Abstract
Hypoxia drives sickle cell disease (SCD) by inducing sickle cell haemoglobin to polymerize and deform red blood cells (RBC) into the sickle shape. A novel carboxyhaemoglobin-based oxygen carrier (PEG-COHb; PP-007) promotes unsickling in vitro by relieving RBC hypoxia. An in vivo rat model of vaso-occlusive crisis (VOC) capable of accommodating a suite of physiological and microcirculatory measurements was used to compare treatment with PEG-COHb to a non-oxygen carrying control solution (lactated ringer’s [LRS]). Male Sprague-Dawley rats were anesthetized and surgically prepared to monitor microvascular interstitial oxygenation (PISFO2), cardiovascular parameters and blood chemistry. Human homozygous SCD RBCs were isolated and exchange transfused into the rats until the distal microcirculation of the exteriorized spinotrapezius muscle was hypoxic and RBC aggregates were visualized. VOC was left untreated (Sham) or treated 15 min later with PEG-COHb or LRS and observed for up to 4 h. Treatment with PEG-COHb showed better improvement of PISFO2, end-point lactate, mean arterial pressure and survival duration compared to Sham and LRS. Restoring PISFO2 was associated with relieving the RBC aggregates driving VOC, which then affected other study metrics. Compared to LRS, PEG-COHb’s oxygen-carrying properties were key to improved outcomes.
Introduction
Sickle cell disease (SCD) arises from red blood cells (RBCs) expressing haemoglobin S (HbS), which, upon conversion to deoxyhaemoglobin, undergoes polymerization and precipitation resulting in sickle-shaped, rigid and brittle RBCs [Citation1,Citation2]. This decreased deformability results in systemic anaemia due to a shortened circulatory half-life (∼10 versus 120 d for normal RBCs [Citation3]) and localized ischaemia from reduced microvascular perfusion [Citation4,Citation5]. The most severe form of SCD is the homozygous haemoglobin S (HbSS) variant where both genes encode for adult haemoglobin S causing the greatest occurrences of both acute and chronic episodes of vaso-occlusive crisis (VOC) [Citation6].
VOC is a painful complication of SCD that arises from a seemingly simple concept of vascular obstruction yet has a complex pathology [Citation7,Citation8]. Briefly, it is the product of sickled RBCs – formed during conditions of hypoxia [Citation9], acidosis and/or dehydration [Citation10] – interacting with the vascular endothelium and plasma constituents [Citation11] to form aggregates that obstruct blood flow. Microvascular perfusion is initially compromised as the small capillary beds where deformable RBCs usually traverse single-file suffer from prolonged RBC transit times. Next, the growing population of sickled RBCs interact with and begin adhering to the vascular endothelium of post-capillary venules in a process involving leukocytes (reviewed here [Citation12]) leading to erythrocyte aggregate formation that further obstructs blood-flow. Microvascular ischaemia leads to additional tissue hypoxia and acidosis, which compounds the rate of sickle cell formation and aggregation. VOC can progress into macroscopic maladies, such as stroke [Citation13], acute coronary syndrome [Citation14], leg ulcers, renal insufficiency, pain syndromes [Citation15] and increased mortality [Citation16,Citation17].
Despite considerable mechanistic knowledge of VOC, treatment remains largely palliative [Citation18]. Acute crises are typically treated for symptom presentation with rest, hydration, non-steroidal anti-inflammatory drugs and opioid analgesics [Citation15], while more severe cases can receive more disease-targeted prophylactic regimens of hydroxyurea (to increase foetal haemoglobin production and dilute the impact of HbS to reduce sickling [Citation11]), and acute treatments of oxygen therapy [Citation19], crystalloids and blood transfusions [Citation20,Citation21]. Crystalloid infusions (i.e. normal saline and lactated ringer’s solution [LRS]) are intended to help relieve vaso-occlusive aggregates by a combination of hydration to prevent additional RBC sickling and increasing plasma volume to relieve hypotension, however, without combination therapy with concentrated RBCs or exchange transfusion (ET) with whole blood, there is a risk of haemodilution and a concomitant decrease in oxygen transport in already anaemic patients [Citation22]. Hyperbaric oxygen is helpful in a narrow set of circumstances in which patients present with a low oxygen saturation. It has not shown an appreciable benefit for general VOC pain management [Citation23,Citation24].
Studying acute therapeutic interventions of VOC may be best approached from a microvascular perspective. We developed a testable VOC rat model via ET of human HbSS RBCs that approximates the clinical manifestations of VOC and allows for a robust battery of microvascular and systemic measurements. In this study, we compare intravascular infusion of LRS to PP-007, which is a novel PEGylated carboxyhaemoglobin-based (PEG-COHb) gas-transfer agent. It has been tested in multiple animal models of acute ischaemia [Citation25,Citation26], and has completed phase I and phase II clinical trials [Citation27,Citation28]. It functions as both a volume expander without evidence of vascular constriction [Citation26,Citation29] and an oxygen delivery vehicle, creating a powerful combination therapy of oxygenation and hydration.
Methods and materials
Human blood donation
HbSS RBCs were sourced from the whole blood donations of consented patients ( for clinical characteristics) by a biological supply company (BioreclamationIVT, Westbury, NY), and shipped to Song Biotechnologies, LLC for use within 24 h of collection. The blood, stored in heparinized tubes, was centrifuged to separate the buffy coat and plasma from the RBCs, both of which were then discarded. The remaining RBCs were triple washed in LRS and resuspended in enough LRS to reach approximately the original volume of blood.
Table 1. Clinical characteristics.
Animal subjects
The following protocol and experimental procedures performed by William Nugent and Bjorn Song (Song Biotechnologies, LLC, Baltimore, MD) were approved by the SoBran Biosciences Inc. IACUC (Fairfax, VA; Protocol # SON-004–2017) and are consistent with the National Institutes of Health guidelines for the humane treatment of laboratory animals, as well as the American Physiological Society’s Guiding Principles in the Care and Use of Animals. SoBran Biosciences operated the animal care vivarium and administered the IACUC. Animals used in this study were 28 male Sprague-Dawley rats (250–300 g; Charles River Laboratories Inc., Wilmington, MA).
Surgical preparation
Animals were inducted in anaesthesia with 1–5% isoflurane in medical air for initial pre-operative preparation and cannulations and were switched over to alfaxalone acetate (Alfaxan, Schering-Plough Animal Health, Welwyn Garden City, UK), which was continuously infused I.V. at 0.1 mg/kg/min through a femoral vein cannula for the duration of the experiment. A femoral artery cannula was connected to a pressure transducer to monitor systemic circulatory variables via a multichannel physiological monitoring system (BIOPAC MP-150, BIOPAC Systems, Goleta, CA). The carotid artery and jugular vein were cannulated for blood withdrawal and HbSS RBC infusions, respectively. A tracheal tube was inserted to maintain airway patency; however, animals were not artificially ventilated. After experimentation, animals were euthanized with a lethal dose of Euthasol (150 mg/kg, pentobarbital component, I.V.; Delmarva, Midlothian, Virginia).
Spinotrapezius preparation
The rat spinotrapezius muscle was exteriorized as described by Gray [Citation30] with some modification to accommodate measurements of microvascular activity and interstitial PO2 (PISFO2) on a thermostable animal platform adapted for intravital and phosphorescence quenching microscopy as described here [Citation29,Citation31,Citation32]. Animals and exteriorized spinotrapezius muscle – isolated from atmospheric contamination by a transparent barrier film (Krehalon (CB-100; Krehalon Limited, Tokyo, Japan) – were maintained at 37 °C and monitored by rectal probe (BIOPAC; Part # SS7L, BIOPAC Systems, Goleta, CA).
Microcirculatory parameters
Observation and measurement of the microvascular networks in the exteriorized spinotrapezius preparation were conducted with an intravital microscope (Axioimager2m, Carl Zeiss AG, Oberkochen, Germany) configured for trans-illumination through a 20X/0.8 objective (Plan-Apochromate, Zeiss, Germany) and custom-modified for phosphorescence quenching microscopy (PQM). 5X/0.25 and 40X/0.75 objectives (N-Achroplan, Zeiss, Germany) were used for visualization of the microvascular networks by focusing in the diametral plane and displaying the image on an ultra-high definition screen (XBR43X830C, Sony, Tokyo, Japan). Images and videos of the microvasculature were captured in real-time by a colour CMOS camera (Axiocam 305 colour, Zeiss, Germany) and stored digitally.
Phosphorescence quenching microscopy
Phosphorescence quenching for the detection of oxygen in biological systems was originally described by Rumsey et al. and Vanderkooi et al. [Citation33,Citation34]. Adaptation of this technology for microscopic measurements of the PISFO2 followed our previous reports [Citation29,Citation32,Citation35,Citation36] with a minor change in excitation source to a green laser diode operating a 520 nm (NDG7475 1W; Nichia Tokushima, Japan). Calibration of our PQM equipment and oxygen probe was performed as described by Golub and Pittman [Citation37].
Treatment solutions
LRS (Hospira Inc., Lake Forest, IL) is a crystalloid mixture of sodium chloride, sodium lactate, potassium chloride and calcium chloride in H2O that is used to treat dehydration and hypotension. It is hypo-oncotic to blood and contains no specific oxygen carrier.
PP-007 (PEGylated carboxyhaemoglobin bovine [PEG-COHb]; tradename: SANGUINATE®; Prolong Pharmaceuticals, LLC, South Plainfield, NJ) is a multi-functional, large volume parenteral that provides fluid and colloidal resuscitation in addition to gas transfer properties which have been described in depth elsewhere [Citation25,Citation26,Citation29]. PP-007 also contains a CO moiety attached to the PEGylated haemoglobin protein.
Experimental protocol for HbSS RBCs
All animals were pre-randomized into one of three treatment groups: 1) bolus (8 ml/kg, I.V.) infusion of PEG-COHb; 2) bolus (8 ml/kg, I.V.) infusion of LRS; or 3) no treatment (Sham). Then, they were subjected to an isovolemic ET (1 ml/kg/min) with triple washed HbSS RBCs suspended in LRS until distal spinotrapezius PISFO2 was non-detectable (<5 mmHg) and microvascular occlusions were visually apparent. Animals were observed for 15 min and then treated. Animals were then observed for up to 4 h.
Measurements of mean arterial pressure (MAP), pulse pressure, heart rate and temperature were recorded continuously. Imaging of the microvasculature and measurement of PISFO2 were conducted at discrete time points throughout each experiment: baseline (BL), end of ET (ET0) and hourly post-treatment (PT0, 60, 120…). Blood gas and chemistry analysis was performed on 65 µl blood samples taken at BL, ET0 and end of experiment using the ABL90 Flex (Radiometer America Inc., Brea, CA). Continuous data were plotted and analysed to align with discrete data collection time points.
ET with washed HbSS RBCs produced erythrocyte aggregation and microvascular hypoxia (hereafter defined as “VOC” in the context of this study). The spinotrapezius was divided into three microvascular measurement zones: distal, medial and proximal. ET was monitored by visual observation of microvascular flow and periodic sampling of PISFO2 of these zones. The distal region showed the earliest formation of reduced capillary perfusion, erythrocyte aggregations, and a non-detectable PISFO2 and served as a stop signal for ET.
Animals in VOC were observed for a 15 min period before treatment to ensure the erythrocyte aggregates did not spontaneously resolve. Animals were randomly assigned to one of three groups: Sham (no treatment), LRS, or PEG-COHb. For treatment groups, a bolus of 8 ml/kg was infused through the femoral vein over 5 min. The measurement series was performed immediately following treatment and then every 60 min for up to 4 h of the PT observation period. At the end of the observation period, or when the animal’s condition had worsened to the point where the MAP was <30 mmHg, an endpoint 65 µl blood sample was taken for ABL 90 analysis. For survival measurements, animals expired without intervention. A provision was included in the protocol that permitted administration of euthanasia in the event of observed distress, but it was not needed.
Study inclusion criteria were as follows: 1) no adverse incidents during surgical preparation, ET, treatment and observation phases, 2) development of “VOC” between 15 and 45% blood volume ET, which was determined as a distal PISFO2<5 mmHg and visual evidence of occlusions in the microvasculature, 3) microvascular RBC aggregates intact after 15 min of “VOC” observation period and 4) detectable PISFO2 in proximal regions of spinotrapezius muscle after ET. Adverse events during the observation period were limited to protocol failures or accidents (human error) that would affect the outcome, since survival was a metric.
Experimental protocol for normal RBCs
RBC isolation, surgical preparation and instrumentation were identical to the above HbSS RBCs protocol. ET was conducted until the first of either distal PISFO2 reaching <5 mmHg or an exchange volume of 60%, and animals were euthanized after 1 h of observation to determine whether RBC aggregates could form without HbSS RBCs.
Statistics
Data are expressed as mean ± standard error of the mean (SEM). Kaplan–Meier was used for the analysis of survival with post-hoc Log-rank Mantel–Cox. One-way ANOVA followed by Tukey’s HSD Prism version 6 (GraphPad Software, La Jolla, CA) were used to detect and describe differences between groups for ancillary data (i.e. weight and ET volume). Comparisons between groups and within groups for time-matched data collected during experimentation was conducted using a Two-way ANOVA where appropriate and further analysed by Tukey’s HSD when (p < .05).
Results
Development of VOC
Of the 36 animals used, 24 met the criteria for inclusion into the study with eight animals evenly divided into the treatment groups: Sham (untreated), LRS and PEG-COHb. Of the 12 excluded animals: three suffered complications during surgery, four did not meet VOC inclusion criteria and five experienced an adverse event that was unrelated to the experimental protocol. ET was similar across groups at 28 ± 2.8, 24 ± 1.5 and 24 ± 4.2% of estimated total blood volume for Sham, LRS and PEG-COHb, respectively, and produced significant reductions in total haemoglobin (Sham: 15–13 g/dl; LRS: 15–13 g/dl; and PEG-COHb: 15–14 g/dl; p < .05) which were not significantly different between groups. ET with washed HbSS RBCs produced visual reductions in microvascular flow and RBC aggregate development () and non-detectable PISFO2 in the distal portion of the spinotrapezius muscle (. A significant depression in MAP was also seen (see , BL – ET0 p < .0001 for all groups).
Figure 1. Microvascular Panel. Images were captured by a colour digital camera and adjusted for brightness and contrast to account for differential thicknesses in spinotrapezius muscles and minor shifts in focus. (A) Images were captured at baseline, immediately following ET and immediately after treatment. Each series is taken from a single site in an animal representative of its respective group. VOC is associated with a decrease in perfused vessels and a “blue shift” of larger vessels. Treatment with PEG-COHb but not LRS causes “red shift,” which is associated with a return of oxygenated haemoglobin to those blood vessels. (B) Each set of images is from a different experiment. The 5X image shows a VOC aggregate – denoted by purple arrow – that shifts position during treatment and then dissolves at the completion of treatment. The 40X image shows this effect in a smaller vessel where the aggregate can be seen undergoing a back-ended dissolution. Direction of flow is right to left in these images.
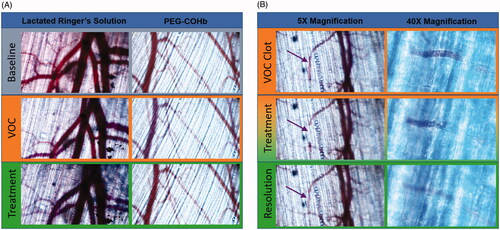
Figure 2. Tissue interstitial oxygenation. Tissue oxygenation was measured in the interstitial fluid bathing the capillary beds of the Distal, Medial and Proximal regions of the spinotrapezius muscle as the photograph diagrams. The Distal region was more sensitive to initial VOC aggregate formation and thus first developed local hypoxia. Other regions were not driven to hypoxia, but still showed reductions in PISFO2 following ET and responses to fluid treatment. PISFO2 was normalized to baseline for better visual comparisons and only intergroup differences are illustrated since tissues remained below baseline levels following ET. N = 8 per group. *p<.05, **p<.01, ***p<.001 and ****p<.0001.
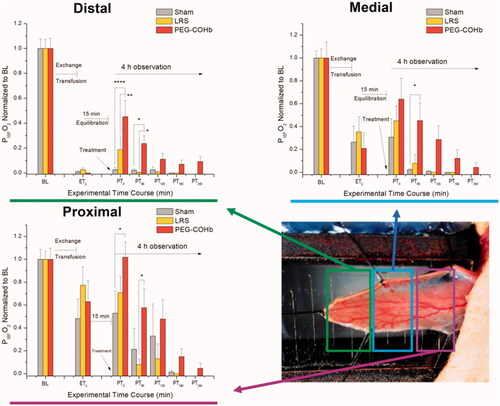
Table 2. Systemic variables.
VOC was observed to begin in the microvascular capillary beds and manifested as a visual reduction in perfusion. RBC aggregates then formed in post-capillary venules, followed by precapillary arterioles. Loss of perfusion progressed proximally down the spinotrapezius muscle followed by a blue-shift (colorimetric visualization due to de-oxygenation of RBCs) of arteriolar and venular blood. Finally, distal PISFO2 measured near zero. ET was stopped before proximal PISFO2 became hypoxic (. Occlusions were difficult to visualize in capillaries where RBC transit was single-file, but frequently presented as purple (deoxygenated) RBC aggregates in arterioles () and venules.
ET with normal (wild type) human RBCs
Four animals underwent ET with normal RBCs suspended in LRS until 60% ET. BL measurements were not significantly different from HbSS groups: PISFO2 – 45 ± 5.8 mmHg, MAP – 118 ± 8.8 mmHg and lactate – 15 ± 1.3 mg/dl. All four animals completed ET before distal PISFO2 registered >5 mmHg, however, in the time between the ET0 and measurement acquisition (∼3 min), distal PISFO2 declined to 2 ± 0.9 mmHg. Lactate and MAP for ET0 were 51 ± 5.0 mg/dl and 54 ± 5.3 mmHg, respectively, which were similar to HbSS ET0 measurements. Microvascular perfusion was visually “sluggish” with a blue-shift in arteriolar and venular blood, but there were no signs of RBC aggregates or occlusions. After an hour of observation, there remained no visual evidence of RBC aggregates or occlusions, although microvascular perfusion remained compromised and blue-shifted. Distal PISFO2 was non-detectable (<1 mmHg) and lactate had not significantly changed (48 ± 5.7 mg/dl). MAP improved from ET0 to 92 ± 4.3 mmHg (p < .01), but remained depressed compared to BL (p < .05).
Treatment of VOC in HbSS groups
VOC remained stable in the distal spinotrapezius for 15 min without progressing into the proximal regions. Untreated Sham animals showed a slow, but progressive development of VOC proximally over the course of the study resulting in the demise of all animals before the 4-h observation period ended. Bolus treatment with LRS or PEG-COHb both produced a noticeable improvement in capillary perfusion, but only PEG-COHb visually caused arteriolar and venular red-shifts indicating the presence of oxygenated haemoglobin ().
Survival
Survival was measured from PT0 until either demise or completion of the 4-h observation. Sham and LRS had mean survival times of 125 ± 23.4 and 131 ± 21.2 min, respectively, and were not significantly different. Additionally, their Kaplan–Meier survival profiles showed considerable overlap (. In the PEG-COHb treatment group, one demise occurred at 216 min PT while seven survived to the end of the 4-h observation period giving PEG-COHB a significantly increased survival curve versus both LRS and Sham groups (p < .001).
Figure 3. Post-treatment survival. Study groups were assessed for duration of survival from the end of treatment. PEG-COHB showed a significant increase in survival versus LRS and Sham. *** Indicates p<.001 result from overall Log-rank Mantel–Cox test between both LRS and Sham versus PEG-COHB groups. N = 8 per group.
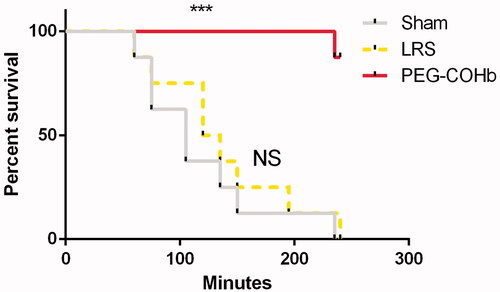
Tissue oxygenation
Measurements of the PO2 in the interstitial fluid bathing capillaries and skeletal muscle tissue were made in three regions running longitudinally from distal to proximal across the spinotrapezius preparation (illustrated in . Distal hypoxia (PISFO2 < 5 mmHg) was used as a stopping point for ET, which preserved the responsiveness to treatment. Distal PISFO2 was most dynamic between groups with PEG-COHb animals showing a return to 45 ± 13.1% of BL values, which was higher than LRS (19 ± 10.9%; p < .01) and Sham (3 ± 1.7%, p < .001). LRS and Sham were not significantly different from each other. At PT60, distal PISFO2 for PEG-COHb remained higher than Sham and LRS (p < .05, see ) . In the medial and proximal regions, PISFO2 did not become hypoxic following ET but was reduced from BL and followed the trends in the distal region (significant differences illustrated in .
Cardiovascular variables
ET caused a drop in MAP (p < .0001), but had no significant impact on pulse pressure, heart rate or temperature in all animals (see ). Sham and LRS maintained a reduced MAP compared to BL at PT0 (p < .0001 and p < .01, respectively). MAP in PEG-COHb-treated animals returned to BL values and remained steady until the conclusion of the 4-h observation period. LRS animals showed a recovery of MAP to BL by PT60 followed by a decline by PT120. PT180 was not significantly different from BL but is reflective of longer-lived animals (n = 3). Likewise, Sham remained hypotensive at PT60 and additional time points representing fewer, longer-lived animals (see . PEG-COHb produced a significantly higher MAP than Sham at PT0 and PT60 and versus LRS at PT120.
Heart rate increased in Sham compared to BL by PT120 (p < .05) and further at PT180 (p < .01), which was consistent with a compensatory mechanism normally seen near the demise of the animal. LRS showed a similar trend in heart rates beyond PT60, but no significant differences compared to BL. Pulse pressure was higher in LRS and PEG-COHb versus Sham at PT60 (p < .05), but otherwise steady. Core temperature did not significantly vary between groups and time points ().
Serum lactate
Lactate, a measure of systemic hypoxia, increased for all groups following ET (ET0; Sham: p < .0001; LRS: p < .001; PEG-COHb p < .01) compared to BL. All groups remained significantly elevated versus BL at the end-point measurement (Sham: p < .0001; LRS: p < .001; PEG-COHb: p < .05), however, when the end reading was compared to ET0, PEG-COHb had not changed whereas both Sham and LRS had risen significantly (p < .0001; p < .001, respectively). Intergroup comparisons are illustrated in . For BL and ET0, there is no significant difference between groups. At the end, PEG-COHb lactate levels were significantly lower than both LRS and Sham.
Figure 4. Lactate. Blood samples were collected at BL, ET0 and the experiment’s end (defined as 4 h post-treatment or a mean arterial pressure of <50 mmHg in demise animals) and assessed for serum lactate as an indicator of systemic metabolism. Induction of VOC produced a significant (not illustrated) increase in lactate across groups. Treatment produced a separation by the end of experiment with PEG-COHb showing no change versus ET0 (not illustrated). Intergroup comparisons are shown. N = 8 per group. ****p<.0001.
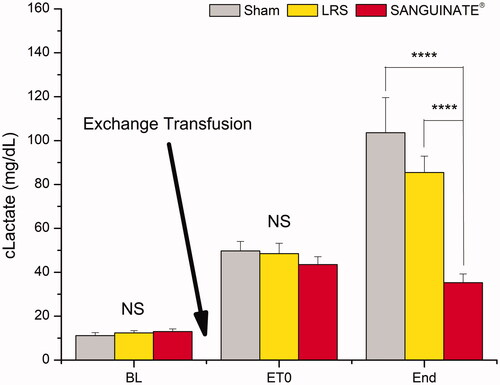
Discussion
A novel rat model of VOC was developed using isovolemic exchange transfused human HbSS RBCs to compare the efficacies of three approaches to treatment: 1) no treatment (Sham), 2) a standard intravascular fluid treatment (LRS) and 3) an oxygen-delivering intravascular plasma expander (PEG-COHb). Brightfield and phosphorescence microscopy assessed changes in the microvasculature with simultaneous recording of systemic variables and blood chemistry to interpret how localized manifestations of RBC sickling and aggregation translate to macroscopic physiological presentation. In vitro, ex vivo and in vivo transgenic murine models of VOC have utilized the microvascular as a focal point of study [Citation38], but to our knowledge, no murine model has combined the robust systemic, blood sampling and microcirculatory approaches afforded by this rat model, which provided a hardier platform for a robust battery of measurements aiming to capture as much of the pathophysiology as possible.
Leukocyte-free washed HbSS RBCs were used to induce VOC. This approach likely prevented the model from gaining insight on the pathology of individual donors in terms of how their innate immune systems may contribute to VOC, but helped create a reproducible, erythrocyte-driven model for testing. Addition of a cross-species immunological challenge via ET of unwashed HbSS blood was not well tolerated and ultimately an unnecessary complication of the study since it fails to model uncomplicated VOC. Human erythrocytes were generally well-tolerated as indicated by the four animals receiving RBCs from normal donors isolated under the same protocol as the HbSS RBCs. ET with normal RBCs produced a characteristic drop in MAP over time but did not have an appreciable impact on microcirculatory perfusion until reaching 60% (versus ∼25% for HbSS) and at no point was there evidence of VOC aggregate formation.
Under normal conditions, RBC sickling is associated with several factors (i.e. hypoxia, dehydration and acidosis) and, once there is a significant reduction in blood flow, can become self-perpetuating. In the case of the rat model, these factors were not specifically introduced, but VOC still developed. One explanation lies with the ET protocol, which is frequently associated with a drop in MAP and PISFO2 even when exchanging with an oxygen-carrying agent (as noted in this study and observed from in-house ET protocols (unpublished data)) . ET was likely responsible for the initial induction of RBC sickling, which resulted in reduced capillary perfusion that caused further local hypoxia and a drop in pH, which sickled more RBCs causing further decreases in perfusion. As ET continued, erythrocyte aggregates appeared in post-capillary venules eventually leading to a failure of RBC drainage from precapillary arterioles () and widespread aggregation. A visible blue-shift occurred as regional RBCs became deoxygenated (as seen colorimetrically in ) under conditions of oxygen supply/demand mismatch, which was then sufficient to ensure sickled RBCs and aggregates remained intact until an external intervention took place.
In humans, VOC is generally not self-amplifying to the point of acute mortality, but it does manifest over a range of severities. Mild or sub-acute manifestations of VOC may be managed by patient self-care at home (i.e. pain medication, sufficient hydration, NSAIDS and warmth), but severe, painful episodes may require intravenous opioids, crystalloids to relieve dehydration, hyperbaric oxygen and/or whole blood (normal type RBCs) or blood component ETs to dilute the population of sickle cells and reduce episodic VOC. In the acute sense, fluid infusion can increase blood flow and thus raise oxygen delivery to hypoxic tissues, which improves the likelihood of de-sickling allowing for normal perfusion to resume. Numeric increases in PISFO2 noted for LRS versus Sham () were insufficient to improve survival or even significantly increase MAP, but may explain why Sham animals showed significant elevations in heart rate versus BL by PT120, which is a classic indicator of cardiovascular distress, while LRS did not. PEG-COHb’s further increase in PISFO2 may have been chiefly responsible for significantly higher survival versus both LRS and Sham. Thus, PISFO2 a key metric for understanding the progression and treatment of VOC.
Interstitial oxygenation proved to be a good indicator for the systemic impact of VOC that was difficult to assess using continuous cardiovascular metrics. During ET, distal hypoxia arose from reaching an inflection point of RBC aggregation where oxygen supply became insufficient to demand and by ET0 was associated with serum lactates ∼45 mg/dl () indicating that VOC was a wide-spread, systemic phenomenon. PISFO2 was also key to demonstrating efficacy of treatment as higher values were associated with lower end-point lactates and longer survival. Regarding lactate, it should be noted that LRS contains 28 mmole/l lactate and can artificially increase serum lactate readings immediately after administration. It has been noted in dogs receiving 180 ml/kg/h LRS that lactate returns to BL values after 60 min [Citation39]. All end-point measurements with the exception of one LRS-treated animal at 50 min were taken well beyond 60 min post-infusion. We have also observed that lactate levels do not significantly rise in rats following a 10% volume top load with LRS (unpublished data) .
PEG-COHb is a biological gas transfer agent, but its small molecular size and oncotic properties may have an additional impact to this model. PEG-COHb essentially consists of haemoglobin molecules – the protein responsible for carrying ∼98% of oxygen in the blood. Each haemoglobin molecule is affixed with eight to ten 5000 kDa polyethylene glycols (PEGs) achieving a total molecular weight of approximately 104,000–114,000 kDa [Citation26,Citation40]. The addition of PEG molecules adds hydrodynamic bulk to limit the rate of extravasation while keeping individual particle size considerably smaller than RBCs (∼5 microns) and able to slip past occlusions in vasculature. Thus, in addition to a substantial increase in oxygen delivery, penetration of RBC aggregates may be key to the observed differences between LRS and PEG-COHb-treated animals. Additionally, PEG-COHb has a higher oncotic pressure compared to LRS, which recruits fluid to the vascular lumen potentially increasing microvascular perfusion. Our measurements showed that PEG-COHb relieved the hypotension associated with VOC without producing hypertension (). Treatment with LRS only produced numeric changes in PISFO2 and MAP indicating a possible utility for fluid support at higher treatment volumes. Likely, solutions with higher oncotic properties than LRS, such as albumin or whole plasma might show a further improvement over Sham, but without oxygen-carrying capability it would be unexpected to be as effective in dispersing VOC aggregates as PEG-COHb.
PEG-COHb contains a carbon monoxide (CO) moiety that affects both the regulation of blood flow and haemoglobin conformation. PEG-COHb is infused as ⁓96% carboxyhaemoglobin, which is then converted to oxyhaemoglobin upon exposure to the oxygenated environment of the blood. The off-loaded CO can then act as a vasodilator or bind to the haemoglobin active sites in sickle cells. Arteriolar vasodilation increases blood flow, which would increase oxygen delivery to hypoxic tissues, but the magnitude of the vasodilatory response to PEG-COHb is difficult to determine. In a previous study, treatment with 20% of the estimated blood volume showed neither vasoconstriction nor vasodilation compared to LRS or a higher oncotic hetastarch [Citation29]. CO binding to haemoglobin shifts the protein into the relaxed state resulting in depolymerization in the same fashion as oxygen binding. Thus, CO may provide an additional benefit to microvascular perfusion via unsickling RBCs. The specific effects of carboxyhaemoglobin versus oxyhaemoglobin were not assessed in this study, but it is acceptable to suggest that the initial delivery of CO is small compared to the continuous cycling of oxygen and oxygen delivery is important for long-term relief of RBC aggregates.
Conclusions
This in vivo animal study coupled systemic and microvascular measurements for both holistic and focal resolution of VOC crisis and the impact of various approaches to treatment. PEG-COHb was effective in relieving hypoxic RBC aggregates as assessed by improvements in PISFO2, serum lactate and survival compared with the standard-of-care LRS and untreated Sham animals. Oxygen delivery along with volume-expansion and small molecular size enabled PEG-COHb, at a clinical dose of 8 ml/kg, to access and relieve VOC occlusions.
This preclinical model of VOC offers a new, robust approach to characterizing the pathology and treatment of SCD. Whether disease-specific biomarkers are translatable from rats to humans is uncertain, but, ultimately, plumbing is plumbing out in the peripheral vasculature.
Limitations
This report describes a preclinical study of VOC in rats, which was designed to approximate, but not actuate the physiology and the pathology of human VOC. This study directly examines tissue oxygenation, which is currently infeasible in humans, and examines supporting variables (i.e. lactate, ScvO2 and methaemoglobin) which may not be translatable. VOC was acutely developed and erythrocyte-driven, which may not provide insight into chronic complications or inflammatory contributions. This study was designed to compare standard of care crystalloid infusions to PEG-COHb – a multi-faceted oxygen therapeutic. Additional research using comparator solutions with similar oncotic properties and presence or absence of CO moieties is needed to tease the impact of various components on RBC aggregation and microvascular perfusion. Additionally, blood flow was not directly assessed.
Disclosure statement
Authors Ronald Jubin, Pete Buontempo and Friedericke Kazo are employees of Prolong Pharmaceuticals, LLC. Authors William Nugent and Bjorn Song are employees of Song Biotechnologies, LLC. Prolong Pharmaceuticals, LLC, sponsored a portion of the study and, therefore, provided compensation to Song Biotechnologies, LLC.
Additional information
Funding
References
- Das R, Mitra A, Bhat V, et al. Application of isotope exchange based mass spectrometry to understand the mechanism of inhibition of sickle hemoglobin polymerization upon oxygenation. J Struct Biol. 2017;199:76–83.
- Noguchi CT, Schechter AN. Sickle hemoglobin polymerization in solution and in cells. Annu Rev Biophys Biophys Chem. 1985;14:239–263.
- Beutler E. The effect of carbon monoxide on red cell life span in sickle cell disease. Blood. 1975;46:253–259.
- Kaul DK, Nagel RL, Baez S. Pressure effects on the flow behavior of sickle (HbSS) red cells in isolated (ex-vivo) microvascular system. Microvasc Res. 1983;26:170–181.
- Haynes J, Jr., Taylor AE, Dixon D, et al. Microvascular hemodynamics in the sickle red blood cell perfused isolated rat lung. Am J Physiol. 1993;264:H484–H489.
- Olabode JO, Shokunbi WA. Types of crises in sickle cell disease patients presenting at the haematology day care unit (HDCU), University College Hospital (UCH), Ibadan. West Afr J Med. 2006;25:284–288.
- Embury SH. The not-so-simple process of sickle cell vasoocclusion. Microcirculation. 2004;11:101–113.
- Barabino GA, Platt MO, Kaul DK. Sickle cell biomechanics. Annu Rev Biomed Eng. 2010;12:345–367.
- Browning JA, Staines HM, Robinson HC, et al. The effect of deoxygenation on whole-cell conductance of red blood cells from healthy individuals and patients with sickle cell disease. Blood. 2007;109:2622–2629.
- Eaton WA, Hofrichter J. Hemoglobin S gelation and sickle cell disease. Blood. 1987;70:1245–1266.
- Steinberg MH. Management of sickle cell disease. N Engl J Med. 1999;340:1021–1030.
- Frenette PS. Sickle cell vasoocclusion: heterotypic, multicellular aggregations driven by leukocyte adhesion. Microcirculation. 2004;11:167–177.
- Pegelow CH, Colangelo L, Steinberg M, et al. Natural history of blood pressure in sickle cell disease: risks for stroke and death associated with relative hypertension in sickle cell anemia. Am J Med. 1997;102:171–177.
- Castro O, Brambilla DJ, Thorington B, et al. The acute chest syndrome in sickle cell disease: incidence and risk factors. The cooperative study of sickle cell disease. Blood. 1994;84:643–649.
- Yale SH, Nagib N, Guthrie T. Approach to the vaso-occlusive crisis in adults with sickle cell disease. Am Fam Physician. 2000;61:1349–1356.
- Afolabi BB, Iwuala NC, Iwuala IC, et al. Morbidity and mortality in sickle cell pregnancies in Lagos, Nigeria: a case control study. J Obstet Gynaecol. 2009;29:104–106.
- Machado RF, Hildesheim M, Mendelsohn L, et al. NT-pro brain natriuretic peptide levels and the risk of death in the cooperative study of sickle cell disease. Br J Haematol. 2011;154:512–520.
- Serjeant GR. The emerging understanding of sickle cell disease. Br J Haematol. 2001;112:3–18.
- Stirnemann J, Letellier E, Aras N, et al. Hyperbaric oxygen therapy for vaso-occlusive crises in nine patients with sickle-cell disease. Diving Hyperb Med. 2012;42:82–84.
- Marouf R. Blood transfusion in sickle cell disease. Hemoglobin. 2011;35:495–502.
- Odesina V. Intravenous support for the patient in sickle cell crisis. J Intraven Nurs. 2001;24:32–37.
- Okpala I. The management of crisis in sickle cell disease. Eur J Haematol. 1998;60:1–6.
- Robie IC, Kellner JD, Coppes MJ, et al. Analgesia in children with sickle cell crisis: comparison of intermittent opioids vs. continuous intravenous infusion of morphine and placebo-controlled study of oxygen inhalation. Pediatr Hematol Oncol. 1992;9:317–326.
- Zipursky A, Robieux IC, Brown EJ, et al. Oxygen therapy in sickle cell disease. Am J Pediatr Hematol Oncol. 1992;14:222–228.
- Ananthakrishnan R, Li Q, O’Shea KM, et al. Carbon monoxide form of PEGylated hemoglobin protects myocardium against ischemia/reperfusion injury in diabetic and normal mice. Artif Cells Nanomed Biotechnol. 2013;41:428–436.
- Klaus JA, Kibler KK, Abuchowski A, et al. Early treatment of transient focal cerebral ischemia with bovine PEGylated carboxy hemoglobin transfusion. Artif Cells Blood Substit Immobil Biotechnol. 2010;38:223–229.
- Abuchowski A. PEGylated bovine carboxyhemoglobin (SANGUINATE): results of clinical safety testing and use in patients. Adv Exp Med Biol. 2016;876:461–467.
- Abuchowski A. SANGUINATE (PEGylated carboxyhemoglobin bovine): mechanism of action and clinical update. Artif Organs. 2017;41:346–350.
- Nugent WH, Cestero RF, Ward K, et al. Effects of sanguinate(R) on systemic and microcirculatory variables in a model of prolonged hemorrhagic shock. Shock. 2017 [Epub ahead of print].
- Gray SD. Rat spinotrapezius muscle preparation for microscopic observation of the terminal vascular bed. Microvasc Res. 1973;5:395–400.
- Golub AS, Pittman RN. Thermostatic animal platform for intravital microscopy of thin tissues. Microvasc Res. 2003;66:213–217.
- Nugent WH, Song BK, Pittman RN, et al. Simultaneous sampling of tissue oxygenation and oxygen consumption in skeletal muscle. Microvasc Res. 2016;105:15–22.
- Rumsey WL, Vanderkooi JM, Wilson DF. Imaging of phosphorescence: a novel method for measuring oxygen distribution in perfused tissue. Science. 1988;241:1649–1651.
- Vanderkooi JM, Maniara G, Green TJ, et al. An optical method for measurement of dioxygen concentration based upon quenching of phosphorescence. J Biol Chem. 1987;262:5476–5482.
- Song BK, Nugent WH, Moon-Massat PF, et al. Effects of top-loading a zero-link bovine hemoglobin, OxyVita, on systemic and microcirculatory variables. Mil Med. 2013;178:570–577.
- Song BK, Nugent WH, Moon-Massat PF, et al. Effects of a hemoglobin-based oxygen carrier (HBOC-201) and derivatives with altered oxygen affinity and viscosity on systemic and microcirculatory variables in a top-load rat model. Microvasc Res. 2014;95:124–130.
- Golub AS, Pittman RN. Barometric calibration of a luminescent oxygen probe. J Appl Physiol. 2016;120:809–816.
- Kaul DK, Finnegan E, Barabino GA. Sickle red cell-endothelium interactions. Microcirculation. 2009;16:97–111.
- Boysen SR, Dorval P. Effects of rapid intravenous 100% L-isomer lactated Ringer’s administration on plasma lactate concentrations in healthy dogs. J Vet Emerg Crit Care. 2014;24:571–577.
- Nho K, Glower D, Bredehoeft S, et al. PEG-bovine hemoglobin: safety in a canine dehydrated hypovolemic-hemorrhagic shock model. Biomater Artif Cells Immobilization Biotechnol. 1992;20:511–524.