Abstract
A variety of surface modification methods are applied to modify titanium implants to improve their biological activity. Micro-arc oxidation (MAO) can relatively simply and efficiently produce porous coatings with high bioactivity and bond strength on titanium surfaces. However, there is no conclusion about the effect of coatings with different pore sizes produced by MAO on bone marrow mesenchymal stem cells (BMSCs). To study the effect of different pore sizes on BMSCs, rat BMSCs were applied to detect the effect of different pore sizes prepared by MAO on cell adhesion and osteogenic differentiation. Three groups of coatings with different pore sizes were successfully prepared, and the pore size was within the range of 3–10 µm. Importantly, the expression of adhesion-related protein integrin β1 and osteogenic-related proteins OSX and ALP increased along with the increase in pore size. This study showed that the porous coating prepared by MAO promotes BMSCs adhesion and osteogenic differentiation. It reveals that the pore size is in the range of 3–10 µm and the larger pores are more beneficial for BMSCs adhesion and osteogenic differentiation. This study is instructive for optimizing the design of biomedical implant surfaces.
Introduction
Since Professor Brånemark found pure titanium had good biocompatibility and then put forward the theory of osseointegration, titanium (Ti) has been applied as a good dental implant material [Citation1,Citation2]. However, there are some problems with Ti-based implant materials, such as lack of osteoinduction and high elastic modulus, which require more time to achieve the effect of satisfactory osseointegration [Citation3]. However, these disadvantages of Ti hinder its development as dental implants to some extent. The interaction between the implant and the surrounding bone tissue first occurs on the surface of the implant, therefore, it is necessary to modify the surface of the implant to solve the problems in titanium and its alloys [Citation4,Citation5].
A variety of surface modification techniques have been applied to improve the surface properties of titanium and its alloys, including physical vapour deposition, sol-gel, ion implantation, anodic oxidation and micro-arc oxidation (MAO) [Citation6–10]. MAO is a relatively simple and efficient technology for the growth of porous oxide ceramic coatings with high bonding strength in situ, and it has been widely applied in the surface modification of Ti and its alloys [Citation11,Citation12]. In addition, the porous coating on Ti prepared by MAO possesses a large number of pores on the surface, which can significantly reduce the elasticity modulus of Ti [Citation13–15]. These pore structures not only decrease the stress concentration of the implant but also guide the growth of bone tissue and increase the mechanical locking force [Citation16,Citation17]. Therefore, a porous structure coating prepared by MAO can optimize the osteoinduction and reduce elastic modulus.
The pore structure not only increases the surface area of the titanium implants but also increases the contact area between the implant and the host bone, and more importantly, its porous structure provides a suitable microenvironment for the growth of cells such as osteoblasts and bone marrow mesenchymal stem cells (BMSCs) [Citation18]. MAO can be applied to change the pore structure of the coating by adjusting parameters, such as the time parameter, that can stably regulate the pore size of the coating [Citation19]. Studies [Citation14,Citation20] showed that with increase in reaction time, the pore size of the coating increased, while the number of pores decreased. In addition, it has been reported that more than 15 min of oxidation time would lead to cracks, which would affect the bond strength of the coating to the base metal [Citation21,Citation22]. Therefore, to prepare coatings with better biological activity and better bond strength, we limited the reaction time to 15 min.
In addition, porous structure shows better biocompatibility than flat implants [Citation23]. Studies have shown that the pore size specificity affects the cell shape, differentiation and extracellular matrix secretion [Citation24,Citation25]. Pores with a size of ∼10 μm can promote bone induction protein adsorption, ion exchange, bone-like apatite formation, and osteoblast adhesion, proliferation and differentiation [Citation26]. Unfortunately, the effect of different pore size coatings prepared by MAO on BMSCs has not been conclusively revealed. Therefore, we prepared porous coatings with different pore sizes on the surface of pure titanium by adjusting the oxidation time of MAO and evaluated the influence of different pore sizes on the adhesion and osteogenic differentiation activities of rat BMSCs to optimize the surface modification designs of titanium implants.
Material and methods
Sample preparation and modification
The pure titanium plates (length and width: 20 mm, thickness: 2 mm) were polished sequentially with 320, 800 and 1200 grit SiC abrasive sandpapers. Then, the titanium plates were cleaned ultrasonically in acetone, ethanol and deionized water, each for 5 min. The micro-arc oxidation (MAO) electrolyte was composed of 0.1 M calcium acetate monohydrate (Ca(CH3COO)2·H2O) and 0.03 M sodium phosphate dibasic dihydrate (NaH2PO4·2H2O). The voltage and frequency of the pulsed AC power were 420 V and 50 Hz, respectively. The time parameters of MAO were set as 0 min, 5 min, 10 min and 15 min, and they were recorded as group Ti, group MAO-1, group MAO-2 and group MAO-3, respectively.
Material characteristics
The surface morphology of the samples was observed by a scanning electron microscope (SEM, SEMS-3400N, Japan). The surface phase composition of the samples was analyzed by X-ray diffraction (XRD, Rigaku, D/max-2550VB/PC, Japan), and an Energy-dispersive X-ray spectroscopy (EDS, EDAX, USA) was used to determine the elemental composition of the samples. ImageJ software (National Institutes of Health, USA) was used to analyze the percentage of the pore size of the samples, and the samples’ roughness was measured by the Perthometer M1 (Marl, Germany) portable roughness measuring instrument.
Preparation of extraction solution
The extract solution was prepared according to the International Standard Organization (ISO/EN10993-5). Briefly, the coating alloys were immersed in Dulbecco's modified Eagle medium (DMEM, Gibco, Grand Island, NY, USA) at a concentration of 3 cm2/mL. The extract solutions were collected.
Cell culture
The tibia and femur of 4-week-old male SPF SD rats (provided by the animal centre of the Ninth People's Hospital, Shanghai JiaoTong University School of Medicine) were used as the source of BMSCs. The rats were placed in alcohol for three minutes after sacrificed by spinal dislocation. Bone marrow was obtained by rinsing the bone marrow cavity with DMEM medium containing 1% penicillin-streptomycin (Thermo Fisher Scientific, USA) and 10% fetal bovine serum (Every green, Zhejiang Tianhang Biotechnology, China) after cutting off the epiphysis at both ends of the bone. The collected bone marrow washing fluid was centrifuged at 1000 rpm for 10 min. The cells were cultured with DMEM in incubators containing 5% CO2 at 37 °C. Passage 2 to passage 4 of the cells were used in this study.
Cell adhesion and extension
To observe the adherence of BMSCs on the four samples, we detected the gene and protein expression of the integrin β1 by immunofluorescence staining and Bio-Rad Quantitative Real-Time PCR system (qRT-PCR; Bio-Rad, MyiQTM, USA), in addition, we counted the number of cells adherent to the sample surface after seeding on the samples for 4 h. The protocols of immunofluorescence staining were as follows: after seeding on the four samples for 4 h, the BMSCs were fixed by 4% paraformaldehyde (PFA) at room temperature for 20 min. Anti-integrin β1 (Abcam, UK, 1:200 dilution) antibody was incubated with the samples at 4 °C overnight after adding 5% bovine serum albumin (BSA) for 1 h. Then, the BMSCs were stained for 1 h by Alexa Fluor 594 Goat Anti-Rabbit IgG (H + L) (Proteintech, USA, 1:200 dilution), and the nuclei were stained with 4',6-diamidino-2-phenylindole (DAPI, Sigma, USA, 1 µg/mL) for 5 min. The number of cells was counted by Image-Pro Plus software (Media Cybernetics, USA). To observe the cells extension, the cells were seeded on samples for 4 h, and β-actin was stained with FITC-phalloidin (Yeasen, China, 1:200 dilution) for 20 min. The extension area of cells was measured by ImageJ. The area of cell fluorescence is expressed as total area/cell number.
Cell activity and proliferation experiment
BMSCs were seeded on the four group samples, and 24 h later, the Calcein-AM/PI Double Stain Kit (Yeasen, China) was applied to detect the cells’ viability. In addition, after seeding on four group samples for 12 h, the EdU solution with a final concentration of 50 μM (DMEM matching, Ribobio, China) was added to the BMSCs for 12 h. Then, the cells were fixed by 4% PFA for 20 min, while cells were permeabilized for another 20 min. Next, the cells were stained with Apollo solution of Cell-Light™ EdU Apollo®567 In Vitro Imaging Kit (Ribobio, China) for 30 min at room temperature. Then, DAPI was used to stain the nuclei for 5 min.
Effect of extract on BMSCs
The extract was used to culture the cells to detect the effect of the extract on the osteogenic differentiation of the BMSCs. 7 days later, ALP staining and semi-quantitative detection were carried out. The detailed protocols of the experiment were as described in section ALP activity and calcium deposition. In addition, the expression of the osteogenic-related genes osterix (OSX) and ALP was measured using the qRT-PCR. The detailed protocols of the experiment were described in section Expression of osteogenic-related genes and proteins.
ALP activity and calcium deposition
BMSCs were seeded on the four different samples for 7 days, then, cells on the substrates were fixed by 4% PFA and stained by an alkaline phosphatase kit (Beyotime, China). For the quantitative ALP assay [Citation18], the cell lysates were incubated with p-nitrophenyl phosphate (p-NPP) (Sigma, USA) at 37 °C for 30 min. ALP activity was tested by detecting the optical density (OD) values at 405 nm, and total protein content was assessed using a Bio-Rad protein assay kit (Bio-Rad, USA) with OD measured at 630 nm and normalized with a series of BSA (Sigma, USA) standards. The ALP activity was finally presented as OD values per milligram of total proteins at 405 nm.
After 3 days, the BMSCs were inoculated on four samples cultured with DMEM, and the DMEM was replaced by osteogenic induction medium. After 21 days, the BMSCs on the substrates were fixed by 4% PFA and stained by 0.1% alizarin red S (ARS). The staining results were observed under an optical microscope. For the quantitative assay, the stained cells were desorbed with 10% cetylpyridinium chloride (Sigma, USA), and the OD values were detected at 590 nm. Calcium deposition results were normalized to the total protein content and expressed as OD values at 590 nm per milligram of total cellular protein.
Expression of osteogenic-related genes and proteins
BMSCs were cultured on the substrates for 7 days, and the total RNA was extracted with TRIzol reagent (Invitrogen, Thermo Fisher Scientific, USA). The cDNAs were generated using a PrimeScript 1st Strand cDNA Synthesis kit (Takara, Japan). The expression of osteogenic-related genes ALP, OCN, OSX and Runx2 was detected by qRT-PCR. The housekeeper gene glyceraldehyde-3-phosphate dehydrogenase (GAPDH) was used for standardization. The sequences of the specific primers used in this study were listed in provided by Sangon Biotech, China. After seeding on the four samples for 7 days, OSX immunofluorescence staining was carried out with reference to immunofluorescence staining protocols of Integrin β1. The primary and secondary antibodies were OSX (Abcam, UK, 1:1000 dilution) and TRITC goat anti-rabbit IgG (H + L) (Proteintech, USA, 1:200 dilution), respectively.
Table 1. Primers for qRT-PCR.
Statistical analysis
All the data from this study were presented as the mean ± standard deviation and analyzed in GraphPad Prism (GraphPad Software, USA). Variance analysis (ANOVA) was used to detect the differences among the four groups [Citation18,Citation27,Citation28]. Differences were considered statistically significant at p < .05, p < .01 and p < .001.
Results
Surface morphology
As shown in , compared with group Ti, there are a large number of micropore structures on the surface of MAO-1, MAO-2 and MAO-3. Below the SEM images, we can see that the frequency distribution of the pore size. The roughness results () showed that the surface roughness of the MAO-treated material increased significantly, but there was no significant difference among the 3 groups. As shown in , the pore size of the MAO-treated material was between 3 and 10 µm; the pore size of the MAO-1 group was the smallest, while for the MAO-3 group, it was the largest. There was also a significant difference among the 3 groups in terms of pore size. The above results showed that the roughness change was not obvious with the prolongation of the oxidation time, but the pore size increased gradually in the range of 3–10 µm.
Figure 1. (a) Scanning electron microscopic images of four sample surfaces and frequency distribution of the pore size. (b) The roughness of the four samples. (c) The pore size of the four samples. (***p < 0.001 compared with Ti; &p < .05 compared with MAO-1; #p < .05 compared with MAO-1 and MAO-2; n = 3).
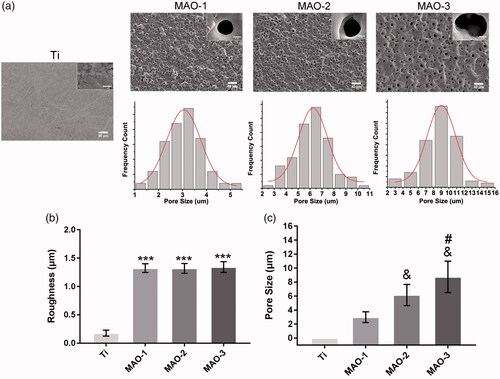
Material characteristics
We analyzed the crystallographic structure of the material surface by XRD and found that the peaks of Ti decreased while new peaks appeared after MAO treatment. After the analysis by jade6.0 software, we found that anatase TiO2 () appeared on the surface of the MAO-treated material; moreover, it was the common crystal phase among the three MAO-treated groups. The element composition of the material surface was further analyzed by EDS (). It was found that the MAO-treated materials contained four elements: Ti, O, Ca and P. There was no significant difference in the ratio of Ca and P among the three MAO-treated groups ().
Cell adhesion and extension
The extension of BMSCs on the four group materials was observed under a fluorescence microscope (). It can be seen that BMSCs adhered to the surface of the four samples for the initial 4 h. As shown in , it was found that the cytoskeleton on the surface of the MAO-treated materials showed better extension compared with the Ti group. The adhesion ability of the BMSCs was detected by the gene and protein expression of integrin β1. The mRNA expression of integrin β1 was detected by qRT-PCR. Compared with the Ti group, the mRNA expression of integrin β1 was significantly up-regulated among the MAO-treated groups (). Obviously, the expression of integrin β1 protein on the surface of the MAO-treated materials was significantly higher than that in the Ti group (). The number of cells initially adhered to the material was detected to further test its cell adhesion activity. More BMSCs adhered to the surface of the MAO-treated materials during the initial 4 h (), and the number of adherent cells in the group MAO-3 was the largest (). The above results showed that the porous surface coating has a promotional effect on cell adhesion. Moreover, it had a stronger effect on the cell adhesion of the MAO-3 group with a larger pore size.
Figure 4. Cell adhesion and extension assay. (a) BMSCs were stained by FITC-phalloidin and DAPI after incubation for 4 h on four samples. Scale bar =50 µm (b) Expression of integrin β1 detected by fluorescence microscope after incubation for 4 h. Scale bar =10 µm (c) Cell nuclei stained with DAPI were imaged by fluorescence microscope for the four samples 4 h after seeding. Scale bar =500 µm (d) The average area of cell extension was determined by ImageJ. (e) After 4 h of incubation on the four samples, expression of integrin β1 was measured by qRT-PCR. (f) Statistical results of cell adhesion on sample surfaces. (*p < .05 and **p < .01 compared with Ti; #p < .05 compared with Ti, MAO-1 and MAO-2; n = 3).
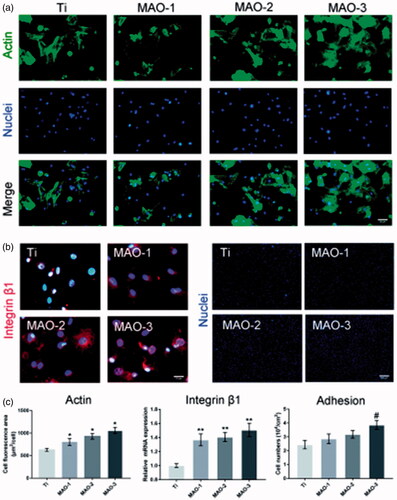
Cell activity and proliferation
As shown in , the cytoplasm of viable cells was stained with green by Calcein-AM and the nuclei of dead cells were stained with red by PI. As shown in , there was no significant difference between the four groups, which indicated that there was no significant difference in cytotoxicity between the MAO-treated groups and the Ti group. The proliferation of BMSCs on different materials was detected by EdU staining. It was not the different proliferation effect of BMSCs on different materials in the initial 12 h. Compared with the Ti group, the proliferation effect of the MAO groups was better (). The statistical results showed that the MAO-2 and MAO-3 groups () had significant differences compared with the Ti group. From the above results, the porous morphology coating promoted cell proliferation without inhibiting cell viability during the initial 12 h.
Figure 5. Cell viability and proliferation assay. (a) After 24 h of culture, a live/dead assay was observed under the fluorescence microscope. Scale bar =100 µm (b) After 12 h of incubation, the proliferation ability was measured by EdU stain under the fluorescence microscope. Scale bar =100 µm (c) Statistical results for live/dead assay. (d) Statistical results of the percentage of the cells stained by EdU. (*p < .05 compared with Ti; n = 3).
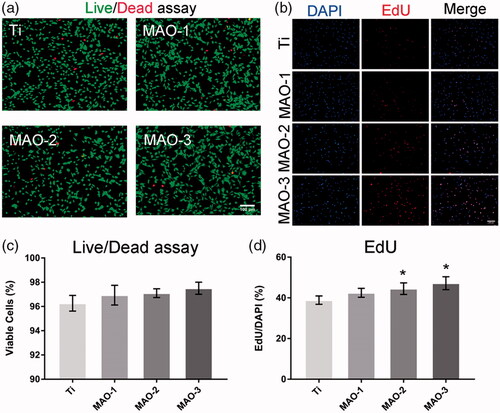
Effect of extract solution on osteogenic differentiation of BMSCs
The results of ALP staining () and quantitative detection () showed that the MAO-treated groups were significantly stronger than the Ti group, but there was no significant difference among the MAO-treated groups. In addition, qRT-PCR was used to detect the osteogenic related genes ALP and OSX. The results showed that the extract from the MAO-treated groups could promote osteogenic differentiation of BMSCs; however, there was no significant difference among the MAO-1, MAO-2 and MAO-3 groups (). The above results showed that the ionic components of MAO-1, MAO-2 and MAO-3 promoted the osteogenic differentiation of BMSCs, but there was no significant difference among the 3 groups.
Effect of material on osteogenic differentiation
We examined the effects of materials on the osteogenic differentiation of BMSCs by ALP staining, calcium deposition and immunofluorescence staining. The results showed that the positive areas of ALP staining in MAO-treated groups were larger than in the Ti group (); moreover, the MAO-3 group showed the best results. ALP quantitative results as shown in indicated that the MAO-3 group was significantly stronger than the other groups, and there was a significant difference between the MAO-3 group and other groups. The ARS and quantitative results were similar to those of ALP (). OSX is a specific nuclear transcription factor associated with osteogenesis, and it is also one of the important transcription factors in regulating osteogenic differentiation. It was found that OSX was expressed in the nucleus (), and there was a difference in the degree of expression among the four samples. The expression level of OSX in the MAO-treated groups was significantly higher than that in the Ti group. Moreover, the expression level in the MAO-3 group was higher than that in the other three groups. qRT-PCR results showed that OSX and ALP expression in the MAO-3 group was significantly higher than in the other three groups, while OCN and Runx2 expression in the MAO-2 group and MAO-3 group was significantly higher than in the Ti group (). The above results indicate that the expression of osteogenic genes and proteins was up-regulated in response to an increase in pore size.
Figure 7. Alkaline phosphatase activity and calcium deposition assay. (a) BMSCs on the four sample surfaces were stained with an alkaline phosphatase kit on the 7th day. After 21 days of osteogenic induction, BMSCs on the four samples were stained with ARS. (b) Alkaline phosphatase activity of BMSCs was measured with a quantitative assay. (c) Calcium deposition status of the cells was assayed using colourimetric quantitative analysis. (#p < .05 compared with Ti, MAO-1 and MAO-2).
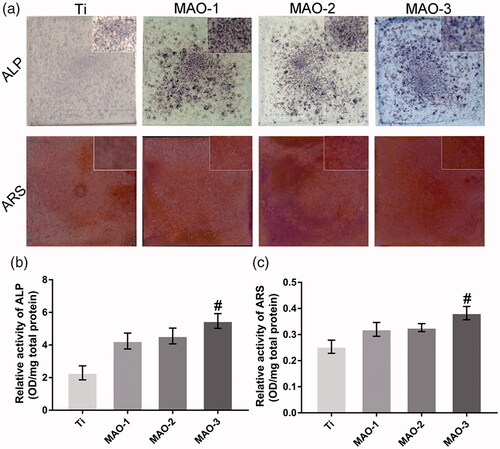
Figure 8. Cell differentiation assay. After 7 days of culture, expression of OSX protein was detected by immunofluorescence (a), and expression of osteogenic-related genes (OSX, ALP, OCN and Runx2) was measured by qRT-PCR (n = 3) (b). Scale bar =100 µm (#p < .05 compared with Ti, MAO-1 and MAO-2; *p < .05 compared with Ti).
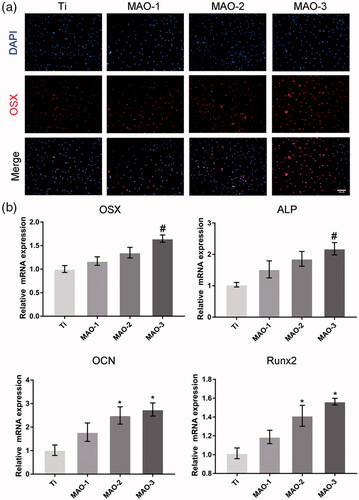
Discussion
In this study, porous surface coatings were prepared on pure titanium by MAO, which is a relatively simple technology. We adjusted the oxidation time to prepare three different pore sizes of coating on the pure titanium surface. It has been observed that the surface of the material shows a similar porous morphology, and there is only a difference in pore size. In addition, the coatings are almost the same in terms of the roughness, crystal phase and surface chemical properties of the MAO-treated groups. Therefore, we can independently evaluate the effect of pore size on rat BMSCs.
The time parameter of MAO has a great influence on the morphology of the coating [Citation20]. When increasing the reaction time, the pore size, the roughness and the crystalline phase will change correspondingly [Citation12]. Previous studies have shown that the porous coating prepared by adjusting the parameters of MAO could promote cell adhesion and osteogenic differentiation [Citation29,Citation30]. However, the effect of different pore sizes prepared by MAO on cells has not previously been reported. Therefore, we adjusted the time parameters to prepare coatings with different pore sizes. As shown in , we successfully prepared three groups of coatings with different pore sizes on the titanium surface. It can be seen that the pore size of the coating is on a micro scale. Micro/nanoscale morphologies can significantly affect protein absorption and regulate cell adhesion, proliferation, differentiation and mineral deposition [Citation4,Citation26]. It was found that the pore size was different among the MAO-treated three groups, while there was no difference in the roughness. The XRD results showed that the crystal structure of all of the MAO-treated three groups was anatase TiO2. Anatase nanotubes have good biological activities such as antibacterial, accelerating mineralization and increasing protein adsorption. Anatase TiO2 not only promotes the production of bioactive calcium phosphate [Citation31,Citation32] but also reduces the adhesion of Streptococcus oris [Citation33].
In the process of osseointegration, the direct contact between implants and BMSCs has been widely accepted [Citation34]. BMSCs exist in bone marrow and other mesenchymal tissues and have the ability of self-renewal and multidirectional differentiation; they can migrate to the injured site or implant site and participate in subsequent osseointegration [Citation35]. It was found that the surface morphology of different materials will directly affect the adhesion of BMSCs [Citation4]. Zhang et al.[Citation36] found that the porous TiO2 coating prepared by MAO is beneficial to the initial adhesion of osteoblast-like cells. When BMSCs participate in the process of osseointegration, the adhesion of cells to the material surface is the first step of the interaction between cells and materials, and moreover, a lack of adhesion may affect the subsequent cell osteogenic differentiation and osseointegration [Citation37]. In general, cell adhesion mainly consists of cell-cell adhesion and cell-ECM adhesion, and the cell-ECM adhesion is mediated by integrins [Citation26]. Integrin β1 participates in the adhesion of osteoblasts to various extracellular matrix proteins, and therefore, it can be regarded as a representative when studying the adhesion mechanisms of osteoblasts and extracellular matrix [Citation18]. Through qRT-PCR and immunofluorescence, we detected the expression of integrin β1, and we found that the MAO group significantly promoted the expression of the integrin β1 gene and protein. In addition, we observed that the number of adhesive cells in the MAO-3 group was significantly higher than that in the other three groups. These results suggest that a porous coating can promote the adhesion of BMSCs and that a larger pore coating is more conducive to the initial adhesion of cells.
The ideal titanium surface not only supports cell growth but also induces osteogenic differentiation to promote rapid osseointegration [Citation38,Citation39]. We detected the expression of bone-related OSX protein by immunofluorescence. It was found that the expression of osteogenic-related protein OSX on the surface of the MAO-treated materials was significantly higher than that in the Ti group, and its levels were higher in the MAO-3 group than in the other groups. The results of ALP staining and calcium deposition showed that the surface colour of the three groups was deepened, and the colour of the MAO-3 group was the deepest. These results indicate that BMSCs are more conducive to osteogenic differentiation with increases in pore size. The qRT-PCR results also showed that the expression of osteogenic related genes increased with increases in pore size. These results indicate that the coatings prepared by MAO promote the osteogenic differentiation of BMSCs, and the effect of the MAO-3 group on osteogenic differentiation is better. There was less difference in the effect of material extracts among the three MAO-treated groups on the osteogenic differentiation of BMSCs, while there was a difference for the MAO coating; however, only the pore size of the coating was different. Thus, we think that pore size plays an important role in the process of cell osteogenic differentiation. The above results indicate that the MAO-3 group with a larger pore size coating had a better effect on osteogenic differentiation of BMSCs.
This study showed that the porous coatings prepared by MAO not only improved the inertness of the titanium-based materials but also had good biocompatibility. This study showed that the pore size of the MAO coating was within the range of 3–10 µm, and meanwhile, a larger pore size coating was more conducive to adhesion and osteogenic differentiation of BMSCs. However, the mechanism of osteogenic differentiation of BMSCs with different pore sizes needs further study.
Conclusions
In this study, three groups of coatings with different pore sizes were prepared on the surface of pure titanium by adjusting the time parameters of micro-arc oxidation technology. The cell experimental results showed that the porous coating behaved good biocompatibility and promoted the osteogenic differentiation of BMSCs. In addition, we prepared coatings on titanium surfaces with a pore size range of 3–10 µm, and our results showed that a larger pore size was more conducive to adhesion and osteogenic differentiation of BMSCs. The results also provide a reference for optimizing the design of biomedical implant surfaces.
Disclosure statement
No potential conflict of interest was reported by the authors.
Additional information
Funding
References
- Branemark PI, Hansson BO, Adell R, et al. Osseointegrated implants in the treatment of the edentulous jaw. Experience from a 10-year period. Scand J Plast Reconstr Surg Suppl. 1977;16:1–132.
- Ekelund JA, Lindquist LW, Carlsson GE, et al. Implant treatment in the edentulous mandible: a prospective study on Branemark system implants over more than 20 years. Int J Prosthodont. 2003;16:602–608.
- Ogawa T, Nishimura I. Genes differentially expressed in titanium implant healing. J Dent Res. 2006;85:566–570.
- Zhang W, Li Z, Huang Q, et al. Effects of a hybrid micro/nanorod topography-modified titanium implant on adhesion and osteogenic differentiation in rat bone marrow mesenchymal stem cells. Int J Nanomedicine. 2013;8:257–265.
- Zhang W, Li Z, Liu Y, et al. Biofunctionalization of a titanium surface with a nano-sawtooth structure regulates the behavior of rat bone marrow mesenchymal stem cells. Int J Nanomedicine. 2012;7:4459–4472.
- Kaluderovic MR, Mandl S, Kohlweyer H, et al. Physical vapour deposition of zirconia on titanium: fabrication, characterization and interaction with human osteoblast cells. J Mater Sci Mater Med. 2015;26:267.
- Kaya S, Cresswell M, Boccaccini AR. Mesoporous silica-based bioactive glasses for antibiotic-free antibacterial applications. Mater Sci Eng C Mater Biol Appl. 2018;83:99–107.
- Kondyurin A, Lau K, Tang F, et al. Plasma ion implantation of silk biomaterials enabling direct covalent immobilization of bioactive agents for enhanced cellular responses. ACS Appl Mater Interfaces. 2018;10:17605–17616.
- Li B, Li Y, Li J, et al. Influence of nanostructures on the biological properties of Ti implants after anodic oxidation. J Mater Sci: Mater Med. 2014;25:199–205.
- Zhang Y, Xiu P, Jia Z, et al. Effect of vanadium released from micro-arc oxidized porous Ti6Al4V on biocompatibility in orthopedic applications. Colloids Surf B Biointerfaces. 2018;169:366–374.
- Khanna R, Kokubo T, Matsushita T, et al. Novel artificial hip joint: A layer of alumina on Ti-6Al-4V alloy formed by micro-arc oxidation. Mater Sci Eng C Mater Biol Appl. 2015;55:393–400.
- Li LH, Kong YM, Kim HW, et al. Improved biological performance of Ti implants due to surface modification by micro-arc oxidation. Biomaterials. 2004;25:2867–2875.
- Heinl P, Muller L, Korner C, et al. Cellular Ti-6Al-4V structures with interconnected macro porosity for bone implants fabricated by selective electron beam melting. Acta Biomater. 2008;4:1536–1544.
- Yao ZQ, Ivanisenko Y, Diemant T, et al. Synthesis and properties of hydroxyapatite-containing porous titania coating on ultrafine-grained titanium by micro-arc oxidation. Acta Biomater. 2010;6:2816–2825.
- Han Y, Chen D, Sun J, et al. UV-enhanced bioactivity and cell response of micro-arc oxidized titania coatings. Acta Biomater. 2008;4:1518–1529.
- Xiu P, Jia Z, Lv J, et al. Tailored surface treatment of 3d printed porous Ti6Al4V by microarc oxidation for enhanced osseointegration via optimized bone in-growth patterns and interlocked bone/implant interface. ACS Appl Mater Interfaces. 2016;8:17964–17975.
- Zhou J, Zhao L. Hypoxia-mimicking Co doped TiO2 microporous coating on titanium with enhanced angiogenic and osteogenic activities. Acta Biomater. 2016;43:358–368.
- Li G, Cao H, Zhang W, et al. Enhanced osseointegration of hierarchical micro/nanotopographic titanium fabricated by microarc oxidation and electrochemical treatment. ACS Appl Mater Interfaces. 2016;8:3840–3852.
- Song WH, Jun YK, Han Y, et al. Biomimetic apatite coatings on micro-arc oxidized titania. Biomaterials. 2004;25:3341–3349.
- Gu Y, Bandopadhyay S, Chen C-f, et al. Effect of oxidation time on the corrosion behavior of micro-arc oxidation produced AZ31 magnesium alloys in simulated body fluid. J Alloys Comp. 2012;543:109–117.
- Guo HF, An MZ, Huo HB, et al. Microstructure characteristic of ceramic coatings fabricated on magnesium alloys by micro-arc oxidation in alkaline silicate solutions. Appl Surf Sci. 2006;252:7911–7916.
- Guo H, An M, Xu S, et al. Formation of oxygen bubbles and its influence on current efficiency in micro-arc oxidation process of AZ91D magnesium alloy. Thin Solid Films. 2005;485:53–58.
- Li X, Xu H, Zhao B, et al. Accelerated and enhanced osteointegration of MAO-treated implants: histological and histomorphometric evaluation in a rabbit model. Int J Oral Sci. 2018;10:11.
- Karageorgiou V, Kaplan D. Porosity of 3D biomaterial scaffolds and osteogenesis. Biomaterials. 2005;26:5474–5491.
- Lee SJ, Choi JS, Park KS, et al. Response of MG63 osteoblast-like cells onto polycarbonate membrane surfaces with different micropore sizes. Biomaterials. 2004;25:4699–4707.
- Li Y, Xiao Y, Liu C. The horizon of materiobiology: a perspective on material-guided cell behaviors and tissue engineering. Chem Rev. 2017;117:4376–4421.
- Chuang CH, Lin RZ, Melero-Martin JM, et al. Comparison of covalently and physically cross-linked collagen hydrogels on mediating vascular network formation for engineering adipose tissue. Artif Cells Nanomed Biotechnol. 2018;1–14.Doi: 10.1080/21691401.2018.1499660.
- Cao L, Kong X, Lin S, et al. Synergistic effects of dual growth factor delivery from composite hydrogels incorporating 2-N,6-O-sulphated chitosan on bone regeneration. Artif Cells Nanomed Biotechnol. 2018;1–17.Doi: 10.1080/21691401.2018.1488721.
- Liu W, Chen D, Jiang G, et al. A lithium-containing nanoporous coating on entangled titanium scaffold can enhance osseointegration through Wnt/beta-catenin pathway. Nanomedicine. 2018;14:153–164.
- Gittens RA, McLachlan T, Olivares-Navarrete R, et al. The effects of combined micron-/submicron-scale surface roughness and nanoscale features on cell proliferation and differentiation. Biomaterials. 2011;32:3395–3403.
- Sollazzo V, Pezzetti F, Scarano A, et al. Anatase coating improves implant osseointegration in vivo. J Craniofac Surg. 2007;18:806–810.
- Hong Y, Yu M, Lin J, et al. Surface hydroxyl groups direct cellular response on amorphous and anatase TiO2 nanodots. Colloids Surf B Biointerfaces. 2014;123:68–74.
- Dizaj SM, Lotfipour F, Barzegar-Jalali M, et al. Antimicrobial activity of the metals and metal oxide nanoparticles. Mater Sci Eng C Mater Biol Appl. 2014;44:278–284.
- Li J, Song Y, Zhang S, et al. In vitro responses of human bone marrow stromal cells to a fluoridated hydroxyapatite coated biodegradable Mg-Zn alloy. Biomaterials. 2010;31:5782–5788.
- Hakelien AM, Bryne JC, Harstad KG, et al. The regulatory landscape of osteogenic differentiation. Stem Cells. 2014;32:2780–2793.
- Zhang R, Elkhooly TA, Huang Q, et al. A dual-layer macro/mesoporous structured TiO2 surface improves the initial adhesion of osteoblast-like cells. Mater Sci Eng C Mater Biol Appl. 2017;78:443–451.
- Zhang W, Wang G, Liu Y, et al. The synergistic effect of hierarchical micro/nano-topography and bioactive ions for enhanced osseointegration. Biomaterials. 2013;34:3184–3195.
- Le Guehennec L, Soueidan A, Layrolle P, et al. Surface treatments of titanium dental implants for rapid osseointegration. Dent Mater. 2007;23:844–854.
- Zhang W, Cao H, Zhang X, et al. A strontium-incorporated nanoporous titanium implant surface for rapid osseointegration. Nanoscale. 2016;8:5291–5301.