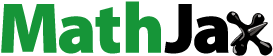
Abstract
Despite the advances in the development of chemotherapeutic agents, resistance to chemotherapy and adverse side effects are still big challenges against successful cancer treatment. To overcome these problems, one strategy is the application of nanomaterials and drug delivery systems to efficiently deliver the anticancer agents to tumour tissues with minimum toxic effects on healthy organs. In this study a graphene oxide nanohybrid (GO/NHs) was designed and fabricated for the delivery of chemotherapeutic agent fluorouracil (FU) to the breast cancer MCF7 cells. After preparation and characterization of GO/NHs, several biological analysis including haemolysis assay, cytotoxicity assay, cellular uptake, apoptosis assay, and protein expression were performed. The cytotoxic effects of FU, FU loaded GO/NHs (FU-GO/NHs), and blank GO/NHs was determined by MTT assay. The results of MTT assay showed no significant cytotoxicity for blank nano-hybrid on MCF7 cells. Furthermore, FU-GO/NHs were more cytotoxic than free FU. The uptake analysis results showed that developed nanocarrier could completely be internalized into the cells in the first hour. Besides, apoptotic effects and nuclear morphology changes of cells was evaluated by DAPI staining under fluorescent microscopy. Protein expression levels of p53, PARP, cleaved PARP, Bcl-2, and Bax were determined by western blot analysis. Western blot results showed higher levels of p53 and cleaved PARP after treatment with FU-GO/NHs, however, no substantial effect was observed for Bax and Bcl-2 protein concentrations.
Introduction
Cancer is a group of diseases with growing of abnormal cells by an uncontrolled way [Citation1]. Cancer can be treated with several approach including surgery, radiotherapy, hormonal therapy, chemotherapy and etc. [Citation2–5]. Some of cancer patients have received one treatment, but most of patients have received combination treatment. Among mentioned treatments, chemotherapy is an important tool for treatment of many types of cancer, however, it has many toxic side effects such as anaemia, nausea, infection and neutropenia [Citation6,Citation7]. To reduce these side effects, researchers in the nanotechnology and nanoscience fields try to design and fabricate carriers with nano size with capability to transfer chemotherapeutic agents to the target cancer. This field of science is named drug delivery systems (DDS) which enables the introduction of a chemotherapeutic agents in the body and enhances its efficacy [Citation8]. Many platforms as drug carriers were developed for using in DDS based on liposomes, polymers, micelles, nanocomposites, magnetic nanoparticles and carbon nanostructures such as carbon nanotubes and graphene oxide [Citation9–15]. Graphene-based drug carriers are being used widely in a broad range of applications especially DDS for delivery of chemotherapeutic agents to cells [Citation16]. However, they have toxicity effects in human body with complex interactions with proteins and solutes. In this case, surface modification of graphene oxide with other molecules or polymeric structures were done to overcome these problems and improve the biodegradability [Citation17]. Therefore, a suitable coating is necessary to improve these situations. Several polymers (synthetic and natural) were used for modification of graphene oxide [Citation18–20]. Temperature and pH-responsive polymers are currently used in many various applications specifically in drug delivery system due to their unique properties which could release therapeutic agents surrounding the tumour tissues [Citation21,Citation22].
In this report, to increase the efficiency of fluorouracil, polyvinylpyrrolidone-NIPPAM-lysine graphene oxide nano-hybrid (GO/NHs) as a pH and temperature responsive anticancer drug carrier on MCF7 cell line was designed, The structural and physicochemical characteristics of the GO/NHs was evaluated by several chemical characterizations such as FTIR, DSC, XRD, SEM, EDX, TEM, DLS and zeta potential analysis. Fluorouracil as a model anticancer drug was chosen to load on the prepared GO/NHs and drug loading and encapsulation efficiencies were calculated. FU-GO/NHs as a drug carrier was used in the cytotoxicity assay, cellular uptake and apoptosis assay on MCF7 cells. Finally, to explore the possible mechanism of apoptosis induction, western blot analysis was performed to determine the protein expression levels of p53, PARP, cleaved PARP, Bcl-2 and Bax proteins.
Experimental section
Materials and methods
Chemical reagents including 2,2′-azobis(2-methylpropionitrile) (AIBN), sodium nitrate (NaNO3), concentrated sulfuric acid (H2SO4; 95–98%), hydrochloric acid (HCl; 37%), potassium permanganate (KMNO4) and hydrogen peroxide (H2O2; 30%) were purchased from Merck Co. and used as received. All solvents used in this study were also obtained from Merck Co. and purified according to the purification standard methods. In all experiments steps, deionized water was used which purified with a Milli-Q water system. Graphite, N-isopropylacrylamide (NIPAAm; 97%), 1-Vinyl-2-pyrrolidinone (VP; 95%), lysine hydrochloride (Lys; 98%), 4',6-diamidino-2-phenylindole dihydrochloride (DAPI), rhodamine B (Rhd-B) and fluorouracil (FU) as an anticancer drug were purchased from Sigma Aldrich Co. Human red blood cells were kindly obtained from Iranian Blood Transfusion Institute which stabilized with ethylenediaminetetraacetic acid (EDTA). All materials which are used in biological methods and assays including MTT (3–(4, 5-dimethylthiazol-2-yl)-2, 5-diphe-nyltetrazolium bromide), trypsin, fetal bovine serum (FBS), and Roswell Park Memorial Institute 1640 growth medium (RPMI) were purchased from Gibco BRL Life Technologies. MCF7 cells as human breast cancer cells were obtained from Cell Bank, Pasteur Institute of Tehran, Tehran, Iran.
Characterizations
Fourier-transform infrared (FTIR) spectroscopy was used as an established tool for determination of the sample's structural characterizations. All samples were recorded on Bruker FTIR instrument manufactured by Shimadzu, Japan. Blank potassium bromide (KBr) disk was used as a background which scanned at wavenumbers ranging from 1000 to 4000 cm−1. All sample disks were previously prepared with KBr at the weight ratio of 1:50 (sample to KBr). X-ray diffraction (XRD) patterns of powder samples were recorded on a Siemens diffractometer with Cu-Kα radiation (λ = 1.542A˚) at 35 kV with the Bragg angle ranging from 2θ = 0–80°. The patterns were analyzed with X'pert Highscore plus software. Differential scanning calorimetry (DSC) was used for monitoring the thermal behaviour of samples using a Mettler DSC 821e(United State) from 35 to 400 °C at a heating rate of 20 °C min−1 under a flowing nitrogen atmosphere. An appropriate amount (5 mg) of a sample was put in the aluminum pan and placed into the instrument for analysis. Dynamic light scattering (DLS) is a technique for determination of particle size distribution and surface charge of samples in aqueous media taken by Zetasizer Nano ZS90, Malvern Instruments, Malvern, UK. In this case, the samples were dispersed in PBS (pH 7.4) solution with an appropriate concentration for analysis. The morphology, size and elemental percentage analysis of samples were measured using a field emission scanning electron microscopy-energy dispersive using X-ray (FESEM-EDX; TESCAN MIRA3, Czech Republic). Prior to examination, powder samples were placed onto a metal stub using a carbon double-sided adhesive tape and covered with a thin layer of gold, with the aid of a direct current sputter technique (Emitechk450X, England). Besides, elemental analysis by Energy Dispersive X-Ray analysis technique was employed to determine the accurately atomic and weight percentages of elements. The TEM analysis was also used to determine the size and morphology of the samples by a CM120 Philips instrument (Germany) with operating at 200 kV. Before analysis, the suspension was ultrasonically dispersed in deionized water using a probe-type ultrasonic generator (400 W) for 10 s. Then, one droplet of the sample suspension was dropped on a copper grid and allowed to vaporize the water.
Preparation graphene oxide (GO)
Hummer's modified method was adopted for preparation of graphene oxide (GO) from graphite with slight modification [Citation23]. In brief, 100 ml of H2SO4 was added to a flask and cooled in an ice-water bath. Subsequently, 4.00 g of graphite powder and 2.12 NaNO3 were added to the acidic solution and allowed to stir vigorously for one hour. Afterward, KMnO4 was added gradually to the mixture in three steps every 10 min (4.15 g in each step). The temperature of the mixture was increased after these steps due to the exothermic reaction occurring. (Caution: avoid from temperature increase over 15 °C). After addition was completed, the suspension was stirred at 35 °C for 72 h until obtaining a brown pasty liquid. Then, 200 ml of cold deionized water was added to the above suspension to dilute the concentrated H2SO4 and the following procedure was continued. 60 ml H2O2 with 110 ml of deionized water were added which color of the suspension was changed to yellow. In the end, the suspension was centrifuged at 6000 rpm for 5 min and washed several times with acidic solution (HCl; 3%) and deionized water. Finally, the obtained GO was dried under vacuum condition at room temperature.
Preparation of GO-Nanohybrid (GO/NHs)
Following procedures were done in two steps for the preparation of graphene oxide nanohybrid (GO/NHs) as a drug carrier.
Step1: In a round bottom flask containing 40 ml DMF, 3.0 g NIPAAm and 1 ml 1-vinyl-2-pyrrolidinone were added and stirred to obtain a homogenous solution. Then, 0.7 g graphene oxide was added to the solution and dispersed in water-bath sonicator for 5 min. Subsequently, 0.012 g of AIBN as a catalyst was added to the suspension, and the polymerization reaction occurred at 70 °C under the nitrogen atmosphere for 48 h. Thereafter, the obtained GO-NIPAAm-VP was dried under vacuum oven at 40 °C for 24 h.
Step 2: In a round bottom flask, prepared GO-NIPAAm-VP and 1.5 g lysine were dispersed in 20 ml deionized water and allowed stirring at room temperature for 24 h to graft lysine on the surface of GO-NIPAAm-VP. Finally, obtained GO-NIPAAm-VP-lysine which named graphene oxide nanohybrid (GO/NHs) was dried and used in drug loading step and other in-vitro evaluations.
Drug loading and release studies
In-vitro drug loading and release were performed according to the following procedures:
Loading step: FU was used as an anticancer drug and loaded on the GO/NHs. Firstly, 10 mg FU was dissolved in 10 ml PBS solution and stirred for 10 min with the magnetic stirrer under dark condition. Afterward, 100 mg GO/NHs as a drug carrier was added to the drug solution and sonicated for 10 min in water bath sonicator. Then, the obtained suspension was allowed to stir for overnight with moderate shaking to load the FU on the GO/NHs. The suspension was centrifuged at 6000 rpm for 5 min and washed three times with PBS to remove the unbounded drug. Finally, FU-GO/NHs was vacuum dried at room temperature for 24 h and used in following releasing step. The drug loading and encapsulation efficiencies were calculated by using the two following formula:
Releasing step: 20 mg FU-GO/NHs was added to a dialysis bag and immersed in 20 ml PBS solution (pH 7.4 and 5.5) at two different temperature 37 and 42 °C. At a certain time, 5 ml PBS solution containing released FU was taken out and 5 ml fresh PBS was added to the media. The concentration of release FU (µg mL−1) was calculated by measuring the absorbance of FU at 264 nm with UV-Vis spectrophotometer and converting the absorbance to the concentration of FU by using calibration curve (absorbance versus concentration). The obtained concentration (Cn) was converted to the corrected concentration (Cc) by using the following formula:
Finally, the drug release percentages of FU-GO/NHs was calculated according to the below-mentioned formula at a certain time.
In-vitro biocompatibility by haemolysis assay
Haemolysis assay was performed for evaluation of blood biocompatibility of prepared GO/NHs as a nanocarrier on human red blood cells [Citation24]. At first, the freshly obtained blood was centrifuged at 5000 rpm for 5 min and the human plasma was discarded. The pellets (human red blood cells) was washed several times with PBS (pH 7.4) (centrifuged at 5000 rpm for 5 min in each time) and diluted 10 times with PBS (pH 7.4). In the second step, serial dilution of GO/NHs suspension was prepared (in PBS; pH =7.4; 0.5 ml) at concentration of 5, 10, 50, 100, 200, 500 and 1000 µg mL−1. Subsequently, 0.5 ml of diluted human red blood cells in PBS was added to each concentration. The samples were incubated at 37 °C for 3 h. Afterward, all samples were centrifuged (5000 rpm for 5 min) and the supernatant was isolated and transferred to the 96-well plate for measuring lysis of hemoglobin via ELISA reader at 570 nm. In the end, haemolysis percentage were calculated by following formula:
where the supernatant absorbance of the sample, negative control (PBS), and the positive control (water) are OD(sample), OD(−), and OD(+), respectively.
Cell culture
MCF7 cells (human breast cancer cells) were obtained from the Pasteur Institute of Iran, Tehran, Iran. The cells were grown in RPMI culture in the presence of 10% FBS, 100 units mL−1 penicillin and 100 μg mL−1 streptomycin. The culture media was also supplied with 1% non-essential amino acids. The cells were incubated (5% CO2; 37 °C; humidified condition) for several days and the cells confluency was monitored by a microscope. After 90% confluency, the cells were trypsinized and collected. The collected cells were re-suspended in culture media containing FBS and centrifuged at 1500 rpm for 5 min. Finally, the cells were dispersed in the culture medium with known concentration and used for further in vitro assays.
Cell viability test by MTT assay
To determine the cytotoxic effects of various samples against MCF7 cells, MTT assay was performed according to the following procedure [Citation25]. In brief, cells were cultured in 96-well plates at the density of 104 cells in each well and maintained in the incubator for 24 h in order to allow the cells attaching at the bottom of the wells and grow. After 24 h, the culture medium was removed and replaced with fresh medium containing various concentrations of free FU, FU-GO/NHs and GO/NHs. All treatments were performed in triplicates and cells received only fresh medium with no treatment were considered as control group. After incubating the cells for 48 h, the medium was replaced with fresh medium containing 100 μg mL−1 MTT and incubated for further 4 h. MTT is a yellow compound which is reduced to dark purple formazan by the mitochondrial succinate dehydrogenase of live cells. To dissolve the formed formazan crystals, the medium was replaced with 200 μl of dimethyl sulfoxide (DMSO) and incubated at dark on the shaker incubator for 30 min. Finally, the absorbance of soluble formazan was measured at the wavelength of 570 nm using an ELISA plate reader (State Fax, 2100, Awareness Technology Inc, Palm City, FL, USA).
Cellular uptake analysis
As rhodamine b (Rhd-B) has fluorescent characteristics, it can be used as a marker for cellular uptake analysis of GO/NHs. After loading of Rhd-B on the prepared GO/NHs (Rho-GO/NHs) using the procedure described in previous articles, it was used in cellular uptake study [Citation26].
Fluorescent microscopy method: MCF7 cells were seeded in 6-well plates containing sterile coverslip for quantification of cellular uptake at a density of 5 × 104 cells per well. When the cells were reached to 70% confluency and ready for treatment, the wells were washed with PBS and 2 ml fresh culture media were added to each well. The MCF7 cells were treated with Rho-GO/NHs at two treated time (1 and 3 h). Then, the coverslips were fixed onto a glass microscope slides and intracellular fluorescent emission associated with nanocarrier uptake was observed using a fluorescence microscope (Olympus microscope Bh2-RFCA, Japan).
Flow-cytometry method: MCF7 cells which dispersed in culture media (as mentioned in cell culture section) were seeded in the 6-well plates at the density of 5 × 105 and incubated for 24 h to attach the bottom of the wells. The seeded cells were treated with Rho-GO/NHs and incubated for 1 and 3 h. After determined time for treating, the cells were washed two times with PBS to get rid of no internalized Rho-GO-NHs into the cells and trypsinzed for 5 min to detach the cells. The appropriate amount of culture media was added to the detached cells and centrifuged at 1500 rpm for 5 min. The cells were re-dispersed and centrifuged in PBS for two times. In the end, the cells were subjected to flow-cytometry analysis using FACScalibur flow-cytometer (Becton Dickinson Immunocytometry Systems, San Jose, CA, USA).
Apoptosis study by DAPI staining
MCF7 cells (4 × 104 cells per well) were incubated with free FU, FU-GO/NHs, and GO/NHs for 48 h. The treated dosage for FU and FU-GO/NHs were their IC50. The cells with no treatment considered as control. After treating for 48 h, cells were washed with PBS and fixed with 4 wt% formaldehyde for 15 min at room temperature. Subsequently, the cells were washed with PBS and permeabilized with Triton X-100 (0.1% w/v) for 5 min. After washing, the cells were stained with 300 ng mL−1 DAPI solution and incubated in the dark condition for 15 min. Finally, the condensed and fragmented DNA of apoptotic cells were observed with a fluorescence microscope (Olympus microscope Bh2-RFCA, Japan).
Protein extraction and Western blotting
To determine the protein expression levels of p53, cleaved PARP, Bcl-2, Bax, and β-actin, western blot analysis was performed [Citation27]. After the treatment of cells with various samples for 48 h, cells were harvested and lysed to extract cell lysate using the RIPA cell lysis buffer containing Tris buffer (0.05 mM), sodium chloride (150 mM), EGTA (0.1%), SDS (1%), and 0.1% antiprotease cocktail (ROCHE). The concentration of protein in each sample was determined using the Bio-Rad protein assay kit and samples were kept at −20 °C until the day of analysis. Each 50 μg of protein samples were boiled for 10 min and then loaded into the 10% sodium dodecyl sulfate-polyacrylamide gel electrophoresis (SDS-PAGE). After the proteins were separated by electrophoresis, they were transferred onto the PVDF membrane, blocked with skim milk and following three steps of washing with TBST (Tris-buffered saline with 0.1% Tween 20), membranes were incubated with primary antibodies for overnight and at 4 °C. Afterward, the membranes were washed again with TBST three times and incubated with horseradish peroxidase (HRP)-conjugated secondary antibodies for 2 h and at room temperature. After the membranes were washed for three further times, they were developed by ECL reagents (Thermo Fisher Scientific, USA) and X-ray films. Finally, the density of each protein band was determined using the ImageJ software.
Statistical analysis
All data were reported as mean ± SD of three replicates. ANOVA or Student’s t-test were used for statistical analysis. p values of less than .05 were considered significant.
Results and discussion
Preparation of GO/NHs as a drug carrier
Firstly, GO was synthesized by a modified Hummer’s method with the thickness of nano size. Then, NIPAAm and VP as the polymerization monomers were polymerized in of GO nano-sheets suspension via common radical polymerization method in the presence of AIBN as a catalyst. Due to the existence of epoxy functional groups on the surface of GO nano-sheets, lysine could be attached on GO from amine functional group moiety. In the end, GO/NHs was achieved with several functional groups on its surface as a drug carrier for delivery of FU as an anticancer agent to the MCF7 cells ().
Characterization and chemical analysis
To confirm and validate the successful preparation of GO/NHs several characterizations including FTIR, XRD, DSC, SEM, TEM, DLS and zeta potential were performed and the obtained results were discussed as follow.
FTIR spectra of GO, GO-NIPAAm-VP, and GO-NIPAAm-VP-lysine (GO/NHs as a drug carrier) were shown in . GO has a broad characteristic peak at about 3370–3450 cm−1 related to the stretching vibration bonds of hydroxyl (–OH) and carboxylic acid (–COOH) functional groups at edge of GO sheet. A strong peak at 1735 cm−1 is attributed to the carbonyl (C = O) of carboxylic acid. Furthermore, other characteristic peaks at 1063, 1281 and 1623 cm−1 are corresponded to the C–O, epoxy group, and C = C, respectively. After surface modification of GO by polymerization of NIPAAm and VP, a new characteristic peak was observed around 2974 cm−1 which related to the C–H stretching vibration bond of poly vinylpyrrolidone-NIPPAm polymeric chains. Amide functional groups (–HN–C = O) in the structure of NIPAAm and VP was shown their peaks at 1670 cm−1 which confirmed that the polymerization was done successfully. Whereas the peak of carboxylic acid was disappeared. Due to the presence of same functional groups in both GO and polymeric chains structure, their peaks were overlapped each other. Lysine has three main functional groups including amine (–NH2), carboxylic acid (–COOH), and methylene (C–H) which all stretching vibration bonds overlapped with polymeric and GO bonds.
Figure 2. FTIR spectra GO (a), GO-NIPAAm-VP (b), and GO-NIPAAm-VP-lysine (GO/NHs) (c) at wavenumbers ranging from 1000 to 4000 cm−1.
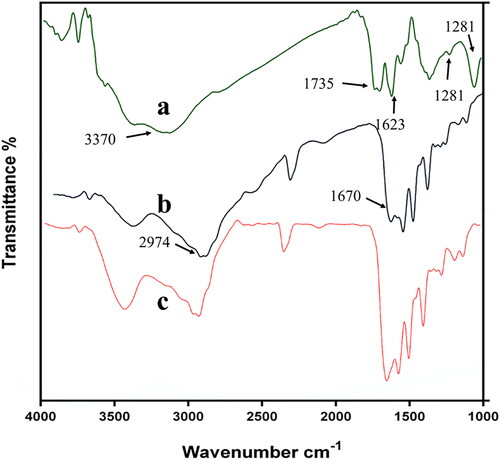
showed the XRD profile of pure GO and GO/NHs (as a drug carrier). GO has a characteristic peak with high intensity at about 2θ = 12° which confirm the crystalline structure of GO. After occurring polymerization process in the GO suspension to obtain GO/NHs, the typical peak of GO was disappeared whereas a new peak around 2θ = 26° appeared. It was concluded that the GO nano-sheet was completely and homogenously dispersed in the prepared nanohybrid polymeric network.
The DSC was applied as a technique for determination of thermal behaviour of prepared GO/NHs as a drug carrier. As seen in , a broad endothermic peak was found around 200 °C which may be attributed to the melting point of the nanohybrid. Another endothermic peak was observed in the DSC graph at about 250 °C and it related to the decomposition of the labile oxygen-containing functional groups (such as –C = O, –COOH, –OH, etc.). Due to the presence of hydrophilic section on the GO sheets the hydrophilic parts of polymeric chains could interact with each other leading to strong interaction between polymeric chain with GO as a cross linker.
Figure 4. Thermal behaviour of GO/NHs by DSC analysis from 35 to 400 °C at a heating rate of 20 °C min−1 under a flowing nitrogen atmosphere.
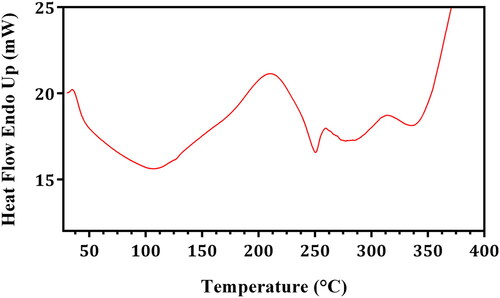
To characterize the size distribution of particles (such as polymers, micelles, vesicles, nanoparticles, etc.) in suspension, dynamic light scattering (DLS) technique was used. The determined size in this method is depend on several factors including particle core, size of surface, particle concentration and type of media. In this regard, we aimed to determine size of our designed GO/NHs in PBS solution (simulated condition of body) at very low concentration about 20 ppm. As seen in , the DLS diagram displayed two particle population at two different diameters and intensity (14.8% for peak 1 and 85.2% for peak 2) and it is showed that the prepared drug carrier has relatively monodispersed. The particle size distribution is about 37 nm which is suitable for drug delivery systems with capability of internalization into the cells.
The surface morphology of GO and GO/NHs were studied and evaluated by SEM analysis which are presented in . The SEM images of GO showed that GO shape are flake layers with low thickness. It was observable that the surface of GO has functionalization by NIPAAm-VP co-polymer with semi-spherical structure which distributed on the GO flakes. As the weight ratio of the GO is less than polymer, it was expected that the flake and GO sheet couldn’t be observed clearly. To validate the synthesis of our GO/NHs, EDS analysis was also taken. To determine the presence of elements on the surface of samples, selected area energy dispersion spectrum (EDS) analysis was used which provide a semi-quantitative view of the elements (). GO has two detectable elements in EDS including carbon (C) and oxygen (O). After preparation of GO/NHs, the results were shown that GO/NHs has three main elements including carbon (C), nitrogen (N) and oxygen (O) with weight percentages of 49.55, 31.35 and 19.10%, respectively.
Figure 6. SEM images of the surface of GO 10 µm (a), 2 µm (b) and prepared GO/NHs 2 µm (c), and 500 nm (d).
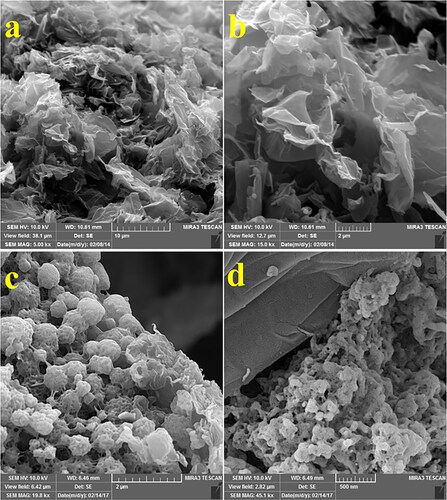
Figure 7. Selected area energy dispersion spectrum (EDS) analysis of GO/NHs as a semi-quantitative view of the elements.
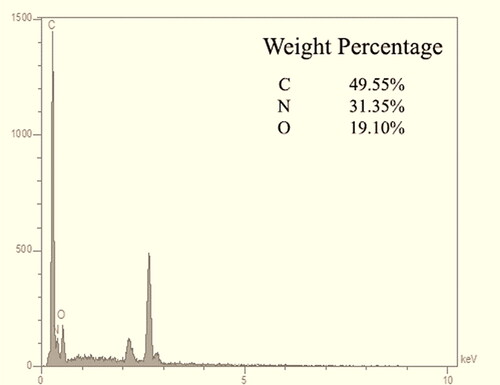
The graphene oxide formation and preparation of GO/NHs were also confirmed with TEM analysis. is the representative image of the GO and GO/NHs which showed that the prepared GO is thin crumpled sheet. As was shown, GO has relatively clear and transparent flakes which confirmed the thin layer of GO suggesting that GO is low or monolayer sheet. By observing the TEM images of GO/NHs, it was clearly demonstrated that some dark spots were formed and distributed on the GO nano-sheet uniformly. Besides, the GO/NHs showed that has thicker than GO mono layer due to the formation of polymeric chains on the GO.
In-vitro loading and release studies
Before in-vitro biological analysis, drug loading and release studies were investigated. Firstly, FU as an anticancer drug was chosen for loading drug on the prepared GO/NHs in PBS solution (pH 7.4). The nanocarrier in this study has several functional groups such as hydroxyl (–OH), carboxylic acid (–COO) and carbon-carbon double bond (C=C) in graphene oxide platform and other functional groups including carbonyl (C=O), amide (–CO–NH–), amine (–NH2) and carboxylic acid (–COOH) on the polymeric backbone and lysine grafting. On the other hand, FU has amide and double carbon-carbon bonds in its structure. After that, FU could be loaded easily on the prepared GO/NHs as a drug carrier via hydrogen bonding, electrostatic interaction, and physical interactions. Some of the drugs were loaded on the GO/NHs via hydrogen bonding or electrostatic physical interactions between polar groups on both structures. Besides, some of the drugs were loaded via π-π interaction between graphene oxide platform and C = C bonds in drug structure. While other parts of drugs were entrapped in the polymeric architecture by physical interactions. After calculation of the amount of drug loading and encapsulation efficiencies with mentioned formula in the experimental section, their amounts were obtained 7.28% and 72.89%, respectively and obtained FU-GO/NHs used as a drug carrier in the release study.
Drug release profile of prepared FU-GO/NHs was investigated in two different pH and temperature values (pH 5.5 and 7.4 and 40 °C for tumour tissues and 37 °C for physiological and normal tissues simulated conditions). As seen in , two different release profile were shown at various temperature with high and moderate release at 40 and 37 °C. It was showed that the designed drug carrier has temperature-responsive property and showed high drug release surrounding simulated tumour tissues condition. Furthermore, the drug release profile of FU-GO/NHs at different pH values was investigated and the results confirmed that there is little difference in drug release profiles at pH 7.4 and 5.5. It was concluded that more parts of drug-carrier interactions aren’t related to electrostatic interactions and could release drugs by altering pH of drug carrier media.
Haemolysis assay
Drug carriers which are used in drug delivery systems should have good compatibility with human red blood cells. As these carriers were injected into the patients, their biocompatibility should be evaluated before using in-vivo applications. In this regard, haemolysis assay as a standard protocol performed to evaluate the compatibly of prepared GO/NHs on human red blood cells (erythrocytes) according to the recent literature [Citation28]. As seen in , human red blood cells were treated with different concentration of GO/NHs including 50, 100, 200, 400 and 800 µg mL−1. Furthermore, to compare the obtained results with positive and negative control, the red blood cells were also treated with water and PBS, respectively. After incubation of erythrocytes with water, they were fully damaged and the hemoglobin was released into the surrounding media. Whereas the erythrocytes which treated with PBS kept their shapes and no changes were seen. On the other hand, prepared GO/NHs have shown the low hemolytic effect on red blood cells with a dose-dependent effect. The haemolysis rate for treated dosage were 1.31, 1.95, 2.77, 3.85 and 5.37% by increasing the GO/NHs concentration. All results confirmed that the prepared drug carrier could use in further in-vivo applications.
Cytotoxicity assay
To determine the cytotoxic effects of free FU, FU-GO/NHs, and GO/NHs, MTT assay was performed. Our results showed that the synthesized drug carrier had no substantial cytotoxicity even when treated in high concentrations which is comparable to the untreated control group, suggesting that developed drug carrier has low cytotoxicity and can be used in in-vivo studies with no concern on cells (). When cells were treated with different concentrations of free FU and FU-GO/NHs, a dose-dependent reduction in the viability of MCF7 cells was observed at both groups after 48 h, indicating that both formulations were effective against these cells. However, a shift to left in the cytotoxicity profile was observed which indicates that our drug carrier was successful in decreasing the IC50 of FU. FU is a widely used chemotherapeutic agent which is being administered for the treatment of many cancers for a long time, however, treatment with this agent is associated with severe toxic effects to healthy tissues which is directly dependent on drug concentration. Therefore, investigating new approaches for decreasing the drug concentration with no effect on its efficiency is a big deal. In this study, we observed the reduced IC50 value of FU when is loaded to GO/NHs, suggesting that the developed drug carrier can decrease the toxic side effects of FU to healthy tissues with no change in its efficiency in cancer cells.
Cell internalization evaluation by fluorescence microscopy and flowcytometry
Rhodamine B isothiocyanate (Rhd-B) as a fluorescent agent provides a label for following cellular uptake of the NHs by fluorescence microscopy. Rho B was adsorbed on the surface of GO sheets via π–π stacking, hydrophobic, and hydrogen-bonding interactions. To confirm the whether the uptake of the Rho-labeled GO/NHs (rh-GO/NHs) was time-dependent endocytosis, the MCF7 cells were treated with rh-GO/NHs for 1 and 2 h. As shown in , rh-GO/NHs could easily internalize into the MCF7 cells which were observed by fluorescent microscopy. As this technique is a qualitative analysis, flow-cytometry analysis as a quantitative technique was used for determination of cellular uptake percentages of rh-GO/NHs into the MCF7 cells. As seen in , in the first one and two hours all GO/NHs were internalized into the cells with 100% with different fluorescent intensity. The results showed that the amount of uptake was increased by over time. Both uptake analyses were confirmed that GO/NHs as a drug carrier could internalize easily into the cells which suggesting that GO/NHs has good capability to permeate into the cells and release the loaded drug.
Apoptosis evaluation by DAPI staining
Nuclear DAPI staining was done and cells were observed under fluorescent microscopy to confirm that apoptosis was occurring. Control cells and treated cells with GO/NHs were found to have an intact nuclear structure with normal morphology. As seen in , cells were homogenously stained which showed that the chromatin was equally distributed in the nucleolus. Furthermore, the population of the treated cells with GO/NHs are relatively equal with control cells revealing no toxicity of the GO/NHs as a nanocarrier on MCF7 cells. Whereas, the cells treated with free FU and FU-GO/NHs exhibit more apparently apoptotic cells and the signal of cell apoptosis (chromatin condensation, nucleolus pyknosis, and nuclear fragmentation) was observed. In addition, a population of the cells in both treated groups were significantly decreased and the apoptotic bodies were subsequently observed more often in the FU-GO/NHs group, indicating that FU-GO/NHs could induce apoptosis in MCF7 cells. These results persuaded us to determine the expression of proteins involved in apoptosis process in order to suggest a possible mechanism for apoptosis-inducing effects of formulations.
Protein expression
The effect of various formulations on protein expression levels of p53, Bcl-2, Bax, PARP and cleaved PARP was evaluated by western blot analysis. Our results showed that treatment of MCF7 cells with FU and FU-GO/NHs for 48 h, increased the expression of p53 and cleaved PARP significantly (), while had no substantial effect on the expression levels of antiapoptotic protein Bcl-2 and proapoptotic protein Bax (). FU-GO/NHs increased the expression of p53 and cleaved PARP more than free FU. Moreover, GO/NHs as a blank nanocarrier had no significant effect on the expression levels of p53, Bcl-2, Bax, PARP, and cleaved PARP. As observed in the results of DAPI staining, cells treated with FU and FU-GO/NHs showed more apoptotic cells in comparison to untreated control group and cells treated with GO/NHs. Apoptosis has two main pathways: external pathway and internal mitochondrial pathway. Mitochondrial pathway occurs after cellular stress and DNA damage. Following DNA damage and fragmentation, p53 is being activated which further upregulates p21 that prevent cell cycle improvement and allows damaged DNA to be corrected by repair mechanisms. P53 also enhances the expression of proapoptotic proteins like Bax and Bak which are permeable across mitochondrial membrane and cause cytochrome c efflux into the cytoplasm which is the initiator of internal apoptosis pathway. Results of DAPI staining showed higher DNA fragmentation in cells treated with FU-GO/NHs in comparison to corresponding free FU and cells treated with GO/NHs. This observation was further confirmed by western blot analysis as we see higher levels of p53 in cells treated with FU-GO/NHs, indicating that p53 has begun the apoptosis process. PARP is an enzyme involved in DNA repair which is activated after facing with damaged DNA. This enzyme can be cleaved by caspases and the presence of cleaved PARP is an indicator of apoptosis. Our results showed the higher concentration of cleaved PARP after treatment with FU and more significantly with FU-GO/NHs which indicates the initiation of the caspase cascade. On the other hand, we did not observe significant changes in Bax and Bcl-2 expression and Bax/Bcl-2 expression ratio. It is probable that the permeabilization of the mitochondrial membrane has occurred by other proapoptotic proteins such as Bak or overexpression of proapoptotic facilitator proteins such as Bid, Bad, PUMA and Noxa, which have not analyzed in this study.
Conclusion
To improve the efficiency of FU as an anticancer agent, a drug carrier based on graphene oxide were designed and used for delivery to the MCF7 breast cancer cells. After loading FU to GO/NHs, in-vitro drug release showed that drug carrier has temperature and pH-responsive properties. The biocompatibility of prepared GO/NHs has evaluated and results showed good compatibility with human red blood cells. Cellular uptake studies were done to confirm the capability of GO/NHs internalization into the cells. The cytotoxicity assay revealed that FU-GO/NHs could decrease the IC50 of FU in MCF7 cells, indicating that the drug carrier decrease the treatment dose of FU with no effect on its efficiency. Furthermore, DAPI staining was done to show the apoptotic effects of FU-GO/NHs to cells in comparison to FU indicating that FU-GO/NHs could induce apoptosis in MCF7 cells. Finally, the effect of various formulations on protein expression levels of p53, Bcl-2, Bax, PARP and cleaved PARP was evaluated by western blot analysis.
Disclosure statement
The authors report no conflicts of interest.
References
- Siegel RL, Miller KD, Jemal A. Cancer statistics, 2015. CA Cancer J Clin. 2015;65:5–29.
- Miller KD, Siegel RL, Lin CC, et al. Cancer treatment and survivorship statistics, 2016. CA Cancer J Clin. 2016;66:271–289.
- Assi T, El Rassy E, Tabchi S, et al. Treatment of cancer patients in their last month of life: aimless chemotherapy. Support Care Cancer. 2016;24:1603–1608.
- Group EBCTC. Effects of chemotherapy and hormonal therapy for early breast cancer on recurrence and 15-year survival: an overview of the randomised trials. The Lancet. 2005;365:1687–1717.
- Sharp III TE, George JC. Stem cell therapy and breast cancer treatment: review of stem cell research and potential therapeutic impact against cardiotoxicities due to breast cancer treatment. Front in Oncol. 2014;4:299.
- Zhang R, Fan Q, Yang M, et al. Engineering melanin nanoparticles as an efficient drug–delivery system for imaging‐guided chemotherapy. Adv Mater. 2015;27:5063–5069.
- Wang Y, et al. ZnO‐functionalized upconverting nanotheranostic agent: multi‐modality imaging‐guided chemotherapy with on‐demand drug release triggered by pH. Angew Chem Int Ed Engl. 2015;54:536–540.
- Li S-Y, Sun R, Wang H-X, et al. Combination therapy with epigenetic-targeted and chemotherapeutic drugs delivered by nanoparticles to enhance the chemotherapy response and overcome resistance by breast cancer stem cells. J Control Release. 2015;205:7–14.
- Vahed SZ, et al. Liposome-based drug co-delivery systems in cancer cells. Mater Sci Eng C. 2017;71:1327–1341.
- Quinn JF, Whittaker MR, Davis TP. Glutathione responsive polymers and their application in drug delivery systems. Polym Chem. 2017;8:97–126.
- Shafiei-Irannejad V, et al. Reversion of multidrug resistance by co-encapsulation of doxorubicin and metformin in poly (lactide-co-glycolide)-d-α-tocopheryl polyethylene glycol 1000 succinate nanoparticles. Pharm Res. 2018;35:119.
- Zeynabad FB, Salehi R, Mahkam M. Design of pH-responsive antimicrobial nanocomposite as dual drug delivery system for tumourtumour therapy. Appl Clay Sci. 2017;141:23–35.
- Rahimi M, Shafiei-Irannejad V, Safa KD, et al. Multi-branched ionic liquid-chitosan as a smart and biocompatible nano-vehicle for combination chemotherapy with stealth and targeted properties. Carbohydr Polym. 2018;196:299–312.
- Rahimi M, Safa KD, Salehi R. Co-delivery of doxorubicin and methotrexate by dendritic chitosan-g-mPEG as a magnetic nanocarrier for multi-drug delivery in combination chemotherapy. Polym Chem. 2017;8:7333–7350.
- Yang K, Feng L, Liu Z. Stimuli responsive drug delivery systems based on nano-graphene for cancer therapy. Adv Drug Deliv Rev. 2016;105:228–241.
- Nanda SS, Papaefthymiou GC, Yi DK. Functionalization of graphene oxide and its biomedical applications. Crit Rev Solid State Mater Sci. 2015;40:291–315.
- Liu J, Cui L, Losic D. Graphene and graphene oxide as new nanocarriers for drug delivery applications. Acta Biomater. 2013;9:9243–9257.
- Huskić M, Bolka S, Vesel A, et al. One-step surface modification of graphene oxide and influence of its particle size on the properties of graphene oxide/epoxy resin nanocomposites. Eur Polym J. 2018;101:211–217.
- Peng S, Liu C, Fan X. Surface modification of graphene oxide by carboxyl-group: preparation, characterization, and application for proteins immobilization. Integr Ferroelectr. 2015;163:42–53.
- Namvari M, Biswas CS, Wang Q, et al. Crosslinking hydroxylated reduced graphene oxide with RAFT-CTA: a nano-initiator for preparation of well-defined amino acid-based polymer nanohybrids. J Colloid Interface Sci. 2017;504:731–740.
- Torchilin VP. Multifunctional, stimuli-sensitive nanoparticulate systems for drug delivery. Nat Rev Drug Discov. 2014;13:813.
- Zhao Y, Zhou Y, Wang D, et al. pH-responsive polymeric micelles based on poly (2-ethyl-2-oxazoline)-poly (D, L-lactide) for tumour-targeting and controlled delivery of doxorubicin and P-glycoprotein inhibitor. Acta Biomater. 2015;17:182–192.
- Guo H-L, Wang X-F, Qian Q-Y, et al. A green approach to the synthesis of graphene nanosheets. ACS Nano. 2009;3:2653–2659.
- Rahimi M, Karimian R, Mostafidi E, et al. Highly branched amine-functionalized p-sulfonatocalix [4] arene decorated with human plasma proteins as a smart, targeted, and stealthy nano-vehicle for the combination chemotherapy of MCF7 cells. New J Chem. 2018;42:13010–13024.
- Shafiei‐Irannejad V, et al. Metformin enhances doxorubicin sensitivity via inhibition of doxorubicin efflux in P‐gp‐overexpressing MCF‐7 cells. Chem Biol Drug Des. 2018;91:269–276.
- Rahimi M, Safa KD, Alizadeh E, et al. Dendritic chitosan as a magnetic and biocompatible nanocarrier for the simultaneous delivery of doxorubicin and methotrexate to MCF-7 cell line. New J Chem. 2017;41:3177–3189.
- Yde CW, Issinger O-G. Enhancing cisplatin sensitivity in MCF-7 human breast cancer cells by down-regulation of Bcl-2 and cyclin D1. Int J Oncol. 2006;29:1397–1404.
- Rahimi M, Shojaei S, Safa KD, et al. Biocompatible magnetic tris (2-aminoethyl)amine functionalized nanocrystalline cellulose as a novel nanocarrier for anticancer drug delivery of methotrexate. New J Chem. 2017;41:2160–2168.