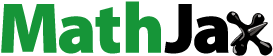
Abstract
The aim of this study was to evaluate anti-cancer properties of hesperetin (Hsp) and hesperetin-loaded poly(lactic-co-glycolic acid) nanoparticles (HspNPs) for glioblastoma treatment. Nanoparticles prepared by single emulsion method had a size of less than 300 nm with 70.7 ± 3.9% reaction yield and 26.4 ± 1.1% Hsp loading capacity. Treatment of C6 glioma cells with HspNPs for 24 and 48 h resulted in dose- and time-dependent decrease in cell viability, with approximate IC50 of 28 and 21 μg/mL, respectively (p = .036 for 24 h, p = .025 for 48 h). The percentage of PCNA positive cells decreased to 20% and 10%, respectively, for Hsp- and HspNP-treated cells at concentration of 100 µg/mL. Treatment with increasing concentrations of HspNPs (25, 50, 75 and 100 µg/mL) resulted in 9.1-, 7-, 12.5- and 12.7-fold in increase in apoptotic cell number. Optimum doses of Hsp and HspNPs were found to increase oxidative damage in C6 glioma cells. MDA levels, an indicator of lipid peroxidation, were found to be significantly elevated at 75 and 100 µg/mL exposure concentration of HspNPs with (p = .002) and (p = .018), respectively for 48-h treatment. The results obtained with this study showed biocompatible polymeric nanoparticle systems has great advantages to enhance anti-cancer activity and poor solubility of therapeutic agents. Overall our findings suggest that Hsp-loaded PLGA nanoparticle systems showed significant anti-cancer activity and HspNPs could be used as promising novel anti-cancer agent.
Introduction
Glioblastomas are one of the most lethal and common type of primary brain tumours which are known to affect 0.02% of population [Citation1,Citation2]. Although glioblastomas are rare tumours, its poor survival rate makes it an important health issue [Citation2]. The standard treatment approach of glioblastomas usually includes surgery followed chemotherapy. However, none of these treatments are considered efficient in managing this disease, resulting in a survival of less than 15 months [Citation3]. Also, drug resistance and toxic effects of chemotherapeutic agents suggest that chemotherapy is insufficient to combat this disease [Citation4]. Additionally, poor drug penetration due to physiological barriers such as the blood–brain barrier (BBB) and the blood–brain tumour barrier is another important limitation in treatment [Citation5]. Therefore, there is a great need to discover novel anticancer agents that have low toxicity and high efficiency for an effective glioblastoma treatment.
Dietary sources of natural products have been attract a great attention due to their potent anti-cancer activities [Citation6]. Flavonoids are a group of natural substances well known for their cell growth inhibiting capacity and anti-cancer properties [Citation7]. Most flavonoids modulate different set of enzymes and receptors in signal transduction pathways related to cell differentiation, proliferation apoptosis, inflammation, angiogenesis, metastasis and reversal of multidrug resistance [Citation8]. Hesperetin (5, 7, 3′-trihydroxy-4′methoxy flavanone) is a bioflavonoid, exhibits wide range of biological and pharmacological activities including antioxidant, anti-inflammatory, anti-hypertensive and antiatherogenic effects [Citation9–11]. Anti-cancer activities of hesperetin (Hsp) has been established in various cell lines including prostate, breast, cervical and colon cancers [Citation12–15]. Although Hsp could be used as a potential chemotherapeutic agent, short plasma half-life, hydrophobic nature and poor water solubility limits the potential of this molecule to be used as an active anti-cancer agent [Citation16,Citation17]. However, delivery of the active agent using nanoparticle systems could potentially overcome aforementioned limitations.
Nanoparticles (NPs) are widely used as drug carrier systems to enhance the efficacy and solubility of many therapeutic agents [Citation18]. Furthermore, NPs-mediated drug delivery is an effective and non-invasive system to treat cerebral diseases [Citation19]. Poly (lactic-co-glycolic acid) (PLGA) is one of the most successfully used biodegradable polymer approved by FDA with low toxicity, biodegradability, biocompatibility and most importantly a controllable and desired release profile properties [Citation20]. Moreover, in vitro and in vivo researches has established significance and success of PLGA in the neural drug delivery by studying the NPs cytotoxicity, the pharmaceutical efficacy of the drugs and brain uptake of PLGA NPs [Citation19]. Many hydrophobic drugs including natural bioflavonoids have been encapsulated in PLGA nanoparticle systems to enhance their potential use as chemotherapeutic agents [Citation21]. Since there are limitations in biological use of Hsp, encapsulation of this molecule in an appropriate nanoparticle systems like PLGA could potentially increase its biological activities by increasing water solubility and enhancing bioavailability.
The aim of this study was to encapsulate Hsp into PLGA nanoparticle systems and to evaluate anti-cancer activity of Hsp-loaded PLGA nanoparticles (HspNPs) in C6 glioma cells in comparison with Hsp. For this aim, synthesis optimization of HspNPs and preliminary evaluation of anticancer activity of HspNPs were studied by our group [Citation22]. Hsp was encapsulated into PLGA nanoparticles by single emulsion (o/w) solvent evaporation method to improve solubility and bioavalibility. In vitro anticancer activity evaluation of HspNPs and Hsp was determined by investigating cytotoxicity, anti-proliferative, apoptotic and anti-oxidant activities on C6 glioma cell line.
Materials and methods
Materials
PLGA (lactide:glycolide = 50:50; inherent viscosity 0.45–0.60 dL/g, Mw ∼ 38–54 kDa), polyvinyl alcohol, Hsp, acetone, and ethanol were purchased from Sigma Aldrich (St. Louis, USA); dichloromethane (DCM) was obtained from Ridel de Haen. All the chemicals and solvents used in nanoparticle preparation were of analytical grade and used without further purification. The C6 rat glioma cell line was obtained from the American Type Culture Collection (ATCC, Manassas,VA).
Nanoparticle preparation
HspNPs were prepared by a modified oil in water (o/w) single emulsion solvent evaporation method described as in our previous study [Citation23]. According to this method, the organic phases consisted of PLGA and hesperetin were dissolved into DCM and acetone, respectively. Firstly, Hsp and PLGA soultions were mixed and stirred until the homogeneous solution was obtained. Then, the organic phase was added on to water phase (PVA, 0.5% w/v) and sonicated over an ice bath for 52.5 s at output power 50 W (Bandelin Sonopuls, Germany). Emulsified solution was added into 0.1% PVA solution. After removal of organic solvent by stirring at 700 rpm in room temperature for overnight, nano-suspensions were centrifuged at 14 000 rpm for 40 min at 4 °C (Hettich-Universal 32 R, Germany). Obtained supernatants were collected and precipitated nanoparticles were washed with 35 mL distilled water three times. Produced nanoparticles freeze-dried at 0.01 mbar, −70 °C, 48 h, without the addition of any cryoprotectant. All lyophilized nanoparticles were stored at −80 °C until used.
Characterization of polymeric nanoparticles
Reaction yield (RY), encapsulation efficiency (EE) and drug loading (DL)
Encapsulation efficiency and drug-loading capacity were determined by indirect method via spectrophotometric evalution of supernatant obtained after centrifugation [Citation24]. The amount of unentrapped hesperetin in external solution was determined using UV-Vis spectroscopy at 374 nm and the hesperetin concentration was calculated using previously constructed standard calibration curve. The encapsulation efficiency and drug loading were determined according to the following equation.
(1)
(1)
Mean particle size (Z-Ave), zeta potential (ζ), polydispersity index (PDI) and surface morphology
Z-Ave and ζ values of the HspNPs were measured with photon correlation spectroscopy using a Zetasizer Nano ZS (Malvern Instruments, Worcestershire, UK) [Citation25]. For the purpose of visualizing surface morphology and size distrubition of nanoparticles, field emission scanning electron microscopy was used (JSM-7001FA, JEOL, Tokyo, Japan) with an acceleration voltage of 10 kV [Citation26]. SEM images were taken after Au coating with magnification of 30.000x.
Fourier-transform infrared (FT-IR) spectroscopy
Functional groups present in the surface of nanoparticles were analysed by Fourier-transform infrared spectroscopy in universal attenuation total reflectance (ATR) mode [Citation27]. The FTIR spectra of free hesperetin, free nanoparticle and HspNPs were obtained with 16 scans per sample and 4 cm−1 resolution in the region of 650–4000 cm−1.
Drug release profile
The in vitro release profile of HspNPs was determined according to a modified version of the dissolution method described previously [Citation28]. Firstly, 5 mg of lyophilized HspNPs were suspended in 5 mL of phosphate buffer saline at pH 7.2 by vortexing and ultrasonication until particles were fully dispersed. Then, NPs were incubated at 37 °C and shaking at 240 rpm. To determine the release profile, NP suspension was centrifuged at 14 000 rpm, on certain time points (1, 2, 3, 6, 24, 48, 72, 96, 120, 144 h) then supernatant was collected and fresh buffer was added each time. Hsp amount was quantified with UV-vis spectrometry performed at 374 nm and results were evaluated cumulatively.
Cell culture conditions
C6 glioma cells were cultured in Dulbecco’s modified Eagle’s medium (DMEM)/Ham’s Nutrient Mixture F-12 medium (1:1) (Sigma Aldrich, St. Louis, MO) supplemented with %5 fetal bovine serum (FBS) (Sigma Aldrich), 100 μg/mL streptomycin and 100 units/ml penicillin and 0.2 mM L-Glutamine. Cells were maintained at 37 °C in a humidified incubator containing 5% CO2. The medium was changed in every two days during incubation.
Cell viability assay
Evaluation of cell viability was assessed using MTT (3–(4,5-dimethylthiazol-2-yl)-2,5-diphenyltetrazolium bromide) assay. Briefly, 1 × 104 C6 glioma cells were seeded to 96 well plates. After the incubation the medium was replaced with a fresh medium containing series of dilution (0.1–200 µg/mL) of Hsp and HspNPs. Following 24 and 48 h of incubation, supernatant from each well was replaced with MTT reagent within 50 µg/mL final concentration of MTT in medium then plates were incubated at 37 °C for 3 h. Formazan crystals that formed in the cells were dissolved by adding 0.1 mL of DMSO and absorbance was measured in a microplate reader at 570 nm. Wells containing untreated cells were used as control cells. Values of metabolic activity are displayed as mean ± SD of a minimum of three times. Cell viability (%) was calculated by the following equation:
Cell viability (%)= (OD value of treated cells/OD value of untreated cells (control)) × 100(3)
Measurement of cell proliferation
C6 glioma cells were seeded at a concentration of 1 × 104 cells on coverslips in 24-well tissue culture plates. Then series of dilutions of HspNPs and Hsp (25, 50, 75, 100 µg/mL) were added to culture and incubated at 37 °C for 24 h and 48 h. Following the incubation, cells were washed with phosphate-buffered saline (PBS) and fixed with methanol for 5 min at −20 °C and blocking solution was added. Proliferating cell nuclear antigen (PCNA) primary antibody (Neomarkers, USA) was applied at 1:300 dilution for overnight at 4 °C. Then cells were washed and biotinylated secondary antibodies and streptavidin, biotinylated horseradish peroxidase (Invitrogen, USA) were applied. Immunoreactivity was revealed by using AEC (Invitrogen, USA), hematoxylene-eosin was used to counterstain and samples were mounted in glycerin gelatin. Cells were imaged by using an Olympus BX-50 bright-field microscope. A minimum of 10 fields were randomly selected, and all cells were counted in each field. The percentage of immunoreactive cells ((the number of immunoreactive cells/total cells)×100) were expressed.
Detection of apoptosis by TUNEL assay
Apoptosis was detected by terminal deoxynucleotidyl transferase–mediated dUTP end labeling (TUNEL) assay kit (Millipore, USA) according to manufacturer’s instructions. Briefly, C6 glioma cells were seeded on coverslips and were incubated with four different concentrations (25, 50, 75, 100 µg/mL) of the HspNPs and Hsp for 24 h and 48 h. Methanol was used to fix cells and equilibration buffer was immediately added on the cells. Reaction buffer was mixed with TdT enzyme and cells were incubated with this mixture at 37 °C for 1 h. Anti-digoxigenin conjugate was applied on cells. The reaction was observed by using diaminobenzidine (DAB) system and hematoxylene-eosin staining was used as counterstain. A minimum of 10 fields were randomly selected, and all cells were counted in each field. The percentage of apoptotic cells ((the number of apoptotic cells/total cells) ×100) were expressed.
Oxidative stress and cellular antioxidant activity
Measurement of intracellular antioxidant activity and oxidative stress were assessed by measuring reactive oxygen species (ROS) production, superoxide dismutase enzyme (SOD) activity and reduced glutation (GSH) levels. C6 glioma cells were seeded in 6-well plates at a concentration of 1 × 105 cells per well. After cells reached standard confluency, a fresh medium containing different concentrations (25, 50, 75, 100 µg/mL) of free Hsp and Hsp loaded nanoparticles was added to the culture and incubated for 24 and 48 h. Then, cells were washed and sonicated in PBS to obtain cell lysates for analyses. Total protein concentration in lysates was measured by the bicinchoninic acid method using bovine serum albumin (BSA) as standard [Citation29].
Measurement of reactive oxygen species (ROS)
Reactive oxygen species (ROS) is constantly produced by all biological systems during aerobic metabolism [Citation30]. Thiobarbituric acid reactive substances (TBARS) assay, which detects malondialdehyde (MDA), is one of the most widely used method for intracellular detection of ROS generation. In TBARS assay, MDA-TBA adduct formed by the reaction of MDA and TBA under high temperature (90–100 °C) and acidic condition [Citation31]. In brief, 100 μL cell lysates was subsequently mixed with 0.2 mL of TBA reactive containing 0.375% (w/v) TBA, 0.15% (w/v) trichloracetic acid (TCA) were boiled for 15 min. After centrifugation, reaction mixtures were measured colorimetrically at 535 nm using a microplate reader. Results were expressed as nmol MDA/mg protein in cells.
Determination of superoxide dismutase (SOD) activity
Intracellular SOD enzyme activity was calculated based on a spectrophotometric method described by Mccord JM and Fridovich with minor modifications [Citation32]. In this method, xanthine-xanthine oxidase is used to generate superoxide radicals (O2•−) and O2•− production is measured by reduction of nitroblue tetrazolium (NBT) to a blue formazan which is an indicator for reaction. Xanthine, nitro-blue tetrazolium, sodium carbonate, BSA, EDTA and XO enzyme was used to prepare reaction mixture, then 490 µL of mixture was added to 100 µL of cell lysates then incubated. Reaction was terminated by addition of 0.2 mL of 0.8 mmol cupric chloride to the mixture. Absorbance of produced formazan was measured at 560 nm by using a blank containing all the reagents except the cell lysate. SOD activity was also expressed as units per milligram protein.
Determination of reduced glutathione (GSH) activity
Total glutathione (GSH) and oxidized glutathione (GSSG) activity was measured by a colorimetric method described by Boyne and Ellman [Citation33]. Metaphosphoric acid was mixed with 200 μL of cell lysate and to deproteinize. In order to reduce glutathione (GSH) 1 mmol 5,5′-dithiobis-(2-nitrobenzoicacid) dissolved in 1% sodium citrate solution (Ellman reactive) was added to cell lysate and absorbance was measured at 412 nm immediately. GSH levels were expressed as μg GSH/mg protein in cells.
Statistical analyses
The data were presented as means ± standard deviation (SD) of at least three separate experiments performed in triplicate. Statistical analysis were performed using the GraphPad Prism software version 6 (GraphPad Software, La Jolla, CA). Two-tailed Mann–Whitney nonparametric test was used to calculate statistical differences for cell proliferation and apoptosis analysis. IC50 values were calculated using GraphPad Prism. Statistical differences between control and treated groups for oxidative stress and cellular antioxidant activity measurements were analyzed by two way-Anova test. p values less than .05 was considered as a statistically significant.
Results and discussion
Characterization of Hsp loaded PLGA nanoparticles
In the present study, HspNPs were synthesized using modified single emulsion solvent evaporation method. The reaction yield (RY) was calculated after weighing of lyophilized nanoparticles as 70.7 ± 3.9%. Also, the drug loading (LC) and encapsulation efficiency (EE) were found as 26.4 ± 1.1% and 80.5 ± 2.4%, respectively. Further characterization regarding Z-Ave, PDI, and ζ using Zetasizer were carried out using photon correlation spectroscopy. shows the particle size distribution of nanoparticles measured as intensity versus diameter. The mean particle size and polydispersity index of HspNPs were measured as 260 ± 20 nm and 0.355 ± 0.006, respectively. Obtained polydispersity index of nanoparticles indicate that synthesized nanoparticles has uniform size dispersity. shows the ζ distribution of nanoparticles versus total counts and it was measured as −42.0 ± 1.3 mV. The highly negative zeta potential (ζ > 30 mV), demonstrated that synthesized nanoparticles are s16 in the colloidal form.
Figure 1. Detailed characterization of HspNPs. Particle size distribution (a) and zeta potential (b) analysis of nanoparticles as obtained by Zetasizer. SEM image (c) and particle size distribution (obtained from SEM) with Gaussian fit (d) of HspNPs, FT-IR analysis of PLGA , Hsp and HspNPs (e), Cumulative release of Hsp form HspNPs (f).
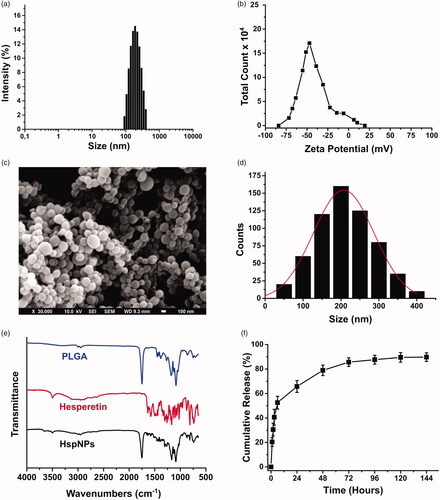
The morphological visualization of HspNPs for providing information about the nanoparticle surface was carried out using FESEM. The nanoparticles were observed after lyophilization without using any cryoprotectant (). Obtained SEM micrograph showed that HspNPs has spherical shape and smooth surface characteristic. The size distrubiton of nanoparticles obtained by SEM was illustrated in . In DLS analysis, a slight increase was found in HspNP particle size compared to SEM analysis. This increase might be a results of PVA hydration on the surface of nanoparticles. The similar results were also previously reported by Sousa et al. [Citation34].
Furthermore, FT-IR analysis was carried out to investigate successful encapsulation of Hsp into PLGA nanoparticles or to identify any chemical interaction between polymer and Hsp during encapsulation process. In , FT-IR spectra were presented for free nanoparticles, Hsp and HspNPs and no change in the position of the bands was observed. Free Hsp showed two bands which are assigned to the functional group of mixed ether of saturated and aromatic C-O at 1040 cm−1 and 1260 cm−1, respectively. Additionally, aromatic multiple stretching of C=C between 1500 cm−1 to 1580 cm−1, carbonyl group (C=O) band at 1635 cm−1, C–H (aromatic) band at 3120 cm−1 and 3039 cm−1, C–H (aliphatic) band at 2890 cm−1, 2957 cm−1, –OH stretching vibration at 3495 cm−1 related to intermolecular hydrogen bonding were identified for Hsp. The similar FT-IR spectra were also previously reported by other researchers [Citation35–37]. It can be seen that, the main characteristic bands of PLGA (2993 cm−1 and 2989 cm−1 peaks corresponds to the aliphatic C–H stretch, 1750 cm−1 peak corresponds to the C=O group and that the peak between 1250 cm−1 and 1100 cm−1 is due to the C=O stretch) were found in HspNPs spectrum. These results indicate that, hesperetin was successfully encapsulated to PLGA nanoparticles without any physical or chemical interaction.
shows the cumulative release pattern of Hsp from HspNPs during 144 h. As detailed in many studies, the release of active agent resulting from degredation of the PLGA nanoparticles is collective process of surface/bulk diffusion and surface/bulk eresion. The results suggest that release of Hsp from nanoparticles is due to the combination of polymer degredation and drug diffusion. Similar to the previous studies [Citation35,Citation38], nanoparticles showed slow hesperetin release profile. Approximately 30.5 ± 5.2% of the burst release occurred in about 2 h due to the short diffusion pathway of Hsp encapsulated in the near-surface of nanoparticles. After 24 h, 65.7 ± 4.7% of Hsp was released from nanoparticles. Furthermore, a constant slow hesperetin release was observed until 89.7 ± 3.5% of within 7 days.
Dose- and time-dependent cytotoxic effect of Hsp and HspNPs on C6 glioma cells
Evaluation of HspNPs induced cell viability was assessed by the decrease in mitochondrial activity by using MTT assay (. C6 glioma cells were cultured with both Hsp and HspNPs within range of 0.1 µg/mL to 200 µg/mL concentrations for 24 h () and 48 h () to evaluate dose- and time-dependent cytotoxic activity. Potent cytotoxicity effect of Hsp have been previously reported in various human cancer lines [Citation12–15] except C6 glioma cells.
Figure 2. Cytotoxic effect of Hsp and HspNPs on C6 glioma cells as determined by MTT assay. Both 24 h (a) and 48 h (b) incubation of C6 glioma cells with Hsp and HspNPs resulted in significant dose and time dependent decrease in cell viability (24h incubation with Hsp and HspNP p = .038, p = .036; 48h incubation with Hsp and HspNP p = .036, p = .028 respectively). Data are shown as mean ± SD of three separate experiments (n = 3).
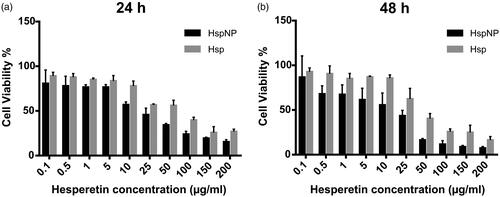
As it is shown in cell viability decreases in relation to increasing concentrations of the HspNPs. In comparison with free Hsp, HspNPs showed higher cytotoxic activity on C6 glioma cells even in the lower concentrations (0.5–5 µg/mL). Moreover, in increasing concentrations starting from 10 µg/mL, HspNPs showed more reduction in mitochondrial function of C6 glioma cells. The IC50 of free Hsp was calculated 72.4 and 52.3 μg/mL for 24 and 48 h, respectively. On the other hand, incubation with HspNPs for 24 and 48 h resulted in dose and time dependent decrease in cell viability, with IC50 of 28 and 21 μg/mL, respectively. In contrast, it has been recently reported that Hsp and HspNPs have showed very low inhibition effect on cell viability of L929 fibroblast cells, which indicates they did not show any significant cytotoxicity to normal cells [Citation23]. At the same concentration, HspNPs drastically decreased cell viability compared to free Hsp. Also, in a time dependent manner (24–48 h) HspNPs has showed increasing cytotoxic effect on C6 glioma cells indicating controlled release of hesperetin from PLGA nanoparticles enhances cytotoxic activity of Hsp (p = .036 for 24 h, p = .025 for 48h).
A previous study used Eudragit® E 100 nanoparticles in the presence of polyvinyl alcohol (PVA) to improve hesperetin’s applicability in cancer therapy against oral carcinoma (KB) cells. Anti-proliferative activity of Hsp loaded Eudragit® nanoparticles were found to be higher than native hesperetin [Citation36]. Another study showed pure Hsp and Hsp loaded gold nanoparticles (AumPEG (5000)-S-HP NPs) were inhibited the growth of Hep3B cells in a dose dependent manner [Citation39]. These results suggests that nanoparticle encapsulated anti-cancer drugs could potentially increase its internalization by cells thus they could have an enhanced cytotoxicity on cancer cells which is parallel with our results.
Effect of Hsp and HspNPs on cell proliferation
Proliferation is an important part of cancer development and progression [Citation40]. Several studies have shown that polyphenols inhibit cell proliferation, which is deregulated in cancer [Citation41,Citation42]. Polyphenols can further act by inhibiting cell proliferation, which is deregulated in cancer. This inhibition has been demonstrated in vitro in many tumor cell lines [Citation43]. PCNA is a well-known marker for detection of cell proliferation and overexpression of PCNA indicates assessment of tumor progression [Citation2].
To establish how Hsp and HspNPs effect cell proliferation on C6 glioma cell line, immunocytochemical PCNA labelling was applied. Immunocytochemical analysis of PCNA has shown a decrease on proliferation rate on C6 glioma cells at all concentrations of Hsp and HspNPs for 24 h treatment (). As its shown in PCNA positive cells decreased in both Hsp (100 µg/mL) and HspNPs (100 µg/mL) group by approximately 40% and 50% respectively when it compared to the untreated cells. 48 h treatment of C6 glioma cells with Hsp and HspNPs has revealed a significant decrease on PCNA-positive cells indicating effect of Hsp on cell proliferation is time dependent (). The percentage of PCNA positive cells for both Hsp and HspNPs group decreased to 20% and 10% (p < .0001) respectively at the concentration of 100 µg/mL. Although, Hsp and HspNPs treated cells showed similar effect on proliferation for 24-h treatment, 48 h treatment with HspNPs (75 and 100 µg/mL) has dramatically decreased cell proliferation rate on C6 glioma cells compared to Hsp.
Figure 3. Dose and time dependent inhibition of cell proliferation on C6 glioma cells by Hsp and HspNPs. (a) 24 h and (b) 48-h treatment with Hsp and HspNPs showed decrease in PCNA positive cell number (p < .05). (c) Brightfield microscope images of C6 glioma cells after 24 and 48 h treatment with Hsp and HspNPs. 48 h incubation with 100 µg/ml HspNPs resulted in significant decrease on proliferation of C6 glioma cells (p < .0001).
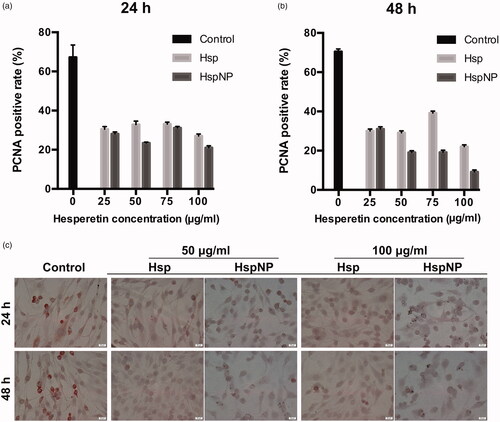
Several studies revealed cell proliferation was inhibited by Hsp in various cancer types including colon cancer, cervival cancer and breast cancer [Citation14,Citation15,Citation39]. Although different studies established anti-proliferative effect of native Hsp and Hsp loaded nanoparticles in different cancer types but no study has been conducted to show anti-proliferative effect of PLGA encapsulated Hsp in C6 glioma cells. Our results revealed HspNPs treatment of C6 glioma cells resulted in a time dependent decrease in proliferating cell numbers depending on controlled release of Hsp from PLGA nanoparticles.
Determination of the apoptotic effect of Hsp and HspNPs on C6 glioma cells
Apoptosis is typically disturbed in human cancers and some phytochemicals might effect cell signaling pathways including activation of apoptosis [Citation44]. TUNEL assay detects apoptotic cells that undergo extensive DNA degradation during the late stages of apoptosis [Citation45]. demonstrates the TUNEL assay generated specific nuclear staining of cells with a morphology consistent with apoptosis. Results showed that Hsp and HspNPs treated C6 glioma cells undergo apoptosis with increasing concentrations of both molecules. 24 h treatment with Hsp (25, 50, 75 and 100 µg/mL) showed 4.2-, 3.1-, 6.3- and 9.9-fold increase in number of apoptotic cells compared to untreated control. On the other hand, treatment with increasing concentrations of HspNPs (25, 50, 75 and 100 µg/mL) resulted in 9.1-, 7-, 12.5- and 12.7-fold in increase in apoptotic cell number. As seen in and , 48-h treatment with Hsp and HspNPs showed similar trend with 24 h treatment (). Apoptotic cell numbers has found to be maximum at 24 h 100 µg/mL HspNPs treatment, which suggests nanoparticle systems has stronger effect to induce apoptosis due to their controlled release nature (p < .0001). A similar study by Yallapu et. al. showed that curcumin loaded PLGA nanoparticle systems increases cellular uptake thus enhance apoptosis in ovarian and metastatic breast cancer cell lines compared to free curcumin [Citation46]. Another study reported that 5-fluorouracil (5-FU)-entrapped PLGA nanoparticles exhibited better apoptosis induction than free drug in a dose-and time-dependent manner [Citation47]. Overall, these studies highlight the importance of nanoparticle systems in order to effectively induce apoptosis in cancer cells. Additionally, proliferating cell numbers were found to be minimum at the same concentration which is parallel with TUNEL assay results.
Figure 4. Apoptotic activitiy results of Hsp and HspNPs by TUNEL assay on C6 glioma cells. (a) 24 h and (b) 48 h treatment with Hsp and HspNPs showed increase in TUNEL positive cell number. (c) The highly condensed and fragmented nuclei that are the index of apoptosis were observed at increasing concentrations of both molecules. *p < .05, **p < .001, ***p < .0001 compared to control.
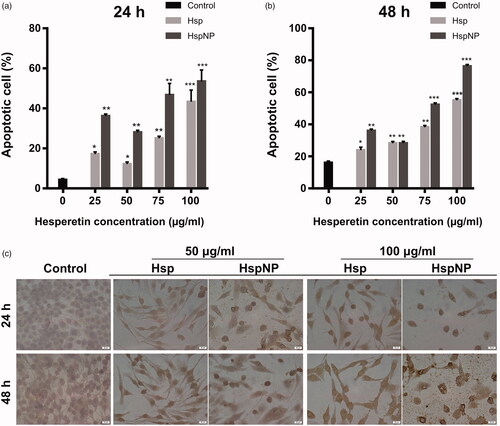
Determination of oxidative stress and cellular antioxidant activity of Hsp and HspNPs
MDA
Oxidative stress is considered to be an important mechanism in cancer and deregulation of cellular redox status plays a vital role in the pro-apoptotic activity of various anti-cancer agents [Citation48]. In order to evaluate the association of ROS accumulation with Hsp and HspNPs induced apoptosis of C6 glioma cells, MDA levels were determined in a dose and time dependent manner (. MDA levels, an indicator of lipid peroxidation, were found to be significantly elevated at 75 and 100 µg/mL exposure concentration of HspNPs with (p = .002) and (p = .018), respectively for 48-h treatment ().
Figure 5. MDA levels were measured to determine intracellular reactive oxygen species (ROS) generation in C6 glioma cells after (a) 24 h and (b) 48-h treatment with Hsp and HspNPs. *indicate statistically significant differences compared to control (p < .05).
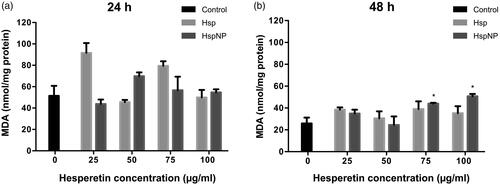
This indicates controlled release of Hsp by PLGA nanoparticles increases the rate of intracellular ROS production. No statistically significant change was observed despite the increase of MDA level at 25 µg/mL of hesperetin incubation for 24 h (). Several mechanishms has been proposed to explain flavonoid mediated apoptosis by ROS generation such as superoxides, hydrogen peroxide, hydroxyl and other oxygen radicals which are capable of directly oxidizing the DNA, proteins and lipids [Citation49]. Additionally, it has been reported that chemotherapeutic agents can trigger apoptosis in cancer cells by increase in ROS generation or decrease in ROS scavenging capacity [Citation50]. There are many evidences showing that oxidative stress is a common mechanism for nanoparticle induced cell damage in different types of cells [Citation49,Citation51,Citation52]. In consistent with literature, our results suggests that HspNPs induced apoptosis could be carried out through ROS generation. However, further studies needed to elucidate the mechanishm of ROS induced apoptosis by HspNPs.
SOD
Total SOD level was increased after treatment of C6 glioma cells within range of 25–100 µg/mL. shows the effect of HspNPs and free Hsp treatment for 24 h and 48 h in C6 glioma cells on SOD activity. It has been shown that SOD activity increased in both Hsp and HspNPs treatment when it compared to control (. Highest SOD activity with 1322,2 U/mg protein has been detected at treatment with 25 µg/mL free Hsp (p = .022). Decreasing activity of SOD at increasing concentrations could be possibly explained by the lower solubility of Hsp in water [Citation16]. Despite, HspNPs has shown lower SOD activity compared to free Hsp, both HspNPs and free Hsp has increased SOD levels comparison to control group in a dose dependent manner. SOD, CAT, GPx, GST and GR are critical intracellular oxidants which combats the reactive oxygen species-induced cell death and tissue damage [Citation53]. Our findings revealed that untreated C6 glioma cells has shown decreased activity of SOD enzyme. Hovewer, both Hsp and HspNPs treatment significantly increased SOD levels. This might be a result of superoxide radical scavenging activity of Hsp.
Figure 6. The effect of Hsp and HspNPs treatment in C6 glioma cells on SOD activity. (a) 24 h (b) 48 h incubation of cells with increasing concentrations Hsp and HspNPs. *indicate statistically significant differences between Hsp and HspNPs (p < .05).
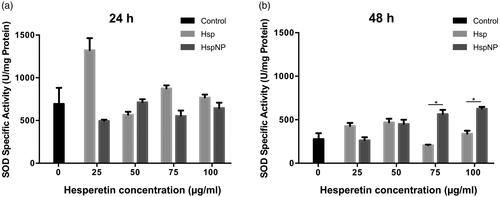
PLGA nanoparticles containing Hsp were found to have lower SOD specific activity than free Hsp on C6 glioma cells in the first 24 h since they released time-dependent. In contrast, 48 h incubation of cells with HspNPs and free Hsp, SOD activity was found higher in HspNPs treated group in all concentrations (). This indicates controlled release of Hsp from nanoparticles has stronger effect on antioxidant activity of glioma cells.
GSH
Evaluation of adaptive response against oxidative stress is generally detected by changes in total GSH level. The role of reduced GSH in tissues is to maintain the cellular levels of Vit C and Vit E in an active form [Citation54]. One of the most important biological activity of these vitamins is to scavenge free radicals in oxygen metabolism, thereby altering the metabolic activation of carcinogenesis [Citation55]. In our study, no statistically significant change was observed in reduced GSH level after 24 h and 48 h treatment with both molecules (). However, 48 h exposure of nanoparticle containing 100 µg/mL Hsp resulted in a significant increase (p = .00023) in total GSH level compared to untreated cells (). This indicate that radical scavenging activity of Hsp might effectively restored the depleted GSH.
Conclusion
Hesperetin has received considerable attention in cancer prevention due to its antiproliferative, antioxidant and apoptosis inducing activities. One of the most common types of brain tumours are gliomas and high permeability of Hsp for blood–brain barrier makes it an attractive molecule for treatment of this disease. However, poor water solubility limits the potential of Hsp to be used as an active cancer agent. On the other hand, nanoparticles could be used to enhance the efficacy and solubility of low soluble therapeutic agents.
In this study, encapsulation of hesperetin into PLGA nanoparticles and detailed characterization of HspNPs was successfully performed. Further, in vitro anticancer activity of HspNPs were carried out in comparison with hesperetin. This is the first report to identify anti-proliferative, apoptotic and antioxidant properties of hesperetin and hesperetin loaded PLGA nanoparticles on C6 glioma cells. Our results showed that HspNPs treatment of C6 glioma cells significantly decreases cell viability, indicating controlled release of hesperetin from PLGA nanoparticles enhances cytotoxic activity of the molecule. Similarly, PCNA labelling was identified decreasing number of proliferating cells after HspNP treatment compared to Hsp treatment and control group. Evaluation of apoptosis by TUNEL assay also revealed increasing number of apoptotic C6 glioma cells after HspNP treatment. Additionally, our results suggest that HspNPs treatment might decrease antioxidant defence mechanism thereby subsequently increases apoptosis by activating lipid peroxidation. This report highlights the importance of encapsulation of hesperetin into biocompatible nanoparticle systems in order to increase molecule’s low solubility, enhance availability in biological systems and disseminate potential applications as chemotherapeutic agent.
Disclosure statement
No potential conflict of interest was reported by the authors.
References
- Hanif F, Muzaffar K, Perveen K. Glioblastoma multiforme: A review of its epidemiology and pathogenesis through clinical presentation and treatment. Asian Pac J Cancer Prev. 2017;18:3.
- Zhang XH, Zhang NN, Meng XB, et al. Hesperetin inhibits the proliferation of cerebrally implanted C6 glioma and involves suppression of HIF-1α/VEGF pathway in rats. Biomed Res. 2017;28:1205-1211.
- Bi WL, Beroukhim R. Beating the odds: extreme long-term survival with glioblastoma. Soc Neuro-Oncol. 2014;16(9):1159-1160.
- Haar CP, Hebbar P, Wallace GC, et al. Drug resistance in glioblastoma: a mini review. Neurochem Res. 2012;37:1192–1200.
- Murphy J, Spencer D, Cheng Y, et al. Gold Nanoparticles for the Treatment of Malignant Gliomas. THE WORLD SCIENTIFIC ENCYCLOPEDIA OF NANOMEDICINE AND BIOENGINEERING I: Volume 1: Noble Metal Nanoparticles for Biomedical Applications: World Scientific; 2017;151–176.
- Prakash O, Kumar A, Kumar P, et al. Anticancer potential of plants and natural products: A. Ajps. 2013;1:104–115.
- Galati G, O'brien PJ. Potential toxicity of flavonoids and other dietary phenolics: significance for their chemopreventive and anticancer properties. Free Radic Biol Med. 2004;37:287–303.
- Ravishankar D, Rajora AK, Greco F, et al. Flavonoids as prospective compounds for anti-cancer therapy. Int J Biochem Cell Biol. 2013;45:2821–2831.
- Galati E, Monforte M, Kirjavainen S, et al. Biological effects of hesperidin, a citrus flavonoid.(Note I): antiinflammatory and analgesic activity. Farmaco. 1994;40:709–712.
- Galati E, Trovato A, Kirjavainen S, et al. Biological effects of hesperidin, a Citrus flavonoid.(Note III): antihypertensive and diuretic activity in rat. Farmaco. 1996;51:219–221.
- Parhiz H, Roohbakhsh A, Soltani F, et al. Antioxidant and anti‐inflammatory properties of the citrus flavonoids hesperidin and hesperetin: an updated review of their molecular mechanisms and experimental models. Phytother Res. 2015;29:323–331.
- Sambantham S, Radha M, Paramasivam A, et al. Molecular mechanism underlying hesperetin-induced apoptosis by in silico analysis and in prostate cancer PC-3 cells. Asian Pac J Cancer Prev. 2013;14:4347–4352.
- Ye L, Chan FL, Chen S, et al. The citrus flavonone hesperetin inhibits growth of aromatase-expressing MCF-7 tumor in ovariectomized athymic mice. J Nutr Biochem. 2012;23:1230–1237.
- Alshatwi AA, Ramesh E, Periasamy V, et al. The apoptotic effect of hesperetin on human cervical cancer cells is mediated through cell cycle arrest, death receptor, and mitochondrial pathways. Fundam Clin Pharmacol. 2013;27:581–592.
- Aranganathan S, Nalini N. Antiproliferative efficacy of hesperetin (citrus flavanoid) in 1, 2‐dimethylhydrazine‐induced colon cancer. Phytother Res. 2013;27:999–1005.
- Liu L, Chen J. Solubility of hesperetin in various solvents from (288.2 to 323.2) K. J Chem Eng Data. 2008;53:1649–1650.
- Erlund I, Meririnne E, Alfthan G, et al. Plasma kinetics and urinary excretion of the flavanones naringenin and hesperetin in humans after ingestion of orange juice and grapefruit juice. J Nutr. 2001;131:235–241.
- Singh R, Lillard JW. Nanoparticle-based targeted drug delivery. Exp Molecul Pathol. 2009;86:215–223.
- Zhou Y, Peng Z, Seven ES. Crossing the blood-brain barrier with nanoparticles. J Control Release. 2017;270:290-303.
- Sadat Tabatabaei Mirakabad F, Nejati-Koshki K, Akbarzadeh A, et al. PLGA-based nanoparticles as cancer drug delivery systems. Asian Pac J Cancer Prev. 2014;15:517–535.
- Hafezi Ghahestani Z, Alebooye Langroodi F, Mokhtarzadeh A, et al. Evaluation of anti-cancer activity of PLGA nanoparticles containing crocetin. Artif Cells Nanomed Biotechnol. 2017;45:955–960.
- Ersoz M, Erdemir A, Uzunoglu D, et al., editors. Investigation of anti-cancer activity of hesperetin and hesperetin loaded polymeric nanoparticles on C6 glioma cells. WILEY 111 RIVER ST, HOBOKEN 07030-5774, NJ USA: FEBS OPEN BIO; 2018.
- Duranoğlu D, Uzunoglu D, Mansuroglu B, et al. Synthesis of hesperetin loaded PLGA nanoparticles by two different experimental design methods and biological evaluation of optimized nanoparticles. Nanotechnol. 2018;29(39).
- Arasoglu T, Derman S. Assessment of the antigenotoxic activity of poly (d, l-lactic-co-glycolic acid) nanoparticles loaded with caffeic acid phenethyl ester using the AMES/Salmonella microsome assay. J Agri Food Chem. 2018;66(24):6196-6204.
- Derman S, Akdeste ZM. Particle size and zeta potential investigation of synthetic peptide-protein conjugates/Sentetik peptid-protein konjugatlarının parçacık boyutu ve zeta potensiyel analizi. Turkish J Biochem. 2015;40:282–289.
- Arasoglu T, Derman S, Mansuroglu B. Comparative evaluation of antibacterial activity of caffeic acid phenethyl ester and PLGA nanoparticle formulation by different methods. Nanotechnol. 2016;27:025103.
- Derman S. Caffeic acid phenethyl ester loaded PLGA nanoparticles: effect of various process parameters on reaction yield, encapsulation efficiency, and particle size. J Nanomater. 2015;16:318.
- Arasoglu T, Derman S, Mansuroglu B, et al. Synthesis, characterization and antibacterial activity of juglone encapsulated PLGA nanoparticles. J Appl Microbiol. 2017;123:1407–1419.
- Walker JM. The Bicinchoninic Acid (BCA) assay for Protein Quantitation. The Protein Protocols Handbook. Totowa, NJ: Springer; 2002. p.11–14.
- Apel K, Hirt H. Reactive oxygen species: metabolism, oxidative stress, and signal transduction. Annu Rev Plant Biol. 2004;55:373–399.
- Esterbauer H, Cheeseman KH. [42] determination of aldehydic lipid peroxidation products: malonaldehyde and 4-hydroxynonenal. Methods in enzymology. Vol.186. New York (NY): Elsevier; 1990. p.407–421.
- McCord JM, Fridovich I. Superoxide dismutase an enzymic function for erythrocuprein (hemocuprein). J Biol Chem. 1969;244:6049–6055.
- Boyne AF, Ellman GL. A methodology for analysis of tissue sulfhydryl components. Anal Biochem. 1972;46:639–653.
- Sousa F, Cruz A, Fonte P, et al. A new paradigm for antiangiogenic therapy through controlled release of bevacizumab from PLGA nanoparticles. Scientific Rep. 2017;7:3736.
- Fathi M, Varshosaz J, Mohebbi M, et al. Hesperetin-loaded solid lipid nanoparticles and nanostructure lipid carriers for food fortification: preparation, characterization, and modeling. Food Bioprocess Technol. 2013;6:1464–1475.
- Gurushankar K, Gohulkumar M, Prasad NR, et al. Synthesis, characterization and in vitro anti-cancer evaluation of hesperetin-loaded nanoparticles in human oral carcinoma (KB) cells. Adv Nat Sci: Nanosci Nanotechnol. 2013;5:015006.
- Ferreira O, Schro¨der B, Pinho SP. Solubility of hesperetin in mixed solvents. J Chem Eng Data. 2013;58:2616–2621.
- Fathi M, Varshosaz J. Novel hesperetin loaded nanocarriers for food fortification: Production and characterization. J Funct Foods. 2013;5:1382–1391.
- Krishnan G, Subramaniyan J, Subramani PC, et al. Nanochemopreventive effect of polymer functionalized gold nanoparticles containing hesperetin drug inhibited proliferation and induced apoptosis in Hep3B cells. J App Pharm Sci. 2016;6:114–123. Vol.
- Evan GI, Vousden KH. Proliferation, cell cycle and apoptosis in cancer. Nature. 2001;411:342.
- Leifert WR, Abeywardena MY. Grape seed and red wine polyphenol extracts inhibit cellular cholesterol uptake, cell proliferation, and 5-lipoxygenase activity. Nutr Res. 2008;28:842–850.
- Ramanathan R, Das N, Tan C. Inhibitory effects of 2-hydroxy chalcone and other flavonoids on human cancer cell-proliferation. Int J Oncol. 1993;3:115–119.
- Yordi EG, Pérez EM, Matos MJ, et al. Antioxidant and pro-oxidant effects of polyphenolic compounds and structure-activity relationship evidence. Nutrition, Well-Being and Health: InTech. 2012.DOI:10.5772/29471
- Srivastava S, Somasagara RR, Hegde M, et al. Quercetin, a natural flavonoid interacts with DNA, arrests cell cycle and causes tumor regression by activating mitochondrial pathway of apoptosis. Scientific Rep. 2016;6:24049.
- Kyrylkova K, Kyryachenko S, Leid M, et al. Detection of Apoptosis by TUNEL Assay. Odontogenesis: Springer; 2012. p. 41–47.
- Yallapu MM, Gupta BK, Jaggi M, et al. Fabrication of curcumin encapsulated PLGA nanoparticles for improved therapeutic effects in metastatic cancer cells. J Colloid Interface Sci. 2010;351:19–29.
- Nair L, Jagadeeshan S, Nair SA, et al. Biological evaluation of 5-fluorouracil nanoparticles for cancer chemotherapy and its dependence on the carrier, PLGA. Int J Nanomed. 2011;6:1685.
- Acharya A, Das I, Chandhok D, et al. Redox regulation in cancer: a double-edged sword with therapeutic potential. Oxidative Med Cell Long. 2010;3:23–34.
- Wang F, Gao F, Lan M, et al. Oxidative stress contributes to silica nanoparticle-induced cytotoxicity in human embryonic kidney cells. Toxicol in Vitro. 2009;23:808–815.
- Khoshtabiat L, Mahdavi M, Dehghan G, et al. Oxidative stress-induced apoptosis in chronic myelogenous leukemia k562 cells by an active compound from the dithio-carbamate family. Asian Pac J Cancer Prev. 2016;17:4267–4273.
- Pulskamp K, Diabaté S, Krug HF. Carbon nanotubes show no sign of acute toxicity but induce intracellular reactive oxygen species in dependence on contaminants. Toxicol Lett. 2007;168:58–74.
- Park E-J, Park K. Oxidative stress and pro-inflammatory responses induced by silica nanoparticles in vivo and in vitro. Toxicol Lett. 2009;184:18–25.
- Bodduluru LN, Kasala ER, Barua CC, et al. Antiproliferative and antioxidant potential of hesperetin against benzo(a)pyrene-induced lung carcinogenesis in Swiss albino mice. Chem Biol Interact. 2015;242:345–352.
- Malhotra A, Nair P, Dhawan D. Modulatory effects of curcumin and resveratrol on lung carcinogenesis in mice. Phytother Res. 2010;24:1271–1277.
- Rahman K. Studies on free radicals, antioxidants, and co-factors. Clin Interven Aging. 2007;2:219.