ABSTRACT
We have previously reported that intermediate monocytes (CD14++/CD16+) were increased in colorectal cancer (CRC) patients, while the subset of pro-angiogenic TIE2-expressing monocytes (TEMs) was not significantly elevated. This study was designed to evaluate changes in frequency and function of intermediate monocytes and TEMs during chemotherapy and anti-angiogenic cancer treatment and their relation to treatment response. Monocyte populations were determined by flow cytometry in 60 metastasized CRC (mCRC) patients who received neoadjuvant chemotherapy with or without bevacizumab. Blood samples were taken before treatment, after two therapy cycles, at the end of neoadjuvant therapy and immediately before surgical resection of liver metastases. Neoadjuvant treatment resulted in a significant increase in circulating intermediate monocytes which was most pronounced after two cycles and positively predicted tumor response (AUC = 0.875, p = 0.005). With a cut-off value set to 1% intermediate monocytes of leukocytes, this parameter showed a predictive sensitivity and specificity of 75% and 88%. Anti-angiogenic therapy with bevacizumab had no impact on monocyte populations including TEMs. In 15 patients and six healthy controls, the gene expression profile and the migratory behavior of monocyte subsets was evaluated. The profile of intermediate monocytes suggested functions in antigen presentation, inflammatory cytokine production, chemotaxis and was remarkably stable during chemotherapy. Intermediate monocytes showed a preferential migratory response to tumor-derived signals in vitro and correlated with the level of CD14+/CD16+ monocytic infiltrates in the resected tumor tissue. In conclusion, the rapid rise of intermediate monocytes during chemotherapy may offer a simple marker for response prediction and a timely change in regimen.
Abbreviations
5FU | = | 5-fluorouracil |
AUC | = | area under the curve |
BSA | = | bovine serum albumin |
CRC | = | colorectal cancer |
CTSD | = | cathepsin D |
EBM2 | = | endothelial basal medium 2 |
FACS | = | fluorescence-activated cell sorting |
LPS | = | lipopolysaccharide |
mCRC | = | metastasized CRC |
MMP | = | matrix-metalloproteinase |
PBMC | = | peripheral blood mononuclear cell |
RECIST | = | response evaluation criteria in solid tumors |
ROC | = | receiver operating characteristic |
TAM | = | tumor-associated macrophage |
TEM | = | TIE2 expressing monocyte |
TIMP | = | tissue inhibitor of matrix-metalloproteinase |
VEGF | = | vascular endothelial growth factor |
Introduction
Metastasis to secondary organs such as the liver is the major cause of CRC related mortality. It is well established that monocytes are actively recruited to primary as well as secondary tumor sites by cytokines and chemokines secreted by tumor and stromal cells. They give rise to tumor-associated macrophages (TAMs) distinct from resident tissue macrophages.Citation1,2 These TAMs may promote tumor growth by stimulation of angiogenesis, inhibition of host immunity, matrix remodeling and promotion of invasion and metastasis.Citation3 A high number of TAMs has been reported to correlate with poor patient prognosis in various malignancies.Citation4-6 However, in early cancer development, they were also shown to be beneficial, because they may react against tumor cells and exert angiostatic effects.Citation7,8 Thus, it is the local cytokine milieu that tunes the function of TAMs to suppress or promote tumor growth. Furthermore, growing evidence shows that monocytes and/or TAMs may enhance or suppress the efficacy of anticancer therapy including cytotoxic as well as anti-angiogenic agents.Citation9 The impact of monocytes on tumor development and treatment is further complicated by the fact that blood monocytes are a very heterogeneous population of cells with different biological properties.
Monocytes are characterized by the expression of CD14, a co-receptor for the bacterial component LPS, and heterogeneity is reflected in the level of CD14 and of co-expressed Fcγ receptor CD16; three different monocyte subtypes have been identified: CD14++CD16− “classical”, CD14++CD16+ “intermediate” and CD14+CD16++ “non-classical” monocytes.Citation10 Recent studies on the gene expression profile of monocyte subsets have suggested a developmental relationship between these populations with gradual changes in surface markers during maturation.Citation11,12 In accordance, the non-classical subset may represent the most progressed stage of differentiation.
Functional properties of classical monocytes (85–90% of total monocytes) include the highest phagocytosis potential and a pronounced response to LPS.Citation11,12 Non-classical monocytes (5%) exhibit a “patrolling” behavior i.e. crawling along vessel walls to screen for integrityCitation13 and they react strongly to viral signals.Citation14 Intermediate monocytes amount to about 5–10% of blood monocytes. Gene expression studies revealed elevated levels of HLA-DR and CD74 thus pointing to a dedicated role in antigen presentation and inflammation. However, angiogenic receptors such as TIE2 were also expressed at highest levels, and intermediate monocytes showed the highest pro-angiogenic potential in vitro. In line, the subset of TEMs was found to be recruited to tumor sites in vivo and to stimulate tumor angiogenesis by the paracrine release of growth factors like VEGF (vascular endothelial growth factor).Citation15
We have previously reported that intermediate monocytes (as opposed to classical and non-classical monocytes) are specifically increased in CRC patients when compared to healthy individuals.Citation16 The intermediate subset of TEMs showed a comparable tendency but was not significantly elevated. Given the pro-inflammatory as well as pro-angiogenic properties of intermediate monocytes, their role in malignant disease seems paradoxical, as they may exert both, pro- and antitumor effects. Importantly, they may also be implicated in the resistance against tumor therapy. For example, an accumulation of TEMs at the tumor site has recently been reported to mediate resistance to anti-angiogenic (anti-VEGF) cancer treatmentCitation17 and may be altered by the therapy.Citation18
Based on our previous study demonstrating a selective rise and a marker potential of intermediate monocytes in CRC patients,Citation16 we further hypothesized that CRC therapy has an impact on the frequency and function of intermediate monocytes (and possibly the TEM subset). Thus, we aimed to: (1) define the phenotypical and functional properties of intermediate monocytes from mCRC patients and (2) investigate if these properties are affected by neoadjuvant chemotherapy with or without the addition of the VEGF inhibitor bevacizumab (Avastin®). We performed an extensive analysis on the frequency of monocyte subsets in blood, their gene expression profile and chemotactic response to tumor-derived stimuli. Furthermore, tumor infiltration by monocytes was investigated in tissue specimens of resected colorectal liver metastases previously treated with neoadjuvant therapy. The investigated parameters were evaluated regarding correlation with and predictive potential for response to chemotherapy and anti-VEGF treatment.
Results
Neoadjuvant therapy triggers a significant increase in intermediate monocytes in the blood of mCRC patients
The study collective was based on 60 CRC patients who underwent neoadjuvant treatment to achieve or improve resectability of liver metastases. They received 5-fluorouracil (5FU) based chemotherapy with (n = 43) or without (n = 17) the addition of bevacizumab. Fifteen patients were subsequently considered unresectable, i.e., were treated in a palliative manner. Patient demographics and therapy regimens are summarized in . Patient groups with or without bevacizumab did not differ substantially in patient characteristics or treatment modalities. 59% of patients showed response (partial remission) to neoadjuvant therapy, while 41% were categorized as non-responders with 31% stable disease and 10% progressive disease (without significant difference between treatment arms).
Table 1. Demographic and clinical variables of study participants.
By direct triple immunostaining of whole blood and flow cytometry, we evaluated the frequency of circulating monocyte populations in mCRC patients. In response to neoadjuvant therapy, the percentage of intermediate monocytes (among leukocytes) significantly increased at all investigated time points (). The rise in intermediate monocytes was most pronounced after two treatment cycles (intra neo), i.e., the percentage of intermediate monocytes doubled from a median baseline value of 0.45% to 1.0% upon therapy. With respect to TEMs, a tendency toward increased values after two cycles of therapy was observed but failed to reach statistical significance (). To assess whether the upregulation of intermediate monocytes was based on a general rise of monocytes in patients during neoadjuvant treatment, we comparatively investigated the changes of total CD14++ monocytes () and CD14++CD16− classical monocytes () under therapy. Since the classical subset constitutes the majority of circulating monocytes, a similar time course was observed. Classical and total monocyte levels were increased at all time points of neoadjuvant treatment. However, in contrast to intermediate monocytes, the peak values were recorded at the end of therapy (post neo): Total CD14++ monocyte counts rose from a median level of 7.0% at baseline to 11.8% after therapy completion. Monocyte levels declined moderately in the treatment-free period immediately prior to surgery (pre OP). Thus, a shift in subset distribution to a higher frequency of intermediate monocytes was limited to the early treatment period (intra neo) as illustrated by the ratio of intermediate to classical monocytes ().
Figure 1. Frequency of circulating monocyte subsets among leukocytes in the course of neoadjuvant therapy. Monocyte subsets were determined by flow cytometry in whole blood samples from patients undergoing neoadjuvant treatment of colorectal liver metastases. The time course of monocyte populations is shown for the entire patient collective without separation into treatment arms, and covers the time points before neoadjuvant treatment (pre neo), after two therapy cycles (intra neo), on the last day of neoadjuvant therapy (post neo) and immediately prior to surgery (pre OP). The percentage of (A) CD14++CD16+ intermediate (IM) monocytes within leukocytes, of (B) CD14++CD16+TIE2+ TEMs, of (C) total CD14++ monocytes, of (D) CD14++CD16− classical (CL) monocytes, as well as the ratio of intermediate to classical monocytes (E) are illustrated by boxplots. p values are the result of the non-parametrical Wilcoxon test.
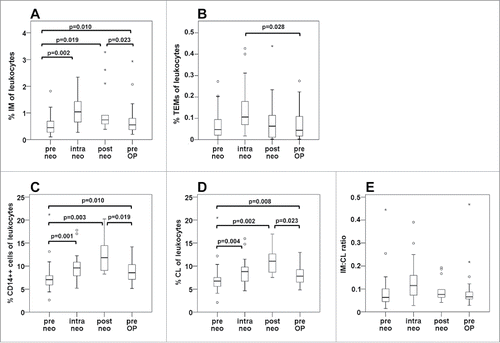
Monocyte alterations in response to chemotherapy are not affected by concomitant anti-VEGF treatment
When comparing the percentage of intermediate monocytes () and TEMs () in patients who had been treated with or without the addition of bevacizumab to chemotherapy, no significant difference was observed between the two treatment arms at any of the investigated time points. A significant rise in circulating intermediate monocytes after two cycles of therapy was confirmed for both, patients with (p = 0.028) and without (p = 0.018) anti-VEGF treatment. Of note, median TEM levels were lower in the bevacizumab group before treatment start and remained consistently (but non-significantly) lower throughout the entire treatment period, while showing a comparable time course. Similarly, no significant difference between the two patient collectives was recorded during neoadjuvant therapy for total CD14++ monocytes, classical monocytes or the ratio of intermediate to classical subsets (). Since no bevacizumab specific effects were observed, data of both treatment arms were combined to a larger collective in further statistical analyses.
Figure 2. Comparison of monocyte subsets between treatment arms of neoadjuvant therapy. The percentage of monocyte subsets within total leukocytes as determined by whole blood staining and flow cytometry was compared between patients undergoing chemotherapy with (gray boxes) or without (white boxes) the addition of bevacizumab. Monitoring included time points prior to neoadjuvant treatment (pre neo), after two therapy cycles (intra neo), on the last day of neoadjuvant therapy (post neo) and immediately before surgery (pre OP). The percentage of (A) CD14++CD16+ intermediate (IM) monocytes within leukocytes, of (B) CD14++CD16+TIE2+ TEMs, of (C) total CD14++ monocytes, of (D) CD14++CD16− classical (CL) monocytes, as well as the ratio of intermediate to classical monocytes (E) are illustrated by boxplots. There were no statistically significant differences between treatment arms at any of the investigated time points as evaluated by Mann–Whitney-U test.
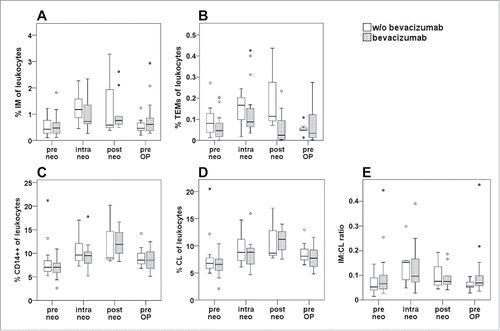
The rise of intermediate monocytes after two chemotherapy cycles significantly correlates with response to neoadjuvant therapy
Patients' response to neoadjuvant therapy was evaluated by RECIST criteria. We found a highly significant correlation of intermediate monocyte levels (recorded after two treatment cycles) with patient response (). While median levels of responders ranged at 1.29%, non-responders showed intermediate monocyte concentrations comparable to baseline levels at 0.54% (p = 0.005). We further evaluated the marker performance of intermediate monocytes for prediction of response to neoadjuvant treatment by ROC analysis. The predictive power was evaluated by the area under the curve (AUC = 0.875, p = 0.005). With a cut-off value set at 1.07%, intermediate monocytes showed a predictive sensitivity and specificity of 75% and 88%, respectively ().
Figure 3. Correlation of intermediate monocytes with treatment response. Response to neoadjuvant therapy was evaluated by the RECIST criteria. Complete or partial response was classified as “responder”, stable disease or progressive disease was classified as “non-responder”. (A) The frequency of intermediate monocytes (IM) as established after two cycles of neoadjuvant therapy is illustrated by boxplot and differs significantly between responders and non-responders (Mann–Whitney-U test). (B) The predictive marker potential was further evaluated by ROC curve analysis (AUC: area under the curve).
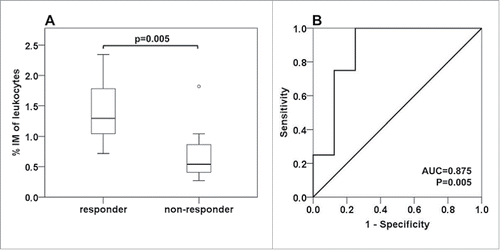
Intermediate monocytes show a gene expression pattern distinct from other subsets which is not affected by neoadjuvant therapy
To gain insight whether the intermediate cell population in mCRC patients differs from other monocyte subsets in terms of gene expression (reflecting cell functions) and whether the expression pattern is altered during neoadjuvant treatment, we isolated the three monocyte subsets (classical, intermediate, non-classical) from patient blood. Since immunostaining was restricted to CD14 and CD16, the detection of non-classical monocytes was prone to contamination with natural killer cells or granulocytes. Hence, we had to apply a more stringent gating strategy () to avoid interference in the FACsorting procedure. Total RNA was extracted and analyzed for the expression of TNFα, CD36, CCR5, HLA-DRA (HLA-DR α chain) and VEGF-A. This panel of proteins was selected, because the respective genes have previously been reported to be differentially expressed between monocyte subsetsCitation11,12 and represent antitumor (TNFα, CD36, HLA-DR) as well as pro-tumor (CCR5, VEGF) monocytic functions. Transcript levels were evaluated by real-time PCR for the sorted monocyte subsets from 15 patients before and after two cycles of therapy. For comparison, monocyte subsets from six age-matched healthy individuals were analyzed.
The expression levels of CD14 and CD16 transcripts differed significantly between the sorted CD14++CD16− classical, CD14++CD16+ intermediate and CD14+CD16++ non-classical subsets and hence confirmed the successful isolation of the distinct monocyte populations (). When evaluating baseline mRNA levels of monocyte populations in mCRC patients, CCR5 and HLA-DRA were expressed at highest levels in intermediate monocytes (). Furthermore, they showed intermediate levels of TNFα and CD36 transcripts, while highest levels of TNFα were found in non-classical monocytes () and maximum CD36 expression was detected in classical monocytes (). VEGF-A expression did not differ among the three monocyte populations (). When baseline levels of the detected mRNAs in mCRC patients were compared with the gene expression profile of healthy individuals, no significant differences were found (). Importantly, gene expression in monocyte subsets from mCRC patients did not change after two cycles of neoadjuvant therapy (). While illustrates the data for TNFα, CCR5 and HLA-DRA, no significant differences were comparably detected for CD14, CD16, CD36 and VEGF-A (data not shown).
Figure 4. Gene expression profile of monocyte subsets from mCRC patients prior to therapy. Classical (CL), intermediate (IM) and non-classical monocytes (NC) from mCRC patients before neoadjuvant treatment were isolated by gradient centrifugation, CD14/CD16 immunostaining and fluorescence-activated cell sorting. Expression of genes involved in monocyte function was determined at the mRNA level by quantitative real-time PCR. Values were normalized to the housekeeping gene β-actin and were calculated in relation to a consistent control sample. Transcripts of (A) CD14, (B) CD16, (C) CCR5, (D) HLA-DRA, (E) TNFα, (F) CD36 and (G) VEGF-A were assessed. Statistical analysis was based on the paired t-test. Please note that boxplot E does not show an extreme value (5.9) of TNFα expression in classical monocytes (scale adjusted to improve resolution) which was, however, included in the statistical evaluation.
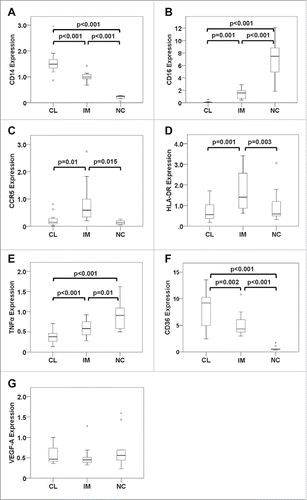
Figure 5. Monocyte gene expression pattern of mCRC patients in relation to healthy individuals or neoadjuvant treatment. Monocyte subsets (CL: classical, IM: intermediate, NC: non-classical) were isolated by FACS and gene expression was determined by real-time PCR for TNFα (A,D), CCR5 (B,E) and HLA-DRA (C,F). (A–C) Transcript levels in healthy volunteers (gray boxes) and mCRC patients (white boxes) before cancer therapy were compared by unpaired t-test. (D–F) The expression pattern of monocyte subsets was evaluated at baseline (white boxes) and after two cycles of neoadjuvant treatment (gray boxes) by paired t-test. Please note that boxplots A and D do not show an extreme value (5.9) of TNFα expression in classical monocytes of mCRC patients prior to therapy (scale adjusted to improve resolution) which was, however, included in the statistical evaluation.
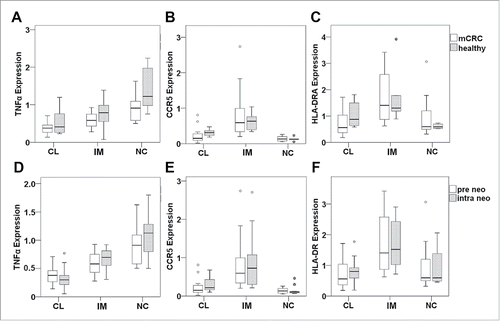
Intermediate monocytes show a strong migratory response which tends to increase in mCRC patients upon neoadjuvant therapy
To address the question whether intermediate monocytes might not only be elevated in patient blood but also respond to tumor-derived guidance cues for extravasation, we investigated the migratory behavior of monocyte subsets in a transendothelial migration assay with peripheral blood mononuclear cells (PBMCs) isolated from mCRC patients (n = 14) before and after two cycles of chemotherapy as well as for six healthy individuals. PBMCs were added to the upper chamber of a transwell and stimulated to traverse a confluent endothelial layer in response to tumor signals (cell culture supernatant of CRC lines HT-29, SW480 or SW620) applied to the lower chamber. Transmigrated monocytes were analyzed for subset composition by flow cytometry. Since the assay was based on isolated PBMCs as opposed to the previously analyzed whole blood samples of patients, monocyte frequency could not be calculated in % of total leukocytes. To possibly detect the preferential migration of monocyte subpopulations, the ratio of transmigrated intermediate to classical monocytes was assessed ( and Fig. S2).
Figure 6. Monocyte migration in vitro and tumor tissue infiltration in vivo. (A) PBMCs were isolated from mCRC patients prior to neoadjuvant treatment (pre neo) or after two therapy cycles (intra neo) as compared to healthy controls (healthy). Transendothelial migration in response to tumor-derived stimuli was assessed in a transwell assay after 4 h of incubation with cell culture supernatant of colorectal cancer lines. The ratio of intermediate to classical monocytes was calculated for the transmigrated population and combined data from all transmigration assays are illustrated by boxplots. Fig. S2 illustrates migration assays separately for SW480, SW620 and HT-29 culture supernatant. Statistical analysis was based on unpaired (pre neo vs. healthy) or paired (pre neo versus intra neo) t-test. (B–E) Tissue sections of colorectal liver metastases were immunostained for CD14 and CD16 (including a DNA dye for cell nucleus detection). (C) Images acquired with a confocal laser scanning microscope show fluorescence signals for DNA in blue, CD16 in green and CD14 in red. An overlay of all three colors is enlarged in (D). The orange line indicates the border between tumor tissue and hepatocytes with high background fluorescence in the green channel. Examples for tumor-infiltrating monocytes positive for CD14 (arrow) or double-positive for CD14 and CD16 (arrow head) are highlighted. (E) A section of the image (white box) is shown in higher magnification. Immunostained tissue slides were scanned with the TissueFAXS device and subjected to automated analysis by TissueQuest software. The frequency of CD14+CD16− vs. CD14+CD16+ monocytes was determined per mm2 of tissue. Tumor area and adjacent border region were evaluated separately (Fig. S5). The correlation between tumor infiltrating CD14+CD16+ monocytes and the intermediate monocyte concentration in the blood of the same patients at the time of surgery was calculated based on Pearson´s correlation coefficient (B).
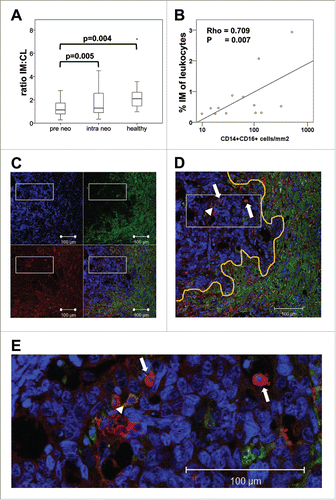
While the median ratio of these monocyte subsets in the peripheral blood of mCRC patients prior to therapy ranged at 0.06 () and illustrated the predominance of >90% classical monocytes in circulation, the fraction of intermediate monocytes within the in vitro migrated cells was substantially higher () and reached a median ratio of 1.13. This ratio corresponds to about 50% of transmigrated intermediate monocytes. Of interest, the ratio of the intermediate to classical subset was even higher (median of 2.09) when blood from healthy donors was tested in the in vitro migration assay indicating a more potent migration of intermediate monocytes in healthy individuals as compared to mCRC patients prior to treatment (p = 0.004 for all CRC lines combined). After two cycles of neoadjuvant chemotherapy of mCRC patients, the ratio tended to increase (p = 0.005 for all CRC lines combined) but remained below the healthy control level (median of 1.29).
The preferential transmigration of intermediate monocytes is associated with the tumor chemokine profile rather than subset expression of migration factors
We further aimed to delineate whether the enhanced migration of the intermediate subset would be a function of endogenous migration regulators such as matrix-metalloproteinases (MMPs) and their inhibitors (TIMPs). Candidate proteins were again selected based on a former gene expression study showing divergent transcript levels in monocyte subsets for MMP-25 (MT6-MMP) and TIMP-1.Citation11 Furthermore, the tumor promoter and matrix-degrading protease cathepsin D (CTSD) was investigated, as it has previously been reported to be induced upon monocyte maturation,Citation19,20 and MMP-14 (MT1-MMP) was included, since it has been specifically connected to monocyte trans-endothelial migration.Citation21 None of the analyzed proteases showed a preferential expression in intermediate monocytes of CRC patients prior to treatment (). In contrast, MMP-25 and cathepsin D were found to be significantly lower while TIMP-1 levels were significantly higher in intermediate than classical monocytes. No difference in parameter expression was observed between CRC patients and healthy controls (Fig. S3) or in CRC patients before and after chemotherapy (Fig. S4).
Figure 7. Expression of migration regulators in monocyte subsets from mCRC patients prior to therapy. Classical (CL), intermediate (IM) and non-classical monocytes (NC) were isolated from mCRC patients before neoadjuvant treatment to extract cellular RNA and generate cDNA for analysis by quantitative real-time PCR. Transcripts levels of (A) MMP-14, (B) MMP-25, (C) cathepsin D and (D) TIMP-1 were determined. Statistical analysis was based on the paired t-test.
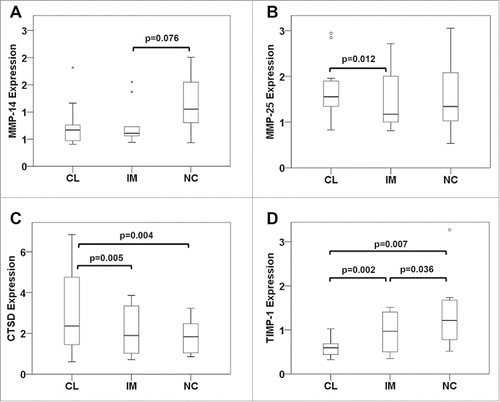
However, when tumor culture supernatants applied in transmigration assays were analyzed for their composition of chemokines by a bead-based multiplex immunoassay detecting CCL2, CCL3, CCL4, CCL5 and CXCL1, CXCL8, CXCL10, CXCL11, CXCL12, a predominance of ligands for the intermediate monocyte CCR5 receptor (CCL4, CCL5) was apparent, while the ligand CCL2 for the classical monocyte receptor CCR2 was low or absent (). Expression levels of CCR5 ligands varied among the three investigated CRC lines, with SW480 cells secreting the highest amounts of CCL5 and SW620 cells releasing highest levels of CCL4. Remarkable concentrations were also recorded for CXCL1, CXCL8 and CXCL12 which represent chemokines with a wider spectrum of responsive cell types including neutrophils and monocytes.Citation22
Table 2. Chemokine composition of CRC culture supernatants.
Tumor tissue infiltration correlates with blood levels of intermediate monocytes
The in vitro investigations on the migratory potential of blood-derived monocytes were further complemented with tissue analyses of resected colorectal liver metastases. Liver sections were immunostained for infiltrating cells expressing CD14 and CD16. Using TissueFAXS technology, digital images of tumor area, border region and surrounding liver tissue were acquired and analyzed for CD14+ monocytes positive or negative for the CD16 surface marker (Fig. S5). Hepatocytes showed substantial autofluorescence but were essentially negative for CD14 and CD16 (). In contrast, CRC metastases showed little background fluorescence and were therefore easily distinguished from the liver cells. The tumor tissue presented with infiltrating CD14 and/or CD16 positive cells. The highest density of infiltrating cells was detected in the border region between tumor and adjacent liver tissue. gives a typical example of tumor infiltrating monocytes positively stained for CD14 with or without the co-expression of CD16. The frequency of these monocyte populations per mm2 of tissue was calculated by automated analysis. It should be noted that TissueFAXS technology did not enable us to distinguish between non-classical and intermediate monocytes. Hence, monocytes were grouped into CD14+CD16− versus CD14+CD16+ populations. However, since non-classical monocytes exhibit very low CD14 expression, they were less likely to be detected in this analysis. Comparing blood and tissue levels of monocyte subsets we found a significant, positive correlation () for intermediate monocytes in patient blood at the time of surgery (pre OP) and CD14+CD16+ monocyte infiltrates in the resected tumor area (Rho = 0.709, p = 0.007) but not in the adjacent border region. No correlation was observed for classical monocytes in blood and tissue.
Discussion
We here report the first comprehensive analysis on the regulation of monocyte subsets in mCRC patients undergoing neoadjuvant therapy. A significant increase of intermediate monocytes was detected in response to 5FU-based chemotherapy irrespective of the addition of anti-VEGF antibody bevacizumab. The rise in intermediate monocytes after two cycles of neoadjuvant treatment showed a highly significant, positive correlation with radiological treatment response as evaluated by RECIST criteria.
Monocytes are actively recruited to the tumor by a wide array of chemokines and growth factors, including MCP-1 (CCL2), MIP-1α (CCL3), MIP-1β (CCL4) and RANTES (CCL5).Citation23 However, the role of monocytes and particular subpopulations in the tumor setting is not fully understood. Microarray studies by Zawada et al.Citation12 have linked the subset of intermediate monocytes to pro-inflammatory as well as pro-angiogenic properties. Therefore, their role in malignant disease seems ambiguous, as this subset is capable of stimulating tumor angiogenesis on the one hand, but may also exert antitumor effects on the other hand by the production of pro-inflammatory cytokines such as TNF-α, IL-1β and IL-6 and by antigen presentation.Citation11,24
In the present study with mCRC patients, intermediate monocytes in blood seem to reflect a systemic immunological response to chemotherapy. Thus, their increase in circulation may be induced by a systemic spillover of mediators released by the therapeutically damaged tumor tissue. Alternatively, chemotherapy may directly trigger changes in the differentiation or recruitment of monocyte subsets which might support the antitumor response. Both scenarios would explain the highly significant correlation of intermediate monocytes in blood with patient response to cancer therapy. Previous studies have shown an increase of intermediate monocytes in other inflammatory conditions including rheumatoid arthritis and sepsis.Citation24,25 Thus, evaluation of monocyte subsets in tumor patients certainly has to take into consideration potential co-existing morbidities. However, the therapy-triggered rise in intermediate monocytes as compared to pretreatment values may identify a tumor-related effect and hence serve as a novel marker for the prediction of treatment response. The subset of classical monocytes comparably showed a late rise during chemotherapy and did not correlate with treatment response. This finding is in line with a proposed pro-tumor function of classical monocytes, as they were recently reported to correlate with poor prognosis of pancreatic cancer patients.Citation26
In contrast to total intermediate monocytes, the potentially pro-angiogenic TEM subset of intermediate monocytes did not significantly increase in response to chemotherapy. In line, when we previously compared TEM frequency in the blood of CRC patients and healthy individuals, no significant difference was detected.Citation16 While this finding may be specific to the respective tumor entity,Citation27 it might also relate to the lower frequency of TEMs (as compared to intermediate monocytes) and hence detection limit in circulation. Since it was previously demonstrated in mouse models that pro-angiogenic, myeloid cell populations could confer resistance to anti-VEGF cancer therapy by providing substitute growth factors,Citation28,29 it was feasible to hypothesize that bevacizumab treatment might alter TEM frequency to compensate for the loss in VEGF activity. However, TEM (as well as intermediate monocyte) frequency remained unchanged in mCRC patients upon the addition of anti-VEGF treatment to chemotherapy. Hence, a potential effect of bevacizumab application on the gene expression profile of TEMs was not further investigated in this study, but all functional characterizations were focused on the chemotherapy-responsive intermediate monocyte subset.
A gene expression analysis of molecules which are considered key players in monocyte function and known to be differentially expressed among the three subsets was conducted for mCRC patients.Citation11,12 Highest TNFα expression was found in non-classical monocytes, consistent with a strong pro-inflammatory potential. HLA-DR and CCR5 were both expressed at highest levels in the intermediate subset, indicating a pronounced role in antigen-presentation as well as chemotactic response to tumor signals, respectively. CD36 was strongest in classical monocytes in line with the reportedly highest phagocytosis activity. Additionally, VEGF-A expression was measured and detected at comparable levels in all three monocyte subsets. While the low and uniform levels of VEGF transcripts were unexpected, all other results were in line with previously published reports on gene expression patterns of monocyte subsets.Citation11,12 Importantly, there were no significant differences in the gene expression profiles between monocyte subsets isolated from healthy controls and mCRC patients, even though we have previously shown that the frequency of subpopulations differs significantly between healthy individuals and mCRC patients.Citation16 Furthermore, chemotherapy did not alter the gene expression profile of monocyte subsets in mCRC patients. We therefore conclude that the presence (and therapy) of a tumor affects the frequency of monocyte subsets without changing their functional phenotype or identity in terms of characteristic transcript pattern. Despite the fact that several central players of monocyte function were investigated, our results and conclusions are limited by the circumstance that only a selection of relevant genes was analyzed, and other genes pertinent to subset function may be deregulated at the transcript or protein level in cancer bearing individuals or may be altered by tumor therapy.
We further conducted transendothelial migration assays with monocytes from healthy controls and mCRC patients before and after two therapy cycles to evaluate the potential of intermediate monocytes for extravasation. As monocyte chemotaxis and migration is a process which may involve numerous cytokines, we used different tumor cell culture supernatants as stimulus to mimic the diversity of signals present during in vivo monocyte recruitment into tumor tissue. While intermediate monocytes constituted only 6% of CD14++ monocytes in the peripheral blood of mCRC patients, they were highly enriched (53%) in the in vitro migrated population. It should be noted, though, that this is not merely a function of preferential transmigration but also a differentiation from classical to intermediate monocytes in response to the tumor-derived signals as we have previously reported.Citation16 When subset-specific gene expression was analyzed for regulators of monocyte migration and invasion, intermediate monocytes did not exhibit a favorable MMP/TIMP balance. Again, this conclusion is restricted to the investigated set of genes. However, the preferential recruitment of intermediate monocytes in the conducted transendothelial migration assays might be explained by the chemokine repertoire of tumor cells. Since the chemokine receptor CCR5 is predominantly present on intermediate monocytes, while CCR2 is among the most highly expressed genes of classical monocytes,Citation11,12 these subsets can be targeted by distinct chemotactic signals. Remarkably, all three investigated CRC lines showed a preference in the secretion of CCR5 over CCR2 ligands. Expression levels for the respective CCR5-interacting chemokines (CCL4 and CCL5) differed among the three tested CRC lines, as would be expected for the biological variation of patient tumors. The notion that circulating intermediate monocytes may indeed be effectively recruited to tumor tissue was further supported by the analysis of tumor infiltrating monocytes in resected liver metastases. We observed a significant positive correlation of intermediate monocytes in the blood at the time of surgery (pre OP), with the CD16+ monocyte infiltration in the tumor area.
In conclusion, we here report the first comprehensive analysis on the regulation and phenotype of intermediate monocytes in mCRC patients during neoadjuvant chemotherapy. In response to treatment, the frequency of intermediate monocytes in circulation increases; they show an enhanced migratory response to tumor signals and are found in the resected tumor tissue. The rise in circulating intermediate monocytes is not associated with alterations in the gene expression profile characteristic for this subset. Importantly, treatment response to neoadjuvant chemotherapy significantly correlates with the increase in intermediate monocytes detected in patient blood after two treatment cycles. These results may point to an antitumor, pro-inflammatory role of intermediate monocytes in mCRC. Furthermore, the blood level of intermediate monocytes may represent a novel marker for response prediction to neoadjuvant chemotherapy of mCRC. In particular, considering patients receiving conversion therapy a simple and fast method to assess treatment efficacy after only two cycles (4 weeks) would allow for a timely change in therapeutic regimen to achieve resectability of liver metastases and minimize hepatotoxicity of consecutive treatment schedules.
Materials and methods
Study design and patient collective
A total of 60 patients with colorectal liver metastases were enrolled at the Department of Surgery, Medical University of Vienna, between 2007 and 2012. Patients underwent neoadjuvant treatment to achieve or improve resectability of liver metastases. They received a 5FU-based chemotherapy regimen with or without the addition of bevacizumab at 5 mg/kg at biweekly intervals. The study was approved by the Institutional Ethics Committee (#300/2006, #437/2006, #791/2010). All study participants gave written informed consent.
Response to therapy was evaluated by computed tomography or magnetic resonance imaging according to the Response Evaluation Criteria in Solid Tumors (RECIST) by a trained radiologist and defined in categories of progressive disease, stable disease, or partial and complete remission. We further categorized patients as “responders” for “complete remission” and “partial remission” and as “non-responders” for “stable disease” and “progressive disease” (considering stable disease as insufficient response for patients receiving conversion therapy).
Sample collection
Blood was drawn at four defined time points in the course of neoadjuvant chemotherapy: immediately before the onset of the first neoadjuvant chemotherapy cycle (pre neo), after two cycles of neoadjuvant chemotherapy which was generally after 4 weeks and immediately before the third therapy cycle (intra neo), prior to administration of the last chemotherapy cycle (post neo) and 1–3 d before surgery (pre op). Blood was always retrieved before administration of chemotherapy.
Flow cytometric analysis of monocyte subsets in whole blood
Surface expression of CD14, CD16 and TIE2 was measured applying direct immunofluorescence staining followed by a lyse-no-wash procedure as we have previously reported.Citation16,30 In brief, 100 μL of EDTA-blood were incubated with the following antibodies at saturating concentrations for 20 min at room temperature: CD14-FITC (Becton-Dickinson #555397), CD16-PC5 (Beckman Coulter #A07767) and TIE2-PE (R&D Systems #FAB3131P). To eliminate erythrocytes, the Versa Lyse solution (Beckman Coulter #A09777) was added for 20 min.
Flow cytometry was immediately performed with an FC500 or Gallios cytometer (Beckman Coulter). Fluorescence gating parameters were established using antibody isotype controls, and values above the 99% negative staining threshold were considered positive. A total of 300,000 leukocytes were measured. Analysis of flow cytometry data was performed with Kaluza software (Beckman Coulter). The gating strategy is documented in Fig. S1A–C. Intermediate monocytes (CD14++CD16+) were identified by high-level expression of CD14 and were further distinguished from classical monocytes (CD14++CD16−) by their co-expression of CD16. TEMs were identified within the intermediate population by the concomitant expression of CD14, CD16 and TIE2 (CD14++CD16+TIE2+ cells). Data are given in % frequency of total leukocytes.
Gene expression analysis
Gene expression of monocyte subsets was analyzed prior to chemotherapy and after two treatment cycles. To this end, 20 mL of EDTA-treated whole blood were collected and PBMCs were isolated by ficoll gradient centrifugation. PBMCs were processed at 4°C, stained for 30 min with CD14-FITC (Becton-Dickinson #555397), CD16-PC5 (Beckman Coulter #A07767) antibodies in saturing concentrations in PBS−/− with 5% fetal calf serum. Cells were washed once and sorted with a FACSAria I device (Becton-Dickinson) at 4°C into classical, intermediate and non-classical monocyte populations according to CD14 and CD16 expression levels. Isotype controls were run in parallel in each experiment. The gating strategy is documented in Fig. S1D–E. Purity of sorted monocyte populations was validated with the same instrument. Purified subsets were immediately lysed in E.Z.N.A.™ TRK lysis buffer (Omega Bio-Tek/VWR #R6831-02) with 2% 2-mercaptoethanol and stored at −20°C until further processing. Total RNA was extracted using E.Z.N.A. MicroElute™ Total RNA Kit (Omega Bio-Tek/VWR #R6831-02). For cDNA synthesis, the DyNAmo™ cDNA Synthesis Kit (Finnzymes #F470L) was applied. Transcript levels of target genes were determined by quantitative real-time PCR as previously described.Citation31 For TNFα and TIE2 (TEK) expression, commercially available real-time PCR primers were used (TaqMan® Gene Expression Assay, Applied Biosystems #4331182). Comparably, validated real-time PCR primer sets for MMP-14, MMP-25, cathepsin D and TIMP-1 (PrimePCR™ SYBR® Green Assay # 10025636) were obtained from Bio-Rad Laboratories. The primer sets for HLA-DRA, CD36, CCR5 and β-actin were designed by Primer Express software (Applied Biosystems), they spanned at least one exon/intron boundary and sequences are given in Table S1. HLA-DRA, CD36, CCR5, VEGF and β-actin transcripts were evaluated by TaqMan technology, CD14, CD16, MMP-14, MMP-25, cathepsin D and TIMP-1 mRNA levels by SYBR Green detection system. The generated cDNA was diluted 1:20 before PCR analysis. Real-time PCR was performed with either ABI SYBR Fast (Applied Biosystems #4385616) or qPCR MasterMix Plus Low ROX (Eurogentec #RT-QP2X-03+WOULR). Oligonucleotide primers for SYBR Green detection were used at 300 nM forward primer and 300 nM reverse primer. For TaqMan assays 450 nM forward primer, 450 nM reverse primer and 200 nM probe were applied. Each sample was assayed in duplicate with the 7500 Fast PCR Detection System (Applied Biosystems) for 45 cycles of 15 sec at 95°C followed by 1 min at 60°C. Denaturing curves were established for all SYBR Green reactions to verify homogeneity of the amplified products. Transcript levels for target genes were determined using the E−ΔΔCt-method. The efficiency of reactions was calculated according to the equation E = 10−1/slope. Values were normalized to the housekeeping gene β-actin and are given in relation to a control sample included on each test plate. RNA extracted from PBMCs of seven different healthy donors and from primary human microvascular endothelial cells was combined and used as the control sample.
Tumor cell culture and supernatant analysis
Human CRC lines SW480, SW620 and HT-29 were obtained from the American Type Culture Collection (LGC Standards) and were seeded at a density of 4 × 105 per cm2 in growth medium with 10% fetal calf serum. After 24 h, medium was changed to endothelial basal medium 2 (EBM2 by Lonza #CC3156) with 0.1% bovine serum albumin (BSA), and tumor cell supernatants were collected after another 24 h. Supernatants were stored frozen in aliquots at −20°C until further use. Chemokine content was assessed with the 9-Plex Human Chemokine Panel I (Affymetrix/eBioscience #EPX090-12187-901) and the MAGPIX® instrument according to manufacturer's instructions.
Monocyte migration assay
The migratory behavior of monocytes was assessed before neoadjuvant therapy and after two cycles of treatment by transmigration of PBMCs through a confluent monolayer of human blood endothelial cells (BECs) seeded on PET cell culture inserts with 3 μm pore size in 30mm-wells (Becton Dickinson #353091). BECs were isolated from human foreskin as previously described.Citation32 6 × 105 cells were seeded on transwell inserts in endothelial medium EGM2-MV (Lonza #CC3202) without growth factor supplements to yield a confluent monolayer within 24 h. The next day, BEC inserts were transferred to culture wells containing the following stimuli for chemotaxis in the lower chamber: supernatant of SW480, SW620 or HT-29 tumor cells or EBM2 with 0.1% BSA as negative control. After 4 h of BEC preconditioning, PBMCs freshly isolated from mCRC patients were applied to the transwell at 2.1 × 106 cells in EBM2 with 0.1% BSA. Co-cultures were incubated for 4 h. Transmigrated PBMCs were then harvested from the lower chamber by collagenase incubation and scratching. PBMCs were stained with CD14-FITC, CD16-PC5 and TIE2-PE antibodies for 30 min at 4°C and fixed with 0.5% formaldehyde. Isotype controls were run in parallel. Flow cytometry was performed with a Gallios flow cytometer (Beckman Coulter). A total of 30,000 PBMCs were measured. Analysis of data was performed with Kaluza software (Beckman Coulter).
Immunofluorescence staining of tissue sections
Formalin-fixed and paraffin-embedded tissue sections of colorectal liver metastases resected from patients after neoadjuvant chemotherapy with (n = 23) or without the addition of bevacizumab (n = 10) were acquired from the local Institute of Pathology. Paraffin was melted at 80°C for 30 min. Sections were deparaffinized in 100% xylol and were rehydrated with decreasing ethanol concentrations. For antigen retrieval slides were autoclaved in 10 mM citrate buffer with 0.05% Tween20 at pH 6.0 for 1 min at 121°C. Permeabilization was achieved with 0.2% Tween20 in PBS. Unspecific background staining was blocked with Ultra-V block (Thermo Fisher Scientific #TA-060-UB) and Avidin/Biotin Blocking Kit (Vector Laboratories #SP2001). Rabbit anti-human CD14 (Novus Biologicals #NBP1-40683) was diluted 1:250 and slides were incubated for 1 h. Biotinylated horse anti-rabbit IgG antibody (Vector Laboratories #BA-1100) at 1:200 dilution was applied for 30 min. Mouse anti-human CD16 (Novocastra #NCL-CD16) was diluted 1:10 and added for 1 h. Further reagents were diluted 1:1000 for Hoechst 33342 (Molecular Probes, Thermo Fisher Scientific #H3570) and donkey anti-mouse Alexa Fluor® 488 (Molecular Probes #A21202) and 1:500 for streptavidin Alexa Fluor® 555 (Molecular Probes #S32355) and were applied for 1 h. Cover glasses were mounted using Fluoromount G (Southern Biotech #0100-01). Stained slides were analyzed with an LSM 700 confocal laser scanning microscope (Carl Zeiss).
Quantification of monocyte tissue infiltration
Slides were analyzed with TissueFAXS, an automated multi-channel immunofluorescence detection system including TissueQuest 4.0 software (TissueGnostics). The 20x/0.5 objective was selected for acquisition and the following filter sets were applied: DAPI filter for detection of Hoechst stained nuclei, FITC filter for detection of Alexa Fluor 488 stained CD16 and Texas Red filter for detection of Alexa Fluor 555 stained CD14. TissueFAXS parameter settings are listed in Table S2. Single cells were identified using an algorithm based on nuclear staining. Staining intensity around a so-called “nuclei mask” was evaluated for detection of CD14 and CD16 expression on the cell surface. The background threshold was determined by selecting positive and negative controls on each slide, and the integrated backward and forward gating tool was applied. Two different areas within tissue sections were analyzed separately: (i) tumor area and (ii) border region between tumor and surrounding normal liver tissue (as characterized by an extensive leukocytic infiltrate). Figure S5 shows a representative tissue specimen with delineated regions of interest: tumor and border region. Values are given as absolute cell count of CD14+, CD16+ and CD14+CD16+ cells per mm2 and the deduced number of CD14+CD16− infiltrating cells.
Statistical analysis
Statistical calculations of monocyte subsets in patient blood were based on non-parametric tests (Mann–Whitney U and Wilcoxon test) using SPSS software version 20 (SPSS Inc.). The Chi-square test or Fisher exact test (if expected frequencies were ≤5 ) were applied for categorical variables. The predictive marker potential was assessed by receiver operating characteristic (ROC) analysis. Statistical evaluation of in vitro assays was performed with paired or unpaired t-test. p values < 0.05 were considered statistically significant and were results of two-sided tests. As the study design was explorative, no correction for multiple testing was performed.
Disclosure of potential conflicts of interest
No potential conflicts of interest were disclosed.
KONI_A_1160185_supplementary_materials.zip
Download Zip (3.6 MB)Acknowledgments
We would like to thank Guenther Hofbauer (Core Facility Flow Cytometry, Medical University of Vienna) for assistance in FACsorting. Confocal images and TissueFAXS scans were acquired with the expert help of Marion Groeger and Sabine Rauscher (Core Facility Imaging, Medical University of Vienna).
Funding
This work was partly supported by the Medical Scientific Fund of the Mayor of the City of Vienna (http://www.wien.gv.at/gesundheit/einrichtungen/med-wiss-fonds/) by grant no. 8064 issued to T. Gruenberger. In addition, a personal award was granted to P. Starlinger by the “Hans and Blanca Moser Foundation” (no. 548) to support the project. The analysis of tumor infiltrating monocytes by TissueQuest software was kindly supported by Diana Mechtcheriakova and Erika Bajna (Institute for Pathophysiology, Medical University of Vienna), Georg Steiner and further representatives of TissueGnostics (Vienna, Austria).
References
- Murdoch C, Muthana M, Coffelt SB, Lewis CE. The role of myeloid cells in the promotion of tumour angiogenesis. Nat Rev Cancer 2008; 8:618-31; PMID:18633355; http://dx.doi.org/10.1038/nrc2444
- Yamashiro S, Takeya M, Nishi T, Kuratsu J, Yoshimura T, Ushio Y, Takahashi K. Tumor-derived monocyte chemoattractant protein-1 induces intratumoral infiltration of monocyte-derived macrophage subpopulation in transplanted rat tumors. Am J Pathol 1994; 145:856-67; PMID:7943176;
- Murdoch C, Giannoudis A, Lewis CE. Mechanisms regulating the recruitment of macrophages into hypoxic areas of tumors and other ischemic tissues. Blood 2004; 104:2224-34; PMID:15231578; http://dx.doi.org/10.1182/blood-2004-03-1109
- Leek RD, Lewis CE, Whitehouse R, Greenall M, Clarke J, Harris AL. Association of macrophage infiltration with angiogenesis and prognosis in invasive breast carcinoma. Cancer Res 1996; 56:4625-9; PMID:8840975
- Lissbrant IF, Stattin P, Wikstrom P, Damber JE, Egevad L, Bergh A. Tumor associated macrophages in human prostate cancer: relation to clinicopathological variables and survival. Int J Oncol 2000; 17:445-51; PMID:10938382; http://dx.doi.org/10.3892/ijo.17.3.445
- Zhu XD, Zhang JB, Zhuang PY, Zhu HG, Zhang W, Xiong YQ, Wu WZ, Wang L, Tang ZY, Sun HC. High expression of macrophage colony-stimulating factor in peritumoral liver tissue is associated with poor survival after curative resection of hepatocellular carcinoma. J Clin Oncol 2008; 26:2707-16; PMID:18509183; http://dx.doi.org/10.1200/JCO.2007.15.6521
- Dong Z, Kumar R, Yang X, Fidler IJ. Macrophage-derived metalloelastase is responsible for the generation of angiostatin in Lewis lung carcinoma. Cell 1997; 88:801-10; PMID:9118223; http://dx.doi.org/10.1016/S0092-8674(00)81926-1
- Tsung K, Dolan JP, Tsung YL, Norton JA. Macrophages as effector cells in interleukin 12-induced T cell-dependent tumor rejection. Cancer Res 2002; 62:5069-75; PMID:12208763
- De Palma M, Lewis CE. Macrophage regulation of tumor responses to anticancer therapies. Cancer Cell 2013; 23:277-86; PMID:23518347; http://dx.doi.org/10.1016/j.ccr.2013.02.013
- Ziegler-Heitbrock L, Ancuta P, Crowe S, Dalod M, Grau V, Hart DN, Leenen PJ, Liu YJ, MacPherson G, Randolph GJ et al. Nomenclature of monocytes and dendritic cells in blood. Blood 2010; 116:e74-80; PMID:20628149; http://dx.doi.org/10.1182/blood-2010-02-258558
- Wong KL, Tai JJ, Wong WC, Han H, Sem X, Yeap WH, Kourilsky P, Wong SC. Gene expression profiling reveals the defining features of the classical, intermediate, and nonclassical human monocyte subsets. Blood 2011; 118:e16-31; PMID:21653326; http://dx.doi.org/10.1182/blood-2010-12-326355
- Zawada AM, Rogacev KS, Rotter B, Winter P, Marell RR, Fliser D, Heine GH. SuperSAGE evidence for CD14++CD16+ monocytes as a third monocyte subset. Blood 2011; 118:e50-61; PMID:21803849; http://dx.doi.org/10.1182/blood-2011-01-326827
- Auffray C, Fogg D, Garfa M, Elain G, Join-Lambert O, Kayal S, Sarnacki S, Cumano A, Lauvau G, Geissmann F. Monitoring of blood vessels and tissues by a population of monocytes with patrolling behavior. Science 2007; 317:666-70; PMID:17673663; http://dx.doi.org/10.1126/science.1142883
- Cros J, Cagnard N, Woollard K, Patey N, Zhang SY, Senechal B, Puel A, Biswas SK, Moshous D, Picard C et al. Human CD14dim monocytes patrol and sense nucleic acids and viruses via TLR7 and TLR8 receptors. Immunity 2010; 33:375-86; PMID:20832340; http://dx.doi.org/10.1016/j.immuni.2010.08.012
- De Palma M, Venneri MA, Galli R, Sergi Sergi L, Politi LS, Sampaolesi M, Naldini L. Tie2 identifies a hematopoietic lineage of proangiogenic monocytes required for tumor vessel formation and a mesenchymal population of pericyte progenitors. Cancer Cell 2005; 8:211-26; PMID:16169466; http://dx.doi.org/10.1016/j.ccr.2005.08.002
- Schauer D, Starlinger P, Reiter C, Jahn N, Zajc P, Buchberger E, Bachleitner-Hofmann T, Bergmann M, Stift A, Gruenberger T et al. Intermediate monocytes but not TIE2-expressing monocytes are a sensitive diagnostic indicator for colorectal cancer. PLoS One 2012; 7:e44450; PMID:22973451; http://dx.doi.org/10.1371/journal.pone.0044450
- Gabrusiewicz K, Liu D, Cortes-Santiago N, Hossain MB, Conrad CA, Aldape KD, Fuller GN, Marini FC, Alonso MM, Idoate MA et al. Anti-vascular endothelial growth factor therapy-induced glioma invasion is associated with accumulation of Tie2-expressing monocytes. Oncotarget 2014; 5:2208-20; PMID:24809734; http://dx.doi.org/10.18632/oncotarget.1893
- Ibberson M, Bron S, Guex N, Faes-van't Hull E, Ifticene-Treboux A, Henry L, Lehr HA, Delaloye JF, Coukos G, Xenarios I et al. TIE-2 and VEGFR kinase activities drive immunosuppressive function of TIE-2-expressing monocytes in human breast tumors. Clin Cancer Res 2013; 19:3439-49; PMID:23649001; http://dx.doi.org/10.1158/1078-0432.CCR-12-3181
- Hu L, Roth JM, Brooks P, Luty J, Karpatkin S. Thrombin up-regulates cathepsin D which enhances angiogenesis, growth, and metastasis. Cancer Res 2008; 68:4666-73; PMID:18559512; http://dx.doi.org/10.1158/0008-5472.CAN-07-6276
- Rouzaut A, Lopez-Moratalla N, de Miguel C. Differential gene expression in the activation and maturation of human monocytes. Arch Biochem Biophys 2000; 374:153-60; PMID:10666293; http://dx.doi.org/10.1006/abbi.1999.1587
- Sithu SD, English WR, Olson P, Krubasik D, Baker AH, Murphy G, D'Souza SE. Membrane-type 1-matrix metalloproteinase regulates intracellular adhesion molecule-1 (ICAM-1)-mediated monocyte transmigration. J Biol Chem 2007; 282:25010-9; PMID:17591781; http://dx.doi.org/10.1074/jbc.M611273200
- Charo IF, Ransohoff RM. The many roles of chemokines and chemokine receptors in inflammation. N Engl J Med 2006; 354:610-21; PMID:16467548; http://dx.doi.org/10.1056/NEJMra052723
- Graves DT, Valente AJ. Monocyte chemotactic proteins from human tumor cells. Biochem Pharmacol 1991; 41:333-7; PMID:1994892; http://dx.doi.org/10.1016/0006-2952(91)90528-D
- Rossol M, Kraus S, Pierer M, Baerwald C, Wagner U. The CD14(bright) CD16+ monocyte subset is expanded in rheumatoid arthritis and promotes expansion of the Th17 cell population. Arthritis Rheum 2012; 64:671-7; PMID:22006178; http://dx.doi.org/10.1002/art.33418
- Lahiri R, Derwa Y, Bashir Z, Giles E, Torrance HD, Owen HC, O'Dwyer MJ, O'Brien A, Stagg AJ, Bhattacharya S et al. Systemic Inflammatory Response Syndrome After Major Abdominal Surgery Predicted by Early Upregulation of TLR4 and TLR5. Ann Surg 2016; 263:1028-37; PMID:26020106; http://dx.doi.org/10.1097/sla.0000000000001248
- Sanford DE, Belt BA, Panni RZ, Mayer A, Deshpande AD, Carpenter D, Mitchem JB, Plambeck-Suess SM, Worley LA, Goetz BD et al. Inflammatory monocyte mobilization decreases patient survival in pancreatic cancer: a role for targeting the CCL2/CCR2 axis. Clin Cancer Res 2013; 19:3404-15; PMID:23653148; http://dx.doi.org/10.1158/1078-0432.CCR-13-0525
- Matsubara T, Kanto T, Kuroda S, Yoshio S, Higashitani K, Kakita N, Miyazaki M, Sakakibara M, Hiramatsu N, Kasahara A et al. TIE2-expressing monocytes as a diagnostic marker for hepatocellular carcinoma correlates with angiogenesis. Hepatology 2013; 57:1416-25; PMID:22815256; http://dx.doi.org/10.1002/hep.25965
- Shojaei F, Wu X, Malik AK, Zhong C, Baldwin ME, Schanz S, Fuh G, Gerber HP, Ferrara N. Tumor refractoriness to anti-VEGF treatment is mediated by CD11b+Gr1+ myeloid cells. Nat Biotechnol 2007; 25:911-20; PMID:17664940; http://dx.doi.org/10.1038/nbt1323
- Shojaei F, Wu X, Qu X, Kowanetz M, Yu L, Tan M, Meng YG, Ferrara N. G-CSF-initiated myeloid cell mobilization and angiogenesis mediate tumor refractoriness to anti-VEGF therapy in mouse models. Proc Natl Acad Sci U S A 2009; 106:6742-7; PMID:19346489; http://dx.doi.org/10.1073/pnas.0902280106
- Schauer D, Starlinger P, Zajc P, Alidzanovic L, Maier T, Buchberger E, Pop L, Gruenberger B, Gruenberger T, Brostjan C. Monocytes with angiogenic potential are selectively induced by liver resection and accumulate near the site of liver regeneration. BMC Immunol 2014; 15:50; PMID:25359527; http://dx.doi.org/10.1186/s12865-014-0050-3
- Buchberger E, El Harchi M, Payrhuber D, Zommer A, Schauer D, Simonitsch-Klupp I, Bilban M, Brostjan C. Overexpression of the transcriptional repressor complex BCL-6/BCoR leads to nuclear aggregates distinct from classical aggresomes. PLoS One 2013; 8:e76845; PMID:24146931; http://dx.doi.org/10.1371/journal.pone.0076845
- Moll HP, Freudenthaler H, Zommer A, Buchberger E, Brostjan C. Neutralizing type I IFN antibodies trigger an IFN-like response in endothelial cells. J Immunol 2008; 180:5250-6; PMID:18390705; http://dx.doi.org/10.4049/jimmunol.180.8.5250