Abstract
Several studies have been devoted to clear functionalization of gold nanoparticles (AuNPs) in different fields such as cellular and molecular biology, microbiology, immunology and physiology. In line with the high diagnostic value of AuNPs, its therapeutic application has been intensively developed in tumour therapy, in recent years. One of the best clinical applications of AuNPs is its use in targeted delivery of anti-cancer drugs. Recent studies have focused on the application of AuNPs to treat melanoma – a malignant neoplasm sourced from melanocytes skin cells – with poor prognosis in advanced stages. Furthermore, early diagnosis can be successfully achieved through utilizing this technique even at early stages with localized distribution. Herein, this study details the previous researches focusing on the use of AuNPs as a novel diagnostic and therapeutic option in management of melanoma.
Introduction
The therapeutic and diagnostic applications of gold nanoparticle (AuNP) as one of the first discovered metals have been initially described by the Eastern scientists [Citation1]. In Europe, colloidal gold was primarily used to treat some mental disorders, rheumatological diseases and even infectious disorders [Citation2]. The first comprehensive book on medical applications of the colloidal gold was presented by Anthony in 1618, containing some information on how to obtain colloidal gold and how to use this particle in medicine [Citation3,Citation4]. Despite its wide application as a therapeutic agent in clinical setting, its structuring and using in form of AuNPs had already been returned to 1971 when antibodies conjugated with colloidal gold were used to directly detect bacterial antigens such as salmonellae [Citation5]. Since then, several studies have been dedicated to clear functionalization of the AuNP in different fields such as cellular and molecular biology, microbiology, immunology and physiology [Citation6–9]. Using these nanoparticles, conjugated with different antibodies and enzymes leads to production of an extremely wide range of AuNP aiming at setting immunoanalysis and photothermolysis of cancer cells and infectious microorganisms – producing the targeted delivery of drugs, and even setting bio-imaging [Citation10]. Thus, various medicinal applications were developed for AuNP in diagnostic, preventive and therapeutic fields which led to publishing several reviews and books as well as creating a wide perspective in application of these particles. These applications are directly beholden to gold specific optical properties such as plasmon resonance giving rise to visualization of these particles, helping identification of different biological agents [Citation10]. In bio-imaging field, owing to high electron density of AuNP, these particles are now applied to assess different bio-specific molecular interactions and study the causative agents of infectious diseases through the detection of their specific membranous antigens [Citation11,Citation12]. Concurrently, preferential binding of AuNP conjugated with antibodies against cancer specific superficial antigens – compared to healthy cells assessed by resonance scattering dark-field microscopy – accurately facilitates diagnosing and tracking and mapping cancer cells [Citation13–15].
Melanoma is a malignant neoplasm associated with the melanocytes in epidermis [Citation16]. The methods of its treatment include surgical resection of tumours, followed by radiation therapy or chemotherapy. Recent studies have been concerned about the application of AuNP in order to treat melanoma even in advanced stages. Moreover, employing this technique has engendered a successfully early diagnosis of diseases even in early stages with localized distribution. This study provides a comprehensive survey of previous publications on AuNP as a new diagnostic and therapeutic option in management of melanoma.
Diagnostic and therapeutic applications of gold nanoparticle
Photothermal therapy
In this regard, photothermal therapy with AuNP by its selective delivering into tumour cells is commonplace in this technical treatment approach and has been developing as a therapy for cancer () [Citation17].
Figure 1. Different applications of gold nanoparticles in diagnosis and therapy. Nanoparticles are used in a variety of contexts such as: photo thermal therapy, targeting, drug delivery, imaging, nucleic acid delivery, toxin and microbial agent removal and as an adjuvant.
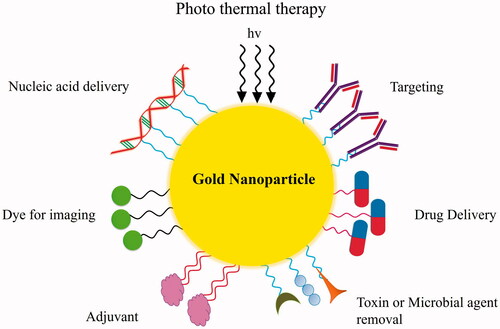
AuNPs have been used for photothermal therapy because of their several major benefits, one of which is its easy link to the thiol and provides connectivity to target ligands and therapeutic molecules. In addition, AuNP absorbs near-infrared (NIR) light, which is suitable for photothermal therapy [Citation18–21]. The nature of this technique is based on maximizing the absorption of AuNP with the optimal optic properties in the visible cellular area, and getting hot when located around the target region so AuNPs are delivered into tumours and are irradiated with laser light and then absorbed light is converted into heat [Citation22]. Selective hyperthermia gives rise to irreversible cell damage by inducing protein denaturation and cellular membrane destruction while it avoids damaging to healthy tissues. Initially, selective damaging of target cells by using 20–30 nm AuNP, radiated by 20 ns laser pulses, was described to achieve selective plasmonic photothermal therapy [Citation18,Citation23], but the photothermal efficiency of applied AuNP depends on their shape, size which are effective on photothermal conversion efficiency, degree of aggregation into the tumour cells, wavelength of the light that absorbed by AuNP, and employing high-intensity resonance pulses along with the major factor including intrinsic absorption of the nanoparticle [Citation24].
AuNPs used in photothermal therapy must display high photothermal conversion efficiency, which depends on AuNPs’ size and shape (structural dimensions). Determination of optimal size for penetration and retention of AuNPs based on the tumour characteristics, is absolutely essential. In studies conducted with various AuNP sizes (20 nm, 60 nm and 100 nm), it has been determined that larger sizes of AuNPs decrease their depth of penetration to the tumours. In addition, using smaller AuNPs is also non-efficient due to their rapid clearance from the extracellular environment [Citation24–27]. In addition to photothermal conversion efficiency, AuNPs used in photothermal therapy should also be designed to absorb light within the NIR light. Wavelengths of this light are within two regions of 650–850 nm and 950–1350 nm that can safely and deeply penetrate healthy tissue to reach AuNPs inside tumours. Interestingly, AuNPs structural dimensions can be effective on maximal absorption within one of these two regions of light spectrum. Most of AuNPs have been designed to maximally absorb within the light with 650–850 nm wavelengths, which can safely penetrate 2–3 cm of tissue. Recently, AuNP designs have emerged in the light with 950–1350 nm wavelengths, which can safely penetrate up to 10 cm [Citation28–30]. NPs made of various materials with different shape, size and absorption that can be employed for PTT (), for example, nanorods (NRs) and nanocages (NCs) with maximally absorb ∼800 nm light are most commonly used for PTT [Citation31,Citation32].
Figure 2. The most frequently employed AuNPs in photothermal therapy. Various types of AuNPs can be used in photothermal therapy. They have different sizes and shapes, and each one has advantages. Nanostars, nanocages, nanorods and nanoshell are the most common nanoparticles in photothermal therapy.
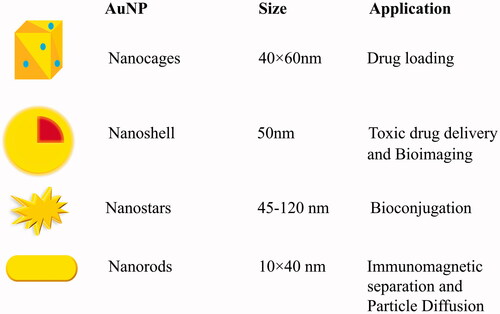
Another feature of AuNPs that should be considered is their ability to bioconjugation with biomolecules or drugs. AuNPs are suitable for bioconjugation because it enables simple gold-thiol bonding. Also one of the advantages of bioconjugation is its ability for combination therapy, bioconjugation has disadvantage as well. For example, they are susceptible to immune recognition or degradation [Citation33]. Various AuNPs with different shapes and sizes are suggested for bioconjugation (), such as NCs and nanostars. Nanostars offer a high surface-to-volume ratio for bioconjugation, and NC has the ability to load therapeutic agents in their own core. But this AuNP is destabilized under NIR irradiation that prevents their repeated use of PTT [Citation32]. To overcome this problem, researchers used NPs that were coated with a silica shell as NRs [Citation34].
One of the problems associated with using AuNPs is that when administered, they are coated with serum proteins which alter their biological identity. In effect, they are recognized by mononuclear phagocytic system leading to their rapid clearance from the blood flow [Citation35,Citation36]. To overcome the problem, AuNPs are coated with some of agents, such as polyethylene glycol (PEG) that leads to reduction of non-specific protein adsorption, extension of circulation time, and enhancement of biocompatibility [Citation37,Citation38]. In some experimental surveys and using tumour-burdened animals, laser irradiation was performed early after the injection of PEG-coated AuNP that resulted in the accumulation of the nanoparticle contrast and thus complete destruction of the tumour cells without destroying normal cells [Citation39]. NSs coated with PEG are mostly used among the different types of AuNPs used for PTT [Citation20,Citation21]. Another point is that irradiation of nanoparticles by high-intensity resonance pulses may destroy own particle that leads to reducing the efficacy of photothermal therapy with AuNP. A way for controlling this event is to maintain nanoparticles properties to achieve optimal irradiation goal [Citation40].
Imaging
In recent years, variety of imaging technology including, computed tomography, ultrasound and magnetic resonance have been developed, which are able to provide accurate information about diagnosis and therapy. AuNPs have unique physical, chemical and biological properties which make them an ideal candidate for imaging () [Citation41].
In bio-imaging field, on account of high electron density of AuNPs, which are now applied – aided by transmission electron microscopy and immune electron spectroscopy – assesses different bio-specific molecular interactions and thus studies the causative agents of infectious diseases by the detection of their specific membranous antigens [Citation11,Citation12]. In fact, the development of visualization methods with combination of AuNP and advanced microscopies has increased its popularity in medical and biological research.
For instance, an interesting subject that has recently attracted the attention of scientists is the ability of AuNP-antibody conjugation for penetration into living cells such as cancer cells, leading the comprehensive evaluation of cancer cells regarding biological behaviours, metastatic tendency and curative susceptibility [Citation42]. And also AuNPs are used as intracellular probes for cellular compartments such as mitochondria, endosomes and the cell nucleus by targeting them as well as to monitor intracellular drug release [Citation43]. This capability is indebted to the existence of two-photon luminescence of AuNPs that provide the possibility of visualizing specific markers on the surface of cancer cells labelled with fluorescence [Citation44]. This ability creates a tremendous transformation in bio-imaging of cancer cells to track these cells and their interactions with pharmaceutical particles [Citation45]. Previous studies have explored that conjugated AuNP could be used as a molecular probe for imaging to detect PSMA-expressing prostate cancer cells with high sensitivity and specificity [Citation46]. As well as, another study showed that conjugated AuNPs have potential for imaging of tumour xenograft in nude mice [Citation47,Citation48]. AuNP-based photoacoustic (PA) imaging is an emerging technology in biomedical imaging that is suited to detect various types of tumours. AuNP-based PA imaging has different applications including; cancer imaging, circulating tumour cell imaging, brain functional imaging, lymph node mapping and cancer cell metastasis imaging. Using AuNPs to image multiple types of cancer has been demonstrated; AuNS could be employed to detect human breast cancer tumour xenograft [Citation49]. Also, it was put into use to detect B16 melanomas [Citation50] as well as U87 brain tumours [Citation51]. Anti-HER2-conjugated AuNRs, silica-coated AuNRs and PEGylated AuNPrs were also successfully used in cancer imaging [Citation52,Citation53]. One of the applications of AuNP-based PA imaging is high sensitive detection of circulating tumour cells that leads to increasing overall patient survival. AuNSs have shown the capability of detecting circulating breast cancer cells [Citation54], melanoma cells, prostate cancer cells [Citation55] and oral squamous carcinoma cells [Citation56]. Lymph node mapping and cancer cell metastasis imaging are other applications of AuNP-based PA imaging and this technique is done using AuNRs as lymph node tracers [Citation57,Citation58].
Targeted drug delivery based on gold nanoparticle
Along with delivery strategy, conjugating nanoparticles with antibodies against tumour markers (such as tumour necrosis factor (TNF) or epidermal growth factor receptor) – as the active delivery method – has more reliability and efficiency [Citation59]. This technique is not only applied for destroying tumour cells, but also can be used for selective damaging of microbial agents such as bacteria or protozoa (). In conjunction with the application of AuNPs in cancer biology, these particles are now used for the detection of microbial cells and their behaviours [Citation59]. The fact of the matter is that labelling microbial cells and their metabolites using AuNP can provide qualitative assessment of these cells and their interactions of pharmaceutical nanoparticles [Citation60]. In this way, various nanoparticles with different shapes and sizes are put into use for this aim.
Recently, to increase the efficiency of conjugated nanoparticles, anti-tumour drugs were delivered in polyelectrolyte capsules on AuNP or were surrounded by polymer Nano gel that disintegrate under laser radiation; thus, delivering drugs to target cells can be maximized [Citation61,Citation62]. Furthermore, using photoactive agents are employed in the capsules to enhance intracellular penetration of the drugs [Citation63]. Moreover, to increase the probability of microbial damages, AuNPs are conjugated with photodynamic dyes [Citation44,Citation64]. In total, to achieve the highest efficiency for microbial or tumour damaging potential, enhancing stability and biocompatibility of conjugated nanoparticles with PEG, enzymes, hormones or dyes should be considered. One of the best clinical applications of AuNP is its use in targeted delivery of drugs. Conjugation of AuNP with antibiotics (for infectious targets) or antitumour agents (for tumour cells) is now applied as the best acceptable approach for delivering these agents to target tissues [Citation59,Citation65]. High efficacy of antitumour agents and also antibiotics conjugated with AuNPs has been clearly determined in recent researches [Citation66]. Undoubtedly, it should be noted that the affinity of different antibiotics and antitumour agents is different and thus the stability of this complex may be influenced by the type of conjugated agents [Citation67]. For instance, conjugation of AuNP with some antibiotics such as penicillin and some cephalosporin members is very unstable, and thus it should not be considered as the choice for creating drug delivery complex; but the efficacy of drug delivery complex containing selective antibiotics can be enhanced by conjugation with AuNP [Citation68]. As previously pointed out, for selecting delivery of AuNP into tumour cells, its conjugation with PEG (named passive delivery) or with antibodies or even hormones to certain tumour-related proteins (named active delivery) is considered as a delivery strategy [Citation69]. By using PEG, AuNP can be stabilized in blood circulation because of its special characteristics, including low lymphatic drainage and low exposing to immune cells [Citation70].
Immunological properties of nanoparticles
Another clinical benefit of AuNP refers to immunological properties of these particles. This immunological characteristic of AuNP is related to its colloidal and physicochemical nature especially in relation to its potential to induce production or inhibition of some antibodies [Citation71,Citation72].
In fact, the effect of colloidal AuNP on the induction of immune reactions such as increase in leukocytes counts and adversely decrease in mononuclear immune cells has been recently evaluated [Citation73]. Gold nanoparticle can be functionalized with molecules that modulate immune responses including humeral immunity, T-cell activation in cellular immunity and dendritic cell (DC) activation [Citation74,Citation75]. Previous studies have reported that macrophage stimulation with AuNP conjugated with specific peptides can induce the production of TNF, IL-1 and IL-6 [Citation76]. In addition, AuNP can be accumulated easily and preferentially in cells, tissues and organs of the immune system [Citation77–80], which results in the production of recent adjuvants applied in antitumour therapies and synthesis of the antiviral vaccines [Citation81]. In fact, conjugation of the AuNP with specific antigens can stimulate the production of antibodies by immune cells, leading to the destruction of pathogens [Citation82]. For instance, Paciotti et al. by intravenous delivery of 33 nm AuNP that conjugated with thiolated polyethylene glycol (PEG-SH) and recombinant human TNF in human prostate tumour-bearing mice, showed that conjugating TNF onto the AuNP could lead to the highest antitumour responses with lower doses of TNF and lessened off-site toxicity in comparison to TNF alone. Moon et al. described how delivery of nanoparticles mediated immunotherapies can improve therapeutic effect and reduce systemic toxicities [Citation83].
Melanoma and treatment approaches
Melanoma is a malignant neoplasm sourced from melanocytes skin cells that its incidence is considerably increasing on a global scale. This tumour has two radial and vertical growths in the epidermis and dermis, respectively, with the ability to distant metastasis in latter phase [Citation84]. Many genes have been discovered related to the existence and development of melanoma such as CDKN2A, CDK4, RB1, RAS and PTEN [Citation85,Citation86]. Paralleled with genetic basis, there are various risk factors involved in appearing malignant melanoma, including exposure to ultraviolet radiation, intense and intermittent blistering sunburns, some viruses and chemical agents, family history of melanoma, the presence of dysplastic nevi, and immunosuppressive conditions [Citation87,Citation88].
Two extremes have been identified therapeutically for melanoma: easily curable lesions through surgical resection with good prognosis on one side of the spectrum and limited treatment possibility because of distant metastasis with very poor prognosis on the other side of the spectrum [Citation89]. Some criteria indicating poor prognosis of these lesions are including a high level of invasion, high mitotic rate, the presence of bleeding or ulceration in the site of lesion, a high number of lymph nodes involved by development of primary lesion, and the presence of distant metastasis, older age, male gender and anatomical site [Citation89,Citation90].
Regarding treatment approaches, early-stage melanoma can be definitely cured by surgical resection [Citation91]. The mainstay of treatment includes surgical local excision with lymph node dissection. In late-stage melanoma with distant metastasis (stages III–IV), known as advanced melanoma, the treatment is focused on adjuvant therapy, or immunotherapy [Citation92]. For immunotherapy, inhibitor-based immunotherapy with some monoclonal antibodies targeting programmed cell death-1 (PD-1) is now considered [Citation93]. The use of adjuvant therapy is now a gold standard for treatment on a late-stage melanoma with distant metastasis, leading a high recurrence-free survival rate [Citation94]. Some of the most applicable adjuvants considered for this therapeutic approach include ipilimumab (a monoclonal antibody that activates the immune system), interferon alfa-2b and granulocyte-macrophage colony-stimulating factor (GM-CSF) [Citation95,Citation96]. In advanced-stage melanoma, chemotherapy with a combination of some chemical drugs (cisplatin, vinblastine, dacarbazine, carmustine, paclitaxel and tamoxifen) is the first selective option; however, because of latent nature of disease in this stage, the survival rate may be low, despite surgical and chemotherapy approaches [Citation89].
Targeted therapy using gold nanoparticles in melanoma
In earlier attempts to early diagnosis of melanoma, the use of AuNP was regarded as a great discovery. As shown by McCormack et al. [Citation97], the viability of AuNP for sensitivity in a photo acoustic detection system was assessed. The ability of AuNP in detecting circulating melanoma cells was tested. First, the melanoma cells were irradiated using Nd:YAG laser and then photo acoustic signals were detected by a special film from both AuNPs tagged melanoma cells and original ones. The study showed a higher rate of detected signals in AuNP tagged cells compared to unmodified cells. Later, Chen et al. [Citation98] in a study assessed the improvement of anticancer efficacy of epigallocatechin-3-gallate (the major bioactive constituent in green tea) conjugated with AuNP in animal models. This herbal agent is shown to inhibit the progression of the tumour. They could successfully show higher efficacy of epigallocatechin-3-gallate conjugated with AuNP as compared to unmodified epigallocatechin-3-gallate in regressing melanoma-cells. In their pathophysiological assessment, this higher cytotoxicity induced by conjugation with AuNP could be mediated by mitochondrial pathway-mediated apoptosis, leading inhibition of tumour growth.
Due to the low response rates to the common cytotoxic drugs in melanoma, nanotechnology is used to increase the effectiveness of anti-cancer treatments (). In this regard, Zhang et al. [Citation99] showed that the intratumoural injection of ultra-small AuNP conjugated with doxorubicin (Au-Dox) has long-lasting effects against melanoma in immunocompetent mice bearing murine B16 melanoma cells and in nude mice bearing human SK-MEL-28 xenograft. In another study, Tawagi et al. [Citation100] found that stable conjugates of Au-Dox might be effective for overcoming Dox resistance of apoptosis-resistant cancer cells, and that it may reduce toxicity to non-target organs, particularly the heart.
Figure 3. Different therapeutic approaches of gold nanoparticles for melanoma treatment. (a) Targeted delivery of several type of anticancer drugs or anticancer agents to tumour cells by gold nanoparticles. (b) Tumour directed drug delivery: increasing cell death by directed delivery of 5-aminolevulinic acid (5ALA)-conjugated gold nanoparticle. (c) Inhibition of melanoma tumour growth: melanoma tumour growth was inhibited by targeting epidermal growth factor receptor 2. (d) siRNA delivery for melanoma treatment: STAT3 siRNA using chitosan coated AuNP was delivered for increasing the killing rate of melanoma cells. siRNA can inhibit the overexpression of signal transducer and activator of transcription 3 (STAT3) protein, leading enhancement of apoptosis of melanoma cells.
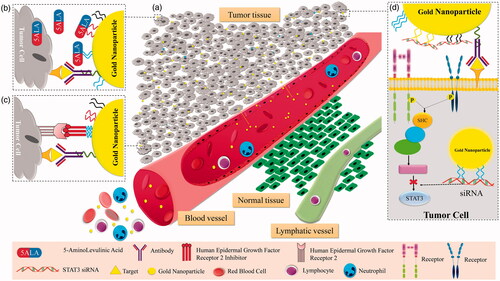
Li et al. [Citation101], developed a Pluronic® F127-based thermosensitive hydrogel (Au-DOX-Gel) loading AuNPs and DOX to improve cancer chemoradiotherapy. The hydrogel showed sustained release of AuNPs and DOX. A substantial reduction in tumour size and inhibition of tumour cell growth and proliferation was seen in melanoma B16 bearing mice by AuNPs combined with DOX under radiation. This study suggests that the Au-DOX-Gel could represent a promising strategy for effective cancer chemoradiotherapy.
As pointed out previously, delivery of adjuvants to target cells affected by melanoma is the main approach for treating melanoma. In this regard, Labala et al. [Citation102] examined the potential of layer-by-layer polymer – coating with AuNP for local delivery of a new adjuvant agent (imatinib) – to target malignant cells. The study could show significant increase in skin penetration of the layer-by-layer polymer coated AuNP compared to passive application.
Non-thermal atmospheric-pressure plasma (NTAPP), also called cold plasma, is defined as a partially ionized gas with electrically charged particles at atmospheric pressure. It has anti-tumoural potential that is used in the treatment of cancer diseases. Choi et al. [Citation103] assessed the targeting NEU Protein (human epidermal growth factor receptor 2: a protein that is overexpressed in several cancer cells) in melanoma cells with coating AuNP (). In this method, cold plasma was applied specifically for killing cancer cells. Having labelled anti-NEU antibodies with AuNP and delivered to melanoma cells, these cells were exposed to cold plasma which led to higher cancer cell death compared to anti-NEU antibodies free of AuNP.
In another study, it has been demonstrated that AuNPs conjugated with antibodies targeting phosphorylated FAK (p-FAK-AuNP) have potential to decrease the viability of G361 melanoma cells in a time dependent manner by inducing apoptosis without affecting normal cells. So as to maximize the lethality of p-FAK-AuNP, NTAPP was employed. It could stimulate the AuNPs within p-FAK-AuNP which inhibits FAK protein activities and consequently p-FAK-AuNP results in cells’ death. It has shown combined treatment with cold plasma and p-FAK-AuNP gives rise to selective lethality against human melanoma cells [Citation104].
As previously mentioned, AuNPs are employed for in vivo PTT owing to the fact that they have strong absorption in the near infra-red regions of the electromagnetic spectrum and they generate a localized heat so as to destroy a region of interest [Citation105]. Heidari et al. [Citation106] have synthesized and characterized a gold-ferrite nanocomposite (GFNC) as a single composite nanomaterial that can be used in various therapeutic modalities. They have demonstrated GFNC enabled to destroy B16F0 melanoma-tumour-bearing mice upon NIR irradiation (via an 808-nm diode laser) without additional organic photosensitizers. Wang et al. [Citation107] developed gold nanoshell capsules, that are efficiently taken up by melanoma cells and it leads to an effective ablation of malignant melanomas after a mild laser irradiation. In several studies, AuNPs are developed as carriers of hydrophobic phthalocyanines, which serve as an example of second-generation photosensitizers [Citation108–110]. Camerin et al. [Citation111] have described the pharmacokinetic behaviour and PDT (photodynamic therapy) treatment efficacy of Zn [II]-phthalocyanine disulphide (C11Pc)–AuNP conjugates in a mouse model of xenograft melanoma. It was revealed that C11Pc–AuNP conjugates had greater accumulation within a subcutaneously implanted amelanotic melanoma than free C11Pc. Photodynamic therapy and electron microscopy studies revealed that the AuNP-conjugated C11Pc could result in the reduction of tumour growth and destruction of the tumour vasculature, respectively. In another study by Camerin et al. [Citation112], hydrophobic phthalocyanine photosensitizer was conjugated with PEGylated AuNP. This photosensitizer is commonly applied to treatment of melanoma with reactive oxygen species on photodynamic technique. In their assay, hydrophobic phthalocyanine photosensitizers coated with nanoparticles were injected intravenously in animal models with melanoma, indicating high retention of the AuNP conjugates in the tumour cells which led to destruction of melanoma cells and complete survival in at least 40% of affected models.
Labala et al. [Citation113] designed another study in 2016 to assess the efficiency of delivering STAT3 siRNA using chitosan coated AuNP for increasing the killing rate of melanoma cells (). As an explanation, siRNA can inhibit the overexpression of signal transducer and activator of transcription 3 (STAT3) protein, leading to enhancement of the apoptosis of melanoma-cells. In fact, chitosan coated AuNP was applied to deliver STAT3 siRNA to targeted cells, leading higher inhibition of the melanocytes growth, as compared to deliver it without labelling antibodies with AuNP.
The peak of studies on the benefits of AuNP in delivering inhibitors in melanoma cells refers to 2017. In a study by Mohammadi et al. [Citation114], the efficacy of photosensitizing effects of 5-aminolevulinic acid (5ALA)-conjugated AuNP was assessed (). The study demonstrated higher tumour cell death when 5ALA-conjugated AuNP was conducted compared to that of 5ALA in original format. Another similar experiment was achieved by Mousavi et al. [Citation115], when radio sensitivity of melanoma cells was enhanced by conjugation with PEGylated AuNP. In their observation, by increasing PEGylated AuNP, the survival fraction rate of the tumour cells significantly decreased, indicating high radiosensitization effects of these nanoparticles. Kim and Kim [Citation116] assessed the efficacy of AuNP to enhance kilo voltage X-ray therapy of melanoma. In their study, treatment using AuNP could elicit dose enhancement effect on melanoma cells exposed to kilo voltage X-rays which led to a high melanoma-cell death. Lastly, the effectiveness of AuNP for conjugating STAT3 siRNA and imatinib as the inhibitors of melanoma cells progression was assessed in recent years; where Labala et al. [Citation117] assessed co-delivery of these two inhibitors by using AuNP. In their study, the combination treatment with STAT3 siRNA and imatinib conjugated with AuNP – led to greater suppression of STAT3 protein and thus higher apoptotic effects of those chemotherapeutic agents compared to using each of agents with and without AuNP. A number of other studies have been conducted and the results of which on the use of nanoparticles in the treatment of melanoma are listed in .
Table 1. A brief review of the studies and main endpoints.
Conclusions
Nanotechnology has the potential to remarkably improve both the diagnosis and treatment of melanoma. AuNPs have various characteristics that make them attractive to be used in cancer therapy. The combination of high surface area, biologically relevant size, low inherent toxicity, enhanced permeability and retention (EPR) effect and ability to easily functionalize with biomolecules, provides them with unique attributes which serves as an empowering tool with effective applications in delivery strategies, imaging and noninvasive disease diagnostics. As highlighted in this review, AuNPs-based drug delivery systems that selectively deliver drugs to melanoma cells have the potential to improve drug delivery. Further investigations are needed to focus on evaluation of in vivo and in vitro targeting efficiency so as to maximize AuNP therapeutic properties in melanoma and other cancers. Also, more detailed studies are required on long-term cytotoxicity to decrease the side effects of these AuNPs in healthy tissues.
Disclosure statement
The authors have no conflicts of interest to disclose.
References
- Galib MB, Mashru M, Jagtap C, et al. Therapeutic potentials of metals in ancient India: a review through Charaka Samhita. J Ayurveda Integr Med. 2011;2:55.
- Kean W, Kean I. Clinical pharmacology of gold. Inflammopharma-cology. 2008;16:112–125.
- Anthony F. Panacea aurea: sive tractatus duo de ipsius auro potabili. Ex Bibliopolio Frobeniano.Hamburgi: ex Bibliopolio Frobeniano; 1618.
- Rao CR, Kulkarni GU, Thomas PJ, et al. Metal nanoparticles and their assemblies. Chem Soc Rev. 2000;29:27–35.
- Peruski AH, Peruski LF. Immunological methods for detection and identification of infectious disease and biological warfare agents. Clin Diagn Lab Immunol. 2003;10:506–513.
- Aryal S, Bisht G. New paradigm for a targeted cancer therapeutic approach: a short review on potential synergy of gold nanoparticles and cold atmospheric plasma. Biomedicines. 2017;5:38.
- Gharatape A, Salehi R. Recent progress in theranostic applications of hybrid gold nanoparticles. Eur J Med Chem. 2017;138:221.
- Carabineiro SAC. Applications of gold nanoparticles in nanomedicine: recent advances in vaccines. Molecules. 2017;22:857.
- Saini M, Masirkar Y, Varshney R, et al. Fluorogen-free aggregation induced NIR emission from gold nanoparticles. Chem Commun (Camb). 2017;53:6199–6202.
- Tao W, Ziemer KS, Gill HS. Gold nanoparticle–M2e conjugate coformulated with CpG induces protective immunity against influenza A virus. Nanomedicine. 2014;9:237–251.
- Takemura K, Adegoke O, Takahashi N, et al. Versatility of a localized surface plasmon resonance-based gold nanoparticle-alloyed quantum dot nanobiosensor for immunofluorescence detection of viruses. Biosens Bioelectron. 2017;89:998–1005.
- Bolhassani A, Javanzad S, Saleh T, et al. Polymeric nanoparticles: potent vectors for vaccine delivery targeting cancer and infectious diseases. Hum Vac Immunother. 2014;10:321–332.
- Gao J, Huang X, Liu H, et al. Colloidal stability of gold nanoparticles modified with thiol compounds: bioconjugation and application in cancer cell imaging. Langmuir. 2012;28:4464–4471.
- Dykman L, Khlebtsov N. Gold nanoparticles in biomedical applications: recent advances and perspectives. Chem Soc Rev. 2012;41:2256–2282.
- Pissuwan D, Niidome T, Cortie MB. The forthcoming applications of gold nanoparticles in drug and gene delivery systems. J Control Release. 2011;149:65–71.
- Maverakis E, Cornelius LA, Bowen GM, et al. Metastatic melanoma – a review of current and future treatment options. Acta Derm Venereol. 2015;95:516–527.
- Eyvazzadeh N, Shakeri-Zadeh A, Fekrazad R, et al. Gold-coated magnetic nanoparticle as a nanotheranostic agent for magnetic resonance imaging and photothermal therapy of cancer. Lasers Med Sci. 2017;32:1469–1477.
- Kennedy LC, Bickford LR, Lewinski NA, et al. A new era for cancer treatment: gold‐nanoparticle‐mediated thermal therapies. Small. 2011;7:169–183.
- Day ES, Zhang L, Thompson PA, et al. Vascular-targeted photothermal therapy of an orthotopic murine glioma model. Nanomedicine. 2012;7:1133–1148.
- Biosciences IN. Pilot study of AuroLase (tm) therapy in refractory and/or recurrent tumors of the head and neck. ClinicalTrials gov [Internet]. Bethesda (MD): National Library of Medicine (US); 2000.
- Biosciences N. Inc. Efficacy study of AuroLase therapy in subjects with primary and/or metastatic lung tumors. ClinicalTrials gov [Internet]. Bethesda (MD): National Library of Medicine; 2000.
- Norregaard K, Jørgensen JT, Simón M, et al. 18F-FDG PET/CT-based early treatment response evaluation of nanoparticle-assisted photothermal cancer therapy. PLoS One. 2017;12:e0177997.
- Huang X, Jain PK, El-Sayed IH, et al. Plasmonic photothermal therapy (PPTT) using gold nanoparticles. Lasers Med Sci. 2008;23:217.
- Perrault SD, Walkey C, Jennings T, et al. Mediating tumor targeting efficiency of nanoparticles through design. Nano Lett. 2009;9:1909–1915.
- Prabhakar U, Maeda H, Jain RK, et al. Challenges and key considerations of the enhanced permeability and retention effect for nanomedicine drug delivery in oncology. AACR. 2013;73:2412?2417.
- Raeesi V, Chan WC. Improving nanoparticle diffusion through tumor collagen matrix by photo-thermal gold nanorods. Nanoscale. 2016;8:12524–12530.
- Park J-H, von Maltzahn G, Xu MJ, et al. Cooperative nanomaterial system to sensitize, target, and treat tumors. Proc Natl Acad Sci. 2010;107:981–986.
- Huang X, El-Sayed IH, Qian W, et al. Cancer cell imaging and photothermal therapy in the near-infrared region by using gold nanorods. J Am Chem Soc. 2006;128:2115–2120.
- Mackey MA, Ali MR, Austin LA, et al. The most effective gold nanorod size for plasmonic photothermal therapy: theory and in vitro experiments. J Phys Chem B. 2014;118:1319–1326.
- Wang S, Xu H, Ye J. Plasmonic rod-in-shell nanoparticles for photothermal therapy. Phys Chem Chem Phys. 2014;16:12275–12281.
- Tchounwou C, Sinha SS, Viraka Nellore BP, et al. Hybrid theranostic platform for second near-IR window light triggered selective two-photon imaging and photothermal killing of targeted melanoma cells. ACS Appl Mater Interfaces. 2015;7:20649–20656.
- Stone J, Jackson S, Wright D. Biological applications of gold nanorods. Wires Nanomed Nanobiotechnol. 2011;3:100–109.
- Chen H, Zhang X, Dai S, et al. Multifunctional gold nanostar conjugates for tumor imaging and combined photothermal and chemo-therapy. Theranostics. 2013;3:633.
- Kim S, Chen Y-S, Luke G, et al. In-vivo ultrasound and photoacoustic image-guided photothermal cancer therapy using silica-coated gold nanorods. IEEE Trans Ultrason Ferroelectr Freq Control. 2014;61:891–897.
- Alkilany AM, Murphy CJ. Toxicity and cellular uptake of gold nanoparticles: what we have learned so far? J Nanopart Res. 2010;12:2313–2333.
- Monopoli MP, Åberg C, Salvati A, et al. Biomolecular coronas provide the biological identity of nanosized materials. Nat Nanotechnol. 2012;7:779–786.
- Dai Q, Walkey C, Chan WC. Polyethylene glycol backfilling mitigates the negative impact of the protein corona on nanoparticle cell targeting. Angew Chem Int Ed. 2014;53:5093–5096.
- Walkey CD, Chan WC. Understanding and controlling the interaction of nanomaterials with proteins in a physiological environment. Chem Soc Rev. 2012;41:2780–2799.
- Wang M, Thanou M. Targeting nanoparticles to cancer. Pharmacol Res. 2010;62:90–99.
- Wei R, Xi W, Wang H, et al. In situ crystal growth of gold nanocrystals on upconversion nanoparticles for synergetic chemo-photothermal therapy. Nanoscale. 2017;9:12885–12896.
- Jing L, Liang X, Deng Z, et al. Prussian blue coated gold nanoparticles for simultaneous photoacoustic/CT bimodal imaging and photothermal ablation of cancer. Biomaterials. 2014;35:5814–5821.
- Mustafaoglu N, Kiziltepe T, Bilgicer B. Site-specific conjugation of antibody on gold nanoparticle surface for one-step diagnosis of prostate specific antigen with dynamic light scattering. Nanoscale. 2017;9:8684?8694.
- Huefner A, Septiadi D, Wilts BD, et al. Gold nanoparticles explore cells: cellular uptake and their use as intracellular probes. Methods. 2014;68:354–363.
- El-Hussein A, Mfouo-Tynga I, Abdel-Harith M, et al. Comparative study between the photodynamic ability of gold and silver nanoparticles in mediating cell death in breast and lung cancer cell lines. J Photochem Photobiol B: Biol. 2015;153:67–75.
- Ai J, Xu Y, Lou B, et al. Multifunctional AS1411-functionalized fluorescent gold nanoparticles for targeted cancer cell imaging and efficient photodynamic therapy. Talanta. 2014;118:54–60.
- Kim D, Jeong YY, Jon S. A drug-loaded aptamer–gold nanoparticle bioconjugate for combined CT imaging and therapy of prostate cancer. ACS Nano. 2010;4:3689–3696.
- Wang H, Zheng L, Peng C, et al. Computed tomography imaging of cancer cells using acetylated dendrimer-entrapped gold nanoparticles. Biomaterials. 2011;32:2979–2988.
- Peng C, Zheng L, Chen Q, et al. PEGylated dendrimer-entrapped gold nanoparticles for in vivo blood pool and tumor imaging by computed tomography. Biomaterials. 2012;33:1107–1119.
- Zhang Q, Iwakuma N, Sharma P, et al. Gold nanoparticles as a contrast agent for in vivo tumor imaging with photoacoustic tomography. Nanotechnology. 2009;20:395102.
- Kim C, Cho EC, Chen J, et al. In vivo molecular photoacoustic tomography of melanomas targeted by bioconjugated gold nanocages. ACS Nano. 2010;4:4559–4564.
- Zhang YS, Wang Y, Wang L, et al. Labeling human mesenchymal stem cells with gold nanocages for in vitro and in vivo tracking by two-photon microscopy and photoacoustic microscopy. Theranostics. 2013;3:532.
- Kim S, Chen Y-S, Luke GP, et al. In vivo three-dimensional spectroscopic photoacoustic imaging for monitoring nanoparticle delivery. Biomed Opt Express. 2011;2:2540–2550.
- Bao C, Beziere N, del Pino P, et al. Gold nanoprisms as optoacoustic signal nanoamplifiers for in vivo bioimaging of gastrointestinal cancers. Small. 2013;9:68–74.
- Thomas T, Dale P, Weight R, et al. Photoacoustic detection of breast cancer cells in human blood. Proc SPIE Int Soc Opt Eng. 2008;1:2.
- Viator JA, Gupta S, Goldschmidt BS, et al. Gold nanoparticle mediated detection of prostate cancer cells using photoacoustic flowmetry with optical reflectance. J Biomed Nanotechnol. 2010;6:187–191.
- Li P-C, Wang C-RC, Shieh D-B, et al. In vivo photoacoustic molecular imaging with simultaneous multiple selective targeting using antibody-conjugated gold nanorods. Opt Express. 2008;16:18605–18615.
- Song KH, Kim C, Cobley CM, et al. Near-infrared gold nanocages as a new class of tracers for photoacoustic sentinel lymph node mapping on a rat model. Nano Lett. 2008;9:183–188.
- Song KH, Kim C, Maslov K, et al. Noninvasive in vivo spectroscopic nanorod-contrast photoacoustic mapping of sentinel lymph nodes. Eur J Radiol. 2009;70:227–231.
- Penders J, Stolzoff M, Hickey DJ, et al. shape-dependent antibacterial effects of non-cytotoxic gold nanoparticles. IJN. 2017;12:2457.
- Khan AU, Yuan Q, Wei Y, et al. Photocatalytic and antibacterial response of biosynthesized gold nanoparticles. J Photochem Photobiol B: Biol. 2016;162:273–277.
- Ke´kicheff P, Schneider GF, Decher G. Size-controlled polyelectrolyte complexes: direct measurement of the balance of forces involved in the triggered collapse of layer-by-layer assembled nanocapsules. Langmuir. 2013;29:10713–10726.
- Oaew S, Charlermroj R, Pattarakankul T, et al. Gold nanoparticles/horseradish peroxidase encapsulated polyelectrolyte nanocapsule for signal amplification in Listeria monocytogenes detection. Biosens Bioelectron. 2012;34:238–243.
- Mäsing F, Mardyukov A, Doerenkamp C, et al. Controlled light-mediated preparation of gold nanoparticles by a Norrish Type I reaction of photoactive polymers. Angew Chem Int Ed Engl. 2015;54:12612–12617.
- Chen R-J, Chen P-C, Prasannan A, et al. Formation of gold decorated porphyrin nanoparticles and evaluation of their photothermal and photodynamic activity. Mater Sci Eng: C. 2016;63:678–685.
- Kim K, Oh KS, Park DY, et al. Doxorubicin/gold-loaded core/shell nanoparticles for combination therapy to treat cancer through the enhanced tumor targeting. J Control Release. 2016;228:141–149.
- Bagga P, Siddiqui H, Akhtar J, et al. Gold nanoparticles conjugated levofloxacin: for improved antibacterial activity over levofloxacin alone. Curr Drug Deliv. 2017;14:1114–1119.
- Li J, Li Q, Ma X, et al. Biosynthesis of gold nanoparticles by the extreme bacterium Deinococcus radiodurans and an evaluation of their antibacterial properties. IJN. 2016;11:5931.
- Jakubec P, Urbanová V, Medříková Z, et al. Advanced sensing of antibiotics with magnetic gold nanocomposite: electrochemical detection of chloramphenicol. Chem Eur J. 2016;22:14279–14284.
- Kannan R, Zambre A, Chanda N, et al. Functionalized radioactive gold nanoparticles in tumor therapy. Wires Nanomed Nanobiotechnol. 2012;4:42–51.
- Gargioni E, Schulz F, Raabe A, et al. Targeted nanoparticles for tumour radiotherapy enhancement—the long dawn of a golden era? Ann Transl Med. 2016;4:523.
- Golchin K, Golchin J, Ghaderi S, et al. Gold nanoparticles applications: from artificial enzyme till drug delivery. Artif Cells Nanomed Biotechnol. 2017;1–5. doi: https://doi.org/10.1080/21691401.2017.1305393 [Epub ahead of print]
- Vetro M, Safari D, Fallarini S, et al. Preparation and immunogenicity of gold glyco-nanoparticles as antipneumococcal vaccine model. Nanomedicine. 2017;12:13–23.
- Devanabanda M, Latheef SA, Madduri R. Immunotoxic effects of gold and silver nanoparticles: inhibition of mitogen-induced proliferative responses and viability of human and murine lymphocytes in vitro. J Immunotoxicol. 2016;13:897–902.
- Cruz LJ, Tacken PJ, Rueda F, et al. 8 targeting nanoparticles to dendritic cells for immunotherapy. Methods Enzymol. 2012;509:143.
- Fallarini S, Paoletti T, Battaglini CO, et al. Factors affecting T cell responses induced by fully synthetic glyco-gold-nanoparticles. Nanoscale. 2013;5:390–400.
- Bastús NG, Sánchez-Tilló E, Pujals S, et al. Peptides conjugated to gold nanoparticles induce macrophage activation. Mol Immunol. 2009;46:743–748.
- Jewell CM, López SCB, Irvine DJ. In situ engineering of the lymph node microenvironment via intranodal injection of adjuvant-releasing polymer particles. Proc Natl Acad Sci. 2011;108:15745–15750.
- Khlebtsov N, Dykman L. Biodistribution and toxicity of engineered gold nanoparticles: a review of in vitro and in vivo studies. Chem Soc Rev. 2011;40:1647–1671.
- Almeida JPM, Chen AL, Foster A, et al. In vivo biodistribution of nanoparticles. Nanomedicine (Lond). 2011;6:815–835.
- Alexis F, Pridgen E, Molnar LK, et al. Factors affecting the clearance and biodistribution of polymeric nanoparticles. Mol Pharm. 2008;5:505–515.
- Paul AM, Shi Y, Acharya D, et al. Delivery of antiviral small interfering RNA with gold nanoparticles inhibits dengue virus infection in vitro. J Gen Virol. 2014;95:1712–1722.
- Ahiwale S, Bankar A, Tagunde S, et al. A bacteriophage mediated gold nanoparticles synthesis and their anti-biofilm activity. Indian J Microbiol. 2017;57:1–7.
- Moon JJ, Huang B, Irvine DJ. Engineering nano- and microparticles to tune immunity. Adv Mater Weinheim. 2012;24:3724–3746.
- Bastian BC. The molecular pathology of melanoma: an integrated taxonomy of melanocytic neoplasia. Annu Rev Pathol Mech Dis. 2014;9:239–271.
- Curtin JA, Fridlyand J, Kageshita T, et al. Distinct sets of genetic alterations in melanoma. N Engl J Med. 2005;353:2135–2147.
- Viros A, Fridlyand J, Bauer J, et al. Improving melanoma classification by integrating genetic and morphologic features. PLoS Med. 2008;5:e120.
- Markovic SN, Erickson LA, Rao RD, et al., editors. Malignant melanoma in the 21st century. Part 1: epidemiology, risk factors, screening, prevention, and diagnosis. Mayo Clin Proc. 2007;82:364–380.
- Rastrelli M, Tropea S, Rossi CR, et al. Melanoma: epidemiology, risk factors, pathogenesis, diagnosis and classification. In Vivo. 2014;28:1005–1011.
- Sandru A, Voinea S, Panaitescu E, et al. Survival rates of patients with metastatic malignant melanoma. J Med Life. 2014;7:572.
- Balch CM, Soong SJ, Atkins MB, et al. An evidence‐based staging system for cutaneous melanoma. CA: Cancer J Clin. 2004;54:131–149.
- Sharif S, Haque AU, Ahmed A. Blue melanoma simulating blue nevus. Int. j. pathol. 2013;11:73–77.
- Trinh VA, Zobniw C, Hwu W-J. The efficacy and safety of adjuvant interferon-alfa therapy in the evolving treatment landscape for resected high-risk melanoma. Expert Opin Drug Saf. 2017;16:933–940.
- Hino R, Kabashima K, Kato Y, et al. Tumor cell expression of programmed cell death-1 ligand 1 is a prognostic factor for malignant melanoma. Cancer. 2010;116:1757–1766.
- Gonzalez RJ, Kudchadkar R, Rao NG, et al. Adjuvant immunotherapy and radiation in the management of high-risk resected melanoma. Ochsner J. 2010;10:108–116.
- Sondak VK, Gonzalez RJ, Kudchadkar R. Adjuvant therapy for melanoma: a surgical perspective. Surg Oncol Clin N Am. 2011;20:105–114.
- Spitler LE, Grossbard ML, Ernstoff MS, et al. Adjuvant therapy of stage III and IV malignant melanoma using granulocyte-macrophage colony-stimulating factor. JCO. 2000;18:1614–1621.
- McCormack DR, Bhattacharyya K, Kannan R, et al. Enhanced photoacoustic detection of melanoma cells using gold nanoparticles. Lasers Surg Med. 2011;43:333–338.
- Chen C-C, Hsieh D-S, Huang K-J, et al. Improving anticancer efficacy of (–)-epigallocatechin-3-gallate gold nanoparticles in murine B16F10 melanoma cells. Drug Des Dev Ther. 2014;8:459.
- Zhang X, Teodoro JG, Nadeau JL. Intratumoral gold-doxorubicin is effective in treating melanoma in mice. Nanomed: Nanotechnol Biol Med. 2015;11:1365–1375.
- Tawagi E, Massmann C, Chibli H, et al. Differential toxicity of gold-doxorubicin in cancer cells vs. cardiomyocytes as measured by real-time growth assays and fluorescence lifetime imaging microscopy (FLIM). Analyst. 2015;140:5732–5741.
- Li T, Zhang M, Wang J, et al. Thermosensitive hydrogel co-loaded with gold nanoparticles and doxorubicin for effective chemoradiotherapy. AAPS J. 2016;18:146–155.
- Labala S, Mandapalli PK, Kurumaddali A, et al. Layer-by-layer polymer coated gold nanoparticles for topical delivery of imatinib mesylate to treat melanoma. Mol Pharm. 2015;12:878–888.
- Choi BB, Kim MS, Song KW, et al. Targeting NEU protein in melanoma cells with non-thermal atmospheric pressure plasma and gold nanoparticles. J Biomed Nanotechnol. 2015;11:900–905.
- Choi BBR, Choi JH, Hong JW, et al. Selective killing of melanoma cells with non-thermal atmospheric pressure plasma and p-FAK antibody conjugated gold nanoparticles. Int J Med Sci. 2017;14:1101.
- Lloyd-Hughes H, Shiatis AE, Pabari A, et al. Current and future nanotechnology applications in the management of melanoma: a review. J Nanomed Nanotechnol. 2015;6:1.
- Heidari M, Sattarahmady N, Azarpira N, et al. Photothermal cancer therapy by gold-ferrite nanocomposite and near-infrared laser in animal model. Lasers Med Sci. 2016;31:221–227.
- Wang H, Zhao R, Li Y, et al. Aspect ratios of gold nanoshell capsules mediated melanoma ablation by synergistic photothermal therapy and chemotherapy. Nanomed: Nanotechnol Biol Med. 2016;12:439–448.
- Olson F, Hunt C, Szoka F, et al. Preparation of liposomes of defined size distribution by extrusion through polycarbonate membranes. Biochim Biophys Acta (BBA)-Biomembr. 1979;557:9–23.
- Sawant RR, Torchilin VP. Challenges in development of targeted liposomal therapeutics. AAPS J. 2012;14:303–315.
- Al‐Jamal WT, Al‐Jamal KT, Bomans PH, et al. Functionalized‐quantum‐dot–liposome hybrids as multimodal nanoparticles for cancer. Small. 2008;4:1406–1415.
- Camerin M, Magaraggia M, Soncin M, et al. The in vivo efficacy of phthalocyanine?nanoparticle conjugates for the photodynamic therapy of amelanotic melanoma. Eur J Cancer. 2010;46:1910–1918.
- Camerin M, Moreno M, Marín MJ, et al. Delivery of a hydrophobic phthalocyanine photosensitizer using PEGylated gold nanoparticle conjugates for the in vivo photodynamic therapy of amelanotic melanoma. Photochem Photobiol Sci. 2016;15:618–625.
- Labala S, Jose A, Venuganti VVK. Transcutaneous iontophoretic delivery of STAT3 siRNA using layer-by-layer chitosan coated gold nanoparticles to treat melanoma. Colloids Surf B: Biointerfaces. 2016;146:188–197.
- Mohammadi Z, Sazgarnia A, Rajabi O, et al. Comparative study of X-ray treatment and photodynamic therapy by using 5-aminolevulinic acid conjugated gold nanoparticles in a melanoma cell line. Artif Cells Nanomed Biotechnol. 2017;45:467–473.
- Mousavi M, Nedaei HA, Khoei S, et al. Enhancement of radiosensitivity of melanoma cells by pegylated gold nanoparticles under irradiation of megavoltage electrons. Int J Radiat Biol. 2017;93:214–221.
- Kim S-R, Kim E-H. Gold nanoparticles as dose-enhancement agent for kilovoltage X-ray therapy of melanoma. Int J Radiat Biol. 2017;93:517–526.
- Labala S, Jose A, Chawla SR, et al. Effective melanoma cancer suppression by iontophoretic co-delivery of STAT3 siRNA and imatinib using gold nanoparticles. Int J Pharm. 2017;525:407–417.