Abstract
Previous studies have focused on the changes of tumour cells in immune escape, and less is known about the effect of tumour microenvironment (TME) on immune escape. Tumour-associated fibroblasts (TAF) is an important part of the TME and has special physiological and biochemical characteristics, but the specific mechanism has not been clarified. In order to investigate the effect of TAF on the expression of PD-L1 in gastric cancer cells, gastric cancer cell lines MNK45, SGC7901 were non-contact co-culturing with TAF 1, 3 and 7 d via transwell. PD-L1 mRNA and protein expression were detected using qRT-PCR and FCM. Then, 95 cases of gastric cancer tissues were selected and evaluated PD-L1 and TAF expressions by immunohistochemical examination. The results showed that the mRNA and protein expression of PD-L1 in the experiment group were significantly higher than that in the control group. PD-L1 expression was associated with massive lymphocyte infiltration, diffuse/mixed histology and intratumoral TAFs in gastric cancers. In conclusion, TAFs promoted the growth in gastric cancer cell lines by increased the PD-L1 expression.
Keywords:
Introduction
Gastric cancer (GC) is one of the world’s most common digestive system malignant tumour, especially in East Asian countries such as South Korea, Japan and China [Citation1–3]. The prognosis for advanced-stage GC is grim, even with the use of chemotherapy or other adjuvant treatments [Citation4–6]. Tumour cells can escape from the immune surveillance. Previous studies have focused on the changes of tumour cells in immune escape, however, little is known about the effect of tumour microenvironment (TME) on immune escape [Citation7–9].
Tumour-associated fibroblasts (TAFs) are the most important host cells in the microenvironment stroma, which take part in extracellular matrix construction and cancer colony development [Citation10,Citation11]. Compared with normal tissue fibroblasts, TAFs have significantly different morphology and protein expression patterns [Citation12]. For instance, TAFs express α-SMA and vimentin and secrete TGF-β, PDGF, FGF, VEGF, IL-11, IL-6 and extracellular matrix components, which can promote cancer cell growth, induce tumour angiogenesis and enhance metastasis and invasion [Citation13–15]. It is now clear that there is continual crosstalk not only between tumour cells and immune cells but also between other stroma cells such as TAFs and immune cells. Because of their preponderance in the tumour microenvironment, TAFs have recently been investigated for their function as a regulator of immune cell recruitment and function.
Programmed death factor 1 (PD-1) and its ligands PD-L1/PD-L2 are negative regular factor, which play a very pivotal role in tumour escape host immune response [Citation16–20]. Expressions of PD-L1 in cancer patients are associated with depth of invasion, lymphatic metastasis, pathological pattern and overall survival. PD-L1 monoclonal antibody in melanoma and non-small cell lung cancer and other tumours have a good curative effect but poor curative effect on the digestive system tumours [Citation21–23]. Recently, PD-L1 has been reported to be expressed in TAFs [Citation24]. TAFs can influence tumour growth by producing growth factors and chemokines [Citation25,Citation26].
To elucidate the effect of tumour-associated fibroblasts in tumour microenvironment on the immunological properties of tumour cells, we observed the expression of the immunosuppressive molecule PD-L1 by constructing the co-culture system of gastric cancer cell lines and gastric cancer-related fibre cells and then relationships between clinicopathological parameters of GC and PD-L1 expression associated with TAFs.
Materials and methods
Tissue samples and cell lines
Ninety-five gastric cancer tissues and adjacent normal tissues which had not treated with radiation therapy or chemotherapy before surgery were obtained from Department of General Surgery, Qingdao University. All these tissues were immediately snap-frozen in liquid nitrogen after surgery. The study was approved by the Ethics Committee of Yuhuangding Hospital (2016081206).
Cell culture
Human gastric cancer cell lines (SGC7901 and MNK45) were purchased from the Type Culture Collection of the Chinese Academy of Sciences (Shanghai, China) and maintained in Dulbecco modified Eagle medium (DMEM, Hyclone, USA) supplemented with 10% fetal bovine serum (FBS; Hyclone, USA), 100 U/mL penicillin and 100 ng/mL streptomycin in condition of at 37 °C temperature with 5% CO2 and 95% air.
Human gastric TAFs were derived from surgical tissue of 95 patients with GC. TAFs were established from the tumour gastric wall, and normal fibroblasts (NFs) were from non-tumoral gastric wall. The fresh specimens were washed three times with PBS containing 100 U/mL penicillin and 100 μg/mL streptomycin and cut into small pieces and digested with collagenase I. Then the mixture was centrifuged and rinsed the cells with PBS, the pellets were re-suspended in DMEM supplemented with 10% FBS and transferred to 100 mm dishes. These cell lines cultured in RPMI 1640 or DMEM containing 10% fetal bovine serum (FBS). For the co-culture assay, the cell lines were cultured in RPMI 1640.
Cell co-culture model
Human gastric cancer cells (SGC7901 and MNK45) and TAFs were non-contact co-cultured in vitro via transwell (Corning Incorporated Life Science, Acton, MA, USA). Two chambers were separated by a semi-permeable membrane with a pore size of 0.4 μm and 4.5 cm2 area (BD Biosciences). Human gastric cancer cells (SGC7901 and MNK45) were cultured at the density of 3 × 105 cells/mL in the upper chambers of the transwell inserts. The lower chambers were cultured in TAFs cells at the density of 5 × 105 cells/mL or contained normal fibroblasts at the density of 5 × 105 cells/mL (for controls).
Cell proliferation assays
Cell proliferation was evaluated by the alamarBlue assay. After culture for 1, 3 and 7 d, the culture medium was replaced by Medium 1640 (GIBCO, Carlsbad, CA, USA) supplemented with 10% FBS and 10% alamarBlue. The cells were incubated at 37 °C in a humidified atmosphere of 5% CO2 for 4 h. The optical density (OD) of the medium was read at 570 nm in a microplate reader (MQX200).
Reverse transcription and quantitative real-time PCR
Total RNA were extracted from cells using TRIzol® reagent (Invitrogen, CA, USA) according to the manufacturer’s instructions. Quantitative reverse transcription-polymerase chain reaction (qRT-PCR) analysis was carried out with Prime Script RT Reagent Kit (TaKaRa, Dalian, China). The PD-L1 primers are: forward, 5′-AAATGGAACCTGGCGAAAGC-3′; reverse, 5′-GATGAGCCCCTCAGGCATTT-3′; GAPDH mRNA primers are: forward, 5′-ACGGATTTGGTCGTATTGGG-3′; reverse, 5′-CGCTCCTGGAAGATGGTGAT-3′.
PD-L1 protein expression
The expressions of PD-L1 were analyzed with FCM in experimental and control groups. The co-cultured or control cultured cells were harvested and washed with FCM buffer (PBS with 5% FBS and 0.1% NaN3) twice. After incubating with fluorescein labelled or isotype control antibodies for 30 min at 4 °C, cells were washed with FCM buffer and analyzed on a Beckman coulter FC500 with submit5.2 software (Beckman Coulter, USA).
Immunohistochemical staining
Immunohistochemistry was performed according to a conventional protocol [Citation27]. The paraffin-embedded specimens were sectioned at a thickness of 4 μm and incubated in a dry oven at 63 °C for 1 h. De-paraffinization and rehydration were then performed. The sections were incubated with the following primary antibodies: polyclonal rabbit anti-human PD-L1 (clone ab82059, abcam, UK; 1:100), monoclonal mouse anti-human α-SMA (clone 4B12, Dako, Denmark; 1:80) were treated for 1 h at room temperature. Negative controls were performed by replacing the specific antibody with mouse IgG isotype (Sigma, St. Louis, MO, USA).
Statistical analysis
All statistical analysis was carried out using the SPSS 18.0 software (SPSS, Inc., Chicago, IL, USA). All values are expressed as the mean ± standard deviation from at least three repeated individual experiments for each group. The significant differences were analyzed using Student’s t-test analysis for difference between two groups, and person’s chi-square test and Fisher’s exact test were used to detect the correlation between PD-L1 expression and other clinicopathological characteristics. Differences were considered significant when p < .05.
Results
Primary culture and characteristic of TAFs and NFs
The fibroblast markers, fibronectin (FN) and α-smooth muscle actin (SMA) were determined by immunofluorescence staining. The results showed that FN positive expression in TAFs and NFs cells. Moreover, α-SMA was high expression in TAFs and a biomarker for myofibroblasts (). Further analyze showed that gastric cancer cells co-cultured with TAFs grew faster compared with the control groups ().
Figure 1. Characterization of tumour-associated fibroblasts (TAFs) and normal fibroblasts (NFs). The primary cultures of TAFs and NFs were isolated from the tumour tissue and the adjacent normal gastric mucosa. Fibronectin (FN), the fibroblast biomarker, is universally expression in TAFs and NFs. α-smooth muscle actin (α-SMA), the biomarker of the activate fibroblast is a high level expression in TAFs and weak expression in NF.
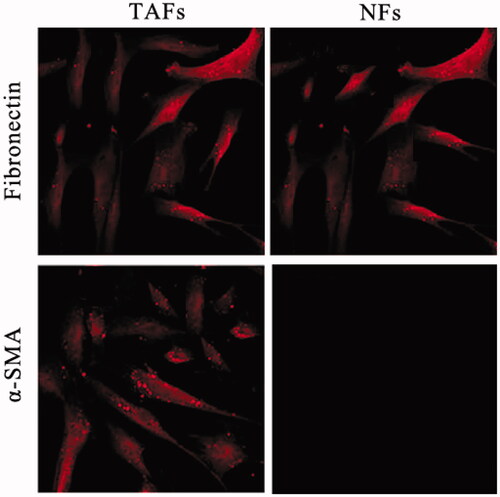
Figure 2. The gastric cancer cells grew faster when co-cultured with TAFs. The proliferation of SGC7901 (A) and MNK45 (B) cells were tested by alamarBlue assay at 1, 3 and 7 d of culture. There was a significant increase in the viability of gastric cancer cells at 3 and 7 d of co-cultured with TAFs.
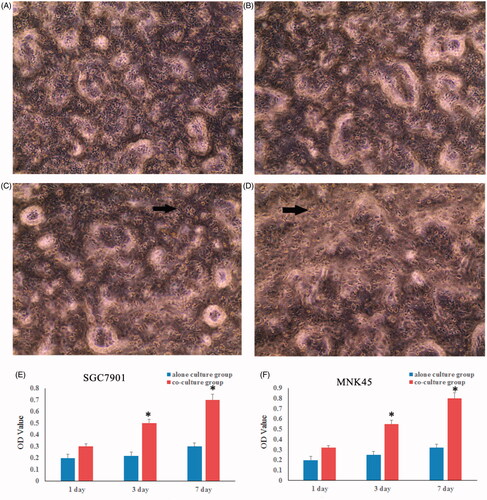
The PD-L1 expression in gastric cancer cells
We further assessed the effects of PD-L1 expression with qRT-PCR and FCM in the gastric cancer cell lines co-cultured with TAFs and the control groups. The results revealed that the mRNA expression of PD-L1 was markedly increased in gastric cancer cell lines co-cultured with TAFs in a time-dependent manner. Similarly, the protein expression of PD-L1 exhibited similar results ().
The expression of PD-L1 in gastric cancer tissues
The expression levels of PD-L1 in gastric cancer tissues were evaluated and its relationship with clinical and pathological of GC patients was analyzed. There was no significant difference between PD-L1 levels and gender (p = .845, ), tumor site (p = .123, ), tumor invasion (p = .634, ) and metastasis (p = .476, ) and tumor-node-metastasis (TNM) staging (p = .675, ). There was a positive correlation between PD-L1 expression and younger age (p = .023, ) and inverse correlation between PD-L1 expression and tumour nodal status (p = .035, ).
Table 1. Correlation between PD-L1 expression and clinicopathological factors.
Relationships between PD-L1 expression and densities of TAFs
TAFs were positive for PD-L1 in 53 cases (82%) and stained in a scattered-dot pattern within the cytoplasm. The density of intratumoral TAFs showed a significant positive correlation with the PD-L1 expression of tumour cells (p = .027, ). TAFs were also positively correlated with age (p = .013, ) and TNM staging (p = .025, ).
Table 2. Correlation between PD-L1 expression and density of ATFs.
Discussion
Gastric cancer is one of the world’s most common digestive system malignant tumour, especially in East Asian countries such as South Korea, Japan and China. Tumour cells can escape from the immune surveillance. Previous studies have focused on the changes of tumour cells in immune escape, and less is known about the effect of tumour microenvironment (TME) on immune escape [Citation28–30].
In early stages of cancer development, various types of cells in tumour stroma prevent tumour growth. In contrast, most of these stromal cells promote tumour progression in advanced stages [Citation31]. TAFs have played a critical role by secreting various soluble factors [Citation32–34]. It has previously revealed that the prevalence of TAFs was closely correlated with the potential of gastric cancer metastasis [Citation35]. Moreover, TGF-β and FGF-7 produced from fibroblasts could increase gastric cancer cell proliferation and invasiveness [Citation36,Citation37]. Several reports have also demonstrated the TAFs express MCP-1/CCL2 when co-cultured with cancer cells and induce cell survival and immunosuppression [Citation26,Citation38]. Together relevant results suggested that TAFs were first educated by immune cells during the initial stages of tumorigenesis but, as tumour progression occurs, they acquire the ability to recruit and regulate immune cells to an eventually immune-suppressed which is compatible with disease progression. However, factors important for cancer immune escape in direct interactions between TAFs and cancer cells remain unknown.
Immunological resistance represents one of the major obstacles for effective tumour biotherapeutic options for a wide variety of human cancers. PD-L1, a key protein located in the tumour microenvironment, may promote the immune escape of tumour cells [Citation39]. It is now well known that PD-L1 over expression in cancer cells could induce tumour evasion from the host T cells [Citation40,Citation41]. Blockade of PD-1/PD-L1 interaction or down-regulation of PD-L1 expression in cancer cells has been reported to promote anti-tumour immunity and inhibit tumour growth [Citation41]. The regulation of PD-L1 expression remains largely unknown. In the present study, we report that TAFs co-cultured could able to up-regulate PD-L1 expression in human gastric cancer cell lines in a time-dependent manner and induced the cancer cells proliferation. The density of intratumoral TAFs showed a significant positive correlation with the PD-L1 expression of tumour cells (p = .027). Combined with previous results, this suggests that the induction of PD-L1 expression by TAFs in cancer cells is a common effect.
Previous studies showed that immunohistochemical PD-L1 expression was strongly positive in 42.2% of 102 human gastric carcinomas, weakly positive in adenoma samples and totally negative in normal gastric tissue [Citation42]. Moreover, PD-L1 expression was significantly correlated to a higher number of lymph node metastasis, larger tumour size, increased depth of invasion and poorer overall survival. Same results were presented that one of the anti-PD-L1 mAbs developed during the investigation significantly reduced the T-cells apoptosis induced by PD-L1 overexpression [Citation43].
Several clinical trials have demonstrated that blocking the PD-1/PD-L1 pathway with a PD-L1 antibody results in antitumor effects [Citation44,Citation45]. With higher grade, tumour cells overexpress PD-L1 in infiltrating ductal carcinoma (p = .012) [Citation46], urothelial carcinoma (p < .001) [Citation29], and intrahepatic cholangiocarcinoma (p < .001) [Citation29]. In our study, the expression of PD-L1 is significantly associated with younger age (p = .023) and diffuse/mixed type (p = .032). There was an inverse correlation between PD-L1 expression and tumour nodal status (p = .035). However, no statistical relationships were found between the expression of PD-L1 and gender, tumour site, tumour invasion and metastasis and TNM staging. The probable reason is the relatively small number of PD-L1 positive cases. Similarity results showed that no statistical relationships were found between the expression of PD-L1 and clinical stage/survival rate in non-small cell lung carcinomas [Citation47,Citation48].
In normal human body, PD-1 and CD28 are in dynamic balance, but PD-1 and its ligand are high expressed in tumour patients [Citation46]. Tumour cells with high expression of PD-L1 inhibited the proliferation and function of CD8+ T cells. Meanwhile, high expression of PD-L1 also inhibited the proliferation and function of NK cells and B cells [Citation49]. When stimulated by PD-L1, Treg cells can enhance their ability to proliferation and up-regulate their functions [Citation50]. All of the above-mentioned findings lead to tumour escape from immune surveillance and promote tumour growth. Therefore, the underlying mechanisms should be explored in the future to fully elucidate the association of PD-L1 with the prognosis of GC patients.
In conclusion, we have demonstrated that the expression of PD-L1 was markedly increased in gastric cancer cell lines co-cultured with TAFs in a time-dependent manner. PD-L1-positive tumour cells were found in most GC patients (68%), and which were associated with the densities of TAFs (82%). Furthermore, our study found that PD-L1 expression was associated with massive lymphocyte infiltration, diffuse/mixed histology and intratumoral TAFs in GC. Taken together, blockade of tumour PD-L1 is expected to enhance the effects of immunotherapy.
Ethics approval and consent to participate
The present study was approved by the Institutional Animal Care and Use Committee of Shandong University (Jinan, China).
Consent for publication
Not applicable.
Acknowledgements
This study was referred in part by Zhou et al (SMC1A recruits tumor-associated-fibroblasts (TAFs) and promotes colorectal cancer metastasis, Cancer letters 2017, 385, 39–45), Harper et al (Regulation of the anti-tumor immune response by cancer-associated fibroblasts, Seminars in Cancer Biology 2014, 25, 67–77), Tan et al (Co-culturing epidermal keratinocytes and dermal fibroblasts on nano-structured titanium surfaces, Materials Science and Engineering: C, 2017, 78, 288–295), Silva et al (The PD-1: PD-L1 immune inhibitory checkpoint in Helicobacter pylori infection and gastric cancer: a comprehensive review and future perspectives, Porto Biomedical Journal, 2016, 1, 4–11). Chen et al (Interferon-γ-induced PD-L1 surface expression on human oral squamous via PKD2 signal pathway, Immunobiology, 2012, 217: 385–393).
Availability of data and materials
The datasets used and/or analyzed during the current study are available from the corresponding author on reasonable request.
Disclosure statement
No potential conflict of interest was reported by the authors.
Additional information
Funding
References
- Jemal A, Bray F, Center MM, et al. Global cancer statistics, 2011. CA Cancer J Clin. 2011;61:69–90.
- Bhat SA, Mir MUR, Majid S, et al. Diagnostic utility of glycosyltransferase mRNA expression in gastric cancer. Hematol Oncol Stem Cell Ther. 2018;11:158–168.
- Ren H, Xu Z, Guo W, et al. Rab3IP interacts with SSX2 and enhances the invasiveness of gastric cancer cells. Biochem Biophys Res Commun. 2018;503:2563–2568.
- Kato Y, Yashiro M, Noda S, et al. Establishment and characterization of a new hypoxia-resistant cancer cell line, OCUM-12/Hypo, derived from a scirrhous gastric carcinoma. Br J Cancer. 2010;102:898–907.
- Zhang W, Song Y. LINC00473 predicts poor prognosis and regulates cell migration and invasion in gastric cancer. Biomed Pharmacother. 2018;107:1–6.
- Pruszynski K, van Sandick JW, Mielko J, et al. Current challenges in gastric cancer surgery: European perspective. Surg Oncol. 2018;27:650–656.
- Liu J, Chen Q, Feng L, et al. Nanomedicine for tumor microenvironment modulation and cancer treatment enhancement. Nano Today. 2018;21:55–73.
- Meng W, Xue S, Chen X. The role of CXCL12 in tumor microenvironment. Gene. 2018;641:105–110.
- Lee NH, Nikfarjam M, He H. Functions of the CXC ligand family in the pancreatic tumor microenvironment. Pancreatology. 2018;18:705–716.
- Servais C, Erez N. From sentinel cells to inflammatory culprits: cancer-associated fibroblasts in tumor-related inflammation. J Pathol. 2013;229:198–207.
- Grimm S, Jennek S, Singh R, et al. Malignancy of bladder cancer cells is enhanced by tumor-associated fibroblasts through a multifaceted cytokine-chemokine loop. Exp Cell Res. 2015;335:1–11.
- Tripathi M, Billet S, Bhowmick NA. Understanding the role of stromal fibroblasts in cancer progression. Cell Adh Migr. 2012;6:231–235.
- Saigusa S, et al. Cancer-associated fibroblasts correlate with poor prognosis in rectal cancer after chemoradiotherapy. Int J Oncol. 2011;38:655–663.
- Ernsting EJ, Hoang B, Lohse I, et al. Targeting of metastasis-promoting tumor-associated fibroblasts and modulation of pancreatic tumor-associated stroma with a carboxymethylcellulose-docetaxel nanoparticle. J Control Release. 2015;206:122–130.
- Leef G, Thomas SM. Molecular communication between tumor-associated fibroblasts and head and neck squamous cell carcinoma. Oral Oncol. 2013;49:381–386.
- Zheng Z, Bu Z, Liu X, et al. Level of circulating PD-L1 expression in patients with advanced gastric cancer and its clinical implications. Chin J Cancer Res. 2014;26:104–111.
- Robert C, Soria JC, Eggermont AMM. Drug of the year: programmed death-1 receptor/programmed death-1 ligand-1 receptor monoclonal antibodies. Eur J Cancer. 2013;49:2968–2971.
- Alaghehbandan R, Stehlik J, Trpkov K, et al. Programmed death-1 (PD-1) receptor/PD-1 ligand (PD-L1) expression in fumarate hydratase-deficient renal cell carcinoma. Ann Diagn Pathol. 2017;29:17–22.
- Okuma Y, Wakui H, Utsumi H, et al. Soluble programmed cell death ligand 1 as a novel biomarker for nivolumab therapy for non-small-cell lung cancer. Clin Lung Cancer. 2018;19:410–417.
- Kim J, Kim S, Lee HS, et al. Prognostic implication of programmed cell death 1 protein and its ligand expressions in endometrial cancer. Gynecol Oncol. 2018;149:381–387.
- Shiraliyeva N, Friedrichs J, Buettner R, et al. PD-L1 expression in HNPCC-associated colorectal cancer. Pathol Res Practice. 2017;213:1552–1555.
- Baptista MZ, Sarian LO, Derchain S, et al. Prognostic significance of PD-L1 and PD-L2 in breast cancer. Human Pathol. 2016;47:78–84.
- Meng X, Liu Y, Zhang J, et al. PD-1/PD-L1 checkpoint blockades in non-small cell lung cancer: new development and challenges. Cancer Lett. 2017;405:29–37.
- Salgado R, Denkert C, Demaria S, et al. The evaluation of tumor-infiltrating lymphocytes (TILs) in breast cancer: recommendations by an International TILs Working Group 2014. Ann Oncol. 2015;26:259–271.
- Vicent S, Sayles LC, Vaka D, et al. Cross-species functional analysis of cancer-associated fibroblasts identifies a critical role for CLCF1 and IL-6 in non-small cell lung cancer in vivo. Cancer Res. 2012;72:5744–5756.
- Lin ZY, Chuang YH, Chuang WL. Cancer-associated fibroblasts up-regulate CCL2, CCL26, IL-6 and LOXL2 genes related to promotion of cancer progression in hepatocellular carcinoma cells. Biomed Pharmacother. 2012;66:525–529.
- Chan JA, Blaszkowsky LS, Enzinger PC, et al. A multicenter phase II trial of single-agent cetuximab in advanced esophageal and gastric adenocarcinoma. Ann Oncol. 2011;22:1367–1373.
- de Haas S, Delmar P, Bansal AT, et al. Genetic variability of VEGF pathway genes in six randomized phase III trials assessing the addition of bevacizumab to standard therapy. Angiogenesis. 2014;17:909–920.
- Noguchi E, Saito N, Kobayashi M, et al. Clinical significance of hepatocyte growth factor/c-Met expression in the assessment of gastric cancer progression. Mol Med Rep. 2015;11:3423–3431.
- Lu Y, Tang L, Zhang Q, et al. MicroRNA-613 inhibits the progression of gastric cancer by targeting CDK9. Artif Cells Nanomed Biotechnol. 2018;46:980–984.
- Jotzu C, Alt E, Welte G, et al. Adipose tissue-derived stem cells differentiate into carcinoma-associated fibroblast-like cells under the influence of tumor-derived factors. Anal Cell Pathol (Amst). 2010;33:61–79.
- Orimo A, Gupta PB, Sgroi DC, et al. Stromal fibroblasts present in invasive human breast carcinomas promote tumor growth and angiogenesis through elevated SDF-1/CXCL12 secretion. Cell. 2005;121:335–348.
- Yu Y, Xiao C-H, Tan L-D, et al. Cancer-associated fibroblasts induce epithelial-mesenchymal transition of breast cancer cells through paracrine TGF-beta signaling. Br J Cancer. 2014;110:724–732.
- Nakazawa K, Yashiro M, Hirakawa K. Keratinocyte growth factor produced by gastric fibroblasts specifically stimulates proliferation of cancer cells from scirrhous gastric carcinoma. Cancer Res. 2003;63:8848–8854.
- Zhi K, Shen X, Zhang H, et al. Cancer-associated fibroblasts are positively correlated with metastatic potential of human gastric cancers. J. Exp. Clin. Cancer Res. 2010;8:66.
- Jung DW, et al. Tumor-stromal crosstalk in invasion of oral squamous cell carcinoma: a pivotal role of CCL7. Int J Cancer. 2010;127:322–344.
- Gharaati-Far N, Tohidkia MR, Dehnad A, et al. Efficiency and cytotoxicity analysis of cationic lipids-mediated gene transfection into AGS gastric cancer cells. Artif Cells Nanomed Biotechnol. 2018;46:1001–1008.
- Wu M-H, Hong H-C, Hong T-M, et al. Targeting galectin-1 in carcimoma-associated fibroblasts inhibits oral squamous cell carcinoma metastasis by downregulating MCP-1/CCL2 expression. Clin Cancer Res. 2011;17:1306–1316.
- Johanna AJ, Jeffrey WP. Microenvironmental regulation of metastasis. Nat Rev Cancer. 2009;9:239–251.
- Dong H, Strome SE, Salomao DR, et al. Tumor-associated B7-H1 promotes T-cell apoptosis: a potential mechanism of immune evasion. Nat Med. 2002;8:793–800.
- Iwai Y, Ishida M, Tanaka Y, et al. Involvement of PD-L1 on tumor cells in the escape from host immune system and tumor immunotherapy by PD-L1 blockade. Proc Natl Acad Sci USA. 2002;99:12293–12297.
- Lei Z, Tan IB, Das K, et al. Identification of molecular subtypes of gastric cancer with different responses to PI3K inhibitor and 5-fluorouracil. Gastroenterology. 2013;145:554–565.
- Scherz-Shouval R, Santagata S, Mendillo ML, et al. The reprogramming of tumor stroma by HSF1 is a potent enabler of malignancy. Cell. 2014;158:564–578.
- Herbst RS, Soria JC, Kowanetz M, et al. Predictive correlates of response to the anti-PD-L1 antibody MPDL3280A in cancer patients. Nature. 2014;515:563–567.
- Zheng Z, Bu Z, Liu X, et al. Level of circulating PD-L1 expression in patients with advanced gastric cancer and its clinical implications. Clin J Cancer Res. 2014;26:104–111.
- Ghebeh H, Barhoush E, Tulbah A, et al. FOXP3+ Tregs and B7-H1+/PD-1+ T lymphocytes co-infiltrate the tumor tissues of high-risk breast cancer patients: implication for immunotherapy. BMC Cancer. 2008;23:57.
- Alsuliman A, Colak D, Al-Harazi O, et al. Bidirectional crosstalk between PD-L1 expression and epithelial to mesenchymal transition: significance in claudin-low breast cancer cells. Mol Cancer. 2015;14:149.
- Muro K, Bang Y, Shankaran V, et al. IBA15A phase IB study of pembrolizumab (PEMBRO;MK-3475) in patients (PTS) with advanced gastric cancer. Ann Oncol. 2014;(Suppl. 4):415–438.
- Ahmadzadeh M, Johnson LA, Heemskerk B, et al. Tumor antigen-specific CD8 T cells infiltrating the tumor express high levels of PD-1 and are functionally impaired. Blood 2009;114:1537–1344.
- Cho YA, Yoon HJ, Lee JI, et al. Relationship between the expressions of PD-L1 and tumor-infiltrating lymphocytes in oral squamous cell carcinoma. Oral Oncol. 2011;47:1148–1153.