ABSTRACT
Overexpression of human epidermal growth factor receptor-2 (HER2) occurs in 20–30% of invasive breast cancers. Monoclonal antibody therapy is effective in treating HER2-driven mammary carcinomas, but its utility is limited by high costs, side effects and development of resistance. Active vaccination may represent a safer, more effective and cheaper alternative, although the induction of strong and durable autoantibody responses is hampered by immune-tolerogenic mechanisms. Using a novel virus-like particle (VLP) based vaccine platform we show that directional, high-density display of human HER2 on the surface of VLPs, allows induction of therapeutically potent anti-HER2 autoantibody responses. Prophylactic vaccination reduced spontaneous development of mammary carcinomas by 50%-100% in human HER2 transgenic mice and inhibited the growth of HER2-positive tumors implanted in wild-type mice. The HER2-VLP vaccine shows promise as a new cost-effective modality for prevention and treatment of HER2-positive cancer. The VLP platform may represent an effective tool for development of vaccines against other non-communicable diseases.
Introduction
Immunotherapy represents a major breakthrough in the treatment of cancer.Citation1 Anticancer immunotherapies are generally directed against tumor-associated antigens overexpressed on malignant cells, but scarcely expressed in normal tissue.Citation2 The human epidermal growth factor receptor-2 (HER2), which mediates proliferative and anti-apoptotic signals,Citation3 is overexpressed in many different cancer types, including carcinomas of the bladder, pancreas, ovary, endometrium, colon, kidney, head and neck, stomach, esophagus, prostate and breast.Citation4 HER2 overexpression occurs in 20–30% of invasive breast carcinomas and is correlated with poor prognosis.Citation5 Passive immunotherapy using monoclonal antibodies (trastuzumab, Herceptin®, RocheCitation6 and pertuzumab, Perjeta®, RocheCitation7) targeting epitopes in the extracellular domain (ECD) of HER2, have resulted in significant improvement in progression-free and overall survival rate of HER2 positive metastatic breast cancer patients.Citation8-10 Unfortunately, treatment of HER2-positive breast cancer with monoclonal antibodies (mAbs) is laborious, expensive and associated with severe side effects. Specifically, the serum half-life (2–4 weeks) of the mAbsCitation8,Citation9 requires that new doses are administered continuously every third week.Citation10 Continuous administration of high doses of mAb often results in immune reactions being raised against the therapeutic mAb. This may lead to hypersensitivity reactions requiring premedication with cortisol or anti-histamineCitation11 and treatment failure.Citation12-14 Also, anti-HER2 mAb therapy can cause cardiac adverse effects via mechanisms, which are not completely understood.Citation15 Finally, the majority of patients with HER2-positive breast cancer acquire resistance to treatment with trastuzumab already within the first year.Citation16-18
These limitations have prompted investigation into strategies for development of anti-HER2 vaccines capable of triggering the patient's own immune system to produce anti-tumor Abs.Citation19-22 In this regard, the main hurdle has been to generate robust and durable anti-tumor immune responses. Monomeric proteins are generally weak immunogensCitation23 and simple subunit vaccines based on a soluble protein antigen in an adjuvant formulation have almost exclusively failed in clinical trials due to insufficient efficacy. In the context of anti-cancer vaccines multiple mechanisms moreover prevent induction of immune responses against self. Central T-cell tolerance prevent the egress of auto-reactive T cells from the thymus and the tumor environment imposes additional immune tolerogenic mechanisms to prevent induction of CD4+ T-helper cells.Citation24 Consequently, auto-reactive B cells, which can be found in the circulation,Citation25 do not receive co-stimulation from CD4+ T-helper cells, which is required for their full activation and subsequent affinity maturation and isotype-switch of the Ab.Citation26 Contrary to monomeric proteins, surface of virus-like particles (VLPs) are highly immunogenic due to sharing key characteristics with live viruses. Their repetitive surface structures facilitate complement fixation and B cell receptor clustering, which activate the innate immune system and leads to greater B cell activation.Citation27,Citation28 Multivalent display (e.g. on the surface of a VLP) can moreover abrogate the ability of the humoral immune system to distinguish between self and foreign and promote class switching, multiplication, affinity maturation, and survival of autoreactive B cells.Citation29,Citation30 Consequently, VLP-display of a self-antigen is critical for inducing strong and durable autoantibody responses.Citation30
We have recently developed a highly versatile antigen display platform that unlike existing technologies, effectively facilitates directional covalent attachment of large vaccine antigens at high density on the surface of VLPs.Citation31 The system employs a tag/catcher conjugation systemCitation32 that was developed by splitting the CnaB2 domain from the fibronectin-binding protein FbaB of Streptococcus pyogenes into a highly reactive peptide (SpyTag) and protein (SpyCatcher) binding partner. Interaction between SpyTag and SpyCatcher results in spontaneous formation of an isopeptide bond, which occurs at high efficiency in a wide variety of protein contexts and buffer conditions. Here, we produced the full HER2 extracellular domain (subdomains I-IV), genetically fused with SpyCatcher, and attached the fusion antigen (SpyCatcher-HER2) to the surface of AP205 phage derived VLPs, each presenting 360 SpyTag peptides ( & ). We demonstrate that the vaccine effectively overcomes B-cell tolerance and induce high-titer therapeutically potent anti-HER2 IgG, which i) prevent tumor growth in wild-type FVB mice grafted with mammary carcinoma cells expressing human HER2 and ii) prevent spontaneous development of human HER2-positive mammary carcinomas in tolerant transgenic mice. Thus, the HER2-VLP vaccine has potential to become a tool for treatment and prevention of HER2-positive cancers. The results also provide strong proof-of-concept for the use of the VLP platform to develop self-antigen based vaccines against non-communicable diseases.
Figure 1. HER2-VLP vaccine design and characterization. A. The HER2 extracellular domain (ECD) was genetically fused at the N-terminus to SpyCatcher (SpyC). TM = trans membrane, ICD = intracellular domain. Arrows indicate HER2 subdomains targeted by licensed mAbs, pertuzumab and trastuzumab B. Schematic showing the HER2-VLP vaccine development process. The Spytagged VLP subunit and the SpyCatcher-HER2 antigen are separately expressed and purified, and then mixed. The tag/catcher conjugation insures a directional, high-density ‘virus-like’ display of HER2 on the surface of the VLPs. C. Reduced SDS-PAGE gel showing individual vaccine components. M = Size marker. Lane 1 contains uncoupled Spytagged VLP. Lane 2 contains subunit SpyCatcher-HER2. Lane 3 shows the resultant SpyCatcher-HER2:VLP conjugates after incubation of the components. Specifically, the lane contains both unconjugated VLP subunit (∼18.5 kDa) and SpyCatcher-HER2 antigen (marked by asterisk) (∼92 kDa) as well as tag/catcher mediated conjugates consisting of a VLP subunit bound to either one or two SpyCatcher-HER2 antigens. Lane 4 shows the final HER2-VLP vaccine product after residual uncoupled SpyCatcher-HER2 has been removed by dialysis. D. Transmission electron microscopy of HER2-VLP showing uniform, non-aggregated particles of approximately 66 nm.
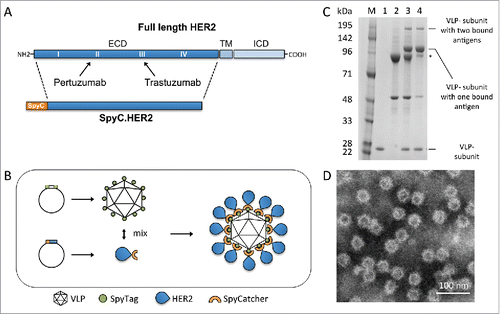
Results
Development and characterization of a VLP-based HER2 vaccine
The SpyCatcher-HER2 fusion antigen comprising the HER2 ECD () was expressed in S2 insect cells. Mixing of SpyCatcher-HER2 with Spytagged VLPs (each presenting a total of 360 complementary SpyTags) at a molar ratio of 1:1 (antigen/VLP subunit) resulted in the formation of stable, non-aggregated VLPs each coated with an average of 183 SpyCatcher-HER2 antigens ( & ). Subsequent dialysis of the reaction mixtures reduced the amount of unconjugated SpyCatcher-HER2 antigen present in the vaccine formulation (). The produced HER2-VLP conjugates appeared stable i.e. particle integrity and aggregation state did not change during storage (2 weeks at 4°C) or during a freeze/thaw cycle, as confirmed by electron microscopy and dynamic light-scattering (data not shown).
Therapy of HER2 mammary carcinoma
We evaluated the immunogenicity and the therapeutic potential of the vaccine in wild type mice grafted with mammary carcinoma cells expressing HER2. Specifically, tumors were developed in FVB mice using either MAMBO89 cells () or HER2-positive tumor fragments from primary transgenic mammary carcinomas (). Biweekly immunizations with HER2-VLP, continued throughout the course of the experiment, elicited high levels of HER2-specific Abs and inhibited tumor growth. It is noteworthy that vaccinations completely prevented tumor growth in 4 of 5 mice inoculated with tumor fragments (). These encouraging results obtained in mice not expressing human HER2 led us to test the vaccine in transgenic mice, which are immunologically tolerant to human HER2.
Figure 2. Therapy of HER2 mammary carcinomas with the HER2-VLP vaccine. Tumors expressing HER2 were established in groups of five female FVB mice by s.c. injection of MAMBO89 cells (A-C) or of mammary carcinoma fragments (D-F), see Materials and Methods for details. HER2-VLP vaccinations were administered in the tibialis anterior muscle every second week starting either 5 weeks after cell injection or 2 weeks after fragment implantation. (A, D) tumor growth curves, each line represents one mouse. (B, E) Kaplan-Meier analysis of data shown in panels A and D. Each line represents the proportion of mice that were either tumor-free or bore tumors smaller than 1 cm3; Significance of difference by the Mantel-Haenszel test: p < 0.01 (B), p = 0.01 (E). (C, F) Serum concentration of Abs against human HER2 extracellular domain, as measured by a specific ELISA; significance of difference by the non-parametric Mann Whitney Rank Sum Test: p<0.01.
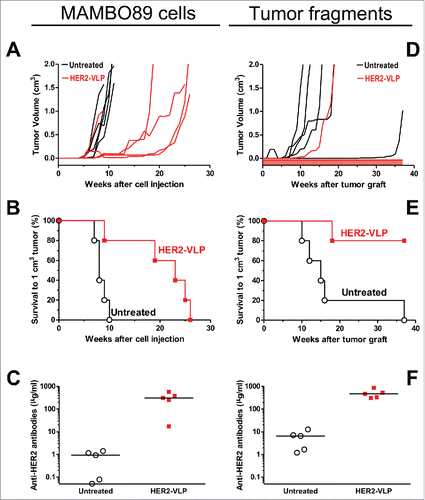
Prevention of HER2 transgenic mammary carcinogenesis
We first tested the effect of the HER2-VLP vaccine on transgenic mice which spontaneously develop mammary carcinomas expressing the Delta16 isoform of the HER2 oncogene.Citation33 The HER2 Delta16 isoform is a splice variant lacking exon 16 and is known to be more oncogenic than the full-length HER2.Citation33-35 Vaccination elicited a strong immune response that completely blocked the onset of mammary carcinomas (). All vaccinated mice remained tumor-free until one year of age, whereas all untreated mice developed carcinomas, which started at two months of age () and increased in multiplicity over time (); mammary carcinogenesis in mice vaccinated with a control preparation containing untagged VLP and HER2 was similar to that of untreated mice, as shown in . A strong antibody response was elicited by vaccination with HER2-VLP, but not by vaccination with untagged VLP and HER2 (). Vaccination with HER2-VLP afforded a durable protection: anti-HER-2 antibody titers remained at very high levels at least until 24 weeks of age (), and protection from tumor onset lasted at least one year (), even though mice in this experiment received only three vaccinations (at 8, 10 and 12 weeks of age).
Figure 3. Prevention of HER2-driven mammary carcinogenesis by HER2-VLP vaccination. Groups of three transgenic mice carrying a human Delta 16 HER2 transgene were vaccinated with HER2-VLP or with monomeric SpyCatcher-HER2 plus untagged VLPs (labeled in graph as Untagged) (A-C). Groups of 7–11 F1 mice carrying a Delta16 and a full-length HER2 transgene were vaccinated with HER2-VLP or with an electroporated, HER2-encoding plasmid, pHuRT (D-F). I.m. vaccinations with HER2-VLP or pHuRT started at 5–8 weeks of age, when mice were healthy and tumor-free, and were administered every second week. (A, D) Tumor-free survival curves (Kaplan-Meier); the survival of mice vaccinated with HER2-VLP was significantly longer than that of all other groups by the Mantel-Haenszel test: p<0.001 (A, D). (B, E) Mean number of mammary carcinomas per mouse (tumor multiplicity). HER2-VLP vaccinated mice had significantly fewer tumors than untreated mice (at all time points beyond 22 weeks of age) and pHURT vaccinated mice (at time points between 11 and 24 weeks) (p <0.05; Student's t test.) (C, F) Kinetics of anti-human HER2 Abs in mouse serum measured by a specific ELISA. Each point represents the mean ± SEM of 3–13 individual sera of different mice. Antibody concentrations elicited by HER2-VLP were significantly higher than those elicited by pHuRT at 2, 4 and 6 weeks, p<0.05 (Student's t test).
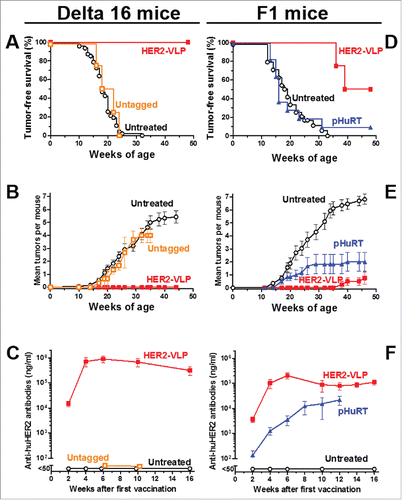
Human breast cancers often co-express Delta16 HER2 and full-length HER2 molecules.Citation34 To model the human condition, we produced an F1 hybrid transgenic mouse strain expressing both HER2 isoforms. These F1 mice were used to benchmark the efficacy of the HER2-VLP vaccine against a HER2-based DNA vaccine (pHuRT), which previously has shown efficacy in various HER2 transgenic murine models,Citation36 including full-length HER2 transgenic mice,Citation37 in which DNA vaccination was more effective at preventing mammary carcinoma onset than passive administration of the anti-HER2 mAb trastuzumab.Citation38 In this study, vaccination with pHuRT had negligible preventive effect on the mammary carcinogenesis of F1 mice (). In contrast, vaccination with the HER2-VLP vaccine significantly inhibited tumor development () as 50% of these mice remained tumor free until one year of age. Moreover, the mice that developed carcinomas had significantly reduced tumor multiplicity compared to both untreated and pHURT-vaccinated mice (). Of note, we did not observe any sign of toxicity or other side effects in mice vaccinated with VLP-HER2. Comparison of anti-HER2 Ab levels in F1 mice revealed that two doses of the HER2-VLP vaccine were enough to reach a plateau of 10ˆ5 ng/ml anti-HER2 IgG whereas pHuRT vaccinated F1 mice never reached as high anti-HER2 Ab titers even after multiple booster vaccinations (). As immunoprevention of mammary carcinogenesis was shown to be mediated by antibodies and T cell cytokines,Citation39 we studied the pattern of cytokines elicited by vaccination in F1 mice. We found that HER2-VLP vaccination induced specific increases in the levels of various cytokines involved in chemotaxis, antigen presentation and type 1 helper T cell response (Supplementary Fig. 2).
A side-by-side comparison of the results obtained in Delta16 mice () and in F1 mice () shows that the vaccine was clearly more effective in the former than in the latter. Even more so when one considers that Delta16 mice received only three vaccinations, vis-à-vis the lifetime vaccination schedule of F1 mice. It should be kept in mind that F1 mice harbor two versions of the transgene, a full-length HER-2 and a Delta 16 isoform, which together could hamper the breakage of immune tolerance and increase tumor resistance to immune attacks. The resistance of F1 mice to immunopreventive vaccines was shown also by the limited effects obtained after pHuRT vaccination, which in different hosts elicited significant protective responses.Citation36,Citation37
Antibodies elicited by the HER2-VLP vaccine bind human HER2-positive tumor cells
All mice vaccinated with HER2-VLPs produced high-titer Abs against HER2 as measured by ELISA ( & and & ). We tested by flow cytometry, whether IgG elicited by the HER2-VLP vaccine bound to human tumor cell lines being HER2-positive or HER2-negative. There was a strong binding to the HER2-positive human tumor cells, but no binding to HER2-negative SJ-RH4 cells ( & ). Staining intensity was comparable to that obtained using an anti-HER2 mAb ().
Figure 4. Antibodies elicited by the HER2-VLP vaccine bind the HER2 extracellular domain on the surface of human tumor cells. (A) Flow cytometry profiles of cells expressing known amounts of HER2, from completely negative (SJ-RH4) to highly positive (SK-OV-3) cells, stained with the serum of one representative F1 mouse vaccinated with HER2-VLP. (B) Comparison of staining either with sera of 3–4 individual F1 mice vaccinated with HER2-VLP (closed diamonds) or with anti-HER2 mAb MGR2 (open diamonds). Fluorescence intensities measured by flow cytometry were normalized by the concentration of IgG against HER2 measured by ELISA in each serum (MFI/HER-2 IgG).
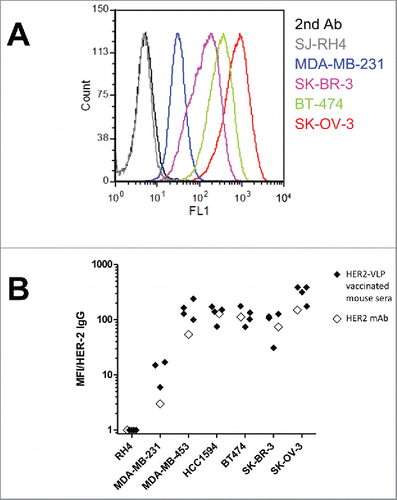
Anti-HER2 antibody isotypes and avidity
To analyze the IgG isotypes elicited by the HER2-VLP vaccine, we took advantage of the high expression of native HER2 molecules by human SK-OV-3 cells. In comparison with pHuRT, the HER2-VLP vaccine induced relatively higher levels of IgG1 compared to levels of IgG2a and IgG2b (). The avidity of anti-HER2 Abs elicited by the two vaccines was further measured using an ELISA-based avidity assay. These results show that the avidity of anti-HER2 Abs induced by the HER2-VLP vaccine increased with the number of immunizations, and at a faster rate compared to immunization with pHURT (). After three and four immunizations the avidity of anti-HER2 antibodies was significantly higher in HER2-VLP vaccinated mice than in mice receiving the pHURT vaccine. To further assess the ability of the HER2-VLP vaccine to induce high-affinity anti-HER2 IgG, kinetic binding experiments were performed using an Attana A200 Quartz Crystal Microbalance Biosensor, which allows for direct and label free analysis of the kinetic properties of the association between different molecules. Recombinant HER2 ECD was immobilized on an amine reactive surface and its binding interaction with i) vaccine-induced anti-HER2 IgGs or ii) trastuzumab mAb was measured. Analysis of vaccine-induced anti-HER2 IgG showed the characteristics of multiple binding factors with different binding kinetics, consistent with a polyclonal IgG population (). Approximately 1000 seconds after sample injection, the (∆Hz) response curve showed a constant dissociation rate, which at this point was in a similar range to that measured for trastuzumab (). This result suggest that the HER2-VLP vaccine is capable of inducing anti-HER2 IgG with affinities comparable to that of trastuzumab.
Figure 5. Subtypes, avidity and affinity of anti-HER2 IgG Abs elicited by vaccination with HER2-VLP. (A) IgG subtypes elicited by the indicated vaccinations, determined by flow cytometry of SK-OV-3 cells after indirect immunofluorescence with subtype-specific secondary Abs. Each bar represents the mean and SEM of 2–5 individual sera of different mice obtained two weeks after the third vaccination. (B) Avidity index, as determined by avidity ELISA, of Abs elicited by the indicated vaccinations. Each point represents the mean±SEM of 3–5 sera. The slopes of linear regression lines are significantly different (p < 0.02). (C, D) The Attana Quartz Crystal Microbalance biosensor was used to measure dissociation rates for the kinetic binding between recombinant HER2 ECD and purified total IgG from VLP-HER2 immunized mice (C) or Trastuzumab mAb (D). Different dilutions of anti-HER2 IgG were flushed over a surface of recombinant HER2 ECD immobilized at 50 µg/mL. Binding is shown as change in frequency over time (ΔHz). The black curve represents the real-time trace, while the red curve shows the fit of the dissociation rate measured for 500 seconds (1000–1500 seconds after injection). Dissociation rate constants (Kd) were obtained by applying a dissociation rate model using the TraceDrawer software.
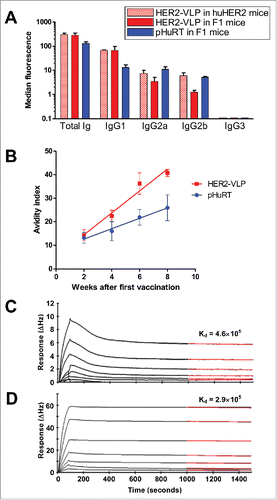
Antibodies elicited by the HER2-VLP vaccine inhibit the growth of both trastuzumab-sensitive and trastuzumab-resistant human breast cancer cells expressing HER2
The functional tumor-inhibitory activity of Abs elicited by HER2-VLP vaccination was studied using the HER2-positive, trastuzumab sensitive BT-474 cell line and its HER2 positive, trastuzumab-resistant clone BT-474 C540. shows that the 3D growth of BT-474 cells was equally and strongly inhibited in the presence of trastuzumab or of sera of mice immunized with HER2-VLPs. As expected, when BT-474 C5 cells were cultured in the same conditions, trastuzumab had no effect. However, serum of HER2-VLP vaccinated mice induced a sizeable inhibition of BT-474 C5 cells.
Figure 6. Antibodies elicited by the HER2-VLP vaccine inhibit 3D growth of human breast cancer cells. HER2-positive BT-474 cells and the trastuzumab-resistant clone BT-474 C5 were seeded in soft agar containing either trastuzumab (10 μg/ml) or sera (diluted 1:100) from two Delta16 mice (labelled as M1 and M2), vaccinated with HER2-VLP. The concentration of anti-HER2 Abs in mouse sera, as determined by ELISA, was comparable to that of trastuzumab (9.7–11.5 μg/ml). (A) Inhibition of agar colony number, counted 18 days after seeding. Each bar represents the mean (and SEM) inhibition of colony number in two independent plates. Inhibition by trastuzumab or anti-HER2 sera was calculated in reference to wells containing medium alone and normal mouse serum, respectively. (B) Dark-field micrographs of colonies in agar. Pictures labelled ‘HER2-VLP’ correspond to serum M1 in panel A. White bar corresponds to 200 μm.
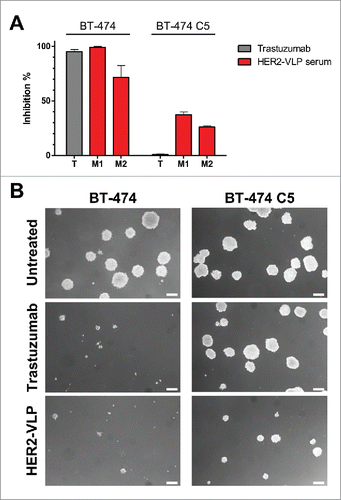
Discussion
HER2 is an important target antigen for vaccine development, and a wide variety of technologies have been deployed over the years.Citation36 However, the inability to overcome immune-tolerogenic mechanisms has severely inhibited the development of self-antigen based vaccines, and despite much effort having been put into vaccine trials, their therapeutic efficacy remains disappointing.Citation41,Citation42 Also taking into account data from clinical trials on other self-antigen based vaccines, it can be concluded that neither the use of potent adjuvants nor the introduction of T helper cell epitopes into the self-antigen are sufficient means to enable autoantibody responses with clinical efficacy.Citation30 Multivalent display (e.g. on a VLP) of a self-antigen is however a highly effective means of overcoming B cell toleranceCitation28,Citation29 and several VLP-based vaccines capable of inducing autoantibodies for therapeutic benefit have entered clinical trials and have demonstrated efficacy.Citation43 Technological constraints have however complicated VLP-display of larger antigens.Citation44 Recently, our group developed a modular VLP-based antigen display platform, which employs a split-protein conjugation system to facilitate high-density directional coupling of even large antigens to the surface of icosahedral VLPs. Using this system we managed to generate stable VLPs presenting multiple copies of the HER2 ECD. Consequently, the VLP-platform provides both ‘T-help’ and ‘antigen multivalency’ that can act in synergy to promote induction of potent polyclonal anti-HER2 Ab responses. Besides, recombinant expression of HER2 in Drosophila S2 insect cells has the potential advantage of improved antigen uptake by APCs due to the addition of insect glycans. Specifically, the paucimannose insect glycan structure terminates in mannans, which has been reported to induce improved uptake by professional APCs and dendritic cells in particular, due to membrane bound mannose receptor expression on these cells.Citation39,Citation45,Citation46 Strikingly, Al-Barwani et al. also demonstrated improved internalization and internal processing of mannosilated VLPs by murine dendritic cells, macrophages and B cells, as well as by human dendritic cells and macrophages.Citation47 To this end, it is notable that administration of the novel HER2-VLP vaccine, using a low antigen dose and a clinically approved adjuvant, elicited strong immune responses against HER2 that fended off mammary carcinoma growth in multiple transgenic mouse systems and experimental setups. It should be noted that expression of AP205 VLPs in E. coli leads to encapsulation of host cell RNA in the lumen of the VLPs. Besides levels of LPS were not measured in the final vaccine preparation. It is thus possible that bacterial RNA (TLR 7/8 agonist) and LPS (TLR 4 agonist) have affected the immune response via activation of TLRs. However, mice vaccinated with a control vaccine consisting of soluble SpyCatcher-HER2 plus untagged AP205 VLPs did not produce anti-HER2 antibodies ().
We have previously demonstrated that prevention of tumor onset in HER2 vaccinated transgenic mice was mediated by anti-HER2 Abs,Citation48 and that protection was dependent on induction of a rapid and strong Ab response to keep at bay the unrelenting mammary carcinogenesis of transgenic mice.Citation49 The polyclonal anti-HER2 response induced by the HER2-VLP vaccine was shown to contain a large fraction of Abs with affinities comparable to that of the standard therapeutic mAb trastuzumab and were shown to react with native HER2 present on the surface of human tumor cells. To appraise the potency of the HER2-VLP vaccine we compared it head-to-head with a highly optimized DNA vaccineCitation50 in a mouse model of aggressive mammary carcinogenesis. The HER2-VLP vaccine was clearly superior to the DNA vaccine, affording a better protection from tumor onset through the induction of a stronger Ab response of higher avidity.
Antibodies elicited by the HER2-VLP vaccine inhibited 3D growth of HER2-positive human breast cancer cells (i.e. BT-474) to a similar extent as trastuzumab, and could even inhibit 3D growth of the trastuzumab-resistant clone BT-474 C5. One of the mechanisms of resistance to trastuzumab BT-474 C5 cells depends on formation of EGFR/HER2 heterodimers.Citation40 Our results thus suggest that the vaccine-induced polyclonal response could harbor Abs, which (unlike trastuzumab) can abrogate heterodimerization. In human clinical trials, treatment with multiple anti-HER2 mAb (i.e. trastuzumab and pertuzumab) has been shown to result in a higher treatment efficacy compared to treatment with either mAb alone,Citation51 due to the different mechanisms of action of the two mAbs.Citation52-54 Moreover, preclinical studies have shown that a polyclonal anti-HER2 Ab response, which simultaneously targets multiple epitopes, is capable of mediating multiple cytotoxic mechanisms.Citation55,Citation56 In a translational perspective, an active vaccination approach capable of inducing a polyclonal anti-HER2 Ab response may thus have a multitude of effects against HER2, which may induce a more comprehensive signaling blockade, and prevent cancer cells in acquiring resistance due to escape mutations. Importantly, mechanisms of resistance to HER2-targeted therapy are not limited to alterations in HER2, but may also involve e.g. bypass signaling through alternative receptor or intracellular signaling pathways, which would not be overcome by induction of a polyclonal anti-HER2 antibody response.
The strong preclinical efficacy data shown in this study underline the potential benefits of an active vaccination approach directed against HER2 and support further clinical evaluation of the HER2-VLP vaccine. To this end, dose-escalation and formulation studies (e.g. including more potent extrinsic adjuvants) should be conducted to assess the effect on safety and efficacy. Moreover, preclinical and early clinical studies suggest that the integration of an effective anti-HER2 vaccine, like HER2-VLP, in current therapeutic regimes could build on the immunogenic tumor cell death induced by therapeutic mAbs and chemotherapy, leading to a powerful and prolonged anti-HER2 immune response.Citation57,Citation58 A phase I dose-escalation clinical trial showed that vaccination with recombinant HER2 protein had an acceptable toxicity profile in breast cancer patientsCitation59 thus suggesting that it is a safe and feasible therapeutic strategy. However, it is possible that the increased immunogenicity of the antigen obtained e.g. by VLP-display may lead to unforeseen side-effects. Also, it is possible that antibodies raised against the VLP backbone may cause off-target effects if they cross-react with self-antigens in the vaccine recipient.
Several cancer-associated antigens have been identified as promising targets for immunotherapy and hence potential vaccine antigens. Passive immunotherapy using licensed mAbs has moreover generated valuable safety and efficacy data to guide rational vaccine design. On the other hand, the lack of a generally effective method to facilitate induction of therapeutically potent immune responses to a self-antigen has hampered progress in the development of anti-cancer vaccines. The tag/catcher-based conjugation approach to mediated VLP-display has been found compatible with a wide variety of very different antigensCitation31 and even allows the use of full-length antigens, eliminating the need for prior structural knowledge e.g. on the location of conformational epitopes. Consequently, the modular VLP-display system represents a simple, versatile and effective tool to overcome B-cell immune tolerance, which can be used as a generic platform for the design of self-antigen based vaccines.
Materials and methods
Design, expression and purification of spy-VLP
The Acinetobacter phage, AP205, displaying two SpyTags per VLP subunit, was designed as previously described.Citation31 In brief, the gene encoding the AP205 coat protein (Gene ID: 956335) had the SpyTag peptide sequence (AHIVMVDAYKPTK) fused to both the N- and C-terminus. Each SpyTag was separated from the coat protein by a flexible linker (GSGTAGGGSGS, N-terminus and GTASGGSGGSG, C-terminus). The Spytagged VLP was expressed in Escherichia coli One Shot® BL21 Star™ (DE3) cells (Thermo Scientific) and purified by density gradient ultracentrifugation using an Optiprep™ step gradient (23, 29 and 35%) (Sigma).
Design, expression and purification of SpyCatcher-HER2 antigen
The HER2 ECD comprising amino acids 23–652 (Gene ID: NP_004439) was designed with the SpyCatcherCitation32 sequence (amino acids 23–139, AFD50637) at the N-terminus and a hexa-histidine purification tag at the C-terminus. Additionally, a flexible (GGS) linker was inserted between the HER2 and the SpyCatcher sequence and flanking BamHI and NotI restriction sequences were added to the N- and C-terminus, respectively. The final SpyCatcher-HER2-HIS gene sequence was codon-optimized for expression in Drosophila melanogaster S2 insect cells and synthesized by GeneArt (ThermoFisher Scientific). The gene was then subcloned into the pExpreS2-2i vector (ExpreS2ion Biotechnologies), which was transfected into ExpreS2 Cells using ExpreS2 Insect-TRx5 transfection reagent (ExpreS2ion Biotechnologies). Hereafter, a polyclonal cell line was selected over a three-week period in EX-CELL 420 insect cell medium (Sigma) supplemented with 10% fetal bovine serum (FBS) (Thermo Fisher Scientific) and 4.0 mg/ml G418 (InvivoGen). Following the selection period, the polyclonal cell line was expanded for production in EX-CELL 420 medium. Harvesting of the cell culture supernatant was done by centrifugation and filtration three days after the final split. Recombinant SpyCatcher-HER2-HIS was finally purified on a Ni2+ sepharose column using an ÄKTAxpress purification system (GE-Healthcare).
Design, expression, purification of pHURT
Chimeric human/rat HER2 plasmid (pHuRT), derived from pVAX1 (Invitrogen), encodes a chimeric protein in which the first 390 extracellular N-terminal residues are from HER2 (1–390 residues) and the remaining extracellular and transmembrane residues from rat HER2/neu60. Large-scale production and purification of the plasmids were performed with EndoFree Plasmid Giga kits (QIAGEN, Valencia, CA, USA) as previously reported.Citation61
Formulation of HER2-VLP and pHuRT vaccines
Spytagged AP205 VLPs and SpyCatcher-HER2 antigen were mixed at a 1:1 molar ratio (VLP subunit per antigen) and incubated for 2 hours at 30°C. Excess unbound antigen was removed by dialysis using a 1000 MWCO dialysis tubing (Spectrum Labs). Densitometric analysis of SDS-PAGE gels was performed to determine the amount of antigen coupled to the VLP. Alhydrogel (2%) (Brenntag) was added to the vaccine formulation 1 hour prior to immunizations. For each mouse, 6 µg of VLP-displayed SpyCatcher-HER2 was injected into the tibial muscles. The control vaccine was prepared accordingly, except using untagged AP205 VLPs instead of Spytagged VLPs.
The pHuRT DNA vaccine consisted of 50 μg plasmid diluted to a final volume of 40 μl per mouse in final concentrations of 0.9% NaCl and 6 mg/ml polyglutamate. The DNA vaccine was injected into the tibial muscles (20 μl in each muscle) then the muscle tissues were immediately subjected to electroporation.Citation37
Cell lines
Cell line MAMBO89, established from a mammary carcinoma of huHER2 transgenic mouse,Citation38 was cultured in DMEM (Thermo Fisher Scientific) additioned with 20% fetal bovine serum (FBS, Thermo Fisher Scientific), 30 µg/ml bovine pituitary extract (Corning) and 5 µl/ml MITO+ SERUM EXTENDER (Corning). MAMBO89 cell line was monitored for murine origin by PCR and for full-length HER2 transgene expression by PCR and flow cytometry. Human ovarian cancer cell line SK-OV-3 and human breast cancer cell lines MDA-MB-453, BT-474 and SKBR3 (all from American Type Culture Collection, ATCC) were kindly given by Dr. Serenella M. Pupa (Istituto Nazionale dei Tumori, Milan, Italy). SK-OV-3, MDA-MB-453 and BT-474, were authenticated by DNA fingerprinting on the 11th November 2010 (performed by DSMZ, Braunschweig, Germany). HCC1954, MDA-MB-231 and BT-474 C5 cell lines were purchased from ATCC. Cells were routinely cultured in RPMI medium (Thermo Fisher Scientific) supplemented with 10% FBS. Human rhabdomyosarcoma cell line SJ-RH4, lacking HER2 expression, was cultured in DMEM additioned with 10% FBS. Cell lines were maintained at 37°C in a humidified 5% CO2 atmosphere, except SJ-RH4, which was kept at 7% CO2.
Mice
HER2 mice, transgenic for the full-length human HER2 isoformCitation37,Citation62 were obtained from Genentech (South San Francisco, CA, USA) and bred in our animal facilities. Delta16 mice, transgenic for an alternative splicing isoform of HER2, Delta16, were kindly gifted by Dr. A. Amici (University of Camerino, Camerino, Italy) and Dr. S. Pupa (Fondazione IRCCS, Istituto Nazionale dei Tumori, Milano, Italy).Citation35,Citation63 Delta16 male mice and HER2 female mice were crossed to obtain a double transgenic human HER2/Delta16 progeny, here referred to as F1 mice.Citation38 All transgenic mice expressed human HER2 and/or Delta16 in the mammary glands under the transcriptional control of mouse mammary tumor virus long terminal repeats, leading to the development of mammary carcinomas.Citation62,Citation63 Non-transgenic FVB female mice (FVB/NCrl) were purchased from Charles River Laboratories (Calco, Como, Italy).
Therapeutic immunization
To study the inhibition of tumor growth two different experiments were performed.
i) Cell line MAMBO89 was previously established from a mammary carcinoma of a HER2 transgenic mouse.Citation38 MAMBO89 cells (5 × 106 cells) were subcutaneously (s.c.) injected in 10-20-week-old FVB female mice. Starting 5 weeks after cell injection, mice were immunized every second week with the HER2-VLP vaccine for the entire lifetime of the mouse. Control group consisted of untreated mice.
ii) Five primary mammary carcinomas of three HER2 transgenic mice were minced with scalpels, pooled together and implanted in the fourth left mammary fat pad of FVB female mice, approximately 20-weeks-old. Starting 2 weeks after fragment implantation, mice were vaccinated every second week with HER2-VLP vaccine for the entire lifetime of the mouse. Control group consisted of untreated mice.
For both experiments tumor volume was calculated as (π/6)(√ab),Citation3 where a = maximal tumour diameter and b = maximal tumour diameter perpendicular to a. Serum samples were collected periodically, one day before every vaccination.
Preventive immunization
HER2-VLP or pHuRT were administered every second week. Delta16 mice received three administrations of HER2-VLP starting from 8 weeks of age. F1 HER2/Delta16 mice were treated with pHuRT vaccine or HER2-VLP every second week, starting from 5–8 weeks of age, for the entire lifetime of the mouse. Control groups consisted of untreated mice or mice treated with monomeric SpyCatcher-HER2 plus untagged VLPs. Serum samples were collected periodically, one day before every vaccination.
Female mice were monitored weekly by palpation and tumor dimensions were measured with calipers. Masses with a mean diameter exceeding 3 mm were considered tumors. Mice were euthanized when tumor burden was equivalent to 10% of body mass.
Antibody response
Serum samples were collected periodically and anti-HER2 Abs were detected by specific enzyme-linked immunosorbent assay (ELISA) as described previously.Citation37,Citation61 Sera were diluted 1:400-1:102400. A standard curve with anti-HER2 murine mAb clone 4D5 (Genentech) was run in parallel (0.04 to 30 ng/ml).
Anti-HER2 total Abs and subclasses were also measured by flow cytometry using the Partec CyFlow® space cytofluorimeter (Sysmex Europe GmbH, Norderstedt, Germany) and analysis was performed with FCS EXPRESS 4 (De Novo Software, Glendale, CA, USA). Sera were diluted 1:65 to detect total anti-HER2 IgG. F(ab’)2 fragments of goat anti-mouse IgG (H+L) labelled with Alexa Fluor®488 (20 µg/ml, Life Technologies) were used as secondary Ab. To detect specific Ab isotypes in sera, diluted 1:20, FITC-conjugated rat anti-mouse IgG1, IgG2a, IgG2b, IgG3 (BD Pharmingen™) were used. The intensity of fluorescence of each serum sample was normalized to the expression of HER2 by the SK-OV-3 target cells (HER2-positive human ovarian carcinoma cells) determined using mouse anti-human HER2 primary Ab, clone MGR-2, (Enzo Life Science, Farmingdale, NY, USA).
Measurement of anti-HER2 IgG avidity ELISA
Anti-HER2 avidity assay was performed as described.Citation31 In brief, recombinant HER2 ECD (2 µg/ml in Carbonate-Bicarbonate buffer, pH 9) was coated on Nunc MaxiSorp plates overnight at 4oC. Following, the plates were blocked with 1% BSA for 1 hour and washed three times. The level of anti-HER2 IgG in individual serum samples (known from previous ELISA) was equalized by dilution (1 hour incubation, followed by 3 washes). Freshly made Urea (8M) was added to the wells for 5 min (reference plates were incubated with PBS) followed by a wash. Finally, HRP-conjugated polyclonal goat anti-mouse IgG (Novex A16072, diluted 1:1000) was added (1 hour incubation, RT). The plates were developed using o-phenylenediamine substrate. The enzymatic reaction was stopped by adding 1 M H2SO4. Optical density was measured at 490 nm using an ELISA plate reader (VersaMax Molecular Devices). IgG avidity index values were calculated as the mean OD value of urea-treated wells (triplicates) divided by the mean OD value of control wells, multiplied by 100.
Purification of IgG from anti-HER2 mouse serum
Protein G columns (Pierce, USA) were used for purification of total IgG from anti-HER2 mouse serum samples. In brief, protein G columns were equilibrated using binding buffer (Immunopure IgG, Pierce, USA). Hereafter, a 1:1 mixture of sera and binding buffer was allowed to flow through the columns by gravity. The columns were then washed and bound IgG was eluted using elution buffer (Immunopure IgG, Pierce, USA). Eluted fractions were collected in 1 M Tris-HCl (pH 9.0, Teknova, USA) buffer and transferred for buffer-exchange into PBS using Amicon centrifugal filters (Millipore, USA).
Quantification of cytokines
Levels of cytokines in sera were measured using the Bio-Plex™ Mouse Cytokine bead assay (Bio-Rad) following the manufacturer's instructions.Citation64
Biosensor affinity analysis
Kinetic analysis of the dissociation rates for the binding between purified total IgG from HER2-VLP immunized mice or trastuzumab mAb to recombinant HER2 ECD was performed on a quartz crystal microbalance biosensor (Attana A200, Attana AB). Recombinant HER2 (50 µg/mL) was immobilized onto a LNB carboxyl chip by amine coupling using EDC and S-NHS. An activated/deactivated surface was used as a reference. Assays were performed with a flow rate of 25 µL/min at 25˚C, using HBS-T (100 mm HEPES, 150 mm NaCl, Tween 20 0.05%, pH = 7, 4) as running buffer. Two-fold dilutions of the analyte, prepared in running buffer, were injected over the surface and the association and dissociation was monitored for 95 s and 1500 s, respectively. Sample injections were performed by the C-Fast auto sampler (Attana AB). The binding surface was regenerated after each injection by applying 10 mm Glycine, pH 2 for 30 s. Data was processed in the Attana Attache software (Attana AB) and curve fitting was performed using the TraceDrawer software (Ridgeview Instruments) applying a dissociation rate model.
Inhibition of 3D soft agar growth
In vitro sensitivity of BT-474 (trastuzumab-sensitive) and BT-474 C5 (trastuzumab-resistant) cells to HER2-VLP-induced antibodies was evaluated in three-dimensional cultures, which better represent tumor architecture than bi-dimensional cultures. Cells were seeded at 500 cells/well in 24-well plates in RPMI + 10% FBS + 0.33% agar (Lonza) containing mouse sera diluted 1:100 or trastuzumab (10 μg/ml, kindly provided by Genentech). Colonies (diameter > 90 μm) were counted and 18–30 days after seeding under an inverted microscope in dark-field. Pictures taken with a Canon EOS 600D were processed with ACDSee Ultimate 8 as follows: Convert to black & white: default parameters; Contrast: 60; Sharpen (Amount: 50; Radius: 5; Mask: 0; Detail: 50; Threshold: 1); Clarity: 50.
Disclosure of potential conflicts of interest
S.T., C.M.J., T.G.T., M.A.N., A.S. and A.F.S. are inventors on a patent application concerning the VLP-display technology (WO2016112921 A1) licensed to AdaptVac.
Ethical statements
All in vivo experiments were approved by the institutional review board of the University of Bologna, authorized by the Italian Ministry of Health and done according to Italian and European laws and guidelines.
supp_data.zip
Download Zip (371.1 KB)Acknowledgments
This work was supported by grant IG15324 to P-L. Lollini from the Italian Association for Cancer Research (AIRC) and by the “Pallotti” Fund, Department of Experimental, Diagnostic and Specialty Medicine (DIMES) of the University of Bologna. Tania Balboni and Veronica Giusti are recipients of Ph.D. fellowships from the University of Bologna. Arianna Palladini and Marianna L. Ianzano are recipients of research fellowships (Assegni di Ricerca) from the University of Bologna. The research was also funded by the Danish Research Councils, The Eurostars program and the European Research Councils (ERC).
Additional information
Funding
References
- Couzin-Frankel J. Breakthrough of the year 2013. Cancer immunotherapy. Science. 2013;342:1432–3.
- Scott AM, Wolchok JD, Old LJ. Antibody therapy of cancer. Nat Rev Cancer. 2012;12:278–87. doi:10.1038/nrc3236. PMID:22437872.
- Bianchini G, Gianni L. The immune system and response to HER2-targeted treatment in breast cancer. Lancet Oncol. 2014;15:e58–68. doi:10.1016/S1470-2045(13)70477-7. PMID:24480556.
- Ménard S, Casalini P, Campiglio M, Pupa S, Agresti R, Tagliabue E. HER2 overexpression in various tumor types, focussing on its relationship to the development of invasive breast cancer. Ann Oncol. 2001;12 Suppl 1:S15–9. doi:10.1093/annonc/12.suppl_1.S15.
- Slamon DJ, Clark GM, Wong SG, Levin WJ., Ullrich A, McGuire WL. Human breast cancer: correlation of relapse and survival with amplification of the HER-2/neu oncogene. Science 1987;235:177–82. doi:10.1126/science.3798106. PMID:3798106.
- Hudis CA. Trastuzumab–mechanism of action and use in clinical practice. N Engl J Med. 2007;357:39–51. doi:10.1056/NEJMra043186. PMID:17611206.
- Sabatier R, Gonçalves A. [Pertuzumab (Perjeta®) approval in HER2-positive metastatic breast cancers]. Bull Cancer. 2014;101:765–71. PMID:25091659.
- Baselga J, Carbonell X, Castañeda-Soto NJ, Clemens M, Green M, Harvey V, Morales S, Barton C, Ghahramani P. Phase II study of efficacy, safety, and pharmacokinetics of trastuzumab monotherapy administered on a 3-weekly schedule. J Clin Oncol. 2005;23:2162–71. doi:10.1200/JCO.2005.01.014. PMID:15800309.
- Garg A, Quartino A, Li J, Jin J, Wada DR, Li H, Cort??s J, McNally V, Ross G, Visich J, et al. Population pharmacokinetic and covariate analysis of pertuzumab, a HER2-targeted monoclonal antibody, and evaluation of a fixed, non-weight-based dose in patients with a variety of solid tumors. Cancer Chemother Pharmacol. 2014;74:819–29. doi:10.1007/s00280-014-2560-3. PMID:25119184.
- Leyland-Jones B. Dose scheduling–Herceptin. Oncology. 2001;61 Suppl 2:31–6. doi:10.1159/000055399.
- Vultaggio A, Castells MC. Hypersensitivity Reactions to Biologic Agents. Immunol Allergy Clin North Am. 2014;34:615–32. doi:10.1016/j.iac.2014.04.008. PMID:25017680.
- Chung CH, Mirakhur B, Chan E, Le Q-T, Berlin J, Morse M, Murphy BA, Satinover SM, Hosen J, Mauro D, et al. Cetuximab-induced anaphylaxis and IgE specific for galactose-alpha-1,3-galactose. N Engl J Med. 2008;358:1109–17. doi:10.1056/NEJMoa074943. PMID:18337601.
- Maier S, Chung CH, Morse M, Platts-Mills T, Townes L, Mukhopadhyay P, Bhagavatheeswaran P, Racenberg J, Trifan OC. A retrospective analysis of cross-reacting cetuximab IgE antibody and its association with severe infusion reactions. Cancer Med. 2015;4:36–42. doi:10.1002/cam4.333. PMID:25296628.
- Castells M, Del Carmen Sancho-Serra M, Simarro M. Hypersensitivity to antineoplastic agents: Mechanisms and treatment with rapid desensitization. In: Cancer Immunology, Immunotherapy. 2012. page 1575–84.
- Pondé NF, Lambertini M, de Azambuja E. Twenty years of anti-HER2 therapy-associated cardiotoxicity. ESMO Open. 2016;1:e000073. doi:10.1136/esmoopen-2016-000073. PMID:27843627.
- Slamon DJ, Leyland-Jones B, Shak S, Fuchs H, Paton V, Bajamonde A, Fleming T, Eiermann W, Wolter J, Pegram M, et al. Use of Chemotherapy Plus a Monoclonal Antibody Against HER2 for Metastatic Breast Cancer That Overexpresses HER2. N Engl J Med. 2001;344:783–92. doi:10.1056/NEJM200103153441101. PMID:11248153.
- Baselga BJ, Tripathy D, Mendelsohn J, Baughman S, Benz CC, Dantis L, Sklarin NT, Seidman AD, Hudis CA, Moore J, et al. Phase II Study of Weekly Intravenous Recombinant Metastatic Breast Cancer. J Clin Oncol 1996;14:737–44. doi:10.1200/JCO.1996.14.3.737. PMID:8622019.
- Luque-Cabal M, García-Teijido P, Fernández-Pérez, Y, Sánchez-Lorenzo L, Palacio-Vázquez I. Mechanisms behind the resistance to trastuzumab in HER2-amplified breast cancer and strategies to overcome It. Clin Med Insights Oncol. 2016;10:21–30. PMID:27042153.
- Farkas AM, Finn OJ. Vaccines based on abnormal self-antigens as tumor-associated antigens: Immune regulation. Semin. Immunol. 2010;22:125–31. doi:10.1016/j.smim.2010.03.003. PMID:20403708.
- Ladjemi MZ, Jacot W, Chardès T, Pèlegrin A, Navarro-Teulon I. Anti-HER2 vaccines: New prospects for breast cancer therapy. Cancer Immunol. Immunother. 2010;59:1295–312. doi:10.1007/s00262-010-0869-2. PMID:20532501.
- Baxevanis CN, Voutsas IF, Gritzapis AD, Perez SA, Papamichail M. HER-2/neu as a target for cancer vaccines. Futur Med. 2010;2:213–26.
- Jensen-Jarolim E, Singer J. Cancer vaccines inducing antibody production: more pros than cons. Expert Rev Vaccines. 2011;10:1281–9. doi:10.1586/erv.11.105. PMID:21919618.
- Schijns VEJC. Immunopotentiators in Modern Vaccines. second edition. The Netherlands: Elsevier;2017
- Nurieva RI, Liu X, Dong C. Molecular mechanisms of T-cell tolerance. Immunol. Rev. 2011;241:133–44. doi:10.1111/j.1600-065X.2011.01012.x. PMID:21488895.
- Wardemann H, Yurasov S, Schaefer A, Young JW, Meffre E, Nussenzweig MC. Predominant autoantibody production by early human B cell precursors. Science. 2003;301:1374–7. doi:10.1126/science.1086907. PMID:12920303.
- Allen CDC, Okada T, Tang HL, Cyster JG. Imaging of germinal center selection events during affinity maturation. Science. 2007;315:528–31. doi:10.1126/science.1136736. PMID:17185562.
- Bachmann MF, Jennings GT. Vaccine delivery: a matter of size, geometry, kinetics and molecular patterns. Nat Rev Immunol. 2010;10:787–96. doi:10.1038/nri2868. PMID:20948547.
- Bachmann MF, Rohrer UH, Kündig TM, Bürki K, Hengartner H, Zinkernagel RM. The influence of antigen organization on B cell responsiveness. Science. 1993;262:1448–51. doi:10.1126/science.8248784. PMID:8248784.
- Chackerian B, Durfee MR, Schiller JT. Virus-Like Display of a Neo-Self Antigen Reverses B Cell Anergy in a B Cell Receptor Transgenic Mouse Model. J Immunol. 2008;180:5816–25. doi:10.4049/jimmunol.180.9.5816. PMID:18424700.
- Chackerian B, Frietze KM. Moving towards a new class of vaccines for non-infectious chronic diseases. Expert Rev Vaccines. 2016;15:561–3. doi:10.1586/14760584.2016.1159136. PMID:26919571.
- Thrane S, Janitzek CM, Matondo S, Resende M, Gustavsson T, de Jongh WA, Clemmensen S, Roeffen W, van de Vegte-Bolmer M, van Gemert GJ, et al. Bacterial superglue enables easy development of efficient virus-like particle based vaccines. J Nanobiotechnology. 2016;14:30. doi:10.1186/s12951-016-0181-1. PMID:27117585.
- Zakeri B, Fierer JO, Celik E, Chittock EC, Schwarz-Linek U, Moy VT, Howarth M. Peptide tag forming a rapid covalent bond to a protein, through engineering a bacterial adhesin. Proc Natl Acad Sci U S A. 2012;109:E690–7. doi:10.1073/pnas.1115485109. PMID:22366317.
- Kwong KY, Hung MC. A novel splice variant of HER2 with increased transformation activity. Mol Carcinog. 1998;23:62–8. doi:10.1002/(SICI)1098-2744(199810)23:2%3c62::AID-MC2%3e3.0.CO;2-O. PMID:9808159.
- Castiglioni F, Tagliabue E, Campiglio M, Pupa SM, Balsari A, Ménard S. Role of exon-16-deleted HER2 in breast carcinomas. Endocr Relat Cancer. 2006;13:221–32. doi:10.1677/erc.1.01047. PMID:16601290.
- Castagnoli L, Iezzi M, Ghedini GC, Ciravolo V, Marzano G, Lamolinara A, Zappasodi R, Gasparini P, Campiglio M, Amici A, et al. Activated d16HER2 homodimers and src kinase mediate optimal efficacy for trastuzumab. Cancer Res. 2014;74:6248–59. doi:10.1158/0008-5472.CAN-14-0983. PMID:25164009.
- Lollini P-L, Cavallo F, De Giovanni C, Nanni P. Preclinical vaccines against mammary carcinoma. Expert Rev Vaccines. 2013;12:1449–63. doi:10.1586/14760584.2013.845530. PMID:24195482.
- De Giovanni C, Nicoletti G, Quaglino E, Landuzzi L, Palladini A, Ianzano ML, Dall'Ora M, Grosso V, Ranieri D, Laranga R, et al. Vaccines against human HER2 prevent mammary carcinoma in mice transgenic for human HER2. Breast Cancer Res. 2014;16:R10. doi:10.1186/bcr3602. PMID:24451168.
- Palladini A, Nicoletti G, Lamolinara A, Dall'Ora M, Balboni T, Ianzano ML, Laranga R, Landuzzi L, Giusti V, Ceccarelli C, et al. HER2 isoforms co-expression differently tunes mammary tumor phenotypes affecting onset, vasculature and therapeutic response. Oncotarget. 2017;8:54444–58. doi:10.18632/oncotarget.17088. PMID:28903354.
- Stahl PD, Ezekowitz R a. The mannose receptor is a pattern recognition receptor involved in host defense. Curr Opin Immunol 1998;10:50–5. doi:10.1016/S0952-7915(98)80031-9. PMID:9523111.
- Ritter CA, Perez-Torres M, Rinehart C, Guix M, Dugger T, Engelman JA, Arteaga CL. Human breast cancer cells selected for resistance to trastuzumab in vivo overexpress epidermal growth factor receptor and ErbB ligands and remain dependent on the ErbB receptor network. Clin Cancer Res. 2007;13:4909–19. doi:10.1158/1078-0432.CCR-07-0701. PMID:17699871.
- Curigliano G, Romieu G, Campone M, Dorval T, Duck L, Canon J-L, Roemer-Becuwe C, Roselli M, Neciosup S, Burny W, et al. A phase I/II trial of the safety and clinical activity of a HER2-protein based immunotherapeutic for treating women with HER2-positive metastatic breast cancer. Breast Cancer Res Treat. 2016;156:301–10. doi:10.1007/s10549-016-3750-y. PMID:26975189.
- Marmé F. Immunotherapy in Breast Cancer. Oncol. Res Treat. 2016;39:335–45. doi:10.1159/000446340.
- Jennings GT, Bachmann MF. Immunodrugs: Therapeutic VLP-Based Vaccines for Chronic Diseases. Annu Rev Pharmacol Toxicol. 2009;49:243–63. doi:10.1146/annurev-pharmtox-061008-103129. PMID:18851683.
- Chackerian B. Virus-like particles: flexible platforms for vaccine development. Expert Rev Vaccines. 2007;6:381–90. doi:10.1586/14760584.6.3.381. PMID:17542753.
- Sallusto F. Dendritic cells use macropinocytosis and the mannose receptor to concentrate macromolecules in the major histocompatibility complex class II compartment: downregulation by cytokines and bacterial products. J Exp Med 1995;182:389–400. doi:10.1084/jem.182.2.389. PMID:7629501.
- Diebold SS, Plank C, Cotten M, Wagner E, Zenke M. Mannose receptor-mediated gene delivery into antigen presenting dendritic cells. Somat Cell Mol. Genet. 2002;27:65–74. doi:10.1023/A:1022975705406. PMID:12774941.
- Al-Barwani F, Young SL, Baird MA, Larsen DS, Ward VK. Mannosylation of virus-like particles enhances internalization by antigen presenting cells. PLoS One. 2014;9. doi:10.1371/journal.pone.0104523.
- Nanni P, Landuzzi L, Nicoletti G, De Giovanni C, Rossi I, Croci S, Astolfi A, Iezzi M, Di Carlo E, Musiani P, et al. Immunoprevention of mammary carcinoma in HER-2/neu transgenic mice is IFN-gamma and B cell dependent. J Immunol. 2004;173:2288–96. doi:10.4049/jimmunol.173.4.2288. PMID:15294941.
- Palladini A, Nicoletti G, Pappalardo F, Murgo A, Grosso V, Stivani V, Ianzano ML, Antognoli A, Croci S, Landuzzi L, et al. In silico modeling and in vivo efficacy of cancer-preventive vaccinations. Cancer Res. 2010;70:7755–63. doi:10.1158/0008-5472.CAN-10-0701. PMID:20924100.
- Iezzi M, Quaglino E, Amici A, Lollini P-L, Forni G, Cavallo F. DNA vaccination against oncoantigens: A promise. Oncoimmunology. 2012;1:316–25. doi:10.4161/onci.19127. PMID:22737607.
- Corteś J, Fumoleau P, Bianchi GV, Petrella TM, Gelmon K, Pivot X, Verma S, Albanell J, Conte P, Lluch A, et al. Pertuzumab monotherapy after trastuzumab-based treatment and subsequent reintroduction of trastuzumab: Activity and tolerability in patients with advanced human epidermal growth factor receptor 2-positive breast cancer. J Clin Oncol. 2012;30:1594–600. doi:10.1200/JCO.2011.37.4207. PMID:22393084.
- Longva KE, Pedersen NM, Haslekås C, Stang E, Madshus IH. Herceptin-induced inhibition of ErbB2 signaling involves reduced phosphorylation of Akt but not endocytic down-regulation of ErbB2. Int J Cancer. 2005;116:359–67. doi:10.1002/ijc.21015. PMID:15800944.
- Hommelgaard AM. Association with Membrane Protrusions Makes ErbB2 an Internalization-resistant Receptor. Mol Biol Cell. 2004;15:1557–67. doi:10.1091/mbc.E03-08-0596. PMID:14742716.
- Austin CD, De Mazière AM, Pisacane PI, van Dijk SM, Eigenbrot C, Sliwkowski MX, Klumperman J, Scheller RH. Endocytosis and sorting of ErbB2 and the site of action of cancer therapeutics trastuzumab and geldanamycin. Mol Biol Cell. 2004;15:5268–82. doi:10.1091/mbc.E04-07-0591. PMID:15385631.
- Clay TM, Osada T, Hartman ZC, Hobeika A, Devi G, Morse MA, Kim Lyerly H. Polyclonal immune responses to antigens associated with cancer signaling pathways and new strategies to enhance cancer vaccines. Immunol Res. 2011;49:235–47. doi:10.1007/s12026-010-8186-6. PMID:21136201.
- Triulzi C, Vertuani S, Curcio C, Antognoli A, Seibt J, Akusjärvi G, Wei WZ, Cavallo F, Kiessling R. Antibody-dependent natural killer cell-mediated cytotoxicity engendered by a kinase-inactive human HER2 adenovirus-based vaccination mediates resistance to breast tumors. Cancer Res. 2010;70:7431–41. doi:10.1158/0008-5472.CAN-10-0493. PMID:20823150.
- Disis ML, Wallace DR, Gooley TA, Dang Y, Slota M, Lu H, Coveler AL, Childs JS, Higgins DM, Fintak PA, et al. Concurrent trastuzumab and HER2/neu-specific vaccination in patients with metastatic breast cancer. J Clin Oncol. 2009;27:4685–92. doi:10.1200/JCO.2008.20.6789. PMID:19720923.
- Chen G, Gupta R, Petrik S, Laiko M, Leatherman JM, Asquith JM, Daphtary MM, Garrett-Mayer E, Davidson NE, Hirt K, et al. A Feasibility Study of Cyclophosphamide, Trastuzumab, and an Allogeneic GM-CSF-Secreting Breast Tumor Vaccine for HER2+ Metastatic Breast Cancer. Cancer Immunol Res. 2014;2:949–61. doi:10.1158/2326-6066.CIR-14-0058. PMID:25116755.
- Limentani SA, Csampone M, Dorval T, Curigliano G, de Boer R, Vogel C, White S, Bachelot T, Canon JL, Disis M, et al. A non-randomized dose-escalation phase i trial of a protein-based immunotherapeutic for the treatment of breast cancer patients with HER2-overexpressing tumors. Breast Cancer Res Treat. 2016;156:319–30. doi:10.1007/s10549-016-3751-x. PMID:26993131.
- Quaglino E, Mastini C, Amici A, Marchini C, lezzi M, Lanzardo S, De Giovanni C, Montani M, Lollini PL, Masucci G, et al. A better immune reaction to Erbb-2 tumors is elicited in mice by DNA vaccines encoding rat/human chimeric proteins. Cancer Res. 2010;70:2604–12. doi:10.1158/0008-5472.CAN-09-2548. PMID:20332241.
- De Giovanni C, Nicoletti G, Palladini A, Croci S, Landuzzi L, Antognoli A, Murgo A, Astolfi A, Ferrini S, Fabbi M, et al. A multi-DNA preventive vaccine for p53/Neu-driven cancer syndrome. Hum Gene Ther. 2009;20:453–64. doi:10.1089/hum.2008.172. PMID:19215191.
- Finkle D, Quan ZR, Asghari V, Kloss J, Ghaboosi N, Mai E, Wong WL, Hollingshead P, Schwall R, Koeppen H, et al. HER2-Targeted Therapy Reduces Incidence and Progression of Midlife Mammary Tumors in Female Murine Mammary Tumor Virus huHER2-Transgenic Mice. Clin Cancer Res. 2004;10:2499–511. doi:10.1158/1078-0432.CCR-03-0448. PMID:15073130.
- Marchini C, Gabrielli F, Iezzi M, Zenobi S, Montani M, Pietrella L, Kalogris C, Rossini A, Ciravolo V, Castagnoli L, et al. The human splice variant Δ16HER2 induces rapid tumor onset in a reporter transgenic mouse. PLoS One. 2011;6:1–7. doi:10.1371/journal.pone.0018727.
- Croci S, Nanni P, Palladini A, Nicoletti G, Grosso V, Benegiamo G, Landuzzi L, Lamolinara A, Ianzano ML, Ranieri D, et al. Interleukin-15 is required for immunosurveillance and immunoprevention of HER2/neu-driven mammary carcinogenesis. Breast Cancer Res. 2015;17:70. doi:10.1186/s13058-015-0588-x. PMID:25997501.