribution of CD4+ T cells to antitumor immunity is contradictory due to their heterogeneity.Citation26 For instance, it was described that IL-4 cytokine, secreted by CD4+ T cells, may exert antitumor effect;Citation27 however, the frequency of antigen-specific CD4+ cells that produce IL-5 has been correlated with progressive growth of melanoma.Citation28 Further investigation of different CD4+ T-cell subsets are required to determine their function and contribution to the tumor progression.
Studying CD4+ effector memory T cells, we found an increase in its frequency in the tumor tissue from mice treated with gp100 construct as well as in the BM of Mix group as compared to the control group. This CD4+ effector memory T-cell subpopulation should be also further analyzed to determine its contribution to the tumor regression.
Tregs have been accepted to play a major role in maintenance of self-tolerance in healthy hosts.Citation29 Moreover, these cells may hamper an effective antitumor immune response in cancer patients and tumor-bearing mice.Citation30,31 Therefore, we monitored the Treg frequency in melanoma lesions and lymphatic organs. Importantly, we found no significant alterations of these immunosuppressive cells, which was in agreement with our previous findings obtained with the same construct in transplantable B16 melanoma model.Citation20
Another crucial immunosuppressive cell population is represented by MDSCs that inhibit antitumor T-cell responses by multiple mechanisms and can be considered as one of the most important components of tumor-induced immunosuppression in melanoma.Citation14,15,32,33 It has been previously described that MDSCs accumulate in peripheral lymphoid organs and migrate to tumor sites, where they contribute to immunosuppression.Citation15,16,33,34 Furthermore, some evidence suggests that MDSCs can induce an expansion of Tregs.Citation35 In addition, a transient elevation in MDSCs in mice following immunization was demonstrated.Citation36,37 Previous studies performed on ret transgenic tumor-bearing mice demonstrated that targeting of MDSC by the phosphodiesterase-5 inhibitor sildenafil or ultra-low dose paclitaxel significantly increased animal survival associated with the restoration of antitumor T-cell functions.Citation23,38 On the other side, it has been reported that, T cells may promote melanoma progression in this mouse model by favoring pro-tumoral properties of tumor-infiltrating myeloid cells that further inhibit functions of immune effector cells.Citation39 In this study, we found no statistically significant changes in MDSC frequencies in melanoma lesions and lymphoid organ upon vaccination with modified DCs, indicating that this immunization does not display side effects dealing with the MDSC accumulation. However, the immunosuppressive activity of these cells has to be monitored in further experiments.
In summary, we have demonstrated that DC vaccination can improve the survival of melanoma-bearing ret transgenic mice and induce an increase in frequencies of CD8+ effector and central memory T cells without any stimulatory effect on immunosuppressive Tregs and MDSCs. Together with our previous data on the stimulation of antitumor activities based on mRNA electroporated DCs,Citation20 our findings demonstrate that this vaccine approach can be efficiently applied for the melanoma immunotherapy. We suggest that the efficiency of DC vaccination could be significantly improved by combining it with targeting of MDSCs and Tregs to neutralize immunosuppressive tumor microenvironment.
Disclosure of potential conflicts of interest
No potential conflicts of interest were disclosed.
Acknowledgment
We thank K. Frank for excellent technical assistance.
References
- Griewank KG, Scolyer RA, Thompson JF, Flaherty KT, Schadendorf D, Murali R. Genetic alterations and personalized medicine in melanoma: Progress and future prospects. JNCI J Natl Cancer Inst 2014; 106:djt435; PMID:24511108; http://dx.doi.org/10.1093/jnci/djt435
- Eggermont AM, Spatz A, Robert C. Cutaneous melanoma. Lancet 2014; 383:816-27; PMID:2405-4424; http://dx.doi.org/10.1016/S0140-6736(13)60802-8
- Gogas H, Polyzos A, Kirkwood J. Immunotherapy for advanced melanoma: Fulfilling the promise. Cancer Treat Rev 2013; 39:879-85; PMID:23725878; http://dx.doi.org/10.1016/j.ctrv.2013.04.006
- Schadendorf D, Hodi FS, Robert C, Weber JS, Margolin K, Hamid O, Patt D, Chen TT, Berman DM, Wolchok JD. Pooled analysis of long-term survival data from phase II and phase III trials of ipilimumab in unresectable or metastatic melanoma. J Clin Oncol 2015; 33:1889-94; PMID:25667295; http://dx.doi.org/10.1200/JCO.2014.56.2736
- Robert C, Schachter J, Long G V, Arance A, Grob JJ, Mortier L, Daud A, Carlino MS, McNeil C, Lotem M, et al. Pembrolizumab versus ipilimumab in advanced melanoma. N Engl J Med 2015; 372:2521-32; PMID:25891173; http://dx.doi.org/10.1056/NEJMoa1503093
- Hodi FS1, O'Day SJ, McDermott DF, Weber RW, Sosman JA, Haanen JB, Gonzalez R, Robert C, Schadendorf D, Hassel JC, Akerley W, van den Eertwegh AJ, Lutzky J, Lorigan P, Vaubel JM, Linette GP, Hogg D, Ottensmeier CH, Lebbé C, Peschel C, Quirt I, Clark JI, Wolchok JD, Weber JS, Tian J, Yellin MJ, Nichol GM, Hoos A, Urba WJ. Improved survival with ipilimumab in patients with metastatic melanoma. N Engl J Med 2010; 363:711-723; PMID:20525992; http://dx.doi.org/10.1056/NEJMoa1003466
- Ramirez-Montagut T, Turk MJ, Wolchok JD, Guevara-Patino JA, Houghton AN. Immunity to melanoma: unraveling the relation of tumor immunity and autoimmunity. Oncogene 2003; 22:3180-7; PMID:12789294; http://dx.doi.org/10.1038/sj.onc.1206462
- Wilgenhof S, Corthals J, Van Nuffel AMT, Benteyn D, Heirman C, Bonehill A, Thielemans K, Neyns B. Long-term clinical outcome of melanoma patients treated with messenger RNA electroporated dendritic cell therapy following complete resection of macrometastases. Cancer Immunol Immunother 2015; 64:381-8; PMID:25548092; http://dx.doi.org/10.1007/s00262-014-1642-8
- Steinman RM. Decisions about dendritic cells: past, present, and future. Annu Rev Immunol 2012; 30:1-22; PMID:22136168; http://dx.doi.org/10.1146/annurev-immunol-100311-102839
- Mac Keon S, Ruiz MS, Gazzaniga S, Wainstok R. Dendritic cell-based vaccination in cancer: Therapeutic implications emerging from murine models. Front Immunol 2015; 6:1-18; PMID:25657648; http://dx.doi.org/10.3389/fimmu.2015.00243
- Palucka K, Banchereau J. Dendritic-cell-based therapeutic cancer vaccines. Immunity 2013; 39:38-48; PMID:23890062; http://dx.doi.org/10.1016/j.immuni.2013.07.004
- Gallois A, Bhardwaj N. Dendritic cell-targeted approaches to modulate immune dysfunction in the tumor microenvironment. Front Immunol 2013; 4:1-8; PMID:23355837; http://dx.doi.org/10.3389/fimmu.2013.00436
- Lutz MB, Rößner S. Factors influencing the generation of murine dendritic cells from bone marrow: The special role of fetal calf serum. Immunobiology 2008; 212:855-62; PMID:18086384; http://dx.doi.org/10.1016/j.imbio.2007.09.001
- Umansky V, Sevko A, Gebhardt C, Utikal J. Myeloid-derived suppressor cells in malignant melanoma. J Dtsch Dermatologische Gesellschaft 2014; 12:1021-7; PMID:25263083; http://dx.doi.org/10.1111/ddg.12411
- Umansky V, Sevko A. Melanoma-induced immunosuppression and its neutralization. Semin Cancer Biol 2012; 22:319-26; PMID:22349515; http://dx.doi.org/10.1016/j.semcancer.2012.02.003
- Poschke I, Kiessling R. On the armament and appearances of human myeloid-derived suppressor cells. Clin Immunol 2012; 144:250-68; PMID:22858650; http://dx.doi.org/10.1016/j.clim.2012.06.003
- Facciabene A, Motz GT, Coukos G. T-regulatory cells: Key players in tumor immune escape and angiogenesis. Cancer Res 2012; 72:2162-71; PMID:22549946; http://dx.doi.org/10.1158/0008-5472.CAN-11-3687
- Savage PA, Malchow S, Leventhal DS. Basic principles of tumor-associated regulatory T cell biology. Trends Immunol 2013; 34:33-40; PMID:22999714; http://dx.doi.org/10.1016/j.it.2012.08.005
- Cafri G, Amram E, Rinott G, Koifman G, Fishman S, Keisari Y, Tzehoval E, Margalit A, Eisenbach L, Gross G. Coupling presentation of MHC class I peptides to constitutive activation of antigen-presenting cells through the product of a single gene. Int Immunol 2011; 23:453-61; PMID:21652516; http://dx.doi.org/10.1093/intimm/dxr033
- Cafri G, Sharbi-Yunger A, Tzehoval E, Alteber Z, Gross T, Vadai E, Margalit A, Gross G, Eisenbach L. mRNA-transfected dendritic cells expressing polypeptides which link MHC-I presentation to constitutive TLR4 activation confer tumor immunity. Mol Ther 2015; 23(8):1391-400; PMID:25997427; http://dx.doi.org/10.1038/mt.2015.90
- Kato M, Takahashi M, Akhand AA, Liu W, Dai Y, Shimizu S, Iwamoto T, Suzuki H, Nakashima I. Transgenic mouse model for skin malignant melanoma. Oncogene 1998; 17:1885-8; PMID:9778055; http://dx.doi.org/10.1038/sj.onc.1202077
- Umansky V, Abschuetz O, Osen W, Ramacher M, Zhao F, Kato M, Schadendorf D. Melanoma-specific memory T cells are functionally active in Ret transgenic mice without macroscopic tumors. Cancer Res 2008; 68:9451-8; PMID:19010920; http://dx.doi.org/10.1158/0008-5472.CAN-08-1464
- Abschuetz O, Osen W, Frank K, Kato M, Schadendorf D, Umansky V. T-cell mediated immune responses induced in ret transgenic mouse model of malignant melanoma. Cancers (Basel) 2012; 4:490-503; PMID:24213320; http://dx.doi.org/10.3390/cancers4020490
- Lutz MB, Kukutsch N, Ogilvie a L, Rössner S, Koch F, Romani N, Schuler G. An advanced culture method for generating large quantities of highly pure dendritic cells from mouse bone marrow. J Immunol Methods 1999; 223:77-92; PMID:10037236; http://dx.doi.org/10.1016/S0022-1759(98)00204-X
- Henao-Tamayo MI, Ordway DJ, Irwin SM, Shang S, Shanley C, Orme IM. Phenotypic definition of effector and memory T-lymphocyte subsets in mice chronically infected with mycobacterium tuberculosis. Clin Vaccine Immunol 2010; 17:618-25; PMID:20107011; http://dx.doi.org/10.1128/CVI.00368-09
- Kim H, Cantor H. CD4 T-cell subsets and tumor immunity: The helpful and the not-so-helpful. Cancer Immunol Res 2014; 2:91-8; PMID:24778273; http://dx.doi.org/10.1158/2326-6066.CIR-13-0216
- Tepper RI, Pattengale PK, Leder P. Murine interleukin-4 displays potent anti-tumor activity in vivo. Cell 1989; 57:503-12; PMID:2785856; http://dx.doi.org/10.1016/0092-8674(89)90925-2
- Tatsumi T, Kierstead LS, Ranieri E, Gesualdo L, Schena FP, Finke JH, Bukowski RM, Mueller-Berghaus J, Kirkwood JM, Kwok WW, et al. Disease-associated bias in T helper type 1 (Th1)/Th2 CD4(+) T cell responses against MAGE-6 in HLA-DRB10401(+) patients with renal cell carcinoma or melanoma. J Exp Med 2002; 196:619-28; PMID:12208877; http://dx.doi.org/10.1084/jem.20012142
- Grant CR, Liberal R, Mieli-Vergani G, Vergani D, Longhi MS. Regulatory T-cells in autoimmune diseases: Challenges, controversies and—yet—unanswered questions. Autoimmun Rev 2015; 14:105-16; PMID:25449680; http://dx.doi.org/10.1016/j.autrev.2014.10.012
- Jethwa H, Adami AA, Maher J. Use of gene-modified regulatory T-cells to control autoimmune and alloimmune pathology: Is now the right time? Clin Immunol 2014; 150:51-63; PMID:24333533; http://dx.doi.org/10.1016/j.clim.2013.11.004
- Zhang X, Kelaria S, Kerstetter J, Wang J. The functional and prognostic implications of regulatory T cells in colorectal carcinoma. J Gastrointest Oncol 2015; 10:307-13; PMID:26029458; http://dx.doi.org/10.3978/j.issn.2078-6891.2015.017
- Katoh H, Watanabe M. Myeloid-derived suppressor cells and therapeutic strategies in cancer. J Mediat Inflamm 2015; 2015:1-12; PMID:26078490; http://dx.doi.org/10.1155/2015/159269
- Draghiciu O, Lubbers J, Nijman HW, Daemen T. Myeloid derived suppressor cells—An overview of combat strategies to increase immunotherapy efficacy. Oncoimmunology 2015; 4:e954829; PMID:25949858; http://dx.doi.org/10.4161/21624011.2014.954829
- Gabrilovich DI, Ostrand-Rosenberg S, Bronte V. Coordinated regulation of myeloid cells by tumours. Nat Rev Immunol 2012; 12:253-68; PMID:22437938; http://dx.doi.org/10.1038/nri3175
- Gabrilovich DI, Nagaraj S. Myeloid-derived-supressor cells as regulators of the immune system. Nat Rev Immunol 2009; 9:162-74; PMID:19197294; http://dx.doi.org/10.1038/nri2506
- Cauley LS, Miller EE, Yen M, Swain SL. Superantigen-induced CD4 T cell tolerance mediated by myeloid cells and IFN-gamma. J Immunol 2000; 165:6056-66; PMID:11086037; http://dx.doi.org/10.4049/jimmunol.165.11.6056
- Bronte V, Wang M, Overwijk WW, Surman DR, Pericle F, Rosenberg SA, Restifo NP. Apoptotic death of CD8+ T lymphocytes after immunization: induction of a suppressive population of Mac-1+/Gr-1+ cells. J Immunol 1998; 161:5313-20; PMID:9820504
- Meyer C, Sevko A, Ramacher M, Bazhin AV, Falk CS, Osen W, Borrello I, Kato M, Schadendorf D, Baniyash M, et al. Chronic inflammation promotes myeloid-derived suppressor cell activation blocking IFN tumor immunity in transgenic mouse melanoma model. Proc Natl Acad Sci 2011; 108:17111-6; PMID:21969559; http://dx.doi.org/10.1073/pnas.1108121108
- Lengagne R, Pommier A, Caron J, Douguet L, Garcette M, Kato M, Avril MF, Abastado JP, Bercovici N, Lucas B, et al. T cells contribute to tumor progression by favoring pro-tumoral properties of intra-tumoral myeloid cells in a mouse model for spontaneous melanoma. PLoS One 2011; 6:1-10; PMID:21633700; http://dx.doi.org/10.1371/journal.pone.0020235