ABSTRACT
The term ‘immunogenic cell death’ (ICD) denotes an immunologically unique type of regulated cell death that enables, rather than suppresses, T cell-driven immune responses that are specific for antigens derived from the dying cells. The ability of ICD to elicit adaptive immunity heavily relies on the immunogenicity of dying cells, implying that such cells must encode and present antigens not covered by central tolerance (antigenicity), and deliver immunostimulatory molecules such as damage-associated molecular patterns and cytokines (adjuvanticity). Moreover, the host immune system must be equipped to detect the antigenicity and adjuvanticity of dying cells. As cancer (but not normal) cells express several antigens not covered by central tolerance, they can be driven into ICD by some therapeutic agents, including (but not limited to) chemotherapeutics of the anthracycline family, oxaliplatin and bortezomib, as well as radiation therapy. In this Trial Watch, we describe current trends in the preclinical and clinical development of ICD-eliciting chemotherapy as partner for immunotherapy, with a focus on trials assessing efficacy in the context of immunomonitoring.
Introduction
It has been more than a decade since the concept of boosting the immunogenic potential of cancer cells by eliciting an immunogenic variant of regulated cell death (RCD), i.e. immunogenic cell death (ICD), has been proposed.1–Citation9 A substantial number of subsequent studies have shed light on the intricate molecular and cellular mechanisms that underlie the ability of cancer cells to undergo (and the host immune system to detect) ICD.Citation8,Citation10–Citation21 Based on a series of fundamental studies, preclinical validations and clinical biomarker assessmentsCitation22-Citation24 ICD can be defined as a functionally unique RCD subtype that is sufficient for the elicitation of adaptive immunity specifically directed toward antigens derived from cell “corpses”.Citation4,Citation9,Citation12,Citation15,Citation21,Citation25–Citation36 It is now well acknowledged that, upon antigenic priming coupled to the emission of damage-associated molecular patterns (DAMPs) and immunostimulatory cytokines,Citation8,Citation9,Citation12,Citation15,Citation19–Citation21,Citation27-Citation29,Citation31,Citation36–Citation50 cancer cells undergoing ICD effectively enable the expansion of (mostly preexisting but possibly also de novo) T cells specific for tumor-associated antigens (TAAs) and/or tumor-specific antigens (TSAs; also known as tumor neoantigens, TNAs).Citation4,Citation12,Citation15,Citation21,Citation51–Citation57 Nevertheless, based on currently available evidence, it is safe to assume that although ICD-elicited antigen-specific T cell clones might be “rich” in terms of TCR diversity (i.e. overall amount of T cells with unique antigen-reactive TCRs),Citation58–Citation63 their “evenness” (i.e. uniform distribution of unique TCR-possessing T cells) might be limited due to various constrains specific to oncological contexts.Citation56,Citation64–Citation67 Such constraints include: (1) the heterogeneous expression of TAAs and TSAs within the same tumor and/or across primary tumors and their metastatic lesions;Citation68–Citation76 (2) the differential ‘editing’ of T cells bearing TAA-directed TCRs by central (e.g. thymic) or peripheral (e.g. tissue) tolerance;Citation77–Citation86 (3) the relatively limited avidity of TAA-specific (and sometimes also TSA-specific) TCRs;Citation87–Citation96 and (4) the ability of cancer cells to compromise cellular fitness and/or effector functions of T cells, hence driving ‘exhaustion’ or ‘anergy’.Citation97–Citation109
Two main criteria should be met for any treatment modality to be classified as a bona fide ICD inducer.Citation20,Citation110 First, ICD-eliciting agents must exhibit superior therapeutic efficacy when employed against mouse tumors growing in immunocompetent, syngeneic (as compared to immunodeficient) hosts.Citation19,Citation111–Citation113 Second, cancer cells undergoing ICD must provide tumor-naïve, syngeneic hosts with immune-mediated prophylactic protection against a subsequent challenge with living cancer cells of the same (but not different) type.Citation4,Citation12,Citation19,Citation114–Citation119 Here, it is important to note that the first approach, while relatively straightforward, cannot be employed to discriminate ICD inducers (i.e. agents that kill cancer cells in an immunogenic manner) from immunostimulatory chemotherapies (i.e. agents that boost immune functions by acting on the host immune system).Citation16,Citation120–Citation131 An additional experimental approach to detect ICD, which can be used only for localized treatments such as radiation therapy (RT) or intra-tumoral drug delivery, consist in assessing the response of non-treated rodent tumors that have been established contra-laterally to treated lesions (so-called “abscopal response”) in immunocompetent, syngeneic hosts.Citation132–Citation134 Some ICD inducers are indeed potent at eliciting adaptive anticancer immunity even in the presence of the immunosuppressive circuitries that are established by developing tumors, and not only in tumor-naïve settings.Citation110,Citation135–Citation146
In addition to these in vivo experiments, which obligatorily rely on rodent cancer cells established in immunocompetent, syngeneic hosts, some in vitro or ex vivo proxy methods are available to estimate the immunogenic potential of dying cancer cells (as long as all appropriate positive and negative controls are thoroughly evaluated).Citation12,Citation19,Citation77,Citation147–Citation150 The main advantage of these methods is that they can be employed for both rodent and human cancer cells. In this context, cancer cells potentially undergoing ICD can be examined for the release or exposure of ICD-associated DAMPs (see below),Citation12,Citation15,Citation21,Citation149,Citation151–Citation153 and/or co-cultured with myeloid cells such as dendritic cells (DCs)Citation21,Citation119,Citation154–Citation156 which are ultimately assessed for: (1) phagocytic activity,Citation157–Citation165 (2) surface activation markers (e.g. CD80, CD83, CD86, CD83, CD40 and/or MHC Class II molecules),Citation166 (3) secretory activity, with specific reference to interleukin 1 beta (IL1B), IL6, IL12 and tumor necrosis factor (TNF),Citation167–Citation172 and (4) T-cell cross-priming.Citation173–Citation181
Of note, performing these ex vivo experiments with human cells generally involves allogeneic settings, since DCs or T cells derived from healthy individuals are typically not HLA-matched to human cancer cell lines.Citation156,Citation182–Citation190 Thus, proper controls are needed for ruling out allogeneic graft-versus-host immune reactions as confounding factors.Citation191 Moreover, ex vivo experiments cannot substitute for vaccination or abscopal tests in vivo, as some compounds are capable of eliciting all the hallmarks of ICD when administered to cancer cells, and yet those cells are unable to initiate anticancer immunity.Citation192–Citation199 Thus, these assays are most suited for screening purposes, which require validation in syngeneic murine models (for rodent cancer cells), or humanized mice models capable of sustaining human myeloid and lymphoid cells (for human cancer cells, especially in the setting of patient-derived xenografts).Citation12,Citation21,Citation117,Citation119,Citation149,Citation200–Citation203
The release or exposure of ICD-associated DAMPs is regulated by multiple processes that occur in dying cancer cells,Citation204,Citation205 but the ultimate immunological success of ICD also relies on the capacity of the host to recognize DAMPs and generate robust TSA/TAA-targeting adaptive immunity.Citation1,Citation12,Citation36,Citation193,Citation206–Citation213 Chemotherapy-driven ICD is typically associated with: (1) surface exposure of calreticulin (CALR), which mediates pro-phagocytic effects,Citation205,Citation214–Citation216 (2) active or passive release of ATP, which operates as a short-range ‘find me’ signal and inflammasome activator;Citation217–Citation221 (3) passive release of the non-histone chromatin-binding protein high-mobility group box 1 (HMGB1), which operates as an agonist of Toll-like receptor 4 (TLR4) and Advanced glycosylation end-product specific receptor (AGER);Citation57,Citation222,Citation223 (4) active or passive release of annexin A1 (ANXA1), a formyl peptide receptor 1 (FPR1) agonist;Citation155 (5) active secretion of immunostimulatory and chemotactic cytokines, including type I interferon (IFN), C-C motif chemokine ligand 2 (CCL2), C-X-C motif chemokine ligand 1 (CXCL1) and CXCL10;Citation5,Citation24,Citation224–Citation231 and (6) passive release of nucleic acids, which can engage TLR3, TLR7/8 and/or TLR9.Citation231–Citation233
These danger signals have been robustly associated with ICD induced by anthracyclines (i.e. idarubicin, epirubicin, doxorubicin, and mitoxantrone), but some minor, context-dependent variations exist for ICD elicited by cyclophosphamide and bortezomib.Citation234 Moreover, ICD driven by other cellular stressors including RT, photodynamic therapy, extracorporeal photochemotherapy and oncolytic virotherapy is not necessarily associated with the emission of the same DAMPs, cytokines and chemokines.Citation12,Citation235–Citation238 Altogether and in combination with an increased microenvironmental availability of TAAs or TSAs, ICD-associated DAMPs pave the way to: (1) abundant recruitment of antigen-presenting cells (APCs) or their precursors (as in the case of ATP, CCL2, CXCL1, ANXA1) and/or T cells (as in the case of CXCL10) to the tumor microenvironment; (2) efficient phagocytic uptake of dead/dying cancer cells and fragment thereof in the context of immunostimulatory signaling (as in the case of CALR); and, (3) potent functional activation of APCs (as in the case of ATP, HMGB1 and nucleic acids).Citation12,Citation24,Citation57,Citation113,Citation119,Citation224,Citation237 Ultimately, APCs engulfing TAAs or TSAs and receiving these immunostimulatory cues acquire an extraordinary ability to cross-present TAA- or TSA-derived epitopes to CD4+ and/or CD8+ T cells in the context of co-stimulation, which enables TAA/TSA-targeting immunity.Citation239–Citation244
In multiple oncological settings, cancer cells capable of undergoing ICD in response to microenvironmental or therapeutic stress are subjected to increased immunological pressure,Citation245 resulting in the selection of poorly immunogenic tumor variants displaying: (1) reduced antigenicity (due to TAA/TSA loss or defects in MHC Class I exposure);Citation246–Citation253 (2) genetic or epigenetic annihilation of the intracellular stress pathways that support the emission of ICD-associated DAMPs, cytokines or chemokines;Citation254 and/or (3) direct genetic or epigenetic silencing of specific DAMPs (e.g. CALR) or type I IFN.Citation57,Citation119,Citation214,Citation221,Citation224,Citation231,Citation255 Alongside general immunological defects, there can also exist disruption in the detection of DAMPs that are normally emitted by cancer cells undergoing ICD.Citation256 Such conditions include: (1) a prominent immunological tolerance determined by the specific anatomical location of the tumor (as in the case of the brain, mucosal surfaces and other immunologically privileged sites);Citation129,Citation257–Citation259 (2) an abundant and persistent release of immunosuppressive cytokines such as IL10;Citation260–Citation264 (3) a robust production of factors that favor immune exclusion, such as transforming growth factor beta 1 (TGFB1);Citation265–Citation270 (4) abundant tumor infiltration by immunosuppressive immune cells like myeloid-derived suppressor cells (MDSCs);Citation271–Citation281 (5) elevated expression of co-inhibitory receptors, such as programmed cell death 1 (PDCD1, best known as PD-1) and hepatitis A virus cellular receptor 2 (HAVCR2, best known as TIM-3);Citation271–Citation273,Citation282-Citation284 (6) lymphoid T cell depletion as a consequence of vascular exclusion;Citation77,Citation79,Citation279,Citation285–Citation287 and (7) cancer cell resistance to RCD driven by immune effector cells.Citation271,Citation285,Citation288
Not surprisingly, only a few chemotherapeutic agents can cause the immunogenic demise of cancer cells.Citation12,Citation21,Citation130 Moreover, there is no clear structure-function relationship to assist the prediction of ICD inducers. Thus, even though cisplatin and oxaliplatin exhibit considerable structural overlap and share modus operandi as for their capacity to elicit ICD,Citation289 the latter but not the former drives bona fide ICD.Citation290 Similar observations apply to the DNA alkylating agents, melphalan (which is unable to cause ICD) and cyclophosphamide (a bona fide ICD inducer).Citation291 The differential ability of cisplatin (or melphalan) and oxaliplatin (or cyclophosplamide) to promote ICD reflects their uneven capacity to cause endoplasmic reticulum (ER) stress and hence favor the exposure of CALR and other ER chaperones on the surface of dying cells.Citation290–Citation294
Common chemotherapeutics that have been demonstrated to cause ICD include (but may not be limited to): (1) idarubicin, which is generally employed for the treatment of acute myeloid leukemia (AML);Citation214,Citation295–Citation297 (2) epirubicin, which is used in women with breast cancer;Citation4,Citation214,Citation296,Citation297 (3) doxorubicin, which is approved for the treatment of AML, acute lymphoblastic leukemia (ALL), Wilms’ tumors, breast cancer, lymphoma, gastric cancer, small cell lung carcinoma, neuroblastoma, multiple myeloma, thyroid cancer, sarcomas, ovarian cancer, and bladder cancer;Citation4,Citation214,Citation298–Citation304 (4) mitoxantrone, which is licensed for use in patients with AML, non-Hodgkin’s lymphoma (NHL), breast cancer, and prostate carcinoma;Citation4,Citation214,Citation296,Citation297 (5) oxaliplatin, which is commonly employed in combinatorial regimens against colorectal carcinoma;Citation289,Citation290,Citation305–Citation311 (6) bortezomib, which is approved for the clinical management of mantle cell lymphoma and multiple myeloma;Citation312–Citation322 (7) cyclophosphamide, which is frequently employed in patients with AML, ALL, chronic myeloid leukemia (CML), chronic lymphocytic leukemia (CLL), lymphoma, multiple myeloma, ovarian cancer, breast cancer, neuroblastoma and retinoblastoma.Citation318,Citation323–Citation332 Finally, some chemotherapeutic agents can enhance the immunogenic potential of RCD to some degree, but not sufficiently to drive robust ICD, owing to a variety of limiting circumstances. This applies to taxanes (e.g. paclitaxel, docetaxel), bleomycin and vinca alkaloids.Citation15,Citation113,Citation333
Of note, even though ICD is a major immunostimulatory pathway activated by the aforementioned chemotherapies (at least in preclinical settings), the same molecules can also boost anticancer immunity by, (1) targeting nonmalignant cells (e.g. immune cells, stromal cells, endothelial cells), and/or (2) by improving the immunogenicity of cancer cells independently of their demise (e.g. by activating the expression of NK cell-activatory ligands).Citation15,Citation141,Citation334–Citation339 Finally, most immuno-oncology trials do not select chemotherapeutics based on their immunostimulatory potential (which in many cases does not manifest at clinically employed dose regimens), but rather based on their use a standard-of-care for selected indications. At least in some cases, such a design precludes the activation of clinically meaningful anticancer immune responses and hence limits the clinical benefit of combinatorial regimens.
In this edition of the Trial Watch series, we discuss recent preclinical and clinical developments on ICD induction by anticancer chemotherapeutics in the context of immuno-oncology. Of note, other inducers of ICD including RT,Citation225,Citation340–Citation344 oncolytic virotherapy,Citation345 high hydrostatic pressure,Citation12,Citation15,Citation346 photodynamic therapy and extracorporeal photochemotherapyCitation119,Citation215,Citation236,Citation237,Citation347 will not be discussed herein.
Recent preclinical advances
Since the publication of the latest Trial Watch dealing with ICD elicited by chemotherapy (September 2017),Citation20 several preclinical studies on this topic have been published in peer-reviewed scientific journals. Amongst these studies, we found the following ones to be of particular (representative) importance.
As compared to past decade (which was dominated by fundamental studies aimed at elucidating the molecular and cellular mechanisms underlying ICD and its detection)Citation20 the majority of the studies published in the last 2 years had a translational approach, largely reflecting the trend of immuno-oncology at large.Citation348,Citation349 That said, at least a few studies provided fresh insights into the fundamentals of ICD. For instance, Bezu et al. (Center de Recherche des Cordeliers, Paris, France) observed that prototypical ICD inducers such as anthracyclines cause the phosphorylation of eukaryotic translation initiation factor 2A (EIF2A, best known as eIF2α) without consistently triggering other manifestations of ER stress, and that EIF2A phosphorylation strongly correlates with surface CALR exposure, de facto constituting a pathognomonic marker of ICD.Citation350 Lecciso and colleagues (University of Bologna, Bologna, Italy) documented that the release of extracellular ATP from daunorubicin-treated AML cells can elicit immunosuppressive (rather than immunostimulatory) effects within the tumor microenvironment by favoring the persistence of the regulatory T (TREG) cells,Citation351 which can be distinguished by increased expression of PD-1, and tolerogenic DCs, which can be identified by increased indoleamine 2,3-dioxygenase 1 (IDO1) and ectonucleoside triphosphate diphosphohydrolase 1 (ENTPD1, best known as CD39) expression.Citation352 These studies highlight the need for better understanding the ‘plasticity’ associated with ICD-linked danger signaling especially in the context of resistance to immunotherapy.Citation353,Citation354
On the translational side, several preclinical studies reported the generation of nanoparticles or other nanoformulations for the improved delivery of ICD-inducing chemotherapies.Citation355 Mastria et al. (Duke University, Durham, NC, USA) documented that a nanoparticle preparation of doxorubicin, i.e. chimeric polypeptide doxorubicin, efficiently enhances anticancer immunity as it favors tumor infiltration by T cells (including CD8+ T cells) and limits primary tumor growth as well as metastatic spread.Citation356 Yang and collaborators (National Institutes of Health, Bethesda, MD, USA) observed that an integrated polymersomal nanoformulation (consisting of a chimeric, cross-linked polymersome encapsulating doxorubicin and a photosensitizer that can be activated via photodynamic therapy) drives in situ, DC-dependent anticancer vaccination, hence retarding the progression of mouse MC38 colorectal tumors.Citation357 Lu and colleagues (University of California, Los Angeles, CA, USA) conjugated an IDO1 inhibitor (indoximod)Citation205 to oxaliplatin-bearing nanovesicles, and demonstrated that this nano-enabled approach (delivered through vaccination, intravenous or intratumoral injection) induces potent anticancer immunity in an orthotopic pancreatic ductal adenocarcinoma (PDAC) mouse model.Citation358 Huang et al. (Hainan Medical College, Haikou, China) developed a system for the ultrasound-controlled release of doxorubicin by liposome-microbubble complexes, resulting in superior ICD induction in lung (LL/2) and colorectal cancer (CT26) syngeneic murine models.Citation205 Finally, Liu and collaborators (University of North Carolina at Chapel Hill, Chapel Hill, NC, USA) achieved the targeted delivery of mitoxantrone and celastrol (a triterpenoid) via a tumor microenvironment-responsive nanocarrier, hence efficiently causing ICD-dependent therapeutic effects in vivo that halted cancer progression, and repressed metastatic spread.Citation359
Beyond these nanotechnology-oriented studies, most of the other translational studies on ICD-inducing chemotherapies published over the past 2 years focused on combining ICD induction with immunotherapy or targeted anticancer agents, in keeping with current clinical trends.Citation360 D’Amico et al. (University of Basel, Basel, Switzerland) found that an antibody specific for Erb-B2 receptor tyrosine kinase 2 (ERBB2, also known as HER2) conjugated to an anthracycline derivate exerts potent anticancer effects that depend on cytotoxic T cells in a ERBB2-expressing syngeneic breast cancer model resistant to standard anti-ERBB2 therapy, and that this therapeutic effect can be further enhanced by PD-1 blockage.Citation205 Fend and collaborators (Gustave Roussy Cancer Campus, Villejuif, France) reported that an engineered oncolytic vaccinia virus, VVWR-TK−RR–Fcu1, can mediate ICD-dependent therapeutic effects (as documented by type I IFN signaling, increased CD8+ T cell infiltration, and improved ratio of effector CD4+ T cells to TREG cells in the tumor microenvironment) that can be potentiated by ICD-inducing chemotherapeutics or immune checkpoint blockers (ICBs).Citation361 Camilio and colleagues (Oslo University Hospital, Oslo, Norway) documented that combining the oncolytic peptide LTX-315 with doxorubicin elicits anticancer immune responses that limit tumor growth along with increased infiltration of CD4+ and CD8+ T cells.Citation362 Groza et al. (University of Vienna, Vienna, Austria) combined “bacterial ghosts” (i.e. empty envelopes of Gram-negative bacteria) with oxaliplatin to elicit therapeutically relevant T cell responses against CT26 mouse colorectal tumors coupled to the establishment of long-term immunological memory.Citation363 Gao and collaborators (University of Science and Technology of China, Hefei, China) found that, as compared to the either agent delivered as standalone therapy, the co-administration of doxorubicin and a small molecule IDO1 inhibitor (NLG919) profoundly inhibits the growth of 4T1 mouse mammary tumors in vivo.Citation364 Gebremeskel and colleagues (Beatrice Hunter Cancer Research Institute, Halifax, Nova Scotia, Canada) combined cyclophosphamide, gemcitabine and α-galactosylceramide (α-GalCer)-loaded DCs (which potently activate NKT cells), achieving disease eradication and long-term immunological protection in mice bearing 4T1 tumors, as demonstrated by their ability to reject a subsequent challenge with the same cancer cells.Citation365 Nam et al. (Korea University, Seoul, Republic of South Korea) employed ICD-inducing chemotherapy, as they boosted the phagocytic activity of APCs by blocking rho-associated coiled-coil containing protein kinase 1 (ROCK1), to activate effectual anti-tumor immunity (distinguished by improved T cell priming by DCs), causing considerable inhibition of tumor growth in multiple mouse models.Citation200 Combes and coauthors (Université de Montpellier, Montpellier, France) found that the resistance of colorectal cancer cells to oxaliplatin can be circumvented by inhibition of ATR serine/threonine kinase (ATR), resulting in robust therapeutic effects that largely depend on immune effectors.Citation366 Finally, Truxova et al. (Sotio, Prague, Czech Republic) demonstrated that AML patients whose blasts naturally expose CALR on the plasma membrane experience a survival advantage that is associated with improved NK cell functions downstream of superior type I IFN secretion and IL15 trans-presentation by myeloid cells.Citation205,Citation216
Altogether, these studies demonstrate that ICD-inducing chemotherapy can initiate robust anticancer immunity, which can be further potentiated by multiple immunotherapeutic regimens currently employed in the clinic. That said some cancers display immunological alterations that prevent the activation of tumor-targeting immunity by malignant cells undergoing ICD. This constitutes a major obstacle to efficacy of ICD-inducing chemotherapy, and further preclinical studies are required for the development of efficacious combinatorial regimens.
Finalized clinical studies
Subsequent to the publication of our previous Trial Watch on ICD-inducing chemotherapeutics (September 2017),Citation20 various clinical studies have assessed the efficacy of bona fide ICD-inducing chemotherapeutics (i.e. doxorubicin, epirubicin, idarubicin, mitoxantrone, bortezomib, cyclophosphamide or oxaliplatin), most often in combination with immunotherapy and in the context of immunomonitoring programs. These clinical reports were identified by querying PubMed (http://www.ncbi.nlm.nih.gov/pubmed) with the string “(cancer OR tumor OR tumor OR neoplasm) AND (oxaliplatin OR cyclophosphamide OR bortezomib OR doxorubicin OR epirubicin OR idarubicin OR mitoxantrone) AND (immune OR immunogenic OR “immunogenic cell death” OR immunological)”, accompanied by an article-type filter (Article types>Clinical trial) and followed by a manual selection of articles for direct relevance to this Trial Watch.
Voorwerk et al. (The Netherlands Cancer Institute, Amsterdam, the Netherlands) treated 67 patients with metastatic triple-negative breast cancer (TNBC), with a PD-1-targeting ICB (nivolumab) in combination with various conventional cancer treatments including ICD inducers (RT, cyclophosphamide, doxorubicin) as well as a non-ICD inducer (cisplatin). In the context of this Phase II trial (TONIC), the best objective response rates (ORRs) were achieved by nivolumab plus doxorubicin (35%), which was superior to nivolumab plus cisplatin (23%).Citation367 In consideration of the multi-arm design of this clinical study, we interpret these data as convincing clinical evidence in favor of combining bona fide ICD-inducing chemotherapeutics with ICBs, at least in the context of TNBC.Citation367 Scurr and collaborators (Cardiff University, Cardiff, UK) reported the results of a randomized Phase I/II study enrolling 55 metastatic colorectal cancer patients. In this context, cyclophosphamide combined with a modified vaccinia virus Ankara-5T4 (MVA-5T4) elicited robust anticancer immunity (as assessed by humoral immunological markers as well as by circulating TREG depletion), culminating with protracted patient survival in the absence of severe toxicities.Citation368 Federico and colleagues (University of Tennessee Health Science Center, Memphis, TN, USA) documented that the combination of various chemotherapeutic regimens (cyclophosphamide plus topotecan, irinotecan plus temozolomide and ifosfamide plus carboplatin plus etoposide) with a monoclonal antibody specific for ganglioside G2 (hu14.18K322A), recombinant cytokines, and adoptively transferred NK cells not only is feasible in children suffering from refractory/recurrent neuroblastoma, but also exhibits robust clinical activity, as demonstrated by an ORR of 61.5%.Citation369
Bota et al. (University of California Irvine, Irvine, CA, USA) reported that combining an allogeneic/autologous vaccine (ERC1671) with recombinant colony stimulating factor 2 (CSF2, best known as GM-CSF), cyclophosphamide and bevacizumab (a monoclonal antibody specific VEGFA)Citation205 results in a clinically-relevant survival benefit in glioblastoma patients (12 months vs. 7.5 months for patients receiving bevacizumab only).Citation370 Kanekiyo and colleagues (Yamaguchi University Graduate School of Medicine, Yamaguchi, Japan) combined a vaccine based on 5 HLA-A*24:02-restricted peptides with oxaliplatin in patients with colorectal cancer, finding humoral responses to multiple peptides that were associated with cytotoxic T-cell responses and/or improved overall survival (OS).Citation371 Geyer and collaborators (Memorial Sloan Kettering Cancer Center, New York, NY, USA) employed CD19-targeting chimeric antigen receptor (CAR) T cells in patients afflicted by residual CLL upon chemotherapy with pentostatin (is a purine analog that inhibits nucleic acid synthesis), cyclophosphamide and rituximab (a CD20-targeting monoclonal antibody).Citation205 This approach achieved 38% ORR, with two patients exhibiting complete responses exceeding 28 months in the absence of severe cytokine release syndromes.Citation372
Other clinical studies focused on assessing biomarkers of immune activation in patients receiving ICD-inducing chemotherapeutics. Foukakis et al. (Karolinska University Hospital, Stockholm, Sweden) documented that clinical responses to anthracycline-based neoadjuvant chemotherapy amongst 109 patients with breast cancer were more frequent when tumors were characterized by a transcriptional signature that the authors named “immune module score”.Citation373 Similarly, Kwa and collaborators (NYU Langone Medical Center, New York, NY, USA) observed that the administration of cyclophosphamide plus exemestane (an aromatase inhibitor, belonging to the class of anti-estrogens agents)Citation205 to women with breast cancer resulted in clinical responses that were accompanied by increases in the circulating levels of various effector T cell subsets (but limited changes in blood-borne TREG cells).Citation374 Conversely, Werter and colleagues (VU University Medical Center, Amsterdam, The Netherlands) were unable to document (in the context of a Phase I clinical trial) any clinical benefit for patients with renal cell carcinoma receiving cyclophosphamide plus everolimus (an MTORC1 inhibitor),Citation205 despite successful depletion of circulating TREG cells.Citation375 Along similar lines, Toulmonde et al. (Institut Bergonié, Bordeaux, France) found that a PD-1-targeting ICB combined with cyclophosphamide has limited clinical activity in patients with advanced soft-tissue sarcoma and gastrointestinal stromal tumor (GIST).Citation376 These latter findings have been attributed to the elevated degree of immunosuppression that characterize at least some subsets of soft-tissue sarcoma and GIST, which are characterized by robust infiltration by macrophages and prominent IDO1 activity.Citation376 Stevens and colleagues (Radboudumc, Nijmegen, The Netherlands) reported that wild-type enhancer of zeste 2 polycomb repressive complex 2 subunit (EZH2) status, chromosome 18 gain, and low amounts of CD68+CD163+ cells were potent predictors of therapeutic failure of multimodal, cyclophosphamide- and doxorubicin-based chemotherapy plus rituximab in patients with follicular lymphoma.Citation377 Finally, Aspeslagh et al. (Gustave Roussy Cancer Campus, Villejuif, France) documented, (1) that cancer patients receiving first-line ICBs against PD-1 or CD274 (best known as PD-L1) obtain limited therapeutic benefit from the subsequent administration of conventional chemotherapy (irrespective of their ability to induce ICD), and that (2) prior exposure to ICD-inducing chemotherapeutics (e.g. oxaliplatin, cyclophosphamide, doxorubicin, epirubicin, bortezomib) did not seem to improve the activity of ICBs administered subsequently.Citation378
Importantly, some clinical studies lent support to the notion that the failure of ICD-inducing chemotherapy does not compromise the activity of immunotherapy, at least in some settings. Thus, Overman et al. (MD Anderson Cancer Center, Houston, TX, USA) treated patients with defective mismatch repair (dMMR) or microsatellite instability-high (MSI-H) metastatic colorectal cancer progressing on standard-of-care chemotherapeutics (including oxaliplatin) with nivolumab, observing 68.9% (51/74) patients on tumor control exceeding 12 weeks.Citation379
Taken together, these studies reveal that ICD-inducing chemotherapy may provide immunological benefits to cancer patients that are accompanied by clinical activity, at least in some settings and especially in the context of immunotherapy. However, there are oncological indications in which the immunological and clinical effects of ICD-inducing chemotherapeutics remain debatable. It will therefore be critical to identify the precise oncological indications and/or contexts in which the initiation of ICD by chemotherapy provides maximal therapeutic advantage. Current evidence points to breast carcinoma and colorectal carcinoma as putative settings in which ICD inducers can be favorably combined with immunotherapy for optimal clinical activity.
Ongoing clinical studies
When the current Trial Watch was redacted the ClinicalTrials.gov database (http://www.clinicaltrials.gov/) listed no less than 103 clinical studies that matched the following criteria: (1) they involved at least one bona fide ICD-inducing chemotherapeutic agent; (2) they were implemented in the context of immunomonitoring; and (3) they were initiated after July 2017 (when the latest Trial Watch on this topic was published)Citation20 (, and ).
Table 1. Contemporary clinical studies assessing the therapeutic and immunological characteristics of ICD-inducing chemotherapies
Table 2. Contemporary clinical studies assessing the therapeutic and immunological characteristics of cyclophosphamide
Figure 1. Current clinical studies testing immunogenic cell death (ICD)-inducing chemotherapy in combination with immunotherapy for oncological indications. Clinical studies were classified based on: (a) immunomonitoring approach, (b) indication, (c) ICD-inducing drug, and (d) combinatorial immunotherapy. CAR, chimeric antigen receptor; CRC, colorectal carcinoma; DC, dendritic cell; DTH, delayed-type hypersensitivity; GBM, glioblastoma; HPV, human papillomavirus; IHC, immunohistochemistry; NK, natural killer; NKT, natural killer T; TIL, tumor-infiltrating lymphocyte; TLR, toll-like receptor
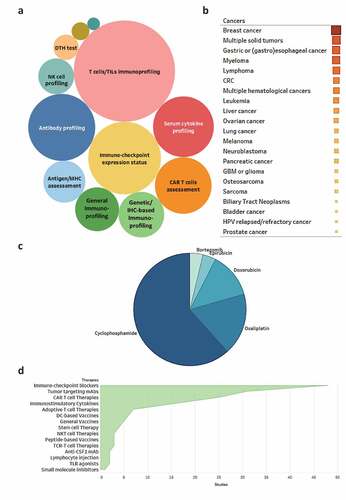
In this context, multiple immunological biomarkers (including several biomarkers of ICD) are being examined (, and ), including: (1) T-cell immunoprofiling, including assessment of T cell activation, suppression, phenotype, and exhaustion, as well as the quantification of tumor-infiltrating or circulating T cells; (2) quantification of immunosuppressive ligands (e.g. PD-L1) and/or their receptors (e.g. PD-1, TIM-3) in the tumor microenvironment; (3) assessment of humoral immune responses specific for TAAs; (4) quantification of blood-borne cytokines relevant for antitumor immunity, including (but not limited to) IL6, TNF, interferon beta 1 (IFNB1), and interferon gamma (IFNG); and (5) immunological assessment of CAR T cell number and activity (mostly in the circulation). In addition, multiple ongoing clinical studies are evaluating immunological biomarkers in an unbiased fashion by harnessing omics approaches such as bulk or single-cell RNA sequencing and multispectral immunohistochemistry. These clinical studies are expected to provide valuable information on genetic signatures or functional patterns that are associated with T cell activation and clinical activity downstream of ICD induction by chemotherapy, potentially leading to the development of prognostic or predictive biomarkers.
In line with previously documented trends,Citation20 breast carcinoma is one of the most common oncological indications for the use of ICD-inducing chemotherapy in combination with immunotherapy. In addition, multiple relatively nonselective “basket trials” have been initiated to test this therapeutic paradigm in cohorts of patients with various solid and hematological malignancies, including gastric and (gastro)esophageal cancer, myeloma, lymphoma, colorectal carcinoma and others (, and ). Overall, clinical trials enrolling individuals with solid tumors are more common as compared to studies accruing patients with hematological malignancies. Although multiple reasons may underlie such an apparent discrepancy, it is tempting to speculate that overall disease incidence and responsiveness to standard-of-care treatment may play a prominent role in this scenario. Of note, ICD-inducing chemotherapeutics are being tested in subjects with hematological tumors most often in combination with CAR T cells, in order to maximize the clinical activity of the latter and/or to expand it to specific malignancies that are not particularly sensitive upfront, such as liver, lung, ovarian or prostate cancers (amongst others).Citation205
Most of the clinical studies charted in this survey plan to administer cyclophosphamide, oxaliplatin, doxorubicin, epirubicin, or bortezomib, not only as archetypally on-label interventions but also as components of standard-of-care regimens (, and ). In a limited fraction of cases, assessment of ICD induction by treatment is a primary objective of the study. Cyclophosphamide is often being used with the principal aim of inhibiting or depleting tumor-infiltrating or circulating TREG cells (). Common combinatorial partners for ICD-eliciting chemotherapeutics include other (non-chemotherapeutic) ICD inducers such as RT, as well as chemotherapeutic agents that boost the immunogenic potential of cancer cells without inducing ICD,Citation380 such as melphalan, docetaxel or paclitaxel ( and ).
Moreover, in line with the contemporary immuno-oncology landscape, most oncologists are combining ICD-inducing chemotherapeutics with active or passive immunotherapy (, and ). These generally consist of: (1) ICBs targeting PD-1, such as nivolumab, pembrolizumab, or SHR-1210; PD-L1, like atezolizumab, durvalumab, or avelumab; cytotoxic T-lymphocyte associated protein 4 (CTLA4; such as ipilimumab or tremelimumab; or killer cell lectin-like receptor C1 (KLRC1, best known as NKG2A, such as monalizumab; (2) immunostimulatory monoclonal antibodies such as CD40 agonistic antibodies; (3) tumor-targeting antibodies specific for CD38, CD20, epidermal growth factor receptor (EGFR), VEGFA, IL6, or ERBB2; (4) adoptively transferred T cells, including T cells engineered to express TAA-specific CARs; (5) immunostimulatory cytokines including GM-CSF, CSF3, IL2, IFNA or IRX-2 (a cell-free mixture of IL1B, IL2, IL6, IL8, IL10, IL12, TNF and IFNG);Citation205 (6) DC-based vaccines, amongst others (, and ). These trends deviate from those we documented in the previous Trial Watch dealing with ICD-inducing chemotherapy,Citation20 in that ICBsCitation271,Citation381,Citation382 and CAR T cellsCitation383-Citation385 have substituted immunostimulatory cytokines as the most common combinatorial partner for ICD inducers. Overall, these trends mirror current expectations on the ability of various immunotherapeutic agents, notably ICBs, to achieve optimal efficacy once ICD is initiated by optimal chemotherapeutic regimens, especially in oncological indications that are poorly sensitive to either approach employed alone. Ongoing clinical studies will shed some light on this possibility, with specific reference to which precise ICD inducer should be employed in combination with which immunotherapeutic agent, for which indication and according to which schedule.
Concluding remarks
Multiple chemotherapeutic agents that induce bona fide ICD are presently approved by regulatory authorities worldwide for use in patients with a wide variety of malignant disorders (encompassing hematological and solid tumors). However, these chemotherapeutics have been largely developed (preclinically) in human xenografts established in highly immunodeficient miceCitation205 and translated to the clinic according to ever more obsolete concept of maximum tolerated dose (MTD), in the absence of any immunomonitoring.Citation386 Thus, ICD inducers are currently employed according to doses and treatment schedules that ensure maximal cytotoxicity in the context of limited side effects on normal tissues, but do not consider potential inputs from the host immune systems. In line with this notion, two of the most common side effects of chemotherapy are neutropenia and lymphopenia, implying that the ICD inducers employed according to current standards are toxic to immune cells and favor (at least some degree of) immunodeficiency.Citation119,Citation154,Citation387–Citation389 Even beyond the induction of ICD, several chemotherapeutics have been shown to elicit on-target or off-target immunostimulatory doses, especially when employed at low doses and/or according to revisited treatment protocols.Citation390–Citation393 As we stand at the apex of the immunotherapy revolution, the immunomodulatory effects of traditional anticancer agents, including chemotherapy, RT and others, can no longer be ignored. Thus, preclinical studies based on immunocompetent models followed by well-designed and highly immunomonitored clinical trialsCitation394 are urgently awaited to identify new doses and treatment schedules that enable maximal immunostimulation by chemotherapy and hence set an optimal stage for combination with ICBs and other forms of immunotherapy.
Disclosure of potential conflicts of interest
DDR received financial assistance from Boehringer Ingelheim, Bristol-Myers Squibb, AstraZeneca, Philips and Olink. He is also in the advisory board of Bristol-Myers Squibb, Celgene, Merck/Pfizer, Roche/Genentech, AstraZeneca, MSD and Seattle Genetics. DDR has been involved in advisory capacity (non-financial) with NOXXON and MOLOGEN. Other authors have no particular conflict of interests to declare with relation to this particular manuscript. LG provides remunerated consulting to Astra Zeneca, Boehringer Ingelheim, Inzen, OmniSEQ, and the Luke Heller TECPR2 Foundation, and receives research funding from Lytix, Phosplatin and Sotio.
Acknowledgments
ADG is supported by Research Foundation Flanders’ (FWO) Excellence of Science (EOS) grant (30837538) for the ‘DECODE’ consortium and the KU Leuven via the C1 grant (C14/19/098) as well as the POR award funds (POR/16/040). ST is supported by Research Foundation Flanders’ (FWO) as a Senior Clinical Investigator and by the Stichting tegen Kanker. GK is supported by the Ligue contre le Cancer (Équipe Labellisée); Agence National de la Recherche (ANR) – Projets blancs; ANR under the frame of E-Rare-2, the ERA-Net for Research on Rare Diseases; Association pour la recherche sur le cancer (ARC); Cancéropôle Ile-de-France; Chancelerie des universités de Paris (Legs Poix), Fondation pour la Recherche Médicale (FRM); a donation by Elior; European Research Area Network on Cardiovascular Diseases (ERA-CVD, MINOTAUR); Gustave Roussy Odyssea, the European Union Horizon 2020 Project Oncobiome; Fondation Carrefour; High-end Foreign Expert Program in China (GDW20171100085 and GDW20181100051), Institut National du Cancer (INCa); Inserm (HTE); Institut Universitaire de France; LeDucq Foundation; the LabEx Immuno-Oncology; the RHU Torino Lumière; the Seerave Foundation; the SIRIC Stratified Oncology Cell DNA Repair and Tumor Immune Elimination (SOCRATE); and the SIRIC Cancer Research and Personalized Medicine (CARPEM). The LG lab is supported by a Breakthrough Level 2 grant from the US Department of Defense (DoD), Breast Cancer Research Program (BCRP) (#BC180476P1), by a startup grant from the Dept. of Radiation Oncology at Weill Cornell Medicine (New York, US), by industrial collaborations with Lytix (Oslo, Norway) and Phosplatin (New York, US), and by donations from Phosplatin (New York, US), the Luke Heller TECPR2 Foundation (Boston, US) and Sotio a.s. (Prague, Czech Republic).
References
- Zhou J, Wang G, Chen Y, Wang H, Hua Y, Cai Z. Immunogenic cell death in cancer therapy: present and emerging inducers. J Cell Mol Med. 2019;23:4854–23. doi:10.1111/jcmm.2019.23.issue-8.
- Bezu L, Sauvat A, Humeau J, Leduc M, Kepp O, Kroemer G. eIF2alpha phosphorylation: A hallmark of immunogenic cell death. Oncoimmunology. 2018;7:e1431089. doi:10.1080/2162402X.2018.1431089.
- Twumasi-Boateng K, Pettigrew JL, Kwok YYE, Bell JC, Nelson BH. Oncolytic viruses as engineering platforms for combination immunotherapy. Nat Rev Cancer. 2018;18:419–432. doi:10.1038/s41568-018-0009-4.
- Casares N, Pequignot MO, Tesniere A, Ghiringhelli F, Roux S, Chaput N, Schmitt E., Hamai A., Hervas-Stubbs S., Obeid M., et al. Caspase-dependent immunogenicity of doxorubicin-induced tumor cell death. J Exp Med. 2005;202:1691–1701. doi:10.1084/jem.20050915.
- Fang S, Agostinis P, Salven P, Garg AD. Decoding cancer cell death-driven immune cell recruitment: an in vivo method for site-of-vaccination analyses. Methods Enzymol Acad Press. 2019. doi:10.1016/bs.mie.2019.04.013.
- Russ A, Hua AB, Montfort WR, Rahman B, Riaz IB, Khalid MU, Carew JS, Nawrocki ST, Persky D, Anwer F, et al. Blocking “don’t eat me” signal of CD47-SIRPalpha in hematological malignancies, an in-depth review. Blood Rev. 2018;32:480–489. doi:10.1016/j.blre.2018.04.005.
- Syn NL, Teng MWL, Mok TSK, Soo RA. De-novo and acquired resistance to immune checkpoint targeting. Lancet Oncol. 2017;18:e731–e41. doi:10.1016/S1470-2045(17)30607-1.
- Galluzzi L, Vitale I, Aaronson SA, Abrams JM, Adam D, Agostinis P, Alnemri E.S., Altucci L., Amelio I., Andrews D.W. Molecular mechanisms of cell death: recommendations of the nomenclature committee on cell death 2018. Cell Death Differ. 2018;25:486–541.
- Tran E, Robbins PF, Rosenberg SA. ‘Final common pathway’ of human cancer immunotherapy: targeting random somatic mutations. Nat Immunol. 2017;18:255–262. doi:10.1038/ni.3682.
- Janicka M, Gubernator J. Use of nanotechnology for improved pharmacokinetics and activity of immunogenic cell death inducers used in cancer chemotherapy. Expert Opin Drug Deliv. 2017;14:1059–1075. doi:10.1080/17425247.2017.1266333.
- Radogna F, Diederich M. Stress-induced cellular responses in immunogenic cell death: implications for cancer immunotherapy. Biochem Pharmacol. 2018;153:12–23. doi:10.1016/j.bcp.2018.02.006.
- Galluzzi L, Buque A, Kepp O, Zitvogel L, Kroemer G. Immunogenic cell death in cancer and infectious disease. Nat Rev Immunol. 2017;17:97–111. doi:10.1038/nri.2016.107.
- Darragh LB, Oweida AJ, Karam SD. Overcoming resistance to combination radiation-immunotherapy: a focus on contributing pathways within the tumor microenvironment. Front Immunol. 2018;9:3154. doi:10.3389/fimmu.2018.03154.
- Petrie EJ, Czabotar PE, Murphy JM. The structural basis of necroptotic cell death signaling. Trends Biochem Sci. 2019;44:53–63. doi:10.1016/j.tibs.2018.11.002.
- Garg AD, Galluzzi L, Apetoh L, Baert T, Birge RB, Bravo-San Pedro JM, Breckpot K, Brough D, Chaurio R, Cirone M, et al. Molecular and translational classifications of damps in immunogenic cell death. Front Immunol. 2015;6:588. doi:10.3389/fimmu.2015.00588.
- Zhao X, Subramanian S. Intrinsic resistance of solid tumors to immune checkpoint blockade therapy. Cancer Res. 2017;77:817–822. doi:10.1158/0008-5472.CAN-16-2379.
- Wilson AL, Plebanski M, Stephens AN. New trends in anti-cancer therapy: combining conventional chemotherapeutics with novel immunomodulators. Curr Med Chem. 2018;25:4758–4784. doi:10.2174/0929867324666170830094922.
- Wang Q, Ju X, Wang J, Fan Y, Ren M, Zhang H. Immunogenic cell death in anticancer chemotherapy and its impact on clinical studies. Cancer Lett. 2018;438:17–23. doi:10.1016/j.canlet.2018.08.028.
- Kepp O, Senovilla L, Vitale I, Vacchelli E, Adjemian S, Agostinis P, Apetoh L, Aranda F, Barnaba V, Bloy N, et al. Consensus guidelines for the detection of immunogenic cell death. Oncoimmunology. 2014;3:e955691. doi:10.4161/21624011.2014.955691.
- Garg AD, More S, Rufo N, Mece O, Sassano ML, Agostinis P, Zitvogel L, Kroemer G, Galluzzi L. Trial watch: immunogenic cell death induction by anticancer chemotherapeutics. Oncoimmunology. 2017;6:e1386829. doi:10.1080/2162402X.2017.1386829.
- Kroemer G, Galluzzi L, Kepp O, Zitvogel L. Immunogenic cell death in cancer therapy. Annu Rev Immunol. 2013;31:51–72. doi:10.1146/annurev-immunol-032712-100008.
- Lin AG, Xiang B, Merlino DJ, Baybutt TR, Sahu J, Fridman A, Snook AE, Miller V. Non-thermal plasma induces immunogenic cell death in vivo in murine CT26 colorectal tumors. Oncoimmunology. 2018;7:e1484978. doi:10.1080/2162402X.2018.1484978.
- Kasikova L, Hensler M, Truxova I, Skapa P, Laco J, Belicova L, Praznovec I, Vosahlikova S, Halaska MJ, Brtnicky T, et al. Calreticulin exposure correlates with robust adaptive antitumor immunity and favorable prognosis in ovarian carcinoma patients. J Immunother Cancer. 2019;7. In Press. doi:10.1186/s40425-019-0781-z.
- Garg AD, Agostinis P. Cell death and immunity in cancer: from danger signals to mimicry of pathogen defense responses. Immunol Rev. 2017;280:126–148. doi:10.1111/imr.2017.280.issue-1.
- Goodman AM, Kato S, Cohen PR, Boichard A, Frampton G, Miller V, Stephens PJ, Daniels GA, Kurzrock R. Genomic landscape of advanced basal cell carcinoma: implications for precision treatment with targeted and immune therapies. Oncoimmunology. 2018;7:e1404217. doi:10.1080/2162402X.2017.1404217.
- Aoto K, Mimura K, Okayama H, Saito M, Chida S, Noda M, Nakajima T, Saito K, Abe N, Ohki S, et al. Immunogenic tumor cell death induced by chemotherapy in patients with breast cancer and esophageal squamous cell carcinoma. Oncol Rep. 2018;39:151–159.
- Grenier JM, Yeung ST, Khanna KM. Combination immunotherapy: taking cancer vaccines to the next level. Front Immunol. 2018;9:610. doi:10.3389/fimmu.2018.00610.
- Khagi Y, Kurzrock R, Patel SP. Next generation predictive biomarkers for immune checkpoint inhibition. Cancer Metastasis Rev. 2017;36:179–190. doi:10.1007/s10555-016-9652-y.
- Byun DJ, Wolchok JD, Rosenberg LM, Girotra M. Cancer immunotherapy - immune checkpoint blockade and associated endocrinopathies. Nat Rev Endocrinol. 2017;13:195–207. doi:10.1038/nrendo.2016.205.
- Stevanovic S, Pasetto A, Helman SR, Gartner JJ, Prickett TD, Howie B, Robins HS, Robbins PF, Klebanoff CA, Rosenberg SA, et al. Landscape of immunogenic tumor antigens in successful immunotherapy of virally induced epithelial cancer. Science. 2017;356:200–205. doi:10.1126/science.aak9510.
- Nishino M, Ramaiya NH, Hatabu H, Hodi FS. Monitoring immune-checkpoint blockade: response evaluation and biomarker development. Nat Rev Clin Oncol. 2017;14:655–668. doi:10.1038/nrclinonc.2017.88.
- Lee J, Lee Y, Xu L, White R, Sullenger BA. Differential induction of immunogenic cell death and interferon expression in cancer cells by structured ssRNAs. Mol Ther. 2017;25:1295–1305. doi:10.1016/j.ymthe.2017.03.014.
- Garrido G, Rabasa A, Sanchez B, Lopez MV, Blanco R, Lopez A, Hernández DR, Pérez R, Fernández LE. Induction of immunogenic apoptosis by blockade of epidermal growth factor receptor activation with a specific antibody. J Immunol. 2011;187:4954–4966. doi:10.4049/jimmunol.1003477.
- Showalter A, Limaye A, Oyer JL, Igarashi R, Kittipatarin C, Copik AJ, Khaled AR. Cytokines in immunogenic cell death: applications for cancer immunotherapy. Cytokine. 2017;97:123–132. doi:10.1016/j.cyto.2017.05.024.
- Garg AD, Dudek-Peric AM, Romano E, Agostinis P. Immunogenic cell death. Int J Dev Biol. 2015;59:131–140. doi:10.1387/ijdb.150061pa.
- Ma Y, Pitt JM, Li Q, Yang H. The renaissance of anti-neoplastic immunity from tumor cell demise. Immunol Rev. 2017;280:194–206. doi:10.1111/imr.2017.280.issue-1.
- Mohme M, Riethdorf S, Pantel K. Circulating and disseminated tumour cells - mechanisms of immune surveillance and escape. Nat Rev Clin Oncol. 2017;14:155–167. doi:10.1038/nrclinonc.2016.144.
- Dyck L, Mills KHG. Immune checkpoints and their inhibition in cancer and infectious diseases. Eur J Immunol. 2017;47:765–779. doi:10.1002/eji.201646875.
- Iurescia S, Fioretti D, Rinaldi M. Targeting cytosolic nucleic acid-sensing pathways for cancer immunotherapies. Front Immunol. 2018;9:711. doi:10.3389/fimmu.2018.00711.
- Nanini HF, Bernardazzi C, Castro F, de Souza HSP. Damage-associated molecular patterns in inflammatory bowel disease: from biomarkers to therapeutic targets. World J Gastroenterol. 2018;24:4622–4634. doi:10.3748/wjg.v24.i41.4622.
- De Lorenzo G, Ferrari S, Cervone F, Okun E. Extracellular DAMPs in plants and mammals: immunity, tissue damage and repair. Trends Immunol. 2018;39:937–950. doi:10.1016/j.it.2018.09.006.
- Roh JS, Sohn DH. Damage-associated molecular patterns in inflammatory diseases. Immune Netw. 2018;18:e27. doi:10.4110/in.2018.18.e27.
- Relja B, Land WG. Damage-associated molecular patterns in trauma. Eur J Trauma Emerg Surg. 2019.
- Escandell I, Martin JM, Jorda E. Novel immunologic approaches to melanoma treatment. Actas Dermosifiliogr. 2017;108:708–720. doi:10.1016/j.ad.2017.01.017.
- Fleshner M, Crane CR. Exosomes, DAMPs and miRNA: features of stress physiology and immune homeostasis. Trends Immunol. 2017;38:768–776. doi:10.1016/j.it.2017.08.002.
- Rodriguez-Nuevo A, Zorzano A. The sensing of mitochondrial DAMPs by non-immune cells. Cell Stress. 2019;3:195–207. doi:10.15698/cst.
- Ventura MT, Casciaro M, Gangemi S, Buquicchio R. Immunosenescence in aging: between immune cells depletion and cytokines up-regulation. Clin Mol Allergy. 2017;15:21. doi:10.1186/s12948-017-0077-0.
- Montico B, Nigro A, Casolaro V, Dal Col J. Immunogenic apoptosis as a novel tool for anticancer vaccine development. Int J Mol Sci. 2018;19.
- Fischer S. Pattern recognition receptors and control of innate immunity: role of nucleic acids. Curr Pharm Biotechnol. 2018;19:1203–1209. doi:10.2174/138920112804583087.
- Patel S. Danger-Associated Molecular Patterns (DAMPs): the derivatives and triggers of inflammation. Curr Allergy Asthma Rep. 2018;18:63. doi:10.1007/s11882-018-0817-3.
- Paroli M, Bellati F, Videtta M, Focaccetti C, Mancone C, Donato T, Antonilli M, Perniola G, Accapezzato D, Napoletano C, et al. Discovery of chemotherapy-associated ovarian cancer antigens by interrogating memory T cells. Int J Cancer. 2014;134:1823–1834. doi:10.1002/ijc.28515.
- Palombo F, Focaccetti C, Barnaba V. Therapeutic implications of immunogenic cell death in human cancer. Front Immunol. 2014;4:503. doi:10.3389/fimmu.2013.00503.
- Gameiro SR, Jammeh ML, Wattenberg MM, Tsang KY, Ferrone S, Hodge JW. Radiation-induced immunogenic modulation of tumor enhances antigen processing and calreticulin exposure, resulting in enhanced T-cell killing. Oncotarget. 2014;5:403–416. doi:10.18632/oncotarget.v5i2.
- Loi S, Pommey S, Haibe-Kains B, Beavis PA, Darcy PK, Smyth MJ, Stagg J. CD73 promotes anthracycline resistance and poor prognosis in triple negative breast cancer. Proc Natl Acad Sci U S A. 2013;110:11091–11096. doi:10.1073/pnas.1222251110.
- Hodge JW, Garnett CT, Farsaci B, Palena C, Tsang KY, Ferrone S, Gameiro SR. Chemotherapy-induced immunogenic modulation of tumor cells enhances killing by cytotoxic T lymphocytes and is distinct from immunogenic cell death. Int J Cancer. 2013;133:624–636. doi:10.1002/ijc.v133.3.
- Dutoit V, Migliorini D, Ranzanici G, Marinari E, Widmer V, Lobrinus JA, Momjian S, Costello J, Walker PR, Okada H, et al. Antigenic expression and spontaneous immune responses support the use of a selected peptide set from the IMA950 glioblastoma vaccine for immunotherapy of grade II and III glioma. Oncoimmunology. 2018;7:e1391972. doi:10.1080/2162402X.2017.1391972.
- Apetoh L, Ghiringhelli F, Tesniere A, Obeid M, Ortiz C, Criollo A, Mignot G, Maiuri MC, Ullrich E, Saulnier P, et al. Toll-like receptor 4-dependent contribution of the immune system to anticancer chemotherapy and radiotherapy. Nat Med. 2007;13:1050–1059. doi:10.1038/nm1622.
- Shindo T, Kitaura K, Ureshino H, Kamachi K, Miyahara M, Doi K, Watanabe T, Sueoka E, Shin-I T, Suzuki R, et al. Deep sequencing of the T cell receptor visualizes reconstitution of T cell immunity in mogamulizumab-treated adult T cell leukemia. Oncoimmunology. 2018;7:e1405204. doi:10.1080/2162402X.2017.1405204.
- De Simone M, Rossetti G, Pagani M. Single cell t cell receptor sequencing: techniques and future challenges. Front Immunol. 2018;9:1638. doi:10.3389/fimmu.2018.01638.
- Jandus C, Usatorre AM, Vigano S, Zhang L, Romero P. The vast universe of T cell diversity: subsets of memory cells and their differentiation. Methods Mol Biol. 2017;1514:1–17.
- Krackhardt AM, Anliker B, Hildebrandt M, Bachmann M, Eichmuller SB, Nettelbeck DM, Renner M, Uharek L, Willimsky G, Schmitt M, et al. Clinical translation and regulatory aspects of CAR/TCR-based adoptive cell therapies-the German cancer consortium approach. Cancer Immunol Immunother CII. 2018;67:513–523. doi:10.1007/s00262-018-2119-y.
- Schrama D, Ritter C, Becker JC. T cell receptor repertoire usage in cancer as a surrogate marker for immune responses. Semin Immunopathol. 2017;39:255–268. doi:10.1007/s00281-016-0614-9.
- Whiteside SK, Snook JP, Williams MA, Weis JJ. Bystander T cells: a balancing act of friends and foes. Trends Immunol. 2018;39:1021–1035. doi:10.1016/j.it.2018.10.003.
- Janikovits J, Muller M, Krzykalla J, Korner S, Echterdiek F, Lahrmann B, Grabe N, Schneider M, Benner A, Doeberitz MVK, et al. High numbers of PDCD1 (PD-1)-positive T cells and B2M mutations in microsatellite-unstable colorectal cancer. Oncoimmunology. 2018;7:e1390640. doi:10.1080/2162402X.2017.1390640.
- Vitale I, Sistigu A, Manic G, Rudqvist NP, Trajanoski Z, Galluzzi L. Mutational and antigenic landscape in tumor progression and cancer immunotherapy. Trends Cell Biol. 2019;29:396–416. doi:10.1016/j.tcb.2019.01.003.
- Postow MA, Manuel M, Wong P, Yuan J, Dong Z, Liu C, Perez S, Tanneau I, Noel M, Courtier A, et al. Peripheral T cell receptor diversity is associated with clinical outcomes following ipilimumab treatment in metastatic melanoma. J Immunother Cancer. 2015;3:23. doi:10.1186/s40425-015-0070-4.
- Zhao Y, Niu C, Cui J. Gamma-delta (gammadelta) T cells: friend or foe in cancer development? J Transl Med. 2018;16:3. doi:10.1186/s12967-017-1378-2.
- Rizvi NA, Hellmann MD, Snyder A, Kvistborg P, Makarov V, Havel JJ, Lee W, Yuan J, Wong P, Ho TS, et al. Cancer immunology. Mutational landscape determines sensitivity to PD-1 blockade in non-small cell lung cancer. Science. 2015;348:124–128. doi:10.1126/science.aaa1348.
- Zingg D, Arenas-Ramirez N, Sahin D, Rosalia RA, Antunes AT, Haeusel J, Sommer L, Boyman O. The histone methyltransferase Ezh2 controls mechanisms of adaptive resistance to tumor immunotherapy. Cell Rep. 2017;20:854–867. doi:10.1016/j.celrep.2017.07.007.
- Turajlic S, Litchfield K, Xu H, Rosenthal R, McGranahan N, Reading JL, Wong YNS, Rowan A, Kanu N, Al Bakir M, et al. Insertion-and-deletion-derived tumour-specific neoantigens and the immunogenic phenotype: a pan-cancer analysis. Lancet Oncol. 2017;18:1009–1021. doi:10.1016/S1470-2045(17)30516-8.
- Parakh S, Gan HK, Parslow AC, Burvenich IJG, Burgess AW, Scott AM. Evolution of anti-HER2 therapies for cancer treatment. Cancer Treat Rev. 2017;59:1–21. doi:10.1016/j.ctrv.2017.06.005.
- O’Rourke DM, Nasrallah MP, Desai A, Melenhorst JJ, Mansfield K, Morrissette JJD, Martinez-Lage M, Brem S, Maloney E, Shen A, et al. A single dose of peripherally infused EGFRvIII-directed CAR T cells mediates antigen loss and induces adaptive resistance in patients with recurrent glioblastoma. Sci Transl Med. 2017;9:eaaa0984. doi:10.1126/scitranslmed.aaa0984.
- Jimenez-Sanchez A, Memon D, Pourpe S, Veeraraghavan H, Li Y, Vargas HA, Gill MB, Park KJ, Zivanovic O, Konner J, et al. Heterogeneous tumor-immune microenvironments among differentially growing metastases in an ovarian cancer patient. Cell. 2017;170:927–38 e20. doi:10.1016/j.cell.2017.07.025.
- Gubin MM, Zhang X, Schuster H, Caron E, Ward JP, Noguchi T, Ivanova Y, Hundal J, Arthur CD, Krebber W-J, et al. Checkpoint blockade cancer immunotherapy targets tumour-specific mutant antigens. Nature. 2014;515:577–581. doi:10.1038/nature13988.
- Kosaloglu-Yalcin Z, Lanka M, Frentzen A, Logandha Ramamoorthy Premlal A, Sidney J, Vaughan K, Greenbaum J, Robbins P, Gartner J, Sette A, et al. Predicting T cell recognition of MHC class I restricted neoepitopes. Oncoimmunology. 2018;7:e1492508. doi:10.1080/2162402X.2018.1492508.
- Azizi AA, Pillai M, Thistlethwaite FC. T-cell receptor and chimeric antigen receptor in solid cancers: current landscape, preclinical data and insight into future developments. Curr Opin Oncol. 2019;31:430–438. doi:10.1097/CCO.0000000000000562.
- Ott PA, Hu Z, Keskin DB, Shukla SA, Sun J, Bozym DJ, Zhang W, Luoma A, Giobbie-Hurder A, Peter L, et al. An immunogenic personal neoantigen vaccine for patients with melanoma. Nature. 2017;547:217–221. doi:10.1038/nature22991.
- Leonard JD, Gilmore DC, Dileepan T, Nawrocka WI, Chao JL, Schoenbach MH, Jenkins MK, Adams EJ, Savage PA. Identification of natural regulatory T cell epitopes reveals convergence on a dominant autoantigen. Immunity. 2017;47:107–17 e8. doi:10.1016/j.immuni.2017.06.015.
- Kumai T, Fan A, Harabuchi Y, Celis E. Cancer immunotherapy: moving forward with peptide T cell vaccines. Curr Opin Immunol. 2017;47:57–63. doi:10.1016/j.coi.2017.07.003.
- Meng YM, Liang J, Wu C, Xu J, Zeng DN, Yu XJ, Ning H, Xu L, Zheng L. Monocytes/Macrophages promote vascular CXCR4 expression via the ERK pathway in hepatocellular carcinoma. Oncoimmunology. 2018;7:e1408745. doi:10.1080/2162402X.2017.1408745.
- Ferretti E, Di Carlo E, Ognio E, Fraternali-Orcioni G, Corcione A, Belmonte B, Ravetti JL, Tripodo C, Ribatti D, Pistoia V, et al. IL-25 dampens the growth of human germinal center-derived B-cell non hodgkin lymphoma by curtailing neoangiogenesis. Oncoimmunology. 2018;7:e1397249. doi:10.1080/2162402X.2017.1397249.
- Bakhru P, Zhu M-L, Wang -H-H, Hong LK, Khan I, Mouchess M, Gulati AS, Starmer J, Hou Y, Sailer D, et al. Combination central tolerance and peripheral checkpoint blockade unleashes antimelanoma immunity. JCI Insight. 2017;2. doi:10.1172/jci.insight.93265
- Iberg CA, Jones A, Hawiger D. Dendritic cells as inducers of peripheral tolerance. Trends Immunol. 2017;38:793–804. doi:10.1016/j.it.2017.07.007.
- Liu M, Li S, Li MO. TGF-beta control of adaptive immune tolerance: a break from treg cells. Bioessays. 2018;40:e1800063. doi:10.1002/bies.201800063.
- McCarville JL, Ayres JS. Disease tolerance: concept and mechanisms. Curr Opin Immunol. 2018;50:88–93. doi:10.1016/j.coi.2017.12.003.
- Nemazee D. Mechanisms of central tolerance for B cells. Nat Rev Immunol. 2017;17:281–294. doi:10.1038/nri.2017.19.
- Nakagawa H, Mizukoshi E, Kobayashi E, Tamai T, Hamana H, Ozawa T, Kishi H, Kitahara M, Yamashita T, Arai K, et al. Association between high-avidity t-cell receptors, induced by alpha-fetoprotein-derived peptides, and anti-tumor effects in patients with hepatocellular carcinoma. Gastroenterology. 2017;152:1395–406.e10. doi:10.1053/j.gastro.2017.02.001.
- Segal G, Prato S, Zehn D, Mintern JD, Villadangos JA. Target density, not affinity or avidity of antigen recognition, determines adoptive T cell therapy outcomes in a mouse lymphoma model. J Immunol. 2016;196:3935–3942. doi:10.4049/jimmunol.1502187.
- Jaigirdar A, Rosenberg SA, Parkhurst M. A high-avidity WT1-reactive T-cell receptor mediates recognition of peptide and processed antigen but not naturally occurring WT1-positive TUMOR CELLS. J Immunol. 2016;39:105–116.
- Zhao Q, Ahmed M, Tassev DV, Hasan A, Kuo T-Y, Guo H-F, O’Reilly RJ, Cheung NKV. Affinity maturation of T-cell receptor-like antibodies for Wilms tumor 1 peptide greatly enhances therapeutic potential. Leukemia. 2015;29:2238–2247. doi:10.1038/leu.2015.125.
- Tassev DV, Hasan A, Kuo TY, Guo HF, O’Reilly RJ, Cheung NK, et al. Quantitative TCR: pMHCDissociation rate assessment by ntamers reveals antimelanoma T cell repertoires enriched for high functional competence. Leukemia. 2015;195:356–366.
- Palmer DC, Guittard GC, Franco Z, Crompton JG, Eil RL, Patel SJ, Ji Y, Van Panhuys N, Klebanoff CA, Sukumar M, et al. Cish actively silences TCR signaling in CD8+ T cells to maintain tumor tolerance. J Exp Med. 2015;212:2095–2113. doi:10.1084/jem.20150304.
- Nakatsugawa M, Yamashita Y, Ochi T, Tanaka S, Chamoto K, Guo T, Butler MO, Hirano N. Specific roles of each TCR hemichain in generating functional chain-centric TCR. J Immunol. 2015;194:3487–3500. doi:10.4049/jimmunol.1401717.
- Hebeisen M, Schmidt J, Guillaume P, Baumgaertner P, Speiser DE, Luescher I, Rufer N. Identification of rare high-avidity, tumor-reactive CD8+ T cells by monomeric TCR-ligand off-rates measurements on living cells. Cancer Res. 2015;75:1983–1991. doi:10.1158/0008-5472.CAN-14-3516.
- Oren R, Hod-Marco M, Haus-Cohen M, Thomas S, Blat D, Duvshani N, Denkberg G, Elbaz Y, Benchetrit F, Eshhar Z, et al. Functional comparison of engineered T cells carrying a native TCR versus TCR-like antibody-based chimeric antigen receptors indicates affinity/avidity thresholds. J Immunol. 2014;193:5733–5743. doi:10.4049/jimmunol.1301769.
- Smith-Garvin JE, Koretzky GA, Jordan MS. T cell activation. Annu Rev Immunol. 2009;27:591–619. doi:10.1146/annurev.immunol.021908.132706.
- Chen DS, Mellman I. Elements of cancer immunity and the cancer-immune set point. Nature. 2017;541:321–330. doi:10.1038/nature21349.
- Patsoukis N, Weaver JD, Strauss L, Herbel C, Seth P, Boussiotis VA. Immunometabolic regulations mediated by coinhibitory receptors and their impact on T Cell immune responses. Eur J Immunol. 2017;8:330.
- Cibrian D, Sanchez-Madrid F. CD69: from activation marker to metabolic gatekeeper. Eur J Immunol. 2017;47:946–953. doi:10.1002/eji.201646837.
- Gerritsen B, Pandit A. The memory of a killer T cell: models of CD8(+) T cell differentiation. Immunol Cell Biol. 2016;94:236–241. doi:10.1038/icb.2015.118.
- Dogra P, Ghoneim HE, Abdelsamed HA, Youngblood B. Generating long-lived CD8(+) T-cell memory: insights from epigenetic programs. Eur J Immunol. 2016;46:1548–1562. doi:10.1002/eji.201545550.
- Almeida L, Lochner M, Berod L, Sparwasser T. Metabolic pathways in T cell activation and lineage differentiation. Semin Immunol. 2016;28:514–524. doi:10.1016/j.smim.2016.10.009.
- Blank CU, Haining WN, Held W, Hogan PG, Kallies A, Lugli E, Lynn RC, Philip M, Rao A, Restifo NP, et al. Defining ‘T cell exhaustion’. Nat Rev Immunol. 2019;19:665–674. doi:10.1038/s41577-019-0221-9.
- Davoodzadeh Gholami M, Kardar GA, Saeedi Y, Heydari S, Garssen J, Falak R. Exhaustion of T lymphocytes in the tumor microenvironment: significance and effective mechanisms. Cell Immunol. 2017;322:1–14. doi:10.1016/j.cellimm.2017.10.002.
- He QF, Xu Y, Li J, Huang ZM, Li XH, Wang X. CD8+ T-cell exhaustion in cancer: mechanisms and new area for cancer immunotherapy. Brief Funct Genomics. 2019;18:99–106. doi:10.1093/bfgp/ely006.
- Kurachi M. CD8(+) T cell exhaustion. Semin Immunopathol. 2019;41:327–337. doi:10.1007/s00281-019-00744-5.
- McKinney EF, Smith KGC. Metabolic exhaustion in infection, cancer and autoimmunity. Nat Immunol. 2018;19:213–221. doi:10.1038/s41590-018-0045-y.
- Philip M, Schietinger A. Heterogeneity and fate choice: T cell exhaustion in cancer and chronic infections. Curr Opin Immunol. 2019;58:98–103. doi:10.1016/j.coi.2019.04.014.
- Saeidi A, Zandi K, Cheok YY, Saeidi H, Wong WF, Lee CYQ, Cheong HC, Yong YK, Larsson M, Shankar EM, et al. T-cell exhaustion in chronic infections: reversing the state of exhaustion and reinvigorating optimal protective immune responses. Front Immunol. 2018;9:2569. doi:10.3389/fimmu.2018.02569.
- Serrano-Del Valle A, Anel A, Naval J, Marzo I. Immunogenic cell death and immunotherapy of multiple myeloma. Front Cell Dev Biol. 2019;7:50. doi:10.3389/fcell.2019.00050.
- Vesely MD, Kershaw MH, Schreiber RD, Smyth MJ. Natural innate and adaptive immunity to cancer. Annu Rev Immunol. 2011;29:235–271. doi:10.1146/annurev-immunol-031210-101324.
- Schreiber RD, Old LJ, Smyth MJ. Cancer immunoediting: integrating immunity’s roles in cancer suppression and promotion. Science. 2011;331:1565–1570. doi:10.1126/science.1203486.
- Senovilla L, Vitale I, Martins I, Tailler M, Pailleret C, Michaud M, Galluzzi L, Adjemian S, Kepp O, Niso-Santano M, et al. An immunosurveillance mechanism controls cancer cell ploidy. Science. 2012;337:1678–1684. doi:10.1126/science.1224922.
- Cruz-Adalia A, Ramirez-Santiago G, Osuna-Perez J, Torres-Torresano M, Zorita V, Martinez-Riano A, Boccasavia V, Borroto A, Martínez Del Hoyo G, González-Granado JM, et al. Conventional CD4+ T cells present bacterial antigens to induce cytotoxic and memory CD8+ T cell responses. Nat Commun. 2017;8:1591. doi:10.1038/s41467-017-01661-7.
- Pasquereau-Kotula E, Habault J, Kroemer G, Poyet JL. The anticancer peptide RT53 induces immunogenic cell death. PLoS One. 2018;13:e0201220. doi:10.1371/journal.pone.0201220.
- Lu J, Liu X, Liao Y-P, Wang X, Ahmed A, Jiang W, Ji Y, Meng H, Nel AE. Breast cancer chemo-immunotherapy through liposomal delivery of an immunogenic cell death stimulus plus interference in the IDO-1 pathway. ACS Nano. 2018;12:11041–11061. doi:10.1021/acsnano.8b05189.
- Tappe KA, Budida R, Stankov MV, Frenz T, RS H, Volz A, Sutter G, Kalinke U, Behrens GMN. Immunogenic cell death of dendritic cells following modified vaccinia virus Ankara infection enhances CD8(+) T cell proliferation. Eur J Immunol. 2018;48:2042–2054. doi:10.1002/eji.v48.12.
- Ogawa M, Tomita Y, Nakamura Y, Lee MJ, Lee S, Tomita S, Nagaya T, Sato K, Yamauchi T, Iwai H, et al. Immunogenic cancer cell death selectively induced by near infrared photoimmunotherapy initiates host tumor immunity. Oncotarget. 2017;8:10425–10436. doi:10.18632/oncotarget.v8i6.
- Garg AD, Vandenberk L, Koks C, Verschuere T, Boon L, Van Gool SW, Agostinis P. Dendritic cell vaccines based on immunogenic cell death elicit danger signals and T cell-driven rejection of high-grade glioma. Sci Transl Med. 2016;8:328ra27. doi:10.1126/scitranslmed.aae0105.
- Chowdhury PS, Chamoto K, Honjo T. Combination therapy strategies for improving PD-1 blockade efficacy: a new era in cancer immunotherapy. J Intern Med. 2018;283:110–120. doi:10.1111/joim.2018.283.issue-2.
- Gupta R, Amanam I, Chung V. Current and future therapies for advanced pancreatic cancer. J Surg Oncol. 2017;116:25–34. doi:10.1002/jso.v116.1.
- Shimizu Y, Suzuki T, Yoshikawa T, Tsuchiya N, Sawada Y, Endo I, Nakatsura T. Cancer immunotherapy-targeted glypican-3 or neoantigens. Cancer Sci. 2018;109:531–541. doi:10.1111/cas.2018.109.issue-3.
- Galluzzi L, Zitvogel L, Kroemer G. Immunological mechanisms underneath the efficacy of cancer therapy. Cancer Immunol Res. 2016;4:895–902. doi:10.1158/2326-6066.CIR-16-0197.
- Somasundaram A, Burns TF. The next generation of immunotherapy: keeping lung cancer in check. J Hematol Oncol. 2017;10:87. doi:10.1186/s13045-017-0456-5.
- Gulley JL, Madan RA, Pachynski R, Mulders P, Sheikh NA, Trager J, Drake CG. Role of antigen spread and distinctive characteristics of immunotherapy in cancer treatment. J Natl Cancer Inst. 2017;109.
- McCall NS, Dicker AP, Lu B. Beyond concurrent chemoradiation: the emerging role of PD-1/PD-L1 inhibitors in stage III lung cancer. Clin Cancer Res off J Am Assoc Cancer Res. 2018;24:1271–1276. doi:10.1158/1078-0432.CCR-17-3269.
- Galluzzi L, Vacchelli E, Bravo-San Pedro JM, Buque A, Senovilla L, Baracco EE, Bloy N, Castoldi F, Abastado J-P, Agostinis P, et al. Classification of current anticancer immunotherapies. Oncotarget. 2014;5:12472–12508. doi:10.18632/oncotarget.v5i24.
- van den Bulk J, Verdegaal EM, de Miranda NF. Cancer immunotherapy: broadening the scope of targetable tumours. Open Biol. 2018;8:180037. doi:10.1098/rsob.180037.
- Casey SC, Baylot V, Felsher DW. MYC: master regulator of immune privilege. Trends Immunol. 2017;38:298–305. doi:10.1016/j.it.2017.01.002.
- Galluzzi L, Bravo-San Pedro JM, Kepp O, Kroemer G. Regulated cell death and adaptive stress responses. Cell Mol Life Sci. 2016;73:2405–2410. doi:10.1007/s00018-016-2209-y.
- Wu J, Waxman DJ. Immunogenic chemotherapy: dose and schedule dependence and combination with immunotherapy. Cancer Lett. 2018;419:210–221. doi:10.1016/j.canlet.2018.01.050.
- Buqué A, Rodriguez-Ruiz ME, Fucikova J, Galluzzi L. Apoptotic caspases cut down the immunogenicity of radiation. OncoImmunology. 2019;8:e1655364. doi:10.1080/2162402X.2019.1655364.
- Formenti SC, Rudqvist N-P, Golden E, Cooper B, Wennerberg E, Lhuillier C, Vanpouille-Box C, Friedman K, Ferrari de Andrade L, Wucherpfennig KW, et al. Radiotherapy induces responses of lung cancer to CTLA-4 blockade. Nat Med. 2018;24:1845–1851. doi:10.1038/s41591-018-0232-2.
- Ko EC, Galluzzi L. Radiation unlocks the therapeutic potential of immune checkpoint blockers in lung cancer patients. Oncoimmunology. 2019;8:1606624. doi:10.1080/2162402X.2019.1606624.
- Ni L, Dong C. New checkpoints in cancer immunotherapy. Immunol Rev. 2017;276:52–65. doi:10.1111/imr.2017.276.issue-1.
- Montico B, Nigro A, Casolaro V, Dal Col J. Immunogenic apoptosis as a novel tool for anticancer vaccine development. Int J Mol Sci. 2018;19:594. doi:10.3390/ijms19020594.
- Rapoport BL, Anderson R. Realizing the clinical potential of immunogenic cell death in cancer chemotherapy and radiotherapy. Int J Mol Sci. 2019;20:959. doi:10.3390/ijms20040959.
- Tanchot C, Terme M, Pere H, Tran T, Benhamouda N, Strioga M, Banissi C, Galluzzi L, Kroemer G, Tartour E, et al. Tumor-infiltrating regulatory T cells: phenotype, role, mechanism of expansion in situ and clinical significance. Cancer Microenviron. 2013;6:147–157. doi:10.1007/s12307-012-0122-y.
- Curran CS, Sharon E. PD-1 immunobiology in autoimmune hepatitis and hepatocellular carcinoma. Semin Oncol. 2017;44:428–432. doi:10.1053/j.seminoncol.2017.12.001.
- Donia M, Pedersen M, Svane IM. Cancer immunotherapy in patients with preexisting autoimmune disorders. Semin Immunopathol. 2017;39:333–337. doi:10.1007/s00281-016-0595-8.
- Vitale I, Manic G, Coussens LM, Kroemer G, Galluzzi L. Macrophages and metabolism in the tumor microenvironment. Cell Metab. 2019;30:36–50. doi:10.1016/j.cmet.2019.06.001.
- Johnson DB, Sullivan RJ, Menzies AM. Immune checkpoint inhibitors in challenging populations. Cancer. 2017;123:1904–1911. doi:10.1002/cncr.v123.11.
- Paluch C, Santos AM, Anzilotti C, Cornall RJ, Davis SJ. Immune checkpoints as therapeutic targets in autoimmunity. Front Immunol. 2018;9:2306. doi:10.3389/fimmu.2018.02306.
- Swoboda A, Nanda R. Immune checkpoint blockade for breast cancer. Cancer Treat Res. 2018;173:155–165.
- Wykes MN, Lewin SR. Immune checkpoint blockade in infectious diseases. Nat Rev Immunol. 2018;18:91–104. doi:10.1038/nri.2017.112.
- Yoon KW. Dead cell phagocytosis and innate immune checkpoint. BMB Rep. 2017;50:496–503. doi:10.5483/BMBRep.2017.50.10.147.
- Vahl JM, Friedrich J, Mittler S, Trump S, Heim L, Kachler K, Balabko L, Fuhrich N, Geppert C-I, Trufa DI, et al. Interleukin-10-regulated tumour tolerance in non-small cell lung cancer. Br J Cancer. 2017;117:1644–1655. doi:10.1038/bjc.2017.336.
- Fan Y, Kuai R, Xu Y, Ochyl LJ, Irvine DJ, Moon JJ. Immunogenic cell death amplified by co-localized adjuvant delivery for cancer immunotherapy. Nano Lett. 2017;17:7387–7393. doi:10.1021/acs.nanolett.7b03218.
- Garg AD, De Ruysscher D, Agostinis P. Immunological metagene signatures derived from immunogenic cancer cell death associate with improved survival of patients with lung, breast or ovarian malignancies: A large-scale meta-analysis. Oncoimmunology. 2016;5:e1069938. doi:10.1080/2162402X.2015.1069938.
- Musetti S, Huang L. Nanoparticle-mediated remodeling of the tumor microenvironment to enhance immunotherapy. ACS Nano. 2018;12:11740–11755. doi:10.1021/acsnano.8b05893.
- Wittwer C, Boeck S, Heinemann V, Haas M, Stieber P, Nagel D, Holdenrieder S. Circulating nucleosomes and immunogenic cell death markers HMGB1, sRAGE and DNAse in patients with advanced pancreatic cancer undergoing chemotherapy. Int J Cancer. 2013;133:2619–2630. doi:10.1002/ijc.28294.
- Siddiqui I, Erreni M, Kamal MA, Porta C, Marchesi F, Pesce S, Pasqualini F, Schiarea S, Chiabrando C, Mantovani A, et al. Differential role of interleukin-1 and interleukin-6 in K-Ras-driven pancreatic carcinoma undergoing mesenchymal transition. Oncoimmunology. 2018;7:e1388485. doi:10.1080/2162402X.2017.1388485.
- Exner R, Sachet M, Arnold T, Zinn-Zinnenburg M, Michlmayr A, Dubsky P, Bartsch R, Steger G, Gnant M, Bergmann M, et al. Prognostic value of HMGB1 in early breast cancer patients under neoadjuvant chemotherapy. Cancer Med. 2016;5:2350–2358. doi:10.1002/cam4.2016.5.issue-9.
- Garg AD, Coulie PG, Van den Eynde BJ, Agostinis P. Integrating next-generation dendritic cell vaccines into the current cancer immunotherapy landscape. Trends Immunol. 2017;38:577–593. doi:10.1016/j.it.2017.05.006.
- Vacchelli E, Ma Y, Baracco EE, Sistigu A, Enot DP, Pietrocola F, Yang H, Adjemian S, Chaba K, Semeraro M, et al. Chemotherapy-induced antitumor immunity requires formyl peptide receptor 1. Science. 2015;350:972–978. doi:10.1126/science.aad0779.
- Fotaki G, Jin C, Ramachandran M, Kerzeli IK, Karlsson-Parra A, Yu D, Essand M. Pro-inflammatory allogeneic DCs promote activation of bystander immune cells and thereby license antigen-specific T-cell responses. Oncoimmunology. 2018;7:e1395126. doi:10.1080/2162402X.2017.1395126.
- Lukacsi S, Nagy-Balo Z, Erdei A, Sandor N, Bajtay Z. The role of CR3 (CD11b/CD18) and CR4 (CD11c/CD18) in complement-mediated phagocytosis and podosome formation by human phagocytes. Immunol Lett. 2017;189:64–72. doi:10.1016/j.imlet.2017.05.014.
- Ohlsson SM, Pettersson Å, Ohlsson S, Selga D, Bengtsson AA, Segelmark M, Hellmark T. Phagocytosis of apoptotic cells by macrophages in anti-neutrophil cytoplasmic antibody-associated systemic vasculitis. Clin Exp Immunol. 2012;170:47–56. doi:10.1111/cei.2012.170.issue-1.
- Ligeon LA, Romao S, Munz C. Analysis of LC3-associated phagocytosis and antigen presentation. Methods Mol Biol. 2017;1519:145–168.
- Choi S-C, Simhadri VR, Tian L, Gil-Krzewska A, Krzewski K, Borrego F, Coligan JE. Cutting edge: mouse CD300f (CMRF-35-like molecule-1) recognizes outer membrane-exposed phosphatidylserine and can promote phagocytosis. J Immunol. 2011;187:3483–3487. doi:10.4049/jimmunol.1101549.
- Ishimoto H, Yanagihara K, Araki N, Mukae H, Sakamoto N, Izumikawa K, Seki M, Miyazaki Y, Hirakata Y, Mizuta Y, et al. Single-cell observation of phagocytosis by human blood dendritic cells. Jpn J Infect Dis. 2008;61:294–297.
- van Bommel PE, He Y, Schepel I, Hendriks M, Wiersma VR, van Ginkel RJ, van Meerten T, Ammatuna E, Huls G, Samplonius DF, et al. CD20-selective inhibition of CD47-SIRPalpha “don’t eat me” signaling with a bispecific antibody-derivative enhances the anticancer activity of daratumumab, alemtuzumab and obinutuzumab. Oncoimmunology. 2018;7:e1386361. doi:10.1080/2162402X.2017.1386361.
- Li F, Lv B, Liu Y, Hua T, Han J, Sun C, Xu L, Zhang Z, Feng Z, Cai Y, et al. Blocking the CD47-SIRPα axis by delivery of anti-CD47 antibody induces antitumor effects in glioma and glioma stem cells. Oncoimmunology. 2018;7:e1391973. doi:10.1080/2162402X.2017.1391973.
- Garg AD, Romano E, Rufo N, Agostinis P. Immunogenic versus tolerogenic phagocytosis during anticancer therapy: mechanisms and clinical translation. Cell Death Differ. 2016;23:938–951. doi:10.1038/cdd.2016.5.
- Morioka S, Perry JSA, Raymond MH, Medina CB, Zhu Y, Zhao L, Serbulea V, Onengut-Gumuscu S, Leitinger N, Kucenas S, et al. Efferocytosis induces a novel SLC program to promote glucose uptake and lactate release. Nature. 2018;563:714–718. doi:10.1038/s41586-018-0735-5.
- Chaoul N, Tang A, Desrues B, Oberkampf M, Fayolle C, Ladant D, Sainz-Perez A, Leclerc C. Lack of MHC class II molecules favors CD8(+) T-cell infiltration into tumors associated with an increased control of tumor growth. Oncoimmunology. 2018;7:e1404213. doi:10.1080/2162402X.2017.1404213.
- McDonnell AM, Cook A, Robinson BWS, Lake RA, Nowak AK. Serial immunomonitoring of cancer patients receiving combined antagonistic anti-CD40 and chemotherapy reveals consistent and cyclical modulation of T cell and dendritic cell parameters. BMC Cancer. 2017;17:417. doi:10.1186/s12885-017-3403-5.
- Chen L, Hasni MS, Jondal M, Yakimchuk K. Modification of anti-tumor immunity by tolerogenic dendritic cells. Autoimmunity. 2017;50:370–376. doi:10.1080/08916934.2017.1344837.
- Castiello L, Sabatino M, Ren J, Terabe M, Khuu H, Wood LV, Berzofsky JA, Stroncek DF Expression of CD14, IL10, and tolerogenic signature in dendritic cells inversely correlate with clinical and immunologic response to TARP vaccination in prostate cancer patients. Autoimmunity. 2017;23:3352–3364.
- Van den Bergh J, Willemen Y, Lion E, Van Acker H, De Reu H, Anguille S, Goossens H, Berneman Z, Van Tendeloo V, Smits E, et al. Transpresentation of interleukin-15 by IL-15/IL-15Rα mRNA-engineered human dendritic cells boosts antitumoral natural killer cell activity. Oncotarget. 2015;6:44123–44133. doi:10.18632/oncotarget.6536.
- Nagaoka K, Hosoi A, Iino T, Morishita Y, Matsushita H, Kakimi K. Dendritic cell vaccine induces antigen-specific CD8(+) T cells that are metabolically distinct from those of peptide vaccine and is well-combined with PD-1 checkpoint blockade. Oncoimmunology. 2018;7:e1395124. doi:10.1080/2162402X.2017.1395124.
- Duggan MC, Campbell AR, McMichael EL, Opheim KS, Levine KM, Bhave N, Culbertson MC, Noel T, Yu L, Carson WE, et al. Co-stimulation of the fc receptor and interleukin-12 receptor on human natural killer cells leads to increased expression of cd25. Oncoimmunology. 2018;7:e1381813. doi:10.1080/2162402X.2017.1381813.
- Briseno CG, Haldar M, Kretzer NM, Wu X, Theisen DJ, Kc W, Durai V, Grajales-Reyes G, Iwata A, Bagadia P, et al. Distinct transcriptional programs control cross-priming in classical and monocyte-derived dendritic cells. Cell Rep. 2016;15:2462–2474. doi:10.1016/j.celrep.2016.05.025.
- Zhang Y, Chen G, Liu Z, Tian S, Zhang J, Carey CD, Murphy KM, Storkus WJ, Falo LD, You Z, et al. Genetic vaccines to potentiate the effective CD103+ dendritic cell-mediated cross-priming of antitumor immunity. J Immunol. 2015;194:5937–5947. doi:10.4049/jimmunol.1500089.
- Osmond TL, Farrand KJ, Painter GF, Ruedl C, Petersen TR, Hermans IF. Activated NKT cells can condition different splenic dendritic cell subsets to respond more effectively to TLR engagement and enhance cross-priming. J Immunol. 2015;195:821–831. doi:10.4049/jimmunol.1401751.
- Leavy O. Cell death: pathways for cross-priming. Nat Rev Immunol. 2015;15:725. doi:10.1038/nri3933.
- Katakam AK, Brightbill H, Franci C, Kung C, Nunez V, Jones C 3rd, Peng I, Jeet S, Wu LC, Mellman I, et al. Dendritic cells require NIK for CD40-dependent cross-priming of CD8+ T cells. Proc Natl Acad Sci U S A. 2015;112:14664–14669. doi:10.1073/pnas.1520627112.
- Stephenson RM, Lim CM, Matthews M, Dietsch G, Hershberg R, Ferris RL. TLR8 stimulation enhances cetuximab-mediated natural killer cell lysis of head and neck cancer cells and dendritic cell cross-priming of EGFR-specific CD8+ T cells. Cancer Immunol Immunother CII. 2013;62:1347–1357. doi:10.1007/s00262-013-1437-3.
- Gamrekelashvili J, Kapanadze T, Han M, Wissing J, Ma C, Jaensch L, Manns MP, Armstrong T, Jaffee E, White AO, et al. Peptidases released by necrotic cells control CD8+ T cell cross-priming. J Clin Invest. 2013;123:4755–4768. doi:10.1172/JCI65698.
- Watson AM, Mylin LM, Thompson MM, Schell TD. Modification of a tumor antigen determinant to improve peptide/MHC stability is associated with increased immunogenicity and cross-priming a larger fraction of CD8+ T cells. J Immunol. 2012;189:5549–5560. doi:10.4049/jimmunol.1102221.
- Valentine FT, Golomb FM, Harris M, Roses DF. A novel immunization strategy using cytokine/chemokines induces new effective systemic immune responses, and frequent complete regressions of human metastatic melanoma. Oncoimmunology. 2018;7:e1386827. doi:10.1080/2162402X.2017.1386827.
- Anwer F, Shaukat -A-A, Zahid U, Husnain M, McBride A, Persky D, Lim M, Hasan N, Riaz IB. Donor origin CAR T cells: graft versus malignancy effect without GVHD, a systematic review. Immunotherapy. 2017;9:123–130. doi:10.2217/imt-2016-0127.
- Hennequart M, Pilotte L, Cane S, Hoffmann D, Stroobant V, Plaen E, Eynde BJVD. Constitutive IDO1 expression in human tumors is driven by cyclooxygenase-2 and mediates intrinsic immune resistance. Cancer Immunol Res. 2017;5:695–709. doi:10.1158/2326-6066.CIR-16-0400.
- Hocine HR, Costa HE, Dam N, Giustiniani J, Palacios I, Loiseau P, Bensussan A, Borlado LR, Charron D, Suberbielle C, et al. Minimizing the risk of allo-sensitization to optimize the benefit of allogeneic cardiac-derived stem/progenitor cells. Sci Rep. 2017;7:41125. doi:10.1038/srep41125.
- Inman CF, Eldershaw SA, Croudace JE, Davies NJ, Sharma-Oates A, Rai T, Pearce H, Sirovica M, Chan YLT, Verma K, et al. Unique features and clinical importance of acute alloreactive immune responses. JCI Insight. 2018;3. doi:10.1172/jci.insight.97219
- Ramadan A, Griesenauer B, Adom D, Kapur R, Hanenberg H, Liu C, Kaplan MH, Paczesny S. Specifically differentiated T cell subset promotes tumor immunity over fatal immunity. J Exp Med. 2017;214:3577–3596. doi:10.1084/jem.20170041.
- Riquelme P, Haarer J, Kammler A, Walter L, Tomiuk S, Ahrens N, Wege AK, Goecze I, Zecher D, Banas B, et al. TIGIT+ iTregs elicited by human regulatory macrophages control T cell immunity. Nat Commun. 2018;9:2858. doi:10.1038/s41467-018-05167-8.
- Schijns V, Pretto C, Strik AM, Gloudemans-Rijkers R, Deviller L, Pierre D, Chung J, Dandekar M, Carrillo JA, Kong XT, et al. Therapeutic immunization against glioblastoma. Int J Mol Sci. 2018;19.
- Stern L, McGuire H, Avdic S, Rizzetto S, Fazekas de St Groth B, Luciani F, Slobedman B, Blyth E. Mass cytometry for the assessment of immune reconstitution after hematopoietic stem cell transplantation. Front Immunol. 2018;9:1672. doi:10.3389/fimmu.2018.01672.
- Tasian SK, Kenderian SS, Shen F, Ruella M, Shestova O, Kozlowski M, Li Y, Schrank-Hacker A, Morrissette JJD, Carroll M, et al. Optimized depletion of chimeric antigen receptor T cells in murine xenograft models of human acute myeloid leukemia. Blood. 2017;129:2395–2407. doi:10.1182/blood-2016-08-736041.
- Du W, Mohammadpour H, O’Neill RE, Kumar S, Chen C, Qiu M, Mei L, Qiu J, McCarthy PL, Lee KP, et al. Serine protease inhibitor 6 protects alloreactive T cells from Granzyme B-mediated mitochondrial damage without affecting graft-versus-tumor effect. Oncoimmunology. 2018;7:e1397247. doi:10.1080/2162402X.2017.1397247.
- Alimbetov D, Askarova S, Umbayev B, Davis T, Kipling D. Pharmacological targeting of cell cycle, apoptotic and cell adhesion signaling pathways implicated in chemoresistance of cancer cells. Int J Mol Sci. 2018;19.
- Filliol A, Piquet-Pellorce C, Raguenes-Nicol C, Dion S, Farooq M, Lucas-Clerc C, Vandenabeele P, Bertrand MJM, Le Seyec J, Samson M, et al. RIPK1 protects hepatocytes from Kupffer cells-mediated TNF-induced apoptosis in mouse models of PAMP-induced hepatitis. J Hepatol. 2017;66:1205–1213. doi:10.1016/j.jhep.2017.01.005.
- Huang AC, Postow MA, Orlowski RJ, Mick R, Bengsch B, Manne S, Xu W, Harmon S, Giles JR, Wenz B, et al. T-cell invigoration to tumour burden ratio associated with anti-PD-1 response. Nature. 2017;545:60–65. doi:10.1038/nature22079.
- Liu D, Jenkins RW, Sullivan RJ. Mechanisms of resistance to immune checkpoint blockade. Am J Clin Dermatol. 2019;20:41–54. doi:10.1007/s40257-018-0389-y.
- Pawelec G. Immune signatures predicting responses to immunomodulatory antibody therapy. Curr Opin Immunol. 2018;51:91–96. doi:10.1016/j.coi.2018.03.003.
- Stanczak MA, Siddiqui SS, Trefny MP, Thommen DS, Boligan KF, von Gunten S, Tzankov A, Tietze L, Lardinois D, Heinzelmann-Schwarz V, et al. Self-associated molecular patterns mediate cancer immune evasion by engaging siglecs on T cells. J Clin Invest. 2018;128:4912–4923. doi:10.1172/JCI120612.
- Wang B, Zhang W, Jankovic V, Golubov J, Poon P, Oswald EM, Gurer C, Wei J, Ramos I, Wu Q, et al. Combination cancer immunotherapy targeting PD-1 and GITR can rescue CD8(+) T cell dysfunction and maintain memory phenotype. Sci Immunol. 2018;3:eaat7061. doi:10.1126/sciimmunol.aat7061.
- Foerster F, Boegel S, Heck R, Pickert G, Russel N, Rosigkeit S, Bros M, Strobl S, Kaps L, Aslam M, et al. Enhanced protection of C57 BL/6 vs Balb/c mice to melanoma liver metastasis is mediated by NK cells. Oncoimmunology. 2018;7:e1409929. doi:10.1080/2162402X.2017.1409929.
- Nam G-H, Lee EJ, Kim YK, Hong Y, Choi Y, Ryu M-J, Woo J, Cho Y, Ahn DJ, Yang Y, et al. Combined Rho-kinase inhibition and immunogenic cell death triggers and propagates immunity against cancer. Nat Commun. 2018;9:2165. doi:10.1038/s41467-018-04607-9.
- Kuryk L, Moller AW, Jaderberg M. Combination of immunogenic oncolytic adenovirus ONCOS-102 with anti-PD-1 pembrolizumab exhibits synergistic antitumor effect in humanized A2058 melanoma huNOG mouse model. Oncoimmunology. 2019;8:e1532763. doi:10.1080/2162402X.2018.1532763.
- Berzofsky JA, Terabe M, Trepel JB, Pastan I, Stroncek DF, Morris JC, Wood LV. Cancer vaccine strategies: translation from mice to human clinical trials. Cancer Immunol Immunother CII. 2018;67:1863–1869. doi:10.1007/s00262-017-2084-x.
- Menger L, Vacchelli E, Adjemian S, Martins I, Ma Y, Shen S, Yamazaki T, Sukkurwala AQ, Michaud M, Mignot G, et al. Cardiac glycosides exert anticancer effects by inducing immunogenic cell death. Sci Transl Med. 2012;4:143ra99. doi:10.1126/scitranslmed.3003807.
- Hernandez C, Huebener P, Schwabe RF. Damage-associated molecular patterns in cancer: a double-edged sword. Oncogene. 2016;35:5931–5941. doi:10.1038/onc.2016.104.
- Gardai SJ, McPhillips KA, Frasch SC, Janssen WJ, Starefeldt A, Murphy-Ullrich JE, Bratton DL, Oldenborg P-A, Michalak M, Henson PM, et al. Cell-surface calreticulin initiates clearance of viable or apoptotic cells through trans-activation of LRP on the phagocyte. Cell. 2005;123:321–334. doi:10.1016/j.cell.2005.08.032.
- Woo SR, Corrales L, Gajewski TF. Innate immune recognition of cancer. Annu Rev Immunol. 2015;33:445–474. doi:10.1146/annurev-immunol-032414-112043.
- Sancho D, Reis E Sousa C. Sensing of cell death by myeloid C-type lectin receptors. Curr Opin Immunol. 2013;25:46–52. doi:10.1016/j.coi.2012.12.007.
- Ahn J, Xia T, Rabasa Capote A, Betancourt D, Barber GN. Extrinsic Phagocyte-Dependent STING Signaling Dictates the Immunogenicity of Dying Cells. Cancer Cell. 2018;33:862–73.e5. doi:10.1016/j.ccell.2018.03.027.
- Bianchi ME, Crippa MP, Manfredi AA, Mezzapelle R, Rovere Querini P, Venereau E. High-mobility group box 1 protein orchestrates responses to tissue damage via inflammation, innate and adaptive immunity, and tissue repair. Immunol Rev. 2017;280:74–82. doi:10.1111/imr.2017.280.issue-1.
- Nuka E, Ohnishi K, Terao J, Kawai Y. ATP/P2X7 receptor signaling as a potential anti-inflammatory target of natural polyphenols. PLoS One. 2018;13:e0204229. doi:10.1371/journal.pone.0204229.
- Parkes EE, Walker SM, Taggart LE, McCabe N, Knight LA, Wilkinson R, McCloskey KD, Buckley NE, Savage KI, Salto-Tellez M, et al. Activation of STING-dependent innate immune signaling by S-phase-specific DNA damage in breast cancer. J Natl Cancer Inst. 2017;109.
- Suek N, Campesato LF, Merghoub T, Khalil DN. Targeted APC activation in cancer immunotherapy to enhance the abscopal effect. Front Immunol. 2019;10:604. doi:10.3389/fimmu.2019.00604.
- Yatim N, Cullen S, Albert ML. Dying cells actively regulate adaptive immune responses. Nat Rev Immunol. 2017;17:262–275. doi:10.1038/nri.2017.9.
- Obeid M, Tesniere A, Ghiringhelli F, Fimia GM, Apetoh L, Perfettini J-L, Castedo M, Mignot G, Panaretakis T, Casares N, et al. Calreticulin exposure dictates the immunogenicity of cancer cell death. Nat Med. 2007;13:54–61. doi:10.1038/nm1523.
- Garg AD, Elsen S, Krysko DV, Vandenabeele P, de Witte P, Agostinis P. Resistance to anticancer vaccination effect is controlled by a cancer cell-autonomous phenotype that disrupts immunogenic phagocytic removal. Oncotarget. 2015;6:26841–26860. doi:10.18632/oncotarget.v6i29.
- Truxova I, Kasikova L, Salek C, Hensler M, Lysak D, Holicek P, Bilkova P, Holubova M, Chen X, Mikyskova R, et al. Calreticulin exposure on malignant blasts correlates with improved natural killer cell-mediated cytotoxicity in acute myeloid leukemia patients. Haematologica. 2019. doi:10.3324/haematol.2019.223933.
- Elliott MR, Chekeni FB, Trampont PC, Lazarowski ER, Kadl A, Walk SF, Park D, Woodson RI, Ostankovich M, Sharma P, et al. Nucleotides released by apoptotic cells act as a find-me signal to promote phagocytic clearance. Nature. 2009;461:282–286. doi:10.1038/nature08296.
- Chekeni FB, Elliott MR, Sandilos JK, Walk SF, Kinchen JM, Lazarowski ER, Armstrong AJ, Penuela S, Laird DW, Salvesen GS, et al. Pannexin 1 channels mediate ‘find-me’ signal release and membrane permeability during apoptosis. Nature. 2010;467:863–867. doi:10.1038/nature09413.
- Martins I, Wang Y, Michaud M, Ma Y, Sukkurwala AQ, Shen S, Kepp O, Métivier D, Galluzzi L, Perfettini J-L, et al. Molecular mechanisms of ATP secretion during immunogenic cell death. Cell Death Differ. 2014;21:79–91. doi:10.1038/cdd.2013.75.
- Galluzzi L, Bravo-San Pedro JM, Demaria S, Formenti SC, Kroemer G. Activating autophagy to potentiate immunogenic chemotherapy and radiation therapy. Nat Rev Clin Oncol. 2017;14:247–258. doi:10.1038/nrclinonc.2016.183.
- Garg AD, Krysko DV, Vandenabeele P, Agostinis P. Extracellular ATP and P(2)X(7) receptor exert context-specific immunogenic effects after immunogenic cancer cell death. Cell Death Dis. 2016;7:e2097. doi:10.1038/cddis.2015.411.
- Scaffidi P, Misteli T, Bianchi ME. Release of chromatin protein HMGB1 by necrotic cells triggers inflammation. Nature. 2002;418:191–195. doi:10.1038/nature00858.
- Liu P, Zhao L, Loos F, Iribarren K, Kepp O, Kroemer G. Epigenetic anticancer agents cause HMGB1 release in vivo. Oncoimmunology. 2018;7:e1431090. doi:10.1080/2162402X.2018.1431090.
- Sistigu A, Yamazaki T, Vacchelli E, Chaba K, Enot DP, Adam J, Vitale I, Goubar A, Baracco EE, Remédios C, et al. Cancer cell-autonomous contribution of type I interferon signaling to the efficacy of chemotherapy. Nat Med. 2014;20:1301–1309. doi:10.1038/nm.3708.
- Vanpouille-Box C, Alard A, Aryankalayil MJ, Sarfraz Y, Diamond JM, Schneider RJ, Inghirami G, Coleman CN, Formenti SC, Demaria S, et al. DNA exonuclease Trex1 regulates radiotherapy-induced tumour immunogenicity. Nat Commun. 2017;8:15618. doi:10.1038/ncomms15618.
- Mackenzie KJ, Carroll P, Martin CA, Murina O, Fluteau A, Simpson DJ, Olova N, Sutcliffe H, Rainger JK, Leitch A, et al. cGAS surveillance of micronuclei links genome instability to innate immunity. Nature. 2017;548:461–465. doi:10.1038/nature23449.
- Harding SM, Benci JL, Irianto J, Discher DE, Minn AJ, Greenberg RA. Mitotic progression following DNA damage enables pattern recognition within micronuclei. Nature. 2017;548:466–470. doi:10.1038/nature23470.
- Sprooten J, Agostinis P, Garg AD. Type I interferons and dendritic cells in cancer immunotherapy. Int Rev Cell Mol Biol Acad Press. 2019. doi:10.1016/bs.ircmb.2019.06.001.
- Cauwels A, Van Lint S, Garcin G, Bultinck J, Paul F, Gerlo S, Van der Heyden J, Bordat Y, Catteeuw D, De Cauwer L, et al. A safe and highly efficient tumor-targeted type I interferon immunotherapy depends on the tumor microenvironment. Oncoimmunology. 2018;7:e1398876. doi:10.1080/2162402X.2017.1398876.
- Krombach J, Hennel R, Brix N, Orth M, Schoetz U, Ernst A, Schuster J, Zuchtriegel G, Reichel CA, Bierschenk S, et al. Priming anti-tumor immunity by radiotherapy: dying tumor cell-derived DAMPs trigger endothelial cell activation and recruitment of myeloid cells. Oncoimmunology. 2019;8:e1523097. doi:10.1080/2162402X.2018.1523097.
- Garg AD, Vandenberk L, Fang S, Fasche T, Van Eygen S, Maes J, Van Woensel M, Koks C, Vanthillo N, Graf N, et al. Pathogen response-like recruitment and activation of neutrophils by sterile immunogenic dying cells drives neutrophil-mediated residual cell killing. Cell Death Differ. 2017;24:832–843. doi:10.1038/cdd.2017.15.
- De Waele J, Marcq E, Van Audenaerde JR, Van Loenhout J, Deben C, Zwaenepoel K, Van de Kelft E, Van der Planken D, Menovsky T, Van den Bergh JM, et al. Poly(I:C) primes primary human glioblastoma cells for an immune response invigorated by PD-L1 blockade. Oncoimmunology. 2018;7:e1407899. doi:10.1080/2162402X.2017.1407899.
- Vanpouille-Box C, Hoffmann JA, Galluzzi L. Pharmacological modulation of nucleic acid sensors - therapeutic potential and persisting obstacles. Nat Rev Drug Discov. 2019. doi:10.1038/s41573-019-0043-2.
- Galluzzi L, Buque A, Kepp O, Zitvogel L, Kroemer G. Immunological Effects of Conventional Chemotherapy and Targeted Anticancer Agents. Cancer Cell. 2015;28:690–714. doi:10.1016/j.ccell.2015.10.012.
- Delaunay T, Violland M, Boisgerault N, Dutoit S, Vignard V, Munz C, Gannage M, Dréno B, Vaivode K, Pjanova D, et al. Oncolytic viruses sensitize human tumor cells for NY-ESO-1 tumor antigen recognition by CD4+ effector T cells. Oncoimmunology. 2018;7:e1407897. doi:10.1080/2162402X.2017.1407897.
- Garg AD, Krysko DV, Verfaillie T, Kaczmarek A, Ferreira GB, Marysael T, Rubio N, Firczuk M, Mathieu C, Roebroek AJM, et al. A novel pathway combining calreticulin exposure and ATP secretion in immunogenic cancer cell death. Embo J. 2012;31:1062–1079. doi:10.1038/emboj.2011.497.
- Garg AD, Dudek AM, Ferreira GB, Verfaillie T, Vandenabeele P, Krysko DV, Mathieu C, Agostinis P. ROS-induced autophagy in cancer cells assists in evasion from determinants of immunogenic cell death. Autophagy. 2013;9:1292–1307. doi:10.4161/auto.25399.
- Pol J, Bloy N, Obrist F, Eggermont A, Galon J, Cremer I, Erbs P, Limacher J-M, Preville X, Zitvogel L, et al. Trial Watch:: oncolytic viruses for cancer therapy. Oncoimmunology. 2014;3:e28694. doi:10.4161/onci.28694.
- Willemen Y, Van den Bergh JM, Lion E, Anguille S, Roelandts VA, Van Acker HH, Heynderickx SDI, Stein BMH, Peeters M, Figdor CG, et al. Engineering monocyte-derived dendritic cells to secrete interferon-alpha enhances their ability to promote adaptive and innate anti-tumor immune effector functions. Cancer Immunol Immunother CII. 2015;64:831–842. doi:10.1007/s00262-015-1688-2.
- Poschke I, Mougiakakos D, Hansson J, Masucci GV, Kiessling R. Immature immunosuppressive CD14+HLA-DR-/low cells in melanoma patients are Stat3hi and overexpress CD80, CD83, and DC-sign. Cancer Res. 2010;70:4335–4345. doi:10.1158/0008-5472.CAN-09-3767.
- Birkholz K, Schwenkert M, Kellner C, Gross S, Fey G, Schuler-Thurner B, Schuler G, Schaft N, Dörrie J. Targeting of DEC-205 on human dendritic cells results in efficient MHC class II-restricted antigen presentation. Blood. 2010;116:2277–2285. doi:10.1182/blood-2010-02-268425.
- Nagahara K, Arikawa T, Oomizu S, Kontani K, Nobumoto A, Tateno H, Watanabe K, Niki T, Katoh S, Miyake M, et al. Galectin-9 increases Tim-3+ dendritic cells and CD8+ T cells and enhances antitumor immunity via galectin-9-Tim-3 interactions. J Immunol. 2008;181:7660–7669. doi:10.4049/jimmunol.181.11.7660.
- Keller AM, Schildknecht A, Xiao Y, van den Broek M, Borst J. Expression of costimulatory ligand CD70 on steady-state dendritic cells breaks CD8+ T cell tolerance and permits effective immunity. Immunity. 2008;29:934–946. doi:10.1016/j.immuni.2008.10.009.
- Idorn M, Olsen M, Halldorsdottir HR, Skadborg SK, Pedersen M, Hogdall C, Høgdall E, Met Ö, thor Straten P. Improved migration of tumor ascites lymphocytes to ovarian cancer microenvironment by CXCR2 transduction. Oncoimmunology. 2018;7:e1412029. doi:10.1080/2162402X.2017.1412029.
- Sprooten J, Ceusters J, Coosemans A, Agostinis P, De Vleeschouwer S, Zitvogel L, Kroemer G, Galluzzi L, Garg AD. Trial watch: dendritic cell vaccination for cancer immunotherapy. OncoImmunology. 2019;8:e1638212. doi:10.1080/2162402X.2019.1638212.
- Lampen MH, van Hall T. Strategies to counteract MHC-I defects in tumors. Curr Opin Immunol. 2011;23:293–298. doi:10.1016/j.coi.2010.12.005.
- Schmid DA, Irving MB, Posevitz V, Hebeisen M, Posevitz-Fejfar A, Sarria JC, Gomez-Eerland R, Thome M, Schumacher TNM, Romero P, et al. Evidence for a TCR affinity threshold delimiting maximal CD8 T cell function. J Immunol. 2010;184:4936–4946. doi:10.4049/jimmunol.1000173.
- Meije CB, Swart GW, Lepoole C, Das PK, Van den Oord JJ. Antigenic profiles of individual-matched pairs of primary and melanoma metastases. Hum Pathol. 2009;40:1399–1407. doi:10.1016/j.humpath.2008.11.018.
- Gubin MM, Artyomov MN, Mardis ER, Schreiber RD. Tumor neoantigens: building a framework for personalized cancer immunotherapy. J Clin Invest. 2015;125:3413–3421. doi:10.1172/JCI80008.
- Zhang Y, Kurupati R, Liu L, Zhou XY, Zhang G, Hudaihed A, Filisio F, Giles-Davis W, Xu X, Karakousis GC, et al. Enhancing CD8+ T cell fatty acid catabolism within a metabolically challenging tumor microenvironment increases the efficacy of melanoma immunotherapy. Cancer Cell. 2017;32:377–91.e9. doi:10.1016/j.ccell.2017.08.004.
- Wennhold K, Thelen M, Schlosser HA, Haustein N, Reuter S, Garcia-Marquez M, Lechner A, Kobold S, Rataj F, Utermöhlen O, et al. Using antigen-specific B cells to combine antibody and T cell-based cancer immunotherapy. Cancer Immunol Res. 2017;5:730–743. doi:10.1158/2326-6066.CIR-16-0236.
- Patel SJ, Sanjana NE, Kishton RJ, Eidizadeh A, Vodnala SK, Cam M, Gartner JJ, Jia L, Steinberg SM, Yamamoto TN, et al. Identification of essential genes for cancer immunotherapy. Nat Immunol. 2017;548:537–542.
- Araki K, Morita M, Bederman AG, Konieczny BT, Kissick HT, Sonenberg N, Ahmed R. Translation is actively regulated during the differentiation of CD8+ effector T cells. Nat Immunol. 2017;18:1046–1057. doi:10.1038/ni.3795.
- De Beck L, Melhaoui S, De Veirman K, Menu E, De Bruyne E, Vanderkerken K, Breckpot K, Maes K. Epigenetic treatment of multiple myeloma mediates tumor intrinsic and extrinsic immunomodulatory effects. Oncoimmunology. 2018;7:e1484981. doi:10.1080/2162402X.2018.1484981.
- Fotaki G, Jin C, Kerzeli IK, Ramachandran M, Martikainen MM, Karlsson-Parra A, Karlsson-Parra A, Yu D, Essand M. Cancer vaccine based on a combination of an infection-enhanced adenoviral vector and pro-inflammatory allogeneic DCs leads to sustained antigen-specific immune responses in three melanoma models. Oncoimmunology. 2018;7:e1397250. doi:10.1080/2162402X.2017.1397250.
- Sharma P, Hu-Lieskovan S, Wargo JA, Ribas A. Primary, adaptive, and acquired resistance to cancer immunotherapy. Cell. 2017;168:707–723. doi:10.1016/j.cell.2017.01.017.
- Joyce JA, Fearon DT. T cell exclusion, immune privilege, and the tumor microenvironment. Science. 2015;348:74–80. doi:10.1126/science.aaa6204.
- Curiel TJ, Coukos G, Zou L, Alvarez X, Cheng P, Mottram P, Evdemon-Hogan M, Conejo-Garcia JR, Zhang L, Burow M, et al. Specific recruitment of regulatory T cells in ovarian carcinoma fosters immune privilege and predicts reduced survival. Nat Med. 2004;10:942–949. doi:10.1038/nm1093.
- Spadoni I, Fornasa G, Rescigno M. Organ-specific protection mediated by cooperation between vascular and epithelial barriers. Nat Rev Immunol. 2017;17:761–773. doi:10.1038/nri.2017.100.
- Chung EY, Liu J, Homma Y, Zhang Y, Brendolan A, Saggese M, Han J, Silverstein R, Selleri L, Ma X, et al. Interleukin-10 expression in macrophages during phagocytosis of apoptotic cells is mediated by homeodomain proteins Pbx1 and Prep-1. Immunity. 2007;27:952–964. doi:10.1016/j.immuni.2007.11.014.
- Fadok VA, Bratton DL, Konowal A, Freed PW, Westcott JY, Henson PM. Macrophages that have ingested apoptotic cells in vitro inhibit proinflammatory cytokine production through autocrine/paracrine mechanisms involving TGF-beta, PGE2, and PAF. J Clin Invest. 1998;101:890–898. doi:10.1172/JCI1112.
- Grivennikov SI, Greten FR, Karin M. Immunity, inflammation, and cancer. Cell. 2010;140:883–899. doi:10.1016/j.cell.2010.01.025.
- Yeku OO, Purdon TJ, Koneru M, Spriggs D, Brentjens RJ. Armored CAR T cells enhance antitumor efficacy and overcome the tumor microenvironment. Sci Rep. 2017;7:10541. doi:10.1038/s41598-017-10940-8.
- Haderk F, Schulz R, Iskar M, Cid LL, Worst T, Willmund KV, Schulz A, Warnken U, Seiler J, Benner A, et al. Tumor-derived exosomes modulate PD-L1 expression in monocytes. Sci Immunol. 2017;2:eaah5509. doi:10.1126/sciimmunol.aah5509.
- Spranger S, Dai D, Horton B, Gajewski TF. Tumor-residing Batf3 dendritic cells are required for effector T cell trafficking and adoptive T cell therapy. Cancer Cell. 2017;31:711–23.e4. doi:10.1016/j.ccell.2017.04.003.
- Serra S, Horenstein AL, Vaisitti T, Brusa D, Rossi D, Laurenti L, D’Arena G, Coscia M, Tripodo C, Inghirami G, et al. CD73-generated extracellular adenosine in chronic lymphocytic leukemia creates local conditions counteracting drug-induced cell death. Blood. 2011;118:6141–6152. doi:10.1182/blood-2011-08-374728.
- McDonald B, Pittman K, Menezes GB, Hirota SA, Slaba I, Waterhouse CC, Beck PL, Muruve DA, Kubes P. Intravascular danger signals guide neutrophils to sites of sterile inflammation. Science. 2010;330:362–366. doi:10.1126/science.1195491.
- Moser B, Wolf M, Walz A, Loetscher P. Chemokines: multiple levels of leukocyte migration control. Trends Immunol. 2004;25:75–84. doi:10.1016/j.it.2003.12.005.
- Taylor NA, Vick SC, Iglesia MD, Brickey WJ, Midkiff BR, McKinnon KP, Reisdorf S, Anders CK, Carey LA, Parker JS, et al. Treg depletion potentiates checkpoint inhibition in claudin-low breast cancer. J Clin Invest. 2017;127:3472–3483. doi:10.1172/JCI90499.
- Li S, Xu F, Zhang J, Wang L, Zheng Y, Wu X, Wang J, Huang Q, Lai M. Tumor-associated macrophages remodeling EMT and predicting survival in colorectal carcinoma. Oncoimmunology. 2018;7:e1380765. doi:10.1080/2162402X.2017.1380765.
- Pardoll DM. The blockade of immune checkpoints in cancer immunotherapy. Nat Rev Cancer. 2012;12:252–264. doi:10.1038/nrc3239.
- Sharma P, Allison JP. The future of immune checkpoint therapy. Science. 2015;348:56–61.
- Garg AD, Vandenberk L, Van Woensel M, Belmans J, Schaaf M, Boon L, De Vleeschouwer S, Agostinis P. Preclinical efficacy of immune-checkpoint monotherapy does not recapitulate corresponding biomarkers-based clinical predictions in glioblastoma. Oncoimmunology. 2017;6:e1295903. doi:10.1080/2162402X.2017.1295903.
- Kuttke M, Sahin E, Pisoni J, Percig S, Vogel A, Kraemmer D, Hanzl L, Brunner JS, Paar H, Soukup K, et al. Myeloid PTEN deficiency impairs tumor-immune surveillance via immune-checkpoint inhibition. Oncoimmunology. 2016;5:e1164918. doi:10.1080/2162402X.2016.1164918.
- Wu M-Z, Cheng W-C, Chen S-F, Nieh S, O’Connor C, Liu C-L, Tsai -W-W, Wu C-J, Martin L, Lin Y-S, et al. miR-25/93 mediates hypoxia-induced immunosuppression by repressing cGAS. Nat Cell Biol. 2017;19:1286–1296. doi:10.1038/ncb3615.
- Turtle CJ, Hay KA, Hanafi LA, Li D, Cherian S, Chen X, Wood B, Lozanski A, Byrd JC, Heimfeld S, et al. Durable molecular remissions in chronic lymphocytic leukemia treated with CD19-specific chimeric antigen receptor-modified T cells after failure of ibrutinib. Nat Cell Biol. 2017;35:3010–3020.
- Manguso RT, Pope HW, Zimmer MD, Brown FD, Yates KB, Miller BC, Collins NB, Bi K, LaFleur MW, Juneja VR, et al. In vivo CRISPR screening identifies Ptpn2 as a cancer immunotherapy target. Sci Signal. 2017;547:413–418.
- Grinberg-Bleyer Y, Oh H, Desrichard A, Bhatt DM, Caron R, Chan TA, Schmid RM, Klein U, Hayden MS, Ghosh S, et al. NF-kappaB c-Rel is crucial for the regulatory T cell immune checkpoint in cancer. Cell. 2017;170:1096–108.e13. doi:10.1016/j.cell.2017.08.004.
- Burr ML, Sparbier CE, Chan YC, Williamson JC, Woods K, Beavis PA, Lam EYN, Henderson MA, Bell CC, Stolzenburg S, et al. CMTM6 maintains the expression of PD-L1 and regulates anti-tumour immunity. Nature. 2017;549:101–105. doi:10.1038/nature23643.
- Budhu S, Schaer DA, Li Y, Toledo-Crow R, Panageas K, Yang X, Zhong H, Houghton AN, Silverstein SC, Merghoub T, et al. Blockade of surface-bound TGF-beta on regulatory T cells abrogates suppression of effector T cell function in the tumor microenvironment. Sci Signal. 2017;10:aak9702. doi:10.1126/scisignal.aak9702.
- Kamran N, Li Y, Sierra M, Alghamri MS, Kadiyala P, Appelman HD, Edwards M, Lowenstein PR, Castro MG. Melanoma induced immunosuppression is mediated by hematopoietic dysregulation. Oncoimmunology. 2018;7:e1408750. doi:10.1080/2162402X.2017.1408750.
- Sharma P, Allison JP. Immune checkpoint targeting in cancer therapy: toward combination strategies with curative potential. Cell. 2015;161:205–214. doi:10.1016/j.cell.2015.03.030.
- Martinenaite E, Mortensen REJ, Hansen M, Orebo Holmstrom M, Munir Ahmad S, Gronne Dahlager Jorgensen N, Met Ö, Donia M, Svane IM, Andersen MH, et al. Frequent adaptive immune responses against arginase-1. Oncoimmunology. 2018;7:e1404215. doi:10.1080/2162402X.2017.1404215.
- Kostine M, Briaire-de Bruijn IH, Cleven AHG, Vervat C, Corver WE, Schilham MW, Van Beelen E, van Boven H, Haas RL, Italiano A, et al. Increased infiltration of M2-macrophages, T-cells and PD-L1 expression in high grade leiomyosarcomas supports immunotherapeutic strategies. Oncoimmunology. 2018;7:e1386828. doi:10.1080/2162402X.2017.1386828.
- Dunn GP, Bruce AT, Ikeda H, Old LJ, Schreiber RD. Cancer immunoediting: from immunosurveillance to tumor escape. Nat Immunol. 2002;3:991–998. doi:10.1038/ni1102-991.
- Henrickson SE, Perro M, Loughhead SM, Senman B, Stutte S, Quigley M, Alexe G, Iannacone M, Flynn M, Omid S, et al. Antigen Availability Determines CD8+ T cell-dendritic cell interaction kinetics and memory fate decisions. Immunity. 2013;39:496–507. doi:10.1016/j.immuni.2013.08.034.
- Nirschl CJ, Suarez-Farinas M, Izar B, Prakadan S, Dannenfelser R, Tirosh I, Liu Y, Zhu Q, Devi KSP, Carroll SL, et al. IFNgamma-dependent tissue-immune homeostasis is co-opted in the tumor microenvironment. Cell. 2017;170:127–41.e15. doi:10.1016/j.cell.2017.06.016.
- Griffith TS, Ferguson TA. Cell death in the maintenance and abrogation of tolerance: the five Ws of dying cells. Immunity. 2011;35:456–466. doi:10.1016/j.immuni.2011.08.011.
- Galluzzi L, Senovilla L, Vitale I, Michels J, Martins I, Kepp O, Castedo M, Kroemer G. Molecular mechanisms of cisplatin resistance. Oncogene. 2012;31:1869–1883. doi:10.1038/onc.2011.384.
- Martins I, Kepp O, Schlemmer F, Adjemian S, Tailler M, Shen S, Michaud M, Menger L, Gdoura A, Tajeddine N, et al. Restoration of the immunogenicity of cisplatin-induced cancer cell death by endoplasmic reticulum stress. Oncogene. 2011;30:1147–1158. doi:10.1038/onc.2010.500.
- Dudek-Peric AM, Ferreira GB, Muchowicz A, Wouters J, Prada N, Martin S, Kiviluoto S, Winiarska M, Boon L, Mathieu C, et al. Antitumor immunity triggered by melphalan is potentiated by melanoma cell surface-associated calreticulin. Cancer Res. 2015;75:1603–1614. doi:10.1158/0008-5472.CAN-14-2089.
- Spranger S, Bao R, Gajewski TF. Melanoma-intrinsic beta-catenin signalling prevents anti-tumour immunity. Nature. 2015;523:231–235. doi:10.1038/nature14404.
- Garg AD, Maes H, van Vliet AR, Agostinis P. Targeting the hallmarks of cancer with therapy-induced endoplasmic reticulum (ER) stress. Mol Cell Oncol. 2015;2:e975089. doi:10.4161/23723556.2014.975089.
- Giglio P, Gagliardi M, Tumino N, Antunes F, Smaili S, Cotella D, Santoro C, Bernardini R, Mattei M, Piacentini M, et al. PKR and GCN2 stress kinases promote an ER stress-independent eIF2alpha phosphorylation responsible for calreticulin exposure in melanoma cells. Oncoimmunology. 2018;7:e1466765. doi:10.1080/2162402X.2018.1466765.
- Fucikova J, Kralikova P, Fialova A, Brtnicky T, Rob L, Bartunkova J, Spisek R. Human tumor cells killed by anthracyclines induce a tumor-specific immune response. Cancer Res. 2011;71:4821–4833. doi:10.1158/0008-5472.CAN-11-0950.
- Sun F, Shi J, Geng C. Dexrazoxane improves cardiac autonomic function in epirubicin-treated breast cancer patients with type 2 diabetes. Medicine. 2016;95:e5228. doi:10.1097/MD.0000000000005228.
- Hemdan T, Johansson R, Jahnson S, Hellstrom P, Tasdemir I, Malmstrom PU. 5-Year outcome of a randomized prospective study comparing bacillus Calmette-Guerin with epirubicin and interferon-alpha2b in patients with T1 bladder cancer. J Urol. 2014;191:1244–1249. doi:10.1016/j.juro.2013.11.005.
- Berry V, Basson L, Bogart E, Mir O, Blay JY, Italiano A, Bertucci F, Chevreau C, Clisant-Delaine S, Liegl-Antzager B, et al. REGOSARC: regorafenib versus placebo in doxorubicin-refractory soft-tissue sarcoma-A quality-adjusted time without symptoms of progression or toxicity analysis. Cancer. 2017;123:2294–2302. doi:10.1002/cncr.v123.12.
- Tap WD, Jones RL, Van Tine BA, Chmielowski B, Elias AD, Adkins D, Agulnik M, Cooney MM, Livingston MB, Pennock G, et al. Olaratumab and doxorubicin versus doxorubicin alone for treatment of soft-tissue sarcoma: an open-label phase 1b and randomised phase 2 trial. Lancet. 2016;388:488–497. doi:10.1016/S0140-6736(16)30587-6.
- Orlowski RZ, Nagler A, Sonneveld P, Blade J, Hajek R, Spencer A, Robak T, Dmoszynska A, Horvath N, Spicka I, et al. Final overall survival results of a randomized trial comparing bortezomib plus pegylated liposomal doxorubicin with bortezomib alone in patients with relapsed or refractory multiple myeloma. Cancer. 2016;122:2050–2056. doi:10.1002/cncr.v122.13.
- Choy E, Flamand Y, Balasubramanian S, Butrynski JE, Harmon DC, George S, Cote GM, Wagner AJ, Morgan JA, Sirisawad M, et al. Phase 1 study of oral abexinostat, a histone deacetylase inhibitor, in combination with doxorubicin in patients with metastatic sarcoma. Cancer. 2015;121:1223–1230. doi:10.1002/cncr.v121.8.
- Prajapati HJ, Xing M, Spivey JR, Hanish SI, El-Rayes BF, Kauh JS, Chen Z, Kim HS. Survival, efficacy, and safety of small versus large doxorubicin drug-eluting beads TACE chemoembolization in patients with unresectable HCC. AJR Am J Roentgenol. 2014;203:W706–14. doi:10.2214/AJR.13.12308.
- Morris PG, Iyengar NM, Patil S, Chen C, Abbruzzi A, Lehman R, Steingart R, Oeffinger KC, Lin N, Moy B, et al. Long-term cardiac safety and outcomes of dose-dense doxorubicin and cyclophosphamide followed by paclitaxel and trastuzumab with and without lapatinib in patients with early breast cancer. Cancer. 2013;119:3943–3951. doi:10.1002/cncr.28284.
- Lipshultz SE, Miller TL, Lipsitz SR, Neuberg DS, Dahlberg SE, Colan SD, Silverman LB, Henkel JM, Franco VI, Cushman LL, et al. Continuous versus bolus infusion of doxorubicin in children with ALL: long-term cardiac outcomes. Pediatrics. 2012;130:1003–1011. doi:10.1542/peds.2012-0727.
- Gulhati P, Raghav K, Shroff RT, Varadhachary GR, Kopetz S, Javle M, Qiao W, Wang H, Morris J, Wolff RA, et al. Bevacizumab combined with capecitabine and oxaliplatin in patients with advanced adenocarcinoma of the small bowel or ampulla of vater: A single-center, open-label, phase 2 study. Cancer. 2017;123:1011–1017. doi:10.1002/cncr.30445.
- Meulendijks D, de Groot JW, Los M, Boers JE, Beerepoot LV, Polee MB, Beeker A, Portielje JEA, Goey SH, de Jong RS, et al. Bevacizumab combined with docetaxel, oxaliplatin, and capecitabine, followed by maintenance with capecitabine and bevacizumab, as first-line treatment of patients with advanced HER2-negative gastric cancer: A multicenter phase 2 study. Cancer. 2016;122:1434–1443. doi:10.1002/cncr.v122.9.
- Leone F, Marino D, Cereda S, Filippi R, Belli C, Spadi R, Nasti G, Montano M, Amatu A, Aprile G, et al. Panitumumab in combination with gemcitabine and oxaliplatin does not prolong survival in wild-type KRAS advanced biliary tract cancer: A randomized phase 2 trial (Vecti-BIL study). Cancer. 2016;122:574–581. doi:10.1002/cncr.v122.4.
- O’Reilly EM, Perelshteyn A, Jarnagin WR, Schattner M, Gerdes H, Capanu M, Tang LH, LaValle J, Winston C, DeMatteo RP, et al. A single-arm, nonrandomized phase II trial of neoadjuvant gemcitabine and oxaliplatin in patients with resectable pancreas adenocarcinoma. Ann Surg. 2014;260:142–148. doi:10.1097/SLA.0000000000000251.
- Leone F, Artale S, Marino D, Cagnazzo C, Cascinu S, Pinto C, Fornarini G, Tampellini M, Di Fabio F, Sartore-Bianchi A, et al. Panitumumab in combination with infusional oxaliplatin and oral capecitabine for conversion therapy in patients with colon cancer and advanced liver metastases. The metapan study. Cancer. 2013;119:3429–3435. doi:10.1002/cncr.28223.
- Kim EJ, Ben-Josef E, Herman JM, Bekaii-Saab T, Dawson LA, Griffith KA, Francis IR, Greenson JK, Simeone DM, Lawrence TS, et al. A multi-institutional phase 2 study of neoadjuvant gemcitabine and oxaliplatin with radiation therapy in patients with pancreatic cancer. Cancer. 2013;119:2692–2700. doi:10.1002/cncr.28117.
- Kidwell KM, Yothers G, Ganz PA, Land SR, Ko CY, Cecchini RS, Kopec JA, Wolmark N. Long-term neurotoxicity effects of oxaliplatin added to fluorouracil and leucovorin as adjuvant therapy for colon cancer: results from national surgical adjuvant breast and bowel project trials C-07 and LTS-01. Cancer. 2012;118:5614–5622. doi:10.1002/cncr.27593.
- Attal M, Lauwers-Cances V, Hulin C, Leleu X, Caillot D, Escoffre M, Arnulf B, Macro M, Belhadj K, Garderet L, et al. Lenalidomide, bortezomib, and dexamethasone with transplantation for myeloma. N Engl J Med. 2017;376:1311–1320. doi:10.1056/NEJMoa1611750.
- Palumbo A, Chanan-Khan A, Weisel K, Nooka AK, Masszi T, Beksac M, Spicka I, Hungria V, Munder M, Mateos MV, et al. Daratumumab, bortezomib, and dexamethasone for multiple myeloma. N Engl J Med. 2016;375:754–766. doi:10.1056/NEJMoa1606038.
- Kumar SK, LaPlant BR, Reeder CB, Roy V, Halvorson AE, Buadi F, Gertz MA, Bergsagel PL, Dispenzieri A, Thompson MA, et al. Randomized phase 2 trial of ixazomib and dexamethasone in relapsed multiple myeloma not refractory to bortezomib. Blood. 2016;128:2415–2422. doi:10.1182/blood-2016-05-717769.
- Jakubowiak A, Offidani M, Pegourie B, De La Rubia J, Garderet L, Laribi K, Bosi A, Marasca R, Laubach J, Mohrbacher A, et al. Randomized phase 2 study: elotuzumab plus bortezomib/dexamethasone vs bortezomib/dexamethasone for relapsed/refractory MM. Blood. 2016;127:2833–2840. doi:10.1182/blood-2016-01-694604.
- Chari A, Htut M, Zonder JA, Fay JW, Jakubowiak AJ, Levy JB, Lau K, Burt SM, Tunquist BJ, Hilder BW, et al. A phase 1 dose-escalation study of filanesib plus bortezomib and dexamethasone in patients with recurrent/refractory multiple myeloma. Cancer. 2016;122:3327–3335. doi:10.1002/cncr.30174.
- Robak T, Huang H, Jin J, Zhu J, Liu T, Samoilova O, Pylypenko H, Verhoef G, Siritanaratkul N, Osmanov E, et al. Bortezomib-based therapy for newly diagnosed mantle-cell lymphoma. N Engl J Med. 2015;372:944–953. doi:10.1056/NEJMoa1412096.
- Offner F, Samoilova O, Osmanov E, Eom H-S, Topp MS, Raposo J, Pavlov V, Ricci D, Chaturvedi S, Zhu E, et al. Frontline rituximab, cyclophosphamide, doxorubicin, and prednisone with bortezomib (VR-CAP) or vincristine (R-CHOP) for non-GCB DLBCL. Blood. 2015;126:1893–1901. doi:10.1182/blood-2015-03-632430.
- Fenske TS, Shah NM, Kim KM, Saha S, Zhang C, Baim AE, Farnen JP, Onitilo AA, Blank JH, Ahuja H, et al. A phase 2 study of weekly temsirolimus and bortezomib for relapsed or refractory B-cell non-Hodgkin lymphoma: A Wisconsin Oncology Network study. Cancer. 2015;121:3465–3471. doi:10.1002/cncr.v121.19.
- Spisek R, Charalambous A, Mazumder A, Vesole DH, Jagannath S, Dhodapkar MV. Bortezomib enhances dendritic cell (DC)-mediated induction of immunity to human myeloma via exposure of cell surface heat shock protein 90 on dying tumor cells: therapeutic implications. Blood. 2007;109:4839–4845. doi:10.1182/blood-2006-10-054221.
- Demaria S, Santori FR, Ng B, Liebes L, Formenti SC, Vukmanovic S. Select forms of tumor cell apopto sis induce dendritic cell maturation. J Leukoc Biol. 2005;77:361–368. doi:10.1189/jlb.0804478.
- Cirone M, Di Renzo L, Lotti LV, Conte V, Trivedi P, Santarelli R, Gonnella R, Frati L, Faggioni A. Primary effusion lymphoma cell death induced by bortezomib and AG 490 activates dendritic cells through CD91. PLoS One. 2012;7:e31732. doi:10.1371/journal.pone.0031732.
- Baz RC, Martin TG 3rd, Lin HY, Zhao X, Shain KH, Cho HJ, Wolf JL, Mahindra A, Chari A, Sullivan DM, et al. Randomized multicenter phase 2 study of pomalidomide, cyclophosphamide, and dexamethasone in relapsed refractory myeloma. Blood. 2016;127:2561–2568. doi:10.1182/blood-2015-11-682518.
- Short NJ, Keating MJ, Wierda WG, Faderl S, Ferrajoli A, Estrov Z, Smith SC, O’Brien SM. Fludarabine, cyclophosphamide, and multiple-dose rituximab as frontline therapy for chronic lymphocytic leukemia. Cancer. 2015;121:3869–3876. doi:10.1002/cncr.29605.
- Rossi D, Terzi-di-Bergamo L, De Paoli L, Cerri M, Ghilardi G, Chiarenza A, Bulian P, Visco C, Mauro FR, Morabito F, et al. Molecular prediction of durable remission after first-line fludarabine-cyclophosphamide-rituximab in chronic lymphocytic leukemia. Blood. 2015;126:1921–1924. doi:10.1182/blood-2015-05-647925.
- Pagnoux C, Quemeneur T, Ninet J, Diot E, Kyndt X, de Wazieres B, Reny J-L, Puéchal X, le Berruyer P-Y, Lidove O, et al. Treatment of systemic necrotizing vasculitides in patients aged sixty-five years or older: results of a multicenter, open-label, randomized controlled trial of corticosteroid and cyclophosphamide-based induction therapy. Arthritis Rheumatol. 2015;67:1117–1127. doi:10.1002/art.v67.4.
- Kastritis E, Gavriatopoulou M, Kyrtsonis MC, Roussou M, Hadjiharissi E, Symeonidis A, Repoussis P, Michalis E, Delimpasi S, Tsatalas K, et al. Dexamethasone, rituximab, and cyclophosphamide as primary treatment of Waldenstrom macroglobulinemia: final analysis of a phase 2 study. Blood. 2015;126:1392–1394. doi:10.1182/blood-2015-05-647420.
- Brown JR, O’Brien S, Kingsley CD, Eradat H, Pagel JM, Lymp J, Hirata J, Kipps TJ. Obinutuzumab plus fludarabine/cyclophosphamide or bendamustine in the initial therapy of CLL patients: the phase 1b GALTON trial. Blood. 2015;125:2779–2785. doi:10.1182/blood-2014-12-613570.
- Geisler CH, van T’ Veer MB, Jurlander J, Walewski J, Tjonnfjord G, Itala Remes M, Kimby E, Kozak T, Polliack A, Wu KL, et al. Frontline low-dose alemtuzumab with fludarabine and cyclophosphamide prolongs progression-free survival in high-risk CLL. Blood. 2014;123:3255–3262. doi:10.1182/blood-2014-01-547737.
- Derosa L, Galli L, Orlandi P, Fioravanti A, Di Desidero T, Fontana A, Antonuzzo A, Biasco E, Farnesi A, Marconcini R, et al. Docetaxel plus oral metronomic cyclophosphamide: a phase II study with pharmacodynamic and pharmacogenetic analyses in castration-resistant prostate cancer patients. Cancer. 2014;120:3923–3931. doi:10.1002/cncr.28953.
- Abrisqueta P, Villamor N, Terol MJ, Gonzalez-Barca E, Gonzalez M, Ferra C, Abella E, Delgado J, García-Marco JA, González Y, et al. Rituximab maintenance after first-line therapy with rituximab, fludarabine, cyclophosphamide, and mitoxantrone (R-FCM) for chronic lymphocytic leukemia. Blood. 2013;122:3951–3959. doi:10.1182/blood-2013-05-502773.
- Schiavoni G, Sistigu A, Valentini M, Mattei F, Sestili P, Spadaro F, Sanchez M, Lorenzi S, D’Urso MT, Belardelli F, et al. Cyclophosphamide synergizes with type I interferons through systemic dendritic cell reactivation and induction of immunogenic tumor apoptosis. Cancer Res. 2011;71:768–778. doi:10.1158/0008-5472.CAN-10-2788.
- Bugaut H, Bruchard M, Berger H, Derangere V, Odoul L, Euvrard R, Ladoire S, Chalmin F, Végran F, Rébé C, et al. Bleomycin exerts ambivalent antitumor immune effect by triggering both immunogenic cell death and proliferation of regulatory T cells. PLoS One. 2013;8:e65181. doi:10.1371/journal.pone.0065181.
- Borgoni S, Iannello A, Cutrupi S, Allavena P, D’Incalci M, Novelli F, Cappello P. Depletion of tumor-associated macrophages switches the epigenetic profile of pancreatic cancer infiltrating T cells and restores their anti-tumor phenotype. Oncoimmunology. 2018;7:e1393596. doi:10.1080/2162402X.2017.1393596.
- McKee SJ, Tuong ZK, Kobayashi T, Doff BL, Soon MS, Nissen M, Lam PY, Keane C, Vari F, Moi D, et al. B cell lymphoma progression promotes the accumulation of circulating Ly6Clo monocytes with immunosuppressive activity. Oncoimmunology. 2018;7:e1393599. doi:10.1080/2162402X.2017.1393599.
- Kersten K, Salvagno C, de Visser KE. Exploiting the immunomodulatory properties of chemotherapeutic drugs to improve the success of cancer immunotherapy. Front Immunol. 2015;6:516. doi:10.3389/fimmu.2015.00516.
- Ivagnes A, Messaoudene M, Stoll G, Routy B, Fluckiger A, Yamazaki T, Iribarren K, Duong CPM, Fend L, Caignard A, et al. TNFR2/BIRC3-TRAF1 signaling pathway as a novel NK cell immune checkpoint in cancer. Oncoimmunology. 2018;7:e1386826. doi:10.1080/2162402X.2017.1386826.
- Coffelt SB, de Visser KE. Immune-mediated mechanisms influencing the efficacy of anticancer therapies. Trends Immunol. 2015;36:198–216. doi:10.1016/j.it.2015.02.006.
- Allen F, Bobanga ID, Rauhe P, Barkauskas D, Teich N, Tong C, Myers J, Huang AY. CCL3 augments tumor rejection and enhances CD8(+) T cell infiltration through NK and CD103(+) dendritic cell recruitment via IFNγ. Oncoimmunology. 2018;7:e1393598. doi:10.1080/2162402X.2017.1393598.
- Dewan MZ, Galloway AE, Kawashima N, Dewyngaert JK, Babb JS, Formenti SC, Demaria S. Fractionated but not single-dose radiotherapy induces an immune-mediated abscopal effect when combined with anti-CTLA-4 antibody. Clin Cancer Res off J Am Assoc Cancer Res. 2009;15:5379–5388. doi:10.1158/1078-0432.CCR-09-0265.
- Bouquet F, Pal A, Pilones KA, Demaria S, Hann B, Akhurst RJ, Babb JS, Lonning SM, DeWyngaert JK, Formenti SC, et al. TGF 1 inhibition increases the radiosensitivity of breast cancer cells in vitro and promotes tumor control by radiation in vivo. Clin Cancer Res off J Am Assoc Cancer Res. 2011;17:6754–6765. doi:10.1158/1078-0432.CCR-11-0544.
- Wennerberg E, Lhuillier C, Vanpouille-Box C, Pilones KA, Garcia-Martinez E, Rudqvist N-P, Formenti SC, Demaria S. Barriers to radiation-induced in situ tumor vaccination. Front Immunol. 2017;8:229. doi:10.3389/fimmu.2017.00229.
- Jarosch A, Sommer U, Bogner A, Reissfelder C, Weitz J, Krause M, Folprecht G, Baretton GB, Aust DE. Neoadjuvant radiochemotherapy decreases the total amount of tumor infiltrating lymphocytes, but increases the number of CD8+/Granzyme B+ (GrzB) cytotoxic T-cells in rectal cancer. Oncoimmunology. 2018;7:e1393133. doi:10.1080/2162402X.2017.1393133.
- Deutsch E, Chargari C, Galluzzi L, Kroemer G. Optimising efficacy and reducing toxicity of anticancer radioimmunotherapy. Lancet Oncol. 2019;20:e452–e63. doi:10.1016/S1470-2045(19)30171-8.
- Bolli E, O’Rourke JP, Conti L, Lanzardo S, Rolih V, Christen JM, Barutello G, Forni M, Pericle F, Cavallo F, et al. A virus-like-particle immunotherapy targeting epitope-specific anti-xCT expressed on cancer stem cell inhibits the progression of metastatic cancer in vivo. Oncoimmunology. 2018;7:e1408746. doi:10.1080/2162402X.2017.1408746.
- Moserova I, Truxova I, Garg AD, Tomala J, Agostinis P, Cartron PF, Vosahlikova S, Kovar M, Spisek R, Fucikova J, et al. Caspase-2 and oxidative stress underlie the immunogenic potential of high hydrostatic pressure-induced cancer cell death. Oncoimmunology. 2017;6:e1258505. doi:10.1080/2162402X.2016.1258505.
- Tatsuno K, Yamazaki T, Hanlon D, Han P, Robinson E, Sobolev O, Yurter A, Rivera-Molina F, Arshad N, Edelson RL, et al. Extracorporeal photochemotherapy induces bona fide immunogenic cell death. Cell Death Dis. 2019;10:578. doi:10.1038/s41419-019-1819-3.
- Adams JL, Smothers J, Srinivasan R, Hoos A. Big opportunities for small molecules in immuno-oncology. Nat Rev Drug Discov. 2015;14:603–622. doi:10.1038/nrd4596.
- Tang J, Shalabi A, Hubbard-Lucey VM. Comprehensive analysis of the clinical immuno-oncology landscape. Ann Oncol. 2018;29:84–91. doi:10.1093/annonc/mdx755.
- Bezu L, Sauvat A, Humeau J, Gomes-da-Silva LC, Iribarren K, Forveille S, Garcia P, Zhao L, Liu P, Zitvogel L, et al. eIF2α phosphorylation is pathognomonic for immunogenic cell death. Cell Death Differ. 2018;25:1375–1393. doi:10.1038/s41418-017-0044-9.
- Cari L, Nocentini G, Migliorati G, Riccardi C. Potential effect of tumor-specific Treg-targeted antibodies in the treatment of human cancers: A bioinformatics analysis. Oncoimmunology. 2018;7:e1387705. doi:10.1080/2162402X.2017.1387705.
- Lecciso M, Ocadlikova D, Sangaletti S, Trabanelli S, De Marchi E, Orioli E, Pegoraro A, Portararo P, Jandus C, Bontadini A, et al. ATP release from chemotherapy-treated dying leukemia cells elicits an immune suppressive effect by increasing regulatory t cells and tolerogenic dendritic cells. Front Immunol. 2017;8:1918. doi:10.3389/fimmu.2017.01918.
- Cao B, Wang Q, Zhang H, Zhu G, Lang J. Two immune-enhanced molecular subtypes differ in inflammation, checkpoint signaling and outcome of advanced head and neck squamous cell carcinoma. Oncoimmunology. 2018;7:e1392427. doi:10.1080/2162402X.2017.1392427.
- Boxberg M, Steiger K, Lenze U, Rechl H, von Eisenhart-rothe R, Wortler K, Weichert W, Langer R, Specht K. PD-L1 and PD-1 and characterization of tumor-infiltrating lymphocytes in high grade sarcomas of soft tissue - prognostic implications and rationale for immunotherapy. Oncoimmunology. 2018;7:e1389366. doi:10.1080/2162402X.2017.1389366.
- Huang FY, Lei J, Sun Y, Yan F, Chen B, Zhang L, Lu Z, Cao R, Lin YY, Wang CC, et al. Induction of enhanced immunogenic cell death through ultrasound-controlled release of doxorubicin by liposome-microbubble complexes. Oncoimmunology. 2018;7:e1446720. doi:10.1080/2162402X.2018.1446720.
- Mastria EM, Cai LY, Kan MJ, Li X, Schaal JL, Fiering S, Gunn MD, Dewhirst MW, Nair SK, Chilkoti A, et al. Nanoparticle formulation improves doxorubicin efficacy by enhancing host antitumor immunity. J Control Release. 2018;269:364–373. doi:10.1016/j.jconrel.2017.11.021.
- Yang W, Zhu G, Wang S, Yu G, Yang Z, Lin L, Zhou Z, Liu Y, Dai Y, Zhang F, et al. In situ dendritic cell vaccine for effective cancer immunotherapy. ACS Nano. 2019;13:3083–3094. doi:10.1021/acsnano.8b08346.
- Lu J, Liu X, Liao YP, Salazar F, Sun B, Jiang W, Chang CH, Jiang J, Wang X, Wu AM, et al. Nano-enabled pancreas cancer immunotherapy using immunogenic cell death and reversing immunosuppression. Nat Commun. 2017;8:1811. doi:10.1038/s41467-017-01651-9.
- Liu Q, Chen F, Hou L, Shen L, Zhang X, Wang D, Huang L. Nanocarrier-mediated chemo-immunotherapy arrested cancer progression and induced tumor dormancy in desmoplastic melanoma. ACS Nano. 2018;12:7812–7825. doi:10.1021/acsnano.8b01890.
- Laubli H, Koelzer VH, Matter MS, Herzig P, Dolder Schlienger B, Wiese MN, Lardinois D, Mertz KD, Zippelius A. The T cell repertoire in tumors overlaps with pulmonary inflammatory lesions in patients treated with checkpoint inhibitors. Oncoimmunology. 2018;7:e1386362. doi:10.1080/2162402X.2017.1386362.
- Fend L, Yamazaki T, Remy C, Fahrner C, Gantzer M, Nourtier V, Préville X, Quéméneur E, Kepp O, Adam J, et al. Immune checkpoint blockade, immunogenic chemotherapy or IFN-α blockade boost the local and abscopal effects of oncolytic virotherapy. Cancer Res. 2017;77:4146–4157. doi:10.1158/0008-5472.CAN-16-2165.
- Camilio KA, Wang M-Y, Mauseth B, Waagene S, Kvalheim G, Rekdal Ø, Sveinbjørnsson B, Mælandsmo GM. Combining the oncolytic peptide LTX-315 with doxorubicin demonstrates therapeutic potential in a triple-negative breast cancer model. Breast Cancer Res. 2019;21:9. doi:10.1186/s13058-018-1092-x.
- Groza D, Gehrig S, Kudela P, Holcmann M, Pirker C, Dinhof C, Schueffl HH, Sramko M, Hoebart J, Alioglu F, et al. Bacterial ghosts as adjuvant to oxaliplatin chemotherapy in colorectal carcinomatosis. Oncoimmunology. 2018;7:e1424676. doi:10.1080/2162402X.2018.1424676.
- Gao J, Deng F, Jia W. Inhibition of indoleamine 2,3-dioxygenase enhances the therapeutic efficacy of immunogenic chemotherapeutics in breast cancer. J Breast Cancer. 2019;22:196–209. doi:10.4048/jbc.2019.22.e23.
- Gebremeskel S, Lobert L, Tanner K, Walker B, Oliphant T, Clarke LE, Dellaire G, Johnston B. Natural killer T-cell immunotherapy in combination with chemotherapy-induced immunogenic cell death targets metastatic breast cancer. Cancer Immunol Res. 2017;5:1086–1097. doi:10.1158/2326-6066.CIR-17-0229.
- Combes E, Andrade AF, Tosi D, Michaud HA, Coquel F, Garambois V, Desigaud D, Jarlier M, Coquelle A, Pasero P, et al. Inhibition of ataxia-telangiectasia mutated and RAD3-Related (ATR) overcomes oxaliplatin resistance and promotes antitumor immunity in colorectal cancer. Cancer Res. 2019;79:2933–2946. doi:10.1158/0008-5472.CAN-18-2807.
- Voorwerk L, Slagter M, Horlings HM, Sikorska K, van de Vijver KK, de Maaker M, Nederlof I, Kluin RJ, Warren S, Ong S, et al. Immune induction strategies in metastatic triple-negative breast cancer to enhance the sensitivity to PD-1 blockade: the TONIC trial. Nat Med. 2019;25:920–928. doi:10.1038/s41591-019-0432-4.
- Scurr M, Pembroke T, Bloom A, Roberts D, Thomson A, Smart K, Bridgeman H, Adams R, Brewster A, Jones R, et al. Effect of modified vaccinia Ankara-5T4 and low-dose cyclophosphamide on antitumor immunity in metastatic colorectal cancer: a randomized clinical trial. JAMA Oncol. 2017;3:e172579. doi:10.1001/jamaoncol.2017.2579.
- Federico SM, McCarville MB, Shulkin BL, Sondel PM, Hank JA, Hutson P, Meagher M, Shafer A, Ng CY, Leung W, et al. A pilot trial of humanized anti-GD2 monoclonal antibody (hu14.18K322A) with chemotherapy and natural killer cells in children with recurrent/refractory neuroblastoma. Clinical cancer research: an official. J Am Assoc Cancer Res. 2017;23:6441–6449.
- Bota DA, Chung J, Dandekar M, Carrillo JA, Kong XT, Fu BD, Hsu FP, Schönthal AH, Hofman FM, Chen TC, et al. Phase II study of ERC1671 plus bevacizumab versus bevacizumab plus placebo in recurrent glioblastoma: interim results and correlations with CD4(+) T-lymphocyte counts. CNS Oncol. 2018;7:CNS22. doi:10.2217/cns-2018-0009.
- Kanekiyo S, Hazama S, Takenouchi H, Nakajima M, Shindo Y, Matsui H, Tokumitsu Y, Tomochika S, Tsunedomi R, Tokuhisa Y, et al. IgG response to MHC class I epitope peptides is a quantitative predictive biomarker in the early course of treatment of colorectal cancer using therapeutic peptides. Oncol Rep. 2018;39:2385–2392. doi:10.3892/or.2018.6288.
- Geyer MB, Riviere I, Senechal B, Wang X, Wang Y, Purdon TJ, Hsu M, Devlin SM, Halton E, Lamanna N, et al. Autologous CD19-targeted CAR T cells in patients with residual CLL following initial purine analog-based therapy. Mol Ther. 2018;26:1896–1905. doi:10.1016/j.ymthe.2018.05.018.
- Foukakis T, Lovrot J, Matikas A, Zerdes I, Lorent J, Tobin N, Suzuki C, Brage SE, Carlsson L, Einbeigi Z, et al. Immune gene expression and response to chemotherapy in advanced breast cancer. Br J Cancer. 2018;118:480–488. doi:10.1038/bjc.2017.446.
- Kwa M, Li X, Novik Y, Oratz R, Jhaveri K, Wu J, Gu P, Meyers M, Muggia F, Speyer J, et al. Serial immunological parameters in a phase II trial of exemestane and low-dose oral cyclophosphamide in advanced hormone receptor-positive breast cancer. Breast Cancer Res Treat. 2018;168:57–67. doi:10.1007/s10549-017-4570-4.
- Werter IM, Huijts CM, Lougheed SM, Hamberg P, Polee MB, Tascilar M, Los M, Haanen JB, Helgason HH, Verheul HM, et al. Metronomic cyclophosphamide attenuates mTOR-mediated expansion of regulatory T cells, but does not impact clinical outcome in patients with metastatic renal cell cancer treated with everolimus. Cancer Immunol Immunother CII. 2019;68:787–798. doi:10.1007/s00262-019-02313-z.
- Toulmonde M, Penel N, Adam J, Chevreau C, Blay JY, Le Cesne A, Bompas E, Piperno-Neumann S, Cousin S, Grellety T, et al. Use of PD-1 targeting, macrophage infiltration, and IDO pathway activation in sarcomas: a phase 2 clinical trial. JAMA Oncol. 2018;4:93–97. doi:10.1001/jamaoncol.2017.1617.
- Stevens WBC, Mendeville M, Redd R, Clear AJ, Bladergroen R, Calaminici M, Rosenwald A, Hoster E, Hiddemann W, Gaulard P, et al. Prognostic relevance of CD163 and CD8 combined with EZH2 and gain of chromosome 18 in follicular lymphoma: a study by the lunenburg lymphoma biomarker consortium. Haematologica. 2017;102:1413–1423. doi:10.3324/haematol.2017.165415.
- Aspeslagh S, Matias M, Palomar V, Dercle L, Lanoy E, Soria JC, Postel-Vinay S. In the immuno-oncology era, is anti-PD-1 or anti-PD-L1 immunotherapy modifying the sensitivity to conventional cancer therapies? Eur J Cancer. 2017;87:65–74. doi:10.1016/j.ejca.2017.09.027.
- Overman MJ, McDermott R, Leach JL, Lonardi S, Lenz HJ, Morse MA, Desai J, Hill A, Axelson M, Moss RA, et al. Nivolumab in patients with metastatic DNA mismatch repair-deficient or microsatellite instability-high colorectal cancer (CheckMate 142): an open-label, multicentre, phase 2 study. Lancet Oncol. 2017;18:1182–1191. doi:10.1016/S1470-2045(17)30422-9.
- Keung EZ, Tsai JW, Ali AM, Cormier JN, Bishop AJ, Guadagnolo BA, Torres KE, Somaiah N, Hunt KK, Wargo JA, et al. Analysis of the immune infiltrate in undifferentiated pleomorphic sarcoma of the extremity and trunk in response to radiotherapy: rationale for combination neoadjuvant immune checkpoint inhibition and radiotherapy. Oncoimmunology. 2018;7:e1385689. doi:10.1080/2162402X.2017.1385689.
- Sabatos-Peyton CA, Nevin J, Brock A, Venable JD, Tan DJ, Kassam N, Xu F, Taraszka J, Wesemann L, Pertel T, et al. Blockade of Tim-3 binding to phosphatidylserine and CEACAM1 is a shared feature of anti-Tim-3 antibodies that have functional efficacy. Oncoimmunology. 2018;7:e1385690. doi:10.1080/2162402X.2017.1385690.
- Vanpouille-Box C, Lhuillier C, Bezu L, Aranda F, Yamazaki T, Kepp O, Fucikova J, Spisek R, Demaria S, Formenti SC, et al. Trial watch: immune checkpoint blockers for cancer therapy. Oncoimmunology. 2017;6:e1373237. doi:10.1080/2162402X.2017.1373237.
- Raikar SS, Fleischer LC, Moot R, Fedanov A, Paik NY, Knight KA, Doering CB, Spencer HT. Development of chimeric antigen receptors targeting T-cell malignancies using two structurally different anti-CD5 antigen binding domains in NK and CRISPR-edited T cell lines. Oncoimmunology. 2018;7:e1407898. doi:10.1080/2162402X.2017.1407898.
- Priceman SJ, Gerdts EA, Tilakawardane D, Kennewick KT, Murad JP, Park AK, Jeang B, Yamaguchi Y, Yang X, Urak R, et al. Co-stimulatory signaling determines tumor antigen sensitivity and persistence of CAR T cells targeting PSCA+ metastatic prostate cancer. Oncoimmunology. 2018;7:e1380764. doi:10.1080/2162402X.2017.1380764.
- Pettitt D, Arshad Z, Smith J, Stanic T, Hollander G, Brindley D. CAR-T cells: a systematic review and mixed methods analysis of the clinical trial landscape. Mol Ther. 2018;26:342–353. doi:10.1016/j.ymthe.2017.10.019.
- Pol J, Vacchelli E, Aranda F, Castoldi F, Eggermont A, Cremer I, Sautes-Fridman C, Fucikova J, Galon J, Spisek R, et al. Trial watch: immunogenic cell death inducers for anticancer chemotherapy. Oncoimmunology. 2015;4:e1008866. doi:10.1080/2162402X.2015.1008866.
- Morano WF, Aggarwal A, Love P, Richard SD, Esquivel J, Bowne WB. Intraperitoneal immunotherapy: historical perspectives and modern therapy. Cancer Gene Ther. 2016;23:373–381. doi:10.1038/cgt.2016.49.
- Shekarian T, Valsesia-Wittmann S, Caux C, Marabelle A. Paradigm shift in oncology: targeting the immune system rather than cancer cells. Mutagenesis. 2015;30:205–211. doi:10.1093/mutage/geu073.
- Lazzari C, Bulotta A, Ducceschi M, Vigano MG, Brioschi E, Corti F, Gianni L, Gregorc V. Historical evolution of second-line therapy in non-small cell lung cancer. Front Med. 2017;4:4. doi:10.3389/fmed.2017.00004.
- Kerbel RS, Shaked Y. The potential clinical promise of ‘multimodality’ metronomic chemotherapy revealed by preclinical studies of metastatic disease. Cancer Lett. 2017;400:293–304. doi:10.1016/j.canlet.2017.02.005.
- Chen YL, Chang MC, Cheng WF. Metronomic chemotherapy and immunotherapy in cancer treatment. Cancer Lett. 2017;400:282–292. doi:10.1016/j.canlet.2017.01.040.
- Weiss T, Weller M, Roth P. Immunological effects of chemotherapy and radiotherapy against brain tumors. Expert Rev Anticancer Ther. 2016;16:1087–1094. doi:10.1080/14737140.2016.1229600.
- Cook AM, Lesterhuis WJ, Nowak AK, Lake RA. Chemotherapy and immunotherapy: mapping the road ahead. Curr Opin Immunol. 2016;39:23–29. doi:10.1016/j.coi.2015.12.003.
- Fucikova J, Truxova I, Hensler M, Becht E, Kasikova L, Moserova I, Vosahlikova S, Klouckova J, Church SE, Cremer I, et al. Calreticulin exposure by malignant blasts correlates with robust anticancer immunity and improved clinical outcome in AML patients. Blood. 2016;128:3113–3124. doi:10.1182/blood-2016-08-731737.