ABSTRACT
Growing evidence indicates that adipose tissue inflammation is an important mechanism whereby obesity promotes cancer risk and progression. Since IL-32 is an important inflammatory and remodeling factor in obesity and is also related to colon cancer (CC) development, the aim of this study was to explore whether IL-32 could function as an inflammatory factor in human obesity-associated CC promoting a microenvironment favorable for tumor growth. Samples obtained from 84 subjects [27 lean (LN) and 57 obese (OB)] were used in the study. Enrolled subjects were further subclassified according to the established diagnostic protocol for CC (49 without CC and 35 with CC). We show, for the first time, that obesity (p = 0.009) and CC (p = 0.026) increase circulating concentrations of IL-32α. Consistently, we further showed that gene (p < 0.05) and protein (p < 0.01) expression levels of IL-32α were upregulated in VAT from obese patients with CC. Additionally, we revealed that IL32 expression levels are enhanced by hypoxia and inflammation-related factors in HT-29 CC cells as well as that IL-32α is involved in the upregulation of inflammation (IL8, TNF, and CCL2) and extracellular matrix (ECM) remodeling (SPP1 and MMP9) genes in HT-29 cancer cells. Additionally, we also demonstrate that the adipocyte-conditioned medium obtained from obese patients stimulates (p < 0.05) the expression of IL32 in human CC cells. These findings provide evidence of the potential involvement of IL-32 in the development of obesity-associated CC as a pro-inflammatory and ECM remodeling cytokine.
Introduction
Although colorectal cancer (CRC) incidence has been declining over the past 20 y, it is still the second leading cause of cancer, prompting a significant morbidity and mortality.Citation1-3 A connection between inflammation and CRC is well established,Citation4 and in the last decade the role of distinct pro-inflammatory factors, cytokines, and other immune mediators have received a great deal of interest in the promotion and progression of CRC.Citation5-7 In this regard, the characteristic chronic low-grade inflammatory state associated to obesity constitutes an important link between excess adiposity and CRC development. Epidemiological data evidence a significant association between increased body mass index (BMI) and several major cancersCitation8-11 and specifically, more than 10% of cancers of the colon have been attributed to excess weight.Citation8
The molecular mechanisms by which obesity-associated inflammation promotes CRC development are still being uncovered but recent works have elucidated that the adipose tissue is a highly active endocrine organ that secretes a variety of adipokines with important roles in tumor initiation and progression.Citation12-18 In this sense, our group has identified that the elevated levels of the recently described cytokine interleukin (IL)-32 in obesity promote adipose tissue inflammation and the remodeling of the extracellular matrix (ECM), suggesting its involvement in the development of obesity-associated comorbidities.Citation19 IL-32 is produced by immune and non-immune cells including natural killer cells, monocytes, macrophages, T-lymphocytes, fibroblasts as well as epithelial and endothelial cells.Citation20-22 Previous studies have demonstrated that different cytokines such as IL-1β, interferon-gamma (IFNγ) or tumor necrosis factor-alpha (TNF-α) induce the expression of IL-32 and, in turn, IL-32 also stimulates IL-8, IL-6, IL-1β, and TNF-α production in different cellular types via the activation of nuclear factor κB subunit 1 (NF-κB) and p38 mitogen-activated protein (MAP) kinase,Citation19,23-25 constituting a classical pro-inflammatory mediator with relevant functions in angiogenesis, ECM remodeling and apoptosis.Citation26
In recent years, the role of IL-32 in certain forms of cancers has been reportedCitation21 with its expression being altered in different forms of gastric,Citation27 lung,Citation28 breast,Citation29 colonCitation30 or liverCitation31 cancer. However, the results are contradictory with IL-32 exhibiting an oncogenic or a tumor suppressive role mainly due to the specific activities and properties of its nine isoforms generated by mRNA alternative splicing.Citation23,32,33 Regarding CRC, IL-32 expression has been correlated with both lymph node and organic metastasis developmentCitation30 and a role in the regulation of the cancer stem cell-like properties promoting tumorigenesis of CRC in an autocrine and paracrine manner has been also described.Citation34 In this sense, the epithelial expression and circulating levels of IL-32α were increased in patients with ulcerative colitis and Crohn's disease.Citation25,35,36 In addition, IL-32 is a potent inducer of prostaglandin E2 release, an important factor for inflammationCitation23,37 and for the promotion of colon cancer (CC) cell growth.Citation38 On the contrary, an enhancement of TNF-α-induced cell death in cases of CC as well as an inhibition of cancer cell growth by blocking the NF-κB and the signal transducer and activator of transcription 3 (STAT3) pathways by IL-32γ has been also reported.Citation39,40
Since IL-32 is reportedly an important inflammatory and remodeling factor in obesityCitation19 and is also related to CRC development, we hypothesized that the increased levels of IL-32 in obese patients may function as a link between obesity and CC development. Therefore, the aim of the present study was to investigate whether obesity can influence the circulating and expression levels of IL-32 in patients with CC, thereby promoting a microenvironment favorable for tumor growth. To gain insight into the molecular mechanisms involved, the effect of pro- and anti-inflammatory factors on the expression levels of IL32 in cultures of human CC cells was further explored, and finally we also aimed to investigate whether IL-32 itself can regulate the inflammatory response in human CC cells.
Results
Obesity and colon cancer increase circulating concentrations and expression levels of IL-32α
Baseline characteristics of the study sample are shown in . As expected, markers of adiposity were higher (p < 0.001) in obese individuals compared with the lean volunteers. No differences in anthropometric measurements were detected between patients with or without CC. Obese patients with CC showed increased (p < 0.001) levels of CRP compared with lean and obese patients without CC. Moreover, fibrinogen concentrations were higher (p < 0.01) in obese patients with CC compared with obese patients without CC. No differences were found in the global WBC regarding obesity but patients with CC exhibited an elevated number of leucocytes (p < 0.05) compared with those without CC. Significant differences in circulating IL-32α concentrations between the experimental groups were observed, being significantly increased due to obesity (p = 0.009) and CC (p = 0.026) (). No sexual dimorphism was found in plasma IL-32α concentrations [(male: 129.9 ± 15.9 arbitrary units (a.u.) and female: 125.0 ± 18.7 a.u.; p = 0.964)].
Table 1. Anthropometric and biochemical characteristics of the subjects included in the study.
Figure 1. IL-32 levels in obesity and colon cancer (CC). (A) Circulating concentrations of IL-32α of lean (LN) and obese (OB) volunteers classified according to the presence or not of CC. Gene (B) and protein levels (C) of IL32 in visceral adipose tissue (VAT). The gene and protein expression in patients without CC was assumed to be 1. Representative blots are shown at the bottom of the histograms. The intensity of the bands was normalized with total protein values. (D) Immunohistochemical detection of IL-32α in histological sections of human VAT. Representative images of at least three separate experiments are shown. (E) Gene expression levels of IL32 in small intestine from OB volunteers classified into three groups [normoglycemia (NG), impaired glucose tolerance (IGT) and type 2 diabetes (T2D)]. Differences between groups were analyzed by two-way ANCOVA, one way ANCOVA as well as by two-tailed unpaired Student's t tests, where appropriate. Bars represent the mean ± SEM. *p < 0.05 and **p < 0.01 vs patients without CC. ††p < 0.01 vs OB-NG and ‡‡p < 0.01 vs OB-IGT.
![Figure 1. IL-32 levels in obesity and colon cancer (CC). (A) Circulating concentrations of IL-32α of lean (LN) and obese (OB) volunteers classified according to the presence or not of CC. Gene (B) and protein levels (C) of IL32 in visceral adipose tissue (VAT). The gene and protein expression in patients without CC was assumed to be 1. Representative blots are shown at the bottom of the histograms. The intensity of the bands was normalized with total protein values. (D) Immunohistochemical detection of IL-32α in histological sections of human VAT. Representative images of at least three separate experiments are shown. (E) Gene expression levels of IL32 in small intestine from OB volunteers classified into three groups [normoglycemia (NG), impaired glucose tolerance (IGT) and type 2 diabetes (T2D)]. Differences between groups were analyzed by two-way ANCOVA, one way ANCOVA as well as by two-tailed unpaired Student's t tests, where appropriate. Bars represent the mean ± SEM. *p < 0.05 and **p < 0.01 vs patients without CC. ††p < 0.01 vs OB-NG and ‡‡p < 0.01 vs OB-IGT.](/cms/asset/8ebc2059-2a08-45f1-9e23-69c823ba954b/koni_a_1328338_f0001_oc.gif)
Since plasma levels of IL-32α are increased by both obesity and CC, we further investigated gene and protein expression of IL-32α in samples of VAT based on the relevance of this adipose tissue depot in obesity-associated inflammation and colon carginogenesis. Obese patients with CC showed increased gene (p < 0.05) and protein (p < 0.01) expression levels of IL-32α compared with obese patients without CC ( and ). To gain further insight into the presence of IL-32 in VAT, its localization was confirmed by immunohistochemistry (). Both adipocytes and SVFC were immunopositive for IL-32α, although a strong staining in SVFC was observed and an enhanced expression was also detected in patients with CC.
In light of the significance of IL-32 in obese patients with CC and the potential role of insulin resistance in the development of CC, we also evaluated its gene expression levels in small intestine samples from obese patients, being significantly higher (p < 0.01) in obese patients with type 2 diabetes compared with both impaired glucose tolerant and normoglycemic obese patients ().
Increased circulating levels of inflammation and ECM remodeling markers in obesity and CC are related to IL-32α
Although the detailed mechanisms that mediate the obesity-driven effect on cancer development in humans are still poorly understood, chronic inflammation is known to participate in cancer progression. In this regard, significant differences in circulating IL-6 concentrations between the experimental groups were observed, being significantly increased due to obesity (p = 0.002) and CC (p < 0.001) (). In this sense, obese patients with CC exhibited higher (p = 0.026) plasma levels of IL-8 compared with normoponderal patients with CC (). Circulating levels of the anti-inflammatory cytokine IL-4 were also affected by obesity (p = 0.038) and CC (p = 0.046) but in an opposite way, exhibiting decreased circulating levels in obese and CC patients (). IL-13 concentrations were diminished (p = 0.016) in obese patients, but no differences were detected regarding the presence or not of CC (). Importantly, circulating levels of IL-32α were significantly associated with the concentrations of the pro-inflammatory cytokine IL-6 (r = 0.37; p = 0.046).
Figure 2. Impact of obesity and colon cancer (CC) on inflammation. Circulating concentrations of (A) interleukin (IL)-6, (B) IL-8, (C) IL-4 and (D) IL-13 of lean (LN) and obese (OB) volunteers classified according to the presence or not of CC. Bars represent the mean ± SEM. Differences between groups were analyzed by two-way ANCOVA or one way ANCOVA followed by Tukey's tests in the case of IL-8 due to interaction (*p < 0.05 vs LN non-CC).
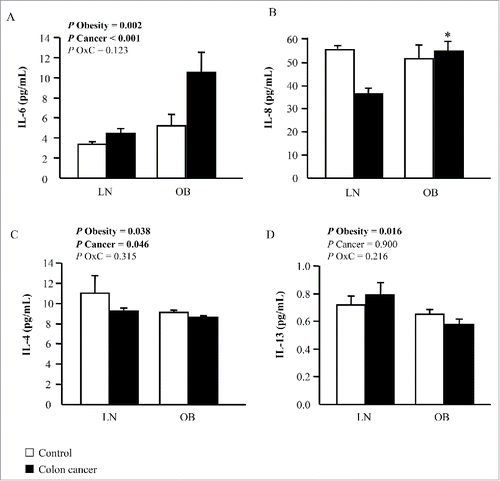
Moreover, changes in the ECM composition of adipose tissue in obesity greatly influence the metabolism and growth of tumor cells, providing a favorable area for tumorigenesis. In this regard, we found that obesity significantly increased (p < 0.05) plasma levels of VEGFA and WISP1 and that patients with CC showed decreased (p = 0.021) circulating concentrations of SPARC (). OPN concentrations were significantly increased due to both obesity (p < 0.01) and CC (p < 0.05). We also found a positive correlation of IL-32α concentrations with serum levels of WISP1 (r = 0.58; p = 0.007) and OPN (r = 0.40; p = 0.043) as well as a negative association with SPARC concentrations (r = −0.60; p = 0.008).
Figure 3. Effect of obesity and colon cancer (CC) on extracellular matrix remodeling factors. Circulating concentrations of (A) vascular endothelial growth factor A (VEGFA), (B) WNT1 inducible signaling pathway protein 1 (WISP1), (C) secreted protein acidic and cysteine rich (SPARC) and (D) secreted phosphoprotein 1 [osteopontin (OPN)] of lean (LN) and obese (OB) volunteers classified according to the presence or not of CC. Bars represent the mean ± SEM. Differences between groups were analyzed by two-way ANCOVA.
![Figure 3. Effect of obesity and colon cancer (CC) on extracellular matrix remodeling factors. Circulating concentrations of (A) vascular endothelial growth factor A (VEGFA), (B) WNT1 inducible signaling pathway protein 1 (WISP1), (C) secreted protein acidic and cysteine rich (SPARC) and (D) secreted phosphoprotein 1 [osteopontin (OPN)] of lean (LN) and obese (OB) volunteers classified according to the presence or not of CC. Bars represent the mean ± SEM. Differences between groups were analyzed by two-way ANCOVA.](/cms/asset/89b6d8ed-94d9-47d1-b4a9-c4df11010048/koni_a_1328338_f0003_b.gif)
Inflammatory-related factors and hypoxia increase IL32 gene expression levels in HT-29 cells
We next evaluated if well-known inflammatory factors that are increased in obesity influence IL32 expression in HT-29 cells ( and ). TNF-α treatment dramatically increased (p < 0.001) the mRNA levels of IL32 and, in the same line, gene expression levels of IL32 were induced by LPS (p < 0.01). No significant changes were observed on IL32 expression in HT-29 cells after their stimulation with the anti-inflammatory cytokines IL-4 and IL-13 ( and ). Next, we showed that gene expression levels of IL32 exhibited a strong upregulation (p < 0.001) in HT-29 cells under hypoxia mimicked by the incubation with the divalent transition-metal ion cobalt at 100 mM ().
Figure 4. Impact of inflammation-related factors and hypoxia on IL32 gene expression levels in HT-29 colon cancer cells. Bar graphs show the effect of TNF-α (A), LPS (B), IL-13, (C), IL-4 (D), and CoCl2 (E) incubated for 24 h on the transcript levels of IL32 in HT-29 cells. Gene expression levels in unstimulated cells were assumed to be 1. Values are the mean ± SEM (n = 6 per group). Differences between groups were analyzed by one-way ANOVA followed by Dunnet's tests. *p < 0.05, **p < 0.01 and ***p < 0.001 vs unstimulated cells.
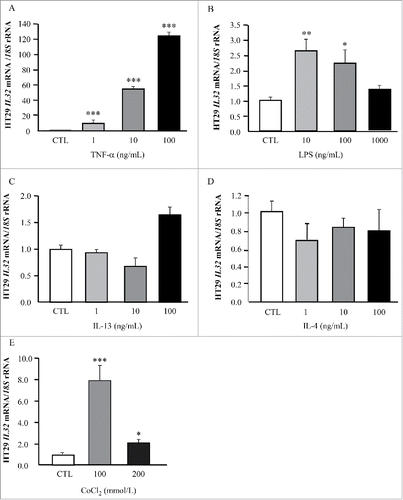
IL-32α induces the expression of inflammation and ECM remodeling markers in HT-29 cells
We further explored whether IL-32α itself can activate the expression of genes involved in the inflammatory response and ECM remodeling in HT-29 cells. Cells were stimulated with increasing concentrations of IL-32α for 24 h. As shown in , IL-32α treatment significantly enhanced the mRNA levels of the inflammatory factors TNF (p < 0.01), CCL2 (p < 0.05) and IL8 (p < 0.05). We also detected a strong upregulation (p < 0.001) of the ECM remodeling genes SPP1 and MMP9 after IL-32α treatment (). Unexpectedly, no differences were found in the regulation of IL1B and VEGFA after treatment with IL-32α.
Figure 5. Effect of IL-32α treatment on the expression of inflammatory and ECM remodeling markers in HT-29 cells. Gene expression levels of pro-inflammatory markers as well as extracellular matrix remodeling-related molecules in HT-29 cells stimulated with recombinant IL-32α (1, 10, and 100 nmol/L) for 24 h. Gene expression levels in unstimulated cells were assumed to be 1. Values are the mean ± SEM (n = 6 per group). Differences between groups were analyzed by one-way ANOVA followed by Dunnet's tests. *p < 0.05, **p < 0.01, and ***p < 0.001 vs unstimulated cells.
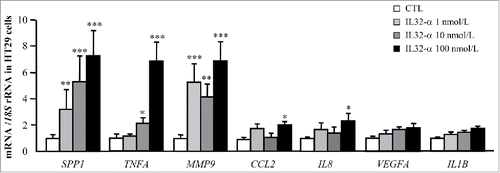
We also explored the impact of IL-32α on the modulation of the expression of specific key genes controlling cell proliferation, growth and adhesion in CC. In this sense, IL-32α treatment significantly reduced gene expression levels of the tumor suppressor gene APC (p < 0.05) and increased mRNA levels of CTNNB1 (p < 0.05). However, no significant differences in the expression levels of KLF4, COX2, HIF1A, TGFB, and MUC2 were found after treatment with IL-32α (Fig. S1).
ACM upregulates gene expression levels of IL32 in HT-29 cells
To explore molecular mechanisms behind the adipocyte-tumoral cell crosstalk, we analyzed the effect of ACM in the expression of IL32 in HT-29 cells. Interestingly, a significant increase (p < 0.05) in the expression levels of IL32 in HT-29 cells preincubated with the adipocyte-derived factors obtained from obese volunteers compared with the tumoral cells treated with control media was observed ().
Figure 6. Adipocyte-conditioned media (ACM) induces gene expression levels of IL32 in HT-29 cells. Bar graphs show the effect of adipocyte CM (20% and 40%) from obese subjects incubated for 24 h on the transcript levels of IL32 in HT-29 cells. Values are the mean ± SEM (n = 6 per group). Differences between groups were analyzed by one-way ANOVA followed by Dunnet's tests. *p < 0.05 vs unstimulated cells.
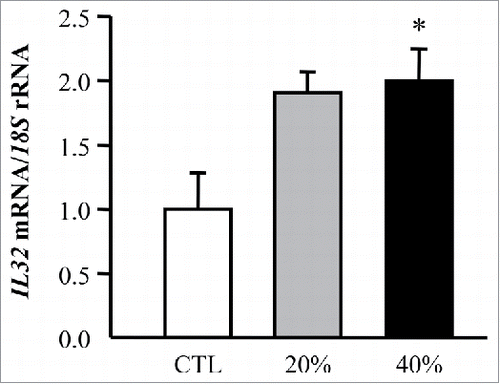
Discussion
Growing evidence indicates that inflammation is a central mechanism through which obesity promotes cancer risk and progression.Citation12,41 In addition to the tumor-promoting effects of systemically dysregulated adipokines as well as metabolic and inflammatory mediators, obesity also promotes tumor progression at the local level via adipose tissue inflammation and associated alterations in the microenvironment.Citation14,41 In this context, increased levels of the pro-inflammatory cytokine IL-32 have been found in obesityCitation19 as well as in several major cancers.Citation27,29,31 The present study provides evidence that the increased IL-32 expression in obesity is related to CC development. In this regard, we found, for the first time, that both obesity and CC increased circulating levels of IL-32. Consistently, we further showed that the IL32 gene and protein expression levels in VAT were also upregulated in obese patients with CC. Additionally, we revealed that IL32 expression levels are regulated by hypoxia and inflammation-related factors in human CC cells as well as that IL-32 is involved in enhancing inflammation and ECM remodeling. Subsequently, we also demonstrate that ACM stimulates the expression of IL32 in human CC cells.
IL-32 is a multifaceted cytokine with relevant cell functions; inducer of proinflammatory cytokines,Citation24 apoptosis,Citation42 and differentiation.Citation43 In the present study, we have found that circulating concentrations of IL-32α are increased due to both obesity and CC, suggesting a link between obesity and this relevant comorbidity. Increased circulating levels of IL-32 have been described in different tumoral typesCitation27-29 although results are contradictory because of the different activity of the major isoforms of IL-32. In this sense, IL32-β and IL-32γ have been related to caspase-8- and caspase-3-induced apoptosis whereas this effect was not observed for the IL-32α isoform.Citation21,32,44 Importantly, IL-32β-induced cell death can be rescued through an enhanced expression of IL-8, a cytokine involved in cell survival whereas IL-32γ, the most potent isoform, has the capacity to block the IL-8-survival signaling pathway.Citation32 An anticancer activity of IL-32γ has been described to induce CC cell death in vitro and tumor regression in vivo by enhancing TNF-α expressionCitation40 and by decreasing the activity of the STAT3 pathway, highly involved in the inflammation-associated carcinogenesis in CC.Citation39 However, an induction of metastasis by the overexpression of IL-32 in CRC has been observedCitation30 and a marked expression of IL-32α has been found in colon mucosa from patients with different inflammatory bowel diseases such as ulcerative colitis and Crohn's disease.Citation36 This paradoxical effect has been explained in transgenic mice expressing human IL-32γ, in which the exacerbated acute inflammation found in early stages of the development of ulcerative colitis is reduced after later stages of the disease.Citation35 IL-32γ is able to increase IL-10 expression, which is involved in the decrease of TNF-α, IL-6 and IL-32 itself, causing a decrease in the inflammatory state.Citation35 Therefore, IL-32γ may be viewed as a cytokine that worsens as well as protects the intestinal integrity.
High circulating levels and expression of IL-32 have been associated with a poor prognosis of gastric cancer mainly as a result of an induction of inflammatory and remodeling factors.Citation27,45 In this context, obese patients with CC included in our study also showed increased levels of the relevant inflammatory markers IL-6, IL-8, and OPN and their strong association with IL-32 levels underscores the involvement of this cytokine in obesity- and CC-associated inflammation. Obese patients showed increased levels of WISP1, a novel adipokine linking obesity to inflammation and insulin resistance.Citation46 The association between IL-32 and WISP1 found in our study strengthens the involvement of IL-32 in obesity-associated inflammation. We also showed reduced levels of SPARC in patients with CC as well as a negative association between SPARC and IL-32. Although the role of SPARC in cancer remains controversial and it is highly cancer-type dependent, SPARC has been considered to be a tumor suppressor in CC,Citation47,48 suggesting a role for IL-32 in colon carcinogenesis.
Adipose tissue represents an extremely active endocrine organ.Citation49 The expanded and dysfunctional adipose tissue in obesity plays an important role for tumor development due to its ability to secrete inflammatory cytokines.Citation12,14,50 In our study, obese patients with CC exhibited increased gene and protein expression levels of IL-32 in VAT compared with obese patients without CC, suggesting that VAT in obesity might be involved in establishing a microenvironment favorable for promoting CC growth and progression through the expression of IL-32. In this regard, diverse dysregulated adipokines and inflammatory factors synthesized and secreted by the adipose tissue in obesity have been linked to CC development and progression including leptin, adiponectin, tenascin C, OPN, IL-6, VEGF, or MMP-9.Citation13,51,52
To investigate regulatory mechanisms underlying IL-32α induction, we used HT-29 cells, a well-characterized and frequently used model of intestinal epithelial cells. Stimulation with LPS and TNF-α enhanced IL32 mRNA expression with a dramatic effect being observed for TNF-α, in agreement with the previous results in another pathologic condition.Citation36 Importantly, hypoxia, a hallmark of cancer and a well-known inducer of inflammation, also increased IL32 gene expression levels in HT-29 cells. In this line, hypoxic conditions reportedly enhanced IL-32β production in breast cancer cells being associated with an increased glycolysis, and thus with the regulation of cancer cell metabolism.Citation53
A typical cytokine receptor for IL-32 has not been identified but proteinase 3 (PR3) as well as αVβ3 and αVβ6 integrins has been reported as IL-32 binding proteins.Citation26,54 IL-32 also acts in a synergistic manner with the nucleotide binding oligomerization domain containing (NOD) pathways for the release of potent pro-inflammatory cytokines aggravating the colon mucosa inflammation.Citation36,55 Data from this study indicate that IL-32 triggers inflammation and cell invasion partially by inducing TNF, CCL2, IL8, SPP1, and MMP9 expression, resulting in the remodeling of the ECM. These results are in line with the previous findings showing that IL-32 is associated with acquisition of an invasive and metastasis phenotype in lung and gastric cancer partly via the MMP-2 and MMP-9 upregulation.Citation27,28,56 Moreover, IL-32 has been described as a factor that promotes angiogenesis mediated by integrin αVβ3 and VEGF-independent in endothelial cells.Citation26 APC and β-catenin are critical in the early stages of colon tumorigenesis. The inactivation or the loss of tumor suppression function of APC has been described as a key and early event observed in CC development and the activation of β-catenin leads to the upregulation of downstream targets with important roles in cell proliferation.Citation57 In this regard, we found reduced gene expression levels of APC and increased mRNA levels of CTNNB1 after IL-32α treatment, suggesting a potential involvement of this cytokine in the early stages of CC development.
A bidirectional cross-talk between tumor-surrounding adipocytes and cancer cells has been described.Citation58 Therefore, we evaluated the effect of ACM obtained from obese volunteers on HT-29 cells. An increase in the expression levels of IL32 was observed, strengthening the interaction between adipocytes and tumoral cells and the contribution of adipocytes to tumor progression probably by inducing inflammation and modifying cancer cell behavior.
A potential limitation of the study is the classification of the volunteers according to BMI, which may misclassify obesity in some subjects with a proportion of obese individuals by body fat actually not being identified.Citation59,60 Body composition assessment was not available for all the subjects included in the study. Therefore, to reduce the potential error, we estimated adiposity by using CUN-BAE, a validated tool designed by our group precisely to be applied for estimating body adiposity in adults in these circumstances with low error rate and good accuracy.Citation61
Inflammatory pathways have emerged as promising targets for cancer therapy. Therefore, new biomarkers of adipose tissue inflammation to identify obese patients at increased risk for cancer development could represent novel therapeutic targets. In the present study, the upregulated levels of IL-32 in patients with obesity and CC as well as its capacity to induce pro-inflammatory and ECM remodeling genes suggest the involvement of IL-32 in the development of obesity-associated CC. Further studies in larger cohorts to better understand the implication of IL-32 in obesity-associated CC are warranted. Furthermore, the thorough characterization of IL-32 isoforms and the possibility to modulate their expression in a tissue-specific way may also represent a novel strategy in cancer therapies.
Material and methods
Patient selection
Blood samples from 84 subjects [27 lean (LN) and 57 obese (OB)] recruited from healthy volunteers and patients attending the Departments of Endocrinology & Nutrition and Surgery at the Clínica Universidad de Navarra were used in the study. Volunteers underwent a clinical assessment including medical history, physical examination and co-morbidity evaluation by a multidisciplinary consultation team. Patients were classified as LN or OB according to BMI (LN: BMI < 25 kg/m2 and OB: BMI ≧ 30 kg/m2). BMI was calculated as weight in kilograms divided by the square of height in meters and body fat percentage (BF) was estimated using the Clinica Universidad de Navarra-Body Adiposity Estimator (CUN-BAE).Citation61 Waist circumference was measured at the midpoint between the iliac crest and the rib cage on the midaxillary line. Enrolled subjects were further subclassified according to the established diagnostic protocol for CC [49 without CC (non-CC) and 35 with CC]. Clinicopathological characteristics of the subjects with CC included in the study are shown in Table S1.
Adipose tissue samples were collected from patients undergoing Nissen fundoplication (for LN volunteers), Roux-en-Y gastric bypass [RYGB (for morbid obesity treatment in OB subjects)] and curative resection for primary colon carcinoma (for CC treatment) at the Clínica Universidad de Navarra. In addition, an intraoperative biopsy from the small intestine was performed in the obese patients during RYGB to analyze IL32 gene expression levels. The control volunteers were healthy, were not on medication, and had no signs or clinical symptoms of cancer, liver alteration or type 2 diabetes. The experimental design was approved, from an ethical and scientific standpoint, by the Hospital's Ethical Committee responsible for research and the written informed consent of participants was obtained.
Analytical procedures
Plasma samples were obtained by venipuncture after an overnight fast to avoid potential confounding influences due to hormonal rhythmicity. Glucose was analyzed by an automated analyzer (Hitachi Modular P800, Roche, Basel, Switzerland). Serum concentrations of triglycerides and free fatty acids (FFA) were measured by enzymatic methods using commercially available kits (Infinity™, Thermo Electron Corporation, Melbourne, Australia). The carcinoembryonic antigen (CEA), high sensitivity C-reactive protein (CRP) and fibrinogen concentrations were determined as previously reported.Citation13 White blood cell (WBC) count was measured using an automated cell counter (Beckman Coulter, Inc., Fullerton, CA). Commercially available ELISA kits were used to assess circulating levels of IL-4, IL-6, IL-8, IL-13, secreted protein acidic and cysteine rich (SPARC), secreted phosphoprotein 1 [osteopontin (OPN)], vascular endothelial growth factor A (VEGFA) and WNT1 inducible signaling pathway protein 1 (WISP1) (RayBiotech, Inc., Norcross, GA), according to the manufacturer's instructions. The intra- and inter-assay coefficients of variation were <10 and <12% for all analyzed molecules.
Real-time PCR
The transcript levels for adenomatosis polyposis coli tumor suppressor (APC), β-catenin (CTNNB1), monocyte chemoattractant protein-1 (CCL2), cyclooxigenase-2 (COX2), hypoxia-inducible factor 1α (HIF1A), IL1B, IL8, IL32, krüppel-like factor 4 (KLF4), matrix metalloproteinase 9 (MMP9), mucin 2 (MUC2), secreted phosphoprotein 1 (SPP1), transforming growth factor β (TGFB), TNF, and VEGFA were quantified by Real-Time PCR (7300 Real Time PCR System, Applied Biosystems, Foster City, CA) as described previously.Citation14 Primers and probes (Table S2) were designed using the software Primer Express 2.0 (Applied Biosystems) and purchased from Genosys (Sigma-Aldrich, Madrid, Spain). Primers or TaqMan® probes encompassing fragments of the areas from the extremes of two exons were designed to ensure the detection of the corresponding transcript avoiding genomic DNA amplification.
Western blot studies
Visceral (VAT) adipose tissue were homogenized and protein content was measured as described previously.Citation62 Adipose tissue (30 μg) or diluted (1:100) blood samples were run out in Any kD™ Mini-Protean® TGX™ precast polyacrylamide gels (Bio-Rad Laboratories, Inc., Hercules, CA), subsequently transferred to nitrocellulose membranes and blocked in Tris-buffered saline (TBS) (50 mmol/L Tris, 0.5 mol/L NaCl, pH 7.36) with 0.05% Tween 20 containing 5% non-fat dry milk for 1 h at room temperature (RT).
Blots were incubated with a rat monoclonal anti-human IL-32α antibody (R&D Systems, Minneapolis, MN) diluted 1:10,000. The antigen–antibody complexes were visualized using horseradish peroxidase-conjugated anti-mouse IgG antibodies (1:20,000) and the enhanced chemiluminescence Pierce ECL Plus Western Blotting Substrate (Thermo Scientific, Rockford, IL). The intensity of the bands was determined by densitometric analysis with the ChemiDoc™ MP imagining system and the Image Lab 4.0.1 software (Bio-Rad) and normalized with total protein values. All assays were performed in duplicate.
Histological analysis of IL-32α
Sections (6 μm) of formalin- fixed paraffin-embedded VAT were dewaxed in xylene, rehydrated in decreasing concentrations of ethanol and treated with 3% H2O2 (Sigma) in absolute methanol for 10 min at RT to quench endogenous peroxidase activity. Then, slides were blocked during 1 h with 1% BSA (Sigma) diluted in TBS to prevent non-specific adsorption. Sections were incubated overnight at 4°C with a rat monoclonal anti-human IL-32α antibody (R&D Systems, Minneapolis, MN) diluted 1:50 in TBS. After washing with TBS, slides were incubated with Dako Real™ EnVision™ HRP-conjugated anti-mouse (Dako, Glostrup, Denmark) for 1 h at RT. After washing in TBS, the peroxidase reaction was visualized with a 3,3′-diaminobenzidine (DAB, Amersham Biosciences, Buckinghamshire, UK)/H2O2 solution (0.5 mg/mL DAB, 0.03% H2O2 diluted in 50 mmol/L Tris–HCl, pH 7.36), as chromogen and Harris hematoxylin solution (Sigma) as counterstaining. Finally, sections were dehydrated, mounted using DePeX mounting medium (Serva, Heidelberg, Germany) and observed under a Zeiss Axiovert CFL optic microscope (Zeiss, Göttingen, Germany). Negative control slides without primary antibody were included to assess non-specific staining.
Adipocyte and HT-29 cell cultures
Human stromovascular fraction cells (SVFCs) were isolated from omental adipose tissue from obese normoglycemic subjects as described previously.Citation62 SVFC were seeded at 2 × 105 cell/well and grown in adipocyte medium [DMEM/F-12 [1:1] (Invitrogen), 17.5 mol/L glucose, 16 μmol/L biotin, 18 μmol/L panthotenate, 100 μmol/L ascorbate, and antibiotic–antimycotic] supplemented with 10% newborn calf serum (NCS). After 4 d, the medium was changed to adipocyte medium supplemented with 3% NCS, 0.5 mmol/L 3-isobutyl-1-methylxanthine (IBMX), 0.1 μmol/L dexamethasone, 1 μmol/L BRL49653 and 10 μg/mL insulin. After a 3-d induction period, cells were fed every 2 d with the same medium but without IBMX and BRL49653 supplementation for the remaining 7 d of adipocyte differentiation. Adipocyte-conditioned media (ACM) was prepared by collecting the supernatant from differentiated adipocytes. The ACM was then centrifuged, diluted (20% and 40%) and frozen at −80°C.
The intestinal epithelial cell line HT-29, derived from a human colorectal adenocarcinoma, was obtained from ATCC® (HTB-38™, Middlesex, UK) and cultured according to the manufacturer's instructions. Briefly, cells were seeded at 3 × 105 cells/well and grown in McCoy's 5A medium with L-glutamine (Sigma) supplemented with 10% fetal bovine serum and antibiotic-antimicotic at 37°C for 24 h. HT-29 cells were serum-starved for 24 h and then treated with increasing concentrations of LPS (10, 100, and 1,000 ng/mL) (Sigma), TNF-α (1, 10, and 100 ng/mL) (Sigma), IL-4 (10, 100, and 1,000 ng/mL) (R&D Systems), IL-13 (10, 100, and 1,000 ng/mL) (R&D Systems), IL-32α (R&D systems) (10, 100, and 1,000 ng/mL), CoCl2 (100 and 200 nmol/L) or ACM (20% and 40%) for 24 h.
Statistical analysis
Data are presented as mean ± standard error of the mean (SEM). Due to their non-normal distribution CRP concentrations were logarithmically transformed. The normal distribution of the other variables was adequate for the use of parametric tests. Differences between groups were assessed by two-way ANOVA and one-way ANOVA followed by Tukey's or Dunnet's post-hoc tests as appropriate. Differences between groups adjusted for age were analyzed by analysis of covariance (ANCOVA). Associations between two variables were computed by Pearson (r) correlation coefficient. Multiple linear regression analysis was performed to evaluate the independent relationship of the studied variables. The calculations were performed using the SPSS/Windows version 15.0 statistical package (SPSS, Chicago, IL). A p value < 0.05 was considered statistically significant.
Disclosure of potential conflicts of interest
No potential conflicts of interest were disclosed.
Author contributions
V.C. designed the study, collected, and analyzed data, wrote the first draft of the manuscript, contributed to discussion, and reviewed the manuscript. J.G.-A., A.R., and S.B. collected and analyzed data, contributed to discussion, and reviewed the manuscript. J.L.H.-L., J.B., F.R., V.V., and J.S. enrolled patients, collected data, contributed to discussion, and reviewed the manuscript. B.R., V.A.O., R.M., and C.S. collected data, contributed to discussion, and reviewed the manuscript. G.F. designed the study, enrolled patients, collected and analyzed data, wrote the first draft of the manuscript, contributed to discussion, and reviewed the manuscript. V.C. and G.F. are guarantors for the contents of the article and had full access to all the data in the study and take responsibility for the integrity of the data and the accuracy of the data analysis.
Supplementary_materials.zip
Download Zip (40.9 KB)Acknowledgments
The authors gratefully acknowledge the valuable collaboration of all the members of the Multidisciplinary Obesity Team, Clínica Universidad de Navarra, Pamplona, Spain.
Funding
This work was supported by ISCIII-Subdirección General de Evaluación y FEDER (PI13/00460, PI14/00950 and PI16/01217; Plan Estatal I+D+I/2013–2016) and by Fundación Caja Navarra (20-2014). CIBER Fisiopatología de la Obesidad y Nutrición (CIBEROBN) is an initiative of the ISCIII, Spain.
References
- Howlader N, Noone AM, Krapcho M. SEER Cancer Statistics Review, 1975–2013. National Cancer Institute. http://seer.cancer.gov/csr/1975_2013/. 2016
- Lin JS, Piper MA, Perdue LA, Rutter CM, Webber EM, O'Connor E, Smith N, Whitlock EP. Screening for colorectal cancer: updated evidence report and systematic review for the US preventive services task force. JAMA 2016; 315:2576-94; PMID:27305422; https://doi.org/10.1001/jama.2016.3332
- Siegel RL, Miller KD, Jemal A. Cancer statistics, 2015. Cancer J Clin 2015; 65:5-29; PMID:25559415; https://doi.org/10.3322/caac.2125410.3322/caac.21314
- Terzic J, Grivennikov S, Karin E, Karin M. Inflammation and colon cancer. Gastroenterology 2010; 138:2101-14 e5; https://doi.org/10.1053/j.gastro.2010.01.058
- Elinav E, Nowarski R, Thaiss CA, Hu B, Jin C, Flavell RA. Inflammation-induced cancer: crosstalk between tumours, immune cells and microorganisms. Nat Rev Cancer 2013; 13:759-71; PMID:24154716; https://doi.org/10.1038/nrc3611
- Grivennikov SI, Greten FR, Karin M. Immunity, inflammation, and cancer. Cell 2010; 140:883-99; PMID:20303878; https://doi.org/10.1016/j.cell.2010.01.025
- Zhong Z, Sanchez-Lopez E, Karin M. Autophagy, inflammation, and immunity: a troika governing cancer and its treatment. Cell 2016; 166:288-98; PMID:27419869; https://doi.org/10.1016/j.cell.2016.05.051
- Bhaskaran K, Douglas I, Forbes H, dos-Santos-Silva I, Leon DA, Smeeth L. Body-mass index and risk of 22 specific cancers: a population-based cohort study of 5.24 million UK adults. Lancet 2014; 384:755-65; PMID:25129328; https://doi.org/10.1016/S0140-6736(14)60892-8
- Frühbeck G, Toplak H, Woodward E, Yumuk V, Maislos M, Oppert JM. Obesity: the gateway to ill health – an EASO position statement on a rising public health, clinical and scientific challenge in Europe. Obes Facts 2013; 6:117-20; PMID:23548858; https://doi.org/10.1159/000350627
- Kaaks R, Kuhn T. Epidemiology: obesity and cancer – the evidence is fattening up. Nat Rev Endocrinol 2014; 10:644-5; PMID:25265978; https://doi.org/10.1038/nrendo.2014.168
- Renehan AG, Tyson M, Egger M, Heller RF, Zwahlen M. Body-mass index and incidence of cancer: a systematic review and meta-analysis of prospective observational studies. Lancet 2008; 371:569-78; PMID:18280327; https://doi.org/10.1016/S0140-6736(08)60269-X
- Catalán V, Gómez-Ambrosi J, Rodríguez A, Frühbeck G. Adipose tissue immunity and cancer. Front Physiol 2013; 4:275; PMID:24106481; https://doi.org/10.3389/fphys.2013.00275
- Catalán V, Gómez-Ambrosi J, Rodríguez A, Ramírez B, Izaguirre M, Hernández-Lizoain JL, Baixauli J, Martí P, Valentí V, Moncada R et al. Increased obesity-associated circulating levels of the extracellular matrix proteins osteopontin, chitinase-3 like-1 and tenascin C are associated with colon cancer. PLoS One 2016; 11:e0162189; PMID:27612200; https://doi.org/10.1371/journal.pone.0162189
- Catalán V, Gómez-Ambrosi J, Rodríguez A, Ramírez B, Silva C, Rotellar F, Hernández-Lizoain JL, Baixauli J, Valentí V, Pardo F et al. Up-regulation of the novel proinflammatory adipokines lipocalin-2, chitinase-3 like-1 and osteopontin as well as angiogenic-related factors in visceral adipose tissue of patients with colon cancer. J Nutr Biochem 2011; 22:634-41; PMID:20961744; https://doi.org/10.1016/j.jnutbio.2010.04.015
- Frühbeck G, Gómez-Ambrosi J. Control of body weight: a physiologic and transgenic perspective. Diabetologia 2003; 46:143-72; PMID:12627314; https://doi.org/10.1007/s00125-003-1053-4
- Lysaght J, van der Stok EP, Allott EH, Casey R, Donohoe CL, Howard JM, McGarrigle SA, Ravi N, Reynolds JV, Pidgeon GP. Pro-inflammatory and tumour proliferative properties of excess visceral adipose tissue. Cancer Lett 2011; 312:62-72; PMID:21890265; https://doi.org/10.1016/j.canlet.2011.07.034
- Pérez-Hernández AI, Catalán V, Gómez-Ambrosi J, Rodríguez A, Frühbeck G. Mechanisms linking excess adiposity and carcinogenesis promotion. Front Endocrinol 2014; 5:65; PMID:24829560; https://doi.org/10.3389/fendo.2014.00065
- Ribeiro R, Monteiro C, Catalán V, Hu P, Cunha V, Rodríguez A, Gómez-Ambrosi J, Fraga A, Principe P, Lobato C et al. Obesity and prostate cancer: gene expression signature of human periprostatic adipose tissue. BMC Med 2012; 10:108; PMID:23009291; https://doi.org/10.1186/1741-7015-10-108
- Catalan V, Gomez-Ambrosi J, Rodriguez A, Ramirez B, Valenti V, Moncada R, Landecho MF, Silva C, Salvador J, Fruhbeck G. Increased interleukin-32 levels in obesity promote adipose tissue inflammation and extracellular matrix remodeling: effect of weight loss. Diabetes 2016; 65:3636-48; PMID:27630206; https://doi.org/10.2337/db16-0287
- Dahl CA, Schall RP, He HL, Cairns JS. Identification of a novel gene expressed in activated natural killer cells and T cells. J Immunol 1992; 148:597-603; PMID:1729377
- Joosten LA, Heinhuis B, Netea MG, Dinarello CA. Novel insights into the biology of interleukin-32. Cell Mol Life Sci 2013; 70:3883-92; PMID:23463238; https://doi.org/10.1007/s00018-013-1301-9
- Ribeiro-Dias F, Saar Gomes R, de Lima Silva LL, Dos Santos JC, Joosten LA. Interleukin 32: a novel player in the control of infectious diseases. J Leukoc Biol 2017; 101:39-52; PMID:27793959; https://doi.org/10.1189/jlb.4RU0416-175RR
- Choi JD, Bae SY, Hong JW, Azam T, Dinarello CA, Her E, Choi WS, Kim BK, Lee CK, Yoon DY et al. Identification of the most active interleukin-32 isoform. Immunology 2009; 126:535-42; PMID:18771438; https://doi.org/10.1111/j.1365-2567.2008.02917.x
- Kim SH, Han SY, Azam T, Yoon DY, Dinarello CA. Interleukin-32: a cytokine and inducer of TNFα. Immunity 2005; 22:131-42; PMID:15664165; https://doi.org/10.1016/S1074-7613(04)00380-210.1016/j.immuni.2004.12.003
- Netea MG, Azam T, Ferwerda G, Girardin SE, Walsh M, Park JS, Abraham E, Kim JM, Yoon DY, Dinarello CA et al. IL-32 synergizes with nucleotide oligomerization domain (NOD) 1 and NOD2 ligands for IL-1β and IL-6 production through a caspase 1-dependent mechanism. Proc Natl Acad Sci USA 2005; 102:16309-14; PMID:16260731; https://doi.org/10.1073/pnas.0508237102
- Nold-Petry CA, Rudloff I, Baumer Y, Ruvo M, Marasco D, Botti P, Farkas L, Cho SX, Zepp JA, Azam T et al. IL-32 promotes angiogenesis. J Immunol 2014; 192:589-602; PMID:24337385; https://doi.org/10.4049/jimmunol.1202802
- Tsai CY, Wang CS, Tsai MM, Chi HC, Cheng WL, Tseng YH, Chen CY, Lin CD, Wu JI, Wang LH et al. Interleukin-32 increases human gastric cancer cell invasion associated with tumor progression and metastasis. Clin Cancer Res 2014; 20:2276-88; PMID:24602839; https://doi.org/10.1158/1078-0432.CCR-13-1221
- Zeng Q, Li S, Zhou Y, Ou W, Cai X, Zhang L, Huang W, Huang L, Wang Q. Interleukin-32 contributes to invasion and metastasis of primary lung adenocarcinoma via NF-κB induced matrix metalloproteinases 2 and 9 expression. Cytokine 2014; 65:24-32; PMID:24140068; https://doi.org/10.1016/j.cyto.2013.09.017
- Wang S, Chen F, Tang L. IL-32 promotes breast cancer cell growth and invasiveness. Oncol Lett 2015; 9:305-7; PMID:25435980; https://doi.org/10.3892/ol.2014.2641
- Yang Y, Wang Z, Zhou Y, Wang X, Xiang J, Chen Z. Dysregulation of over-expressed IL-32 in colorectal cancer induces metastasis. World J Surg Oncol 2015; 13:146; PMID:25889282; https://doi.org/10.1186/s12957-015-0552-3
- Moschen AR, Fritz T, Clouston AD, Rebhan I, Bauhofer O, Barrie HD, Powell EE, Kim SH, Dinarello CA, Bartenschlager R et al. Interleukin-32: a new proinflammatory cytokine involved in hepatitis C virus-related liver inflammation and fibrosis. Hepatology 2011; 53:1819-29; PMID:21381070; https://doi.org/10.1002/hep.24285
- Heinhuis B, Plantinga TS, Semango G, Kusters B, Netea MG, Dinarello CA, Smit JW, Netea-Maier RT, Joosten LA. Alternatively spliced isoforms of IL-32 differentially influence cell death pathways in cancer cell lines. Carcinogenesis 2016; 37:197-205; PMID:26678222; https://doi.org/10.1093/carcin/bgv172
- Khawar MB, Abbasi MH, Sheikh N. IL-32: a novel pluripotent inflammatory interleukin, towards gastric inflammation, gastric cancer, and chronic rhino sinusitis. Mediat Inflamm 2016; 2016:8413768; PMID:27143819; https://doi.org/10.1155/2016/8413768
- Chang CJ, Chien Y, Lu KH, Chang SC, Chou YC, Huang CS, Chang CH, Chen KH, Chang YL, Tseng LM et al. Oct4-related cytokine effects regulate tumorigenic properties of colorectal cancer cells. Biochem Biophys Res Commun 2011; 415:245-51; PMID:22037460; https://doi.org/10.1016/j.bbrc.2011.10.024
- Choi J, Bae S, Hong J, Ryoo S, Jhun H, Hong K, Yoon D, Lee S, Her E, Choi W et al. Paradoxical effects of constitutive human IL-32γ in transgenic mice during experimental colitis. Proc Natl Acad Sci USA 2010; 107:21082-6; PMID:21078994; https://doi.org/10.1073/pnas.1015418107
- Shioya M, Nishida A, Yagi Y, Ogawa A, Tsujikawa T, Kim-Mitsuyama S, Takayanagi A, Shimizu N, Fujiyama Y, Andoh A. Epithelial overexpression of interleukin-32α in inflammatory bowel disease. Clin Exp Immunol 2007; 149:480-6; PMID:17590175; https://doi.org/10.1111/j.1365-2249.2007.03439.x
- Joosten LA, Netea MG, Kim SH, Yoon DY, Oppers-Walgreen B, Radstake TR, Barrera P, van de Loo FA, Dinarello CA, van den Berg WB. IL-32, a proinflammatory cytokine in rheumatoid arthritis. Proc Natl Acad Sci USA 2006; 103:3298-303; PMID:16492735; https://doi.org/10.1073/pnas.0511233103
- Castellone MD, Teramoto H, Williams BO, Druey KM, Gutkind JS. Prostaglandin E2 promotes colon cancer cell growth through a Gs-axin-β-catenin signaling axis. Science 2005; 310:1504-10; PMID:16293724; https://doi.org/10.1126/science.1116221
- Oh JH, Cho MC, Kim JH, Lee SY, Kim HJ, Park ES, Ban JO, Kang JW, Lee DH, Shim JH et al. IL-32γ inhibits cancer cell growth through inactivation of NF-κB and STAT3 signals. Oncogene 2011; 30:3345-59; PMID:21423208; https://doi.org/10.1038/onc.2011.52
- Park ES, Yoo JM, Yoo HS, Yoon DY, Yun YP, Hong J. IL-32γ enhances TNF-α-induced cell death in colon cancer. Mol Carcinog 2014; 53(Suppl 1):E23-35; PMID:23255489; https://doi.org/10.1002/mc.21990
- Iyengar NM, Gucalp A, Dannenberg AJ, Hudis CA. Obesity and cancer mechanisms: tumor microenvironment and inflammation. J Clin Oncol 2016; 34:4270-6; PMID:27903155; https://doi.org/10.1200/JCO.2016.67.4283
- Bai X, Kinney WH, Su WL, Bai A, Ovrutsky AR, Honda JR, Netea MG, Henao-Tamayo M, Ordway DJ, Dinarello CA et al. Caspase-3-independent apoptotic pathways contribute to interleukin-32γ-mediated control of Mycobacterium tuberculosis infection in THP-1 cells. BMC Microbiol 2015; 15:39; PMID:25887904; https://doi.org/10.1186/s12866-015-0366-z
- Netea MG, Lewis EC, Azam T, Joosten LA, Jaekal J, Bae SY, Dinarello CA, Kim SH. Interleukin-32 induces the differentiation of monocytes into macrophage-like cells. Proc Natl Acad Sci USA 2008; 105:3515-20; PMID:18296636; https://doi.org/10.1073/pnas.0712381105
- Heinhuis B, Koenders MI, van den Berg WB, Netea MG, Dinarello CA, Joosten LA. Interleukin 32 (IL-32) contains a typical α-helix bundle structure that resembles focal adhesion targeting region of focal adhesion kinase-1. J Biol Chem 2012; 287:5733-43; PMID:22203669; https://doi.org/10.1074/jbc.M111.288290
- Chang WJ, Du Y, Zhao X, Ma LY, Cao GW. Inflammation-related factors predicting prognosis of gastric cancer. World J Gastroenterol 2014; 20:4586-96; PMID:24782611; https://doi.org/10.3748/wjg.v20.i16.4586
- Murahovschi V, Pivovarova O, Ilkavets I, Dmitrieva RM, Docke S, Keyhani-Nejad F, Gogebakan O, Osterhoff M, Kemper M, Hornemann S et al. WISP1 is a novel adipokine linked to inflammation in obesity. Diabetes 2015; 64:856-66; PMID:25281430; https://doi.org/10.2337/db14-0444
- Liu YP, Hsiao M. Exercise-induced SPARC prevents tumorigenesis of colon cancer. Gut 2013; 62:810-1; PMID:23086830; https://doi.org/10.1136/gutjnl-2012-303235
- Tai IT, Dai M, Owen DA, Chen LB. Genome-wide expression analysis of therapy-resistant tumors reveals SPARC as a novel target for cancer therapy. J Clin Invest 2005; 115:1492-502; PMID:15902309; https://doi.org/10.1172/JCI23002
- Rodríguez A, Ezquerro S, Mendez-Gimenez L, Becerril S, Frühbeck G. Revisiting the adipocyte: a model for integration of cytokine signaling in the regulation of energy metabolism. Am J Physiol Endocrinol Metab 2015; 309:E691-714; PMID:26330344; https://doi.org/10.1152/ajpendo.00297.2015
- Gómez-Ambrosi J, Salvador J, Páramo JA, Orbe J, de Irala J, Diez-Caballero A, Gil MJ, Cienfuegos JA, Frühbeck G. Involvement of leptin in the association between percentage of body fat and cardiovascular risk factors. Clin Biochem 2002; 35:315-20; PMID:12135695; https://doi.org/10.1016/S0009-9120(02)00320-X
- Hillenbrand A, Fassler J, Huber N, Xu P, Henne-Bruns D, Templin M, Schrezenmeier H, Wolf AM, Knippschild U. Changed adipocytokine concentrations in colorectal tumor patients and morbidly obese patients compared to healthy controls. BMC Cancer 2012; 12:545; PMID:23173608; https://doi.org/10.1186/1471-2407-12-545
- Nieman KM, Kenny HA, Penicka CV, Ladanyi A, Buell-Gutbrod R, Zillhardt MR, Romero IL, Carey MS, Mills GB, Hotamisligil GS et al. Adipocytes promote ovarian cancer metastasis and provide energy for rapid tumor growth. Nat Med 2011; 17:1498-503; PMID:22037646; https://doi.org/10.1038/nm.2492
- Park JS, Lee S, Jeong AL, Han S, Ka HI, Lim JS, Lee MS, Yoon DY, Lee JH, Yang Y. Hypoxia-induced IL-32β increases glycolysis in breast cancer cells. Cancer Lett 2015; 356:800-8; PMID:25449783; https://doi.org/10.1016/j.canlet.2014.10.030
- Novick D, Rubinstein M, Azam T, Rabinkov A, Dinarello CA, Kim SH. Proteinase 3 is an IL-32 binding protein. Proc Natl Acad Sci USA 2006; 103:3316-21; PMID:16488976; https://doi.org/10.1073/pnas.0511206103
- Dinarello CA, Kim SH. IL-32, a novel cytokine with a possible role in disease. Ann Rheum Dis 2006; 65(Suppl 3):iii61-4; PMID:17038476; https://doi.org/10.1136/ard.2006.058511
- Sorrentino C, Di Carlo E. Expression of IL-32 in human lung cancer is related to the histotype and metastatic phenotype. Am J Respir Crit Care Med 2009; 180:769-79; PMID:19628777; https://doi.org/10.1164/rccm.200903-0400OC
- Zhang L, Shay JW. Multiple roles of APC and its therapeutic implications in colorectal cancer. J Natl Cancer Inst 2017; 109(8); PMID:28423402; https://doi.org/10.1093/jnci/djw332
- Dirat B, Bochet L, Dabek M, Daviaud D, Dauvillier S, Majed B, Wang YY, Meulle A, Salles B, Le Gonidec S et al. Cancer-associated adipocytes exhibit an activated phenotype and contribute to breast cancer invasion. Cancer Res 2011; 71:2455-65; PMID:21459803; https://doi.org/10.1158/0008-5472.CAN-10-3323
- Gómez-Ambrosi J, Silva C, Galofré JC, Escalada J, Santos S, Gil MJ, Valentí V, Rotellar F, Ramírez B, Salvador J et al. Body adiposity and type 2 diabetes: increased risk with a high body fat percentage even having a normal BMI. Obesity 2011; 19:1439-44; PMID:21394093; https://doi.org/10.1038/oby.2011.36
- Gómez-Ambrosi J, Silva C, Galofré JC, Escalada J, Santos S, Millán D, Vila N, Ibañez P, Gil MJ, Valentí V et al. Body mass index classification misses subjects with increased cardiometabolic risk factors related to elevated adiposity. Int J Obes 2012; 36:286-94; PMID:1587201; https://doi.org/10.1038/ijo.2011.100
- Gómez-Ambrosi J, Silva C, Catalán V, Rodríguez A, Galofré JC, Escalada J, Valentí V, Rotellar F, Romero S, Ramírez B et al. Clinical usefulness of a new equation for estimating body fat. Diabetes Care 2012; 35:383-8; PMID:22179957; https://doi.org/10.2337/dc11-1334
- Rodríguez A, Catalán V, Gómez-Ambrosi J, García-Navarro S, Rotellar F, Valentí V, Silva C, Gil MJ, Salvador J, Burrell MA et al. Insulin- and leptin-mediated control of aquaglyceroporins in human adipocytes and hepatocytes is mediated via the PI3K/Akt/mTOR signaling cascade. J Clin Endocrinol Metab 2011; 96:E586-97; PMID:21289260; https://doi.org/10.1210/jc.2010-1408