Abstract
A new method has been developed for the simple, fluorescence, turn-on detection of melamine, which utilizes DNA-templated silver nanoclusters (DNA-AgNCs) as a key component. In the sensor, melamine exhibits the dual functions: one is to enhance the fluorescence signal of DNA-AgNCs by its specific interaction with thymine residues in DNA template, and the other is to prevent Hg(II)-induced fluorescence quenching of DNA-AgNCs via its strong coordination with Hg(II). These consequently enable the sensitive and selective detection of melamine. By exploiting such novel features of melamine, we significantly increased the fluorescence response up to 360%, compared to the previous counterpart that relies on DNA-AgNCs only, and successfully determined melamine down to ca. 49 nM, a value that is 400 times lower than the safety level of 20 μM set by the US Food and Drug Administration. In addition, it was confirmed that the proposed approach works fine even in the real milk samples without any additional pre-treatment steps.
Introduction
Since 1930, melamine has been widely used in the industry for the production of certain plastics [Citation1]. In addition, melamine with the highly rich-nitrogen composition has been artificially added to the milk or animal feed in order to increase the total nitrogen content in food [Citation2,Citation3]. However, melamine has severe toxicity, and thus the ingested melamine poses serious health problems such as kidney stone formation, urolithiasis, and bladder cancer [Citation4]. For these reasons, it is highly required to develop the simple, but efficient analysis system for melamine [Citation5–7].
Notably, fluorescence methods, compared to other analytical methods such as chromatography, mass spectrometry, and NMR spectroscopy, have gained the considerable attention due to their intrinsic simplicity, robustness, and high sensitivity [Citation8–13]. The representative example in this type sensing strategy relies on DNA-templated silver nanoclusters (DNA-AgNCs) that possess the unique fluorescence properties modulated by DNA sequence and structure [Citation14–17]. Based on the specific interaction between melamine and thymine residues of DNA-AgNCs, the fluorescence detection system for melamine was devised [Citation18]. Although it produced the promising results, the signal enhancement induced by melamine was quite small due to the high background signal, limiting the wide-spread and practical applications. To overcome this limitation, Xie et al. recently proposed that Hg(II)-quenching of DNA-AgNCs could be utilized to detect melamine by reducing the background signal [Citation19]. In a similar manner, BSA-templated fluorescent gold nanoclusters that are quenched by Hg(II) was confirmed to detect melamine with the reduced background signal [Citation20].
In this work, we advanced the fluorescence strategy for the sensitive detection of melamine by designing the DNA sequence that not only serves as the template for the synthesis of fluorescent DNA-AgNCs but also interacts with melamine. Compared to the previous methods in which melamine only works as either the fluorescence enhancer of DNA-AgNCs or the scavenger to reverse Hg(II)-quenching of DNA-AgNCs [Citation18,Citation19], melamine in this work has dual functions, both for enhancing the fluorescence signal of DNA-AgNCs by its specific interaction with thymine residues and preventing Hg(II)-induced fluorescence quenching of DNA-AgNCs via its strong coordination with Hg(II) [Citation21]. With this working principle, we successfully determined melamine even in real milk samples down to ca. 49 nM, which is two or three times more sensitive than those of the previous, Hg(II)-quenching methods [Citation19,Citation20].
Experimental
Reagents and materials
Oligonucleotide (5′-CCCTTAATCCCC-3′) was synthesized using Bioneer (Daejeon, Korea) and purified via Bio-RP. All amino acids, monobasic, and dibasic phosphates were purchased from Samchun Chemical (Seoul, Korea). Melamine, mercury (II) nitrate monohydrate (Hg(NO3)2·H2O), sodium borohydride (NaBH4), and silver nitrate (AgNO3) were purchased from Sigma-Aldrich (Goyang, Korea). All buffer solutions and reagents were prepared in Ultrapure DNase/RNase-free distilled water (D.W.) purchased form Bioneer (Daejeon, Korea).
Preparation of DNA-AgNCs
To synthesize 1X DNA-AgNCs, DNA (6 µM) was incubated with AgNO3 (36 µM) in 1X phosphate buffer (10 mM, pH 7.4) for 30 min at room temperature in the dark. To this solution, NaBH4 (18 µM) was added in a total volume of 100 µL, which were stored at refrigerator at 4 °C before use. The molar ratio of DNA: AgNO3:NaBH4 was 1:6:3.
Detection of melamine
Melamine at different concentrations or amino acids (30 µM) were incubated with mercury (150 nM) for 10 min, which was then mixed with the prepared 0.2X DNA-AgNCs in 1X phosphate buffer (10 mM, pH 7.4) to make a total volume of 100 µL. Following the incubation of the prepared mixtures for 10 min at room temperature, the resulting fluorescence signal was measured at the excitation wavelength of 597 nm in a microplate reader (Spectramax iD5 multi-mode microplate reader, Molecular Devices, USA).
Detection of melamine in milk sample
Melamine at different concentrations or amino acids (30 µM) were incubated with mercury (150 nM) for 10 min, which was then mixed with the prepared 0.2X DNA-AgNCs in 1X phosphate buffer (10 mM, pH 7.4) to make a total volume of 100 µL. Following the incubation of the prepared mixtures for 10 min at room temperature, the resulting fluorescence signal was measured at the excitation wavelength of 597 nm in a microplate reader (Spectramax iD5 multi-mode microplate reader, Molecular Devices, USA).
Results and discussion
Melamine detection based on its dual functions
The working principle of the fluorescence, turn-on strategy for the sensitive detection of melamine is illustrated in . As a key component, DNA template, 5′-CCCTTAATCCCC-3′, is utilized to synthesize fluorescent DNA-AgNCs. We assumed that the presence of melamine itself enhances the fluorescence signal of DNA-AgNCs on the basis of the specific interaction between melamine and thymine residues of DNA template [Citation18]. In addition, Hg(II)-induced fluorescence quenching of DNA-AgNCs is employed to reduce the background signal, which is expected to maximize the signal enhancement effect induced by melamine that forms the complexes with Hg(II) [Citation19–21]. As a result, the melamine, which possesses the dual functions as fluorescence enhancer of DNA-AgNCs and Hg(II)-scavenger can be sensitively determined with the signal-on fluorescence response as shown in .
Figure 1. (a) The schematic illustration for the fluorescence, turn-on detection of melamine based on its dual functions as fluorescence enhancer of DNA-AgNCs and Hg(II) scavenger. (b) Photographs under UV light (354 nm). The left and right samples show the case in the absence and presence of melamine, respectively.
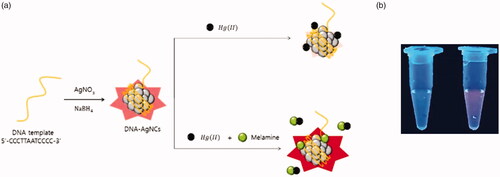
Detection feasibility
First, the detection feasibility of the designed sensor was confirmed by measuring the fluorescence emission intensities at 670 nm (F670), an emission maximum of DNA-AgNCs. As shown in , the presence of Hg(II) significantly reduced the background fluorescence signal of DNA-AgNCs (i and iii). On the other hand, the presence of melamine together with Hg(II) significantly enhanced the fluorescence signal even higher than that of DNA-AgNCs only (i, iii, and iv). Importantly, the degree of signal enhancement, defined as F/F0, where F0 and F are the fluorescence emission intensities at 670 nm in the absence and presence of melamine, respectively, was increased up to 360%, compared to the one in the absence of Hg(II) (i–iv), proving that the developed method is highly advanced for the sensitive determination of melamine. In addition, the control DNA in which thymine residues are not included was utilized to synthesize DNA-AgNCs and the effect of melamine on the fluorescence enhancement of DNA-AgNCs was evaluated. The results in Figure S1 show that the presence of melamine produces the negligible fluorescence enhancement in both cases without (i and ii) and with Hg(II) (iii and iv) (Figure S1), supporting the importance of dual functions of melamine in the proposed system.
Figure 2. The detection feasibility of the developed method. (a) The fluorescence emission spectra and (b) fluorescence intensity at 670 nm (F670) of DNA-AgNCs (i), DNA-AgNCs + melamine (ii), DNA-AgNCs + Hg(II) (iii), and DNA-AgNCs + melamine + Hg(II) (iv).
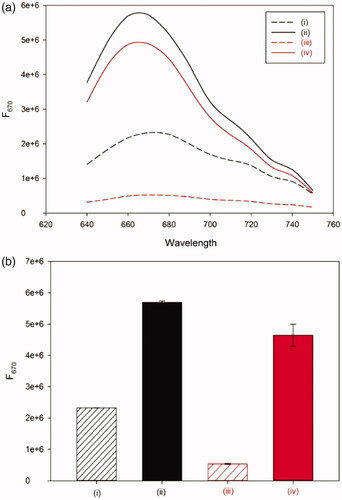
Next, the optimal conditions including the concentration of Hg(II), DNA-AgNCs and the addition steps of reagents were investigated for the efficient analysis of melamine. The results in Figure S2 show that the fluorescence signal of DNA-AgNCs is decreased as the concentration of Hg(II) is increased due to the quenching effect of Hg(II). Because the excessive amount of Hg(II) could lead to the non-productive complexation of Hg(II) with melamine, 150 nM was selected as the optimal concentration of Hg(II). In the same manner, the optimal concentration of DNA-AgNCs (0.2×) was selected by varying the amount of DNA-AgNCs in the presence of Hg(II) at the concentration of 150 nM (Figure S3). In addition, it was demonstrated that the addition steps of melamine to the assay solution consisting of DNA-AgNCs and Hg(II) could affect the detection performance. As shown in Figure S4, when melamine was first applied to DNA-AgNCs, followed by the addition of Hg(II) (i), the signal-to-background ratio defined as (F–F0)/F0, where F0 and F are the fluorescence emission intensities at 670 nm in the absence and presence of melamine, respectively, was ca. 4.6, which was higher than that of the reversed case in which Hg(II) was first applied, followed by the addition of melamine (ii). Importantly, the highest fluorescence enhancement (ca. 7.9) was observed when melamine and Hg(II) were first mixed and then applied to DNA-AgNCs (iii). Based on these optimization results, the assay procedure that involves the addition of DNA-AgNCs to the pre-mixed solution of melamine with Hg(II) was selected for further experiments (See Materials and Methodsfor details).
Figure 3. Detection sensitivity of the developed method. (a) The fluorescence emission spectra and (b) signal-to-background ratio of DNA-AgNCs in presence of melamine at different concentrations (From bottom to top: 0, 150 nM, 1.5 μM, 3 μM, 7.5 μM, 15 μM, 30 μM, 75 μM, and 150 μM). The signal-to-background ratio is defined as (F–F0)/F0, where F0 and F are the fluorescence emission intensities at 670 nm (F670) in the absence and presence of melamine, respectively.
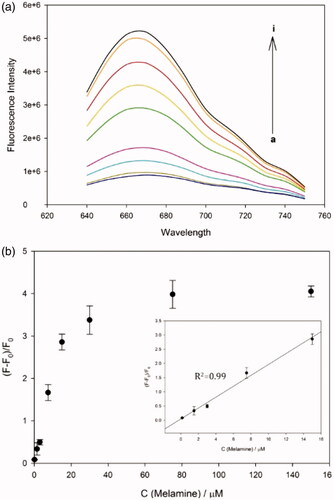
Detection sensitivity and selectivity
Under the optimized conditions, the detection sensitivity of the developed system was evaluated by measuring F670 in the presence of melamine at different concentrations. The results in show that the signal-to-background ratio, (F–F0)/F0, increases as the concentration of melamine increases up to 75 μM, over which it reaches a plateau. The excellent linear relationship (R2 = 0.99) was established in the range from 0 to 15 μM by the linear equation, (F–F0)/F0 = 0.1918 × C (Melamine) + 0.0465. The detection limit was calculated to be ca. 49 nM (3σ/slope), a value that is superior to those in the previous methods that rely on Hg(II)-quenching of fluorescent nanomaterials [Citation19,Citation20] (Table S1). These results confirm that the dual functions of melamine as the fluorescence enhancer of DNA-AgNCs and Hg(II) scavenger are critical for the sensitive determination of melamine.
Table 1. Determination of melamine in milk samples.
To assess the detection selectivity of the developed strategy, amino acids including Cys, Trp, Pro, Glu, Gly, Lys, Met, Arg, and Phe was employed instead melamine. As shown in , the presence of melamine significantly enhanced the fluorescence signal, while amino acids resulted in the negligible fluorescence enhancement, which was clearly distinguished from that observed in the presence of melamine.
Practical application to the analysis of real milk samples
Finally, the practical applicability of the proposed method was demonstrated by performing the recovery tests with the melamine spiked into real milk samples. Based on the calibration curve created with the standard samples (Figure S5), the concentration of melamine in milk samples was determined by measuring F670 from unknown samples. The results in verified that the melamine in the milk samples was analyzed with high precision and reproducibility, which was evidenced by the coefficients of variation (CV) less than 3.5% and the recovery ratio between 96% and 111%. It should be noted that the developed method does not involve the tedious procedures to separate the proteins using acetonitrile or trichloroacetic acids that are generally required in the previous methods [Citation19,Citation20,Citation22,Citation23].
Conclusion
In summary, we developed a simple, fluorescence, turn-on strategy for the sensitive detection of melamine based on its dual functions as fluorescence enhancer of DNA-AgNCs and Hg(II) scavenger. This strategy significantly improved the signal enhancement effect promoted by melamine up to 360%, compared to the counterpart that relies on DNA-AgNCs only. In addition, melamine was analyzed with the high sensitivity and selectivity, which was confirmed by the low detection limit of 49 nM, two or three times more sensitive than those of the previous, Hg(II)-quenching methods. Finally, the practical applicability of the developed method was successfully demonstrated by detecting melamine in milk samples. We believe that this method which relies on the dual functions of melamine will facilitate the sensitive analysis of melamine and will be utilized as a practical tool in the food industry.
ACNB_Supplementary-Park.doc
Download ()Disclosure statement
No potential conflict of interest was reported by the authors.
Additional information
Funding
References
- Domene LAF, Ayres RU. Nitrogen’s role in industrial system. J Ind Ecol. 2001;5:77–103.
- Andersen WC, Turnipseed SB, Karbiwnyk CM, et al. Determination and confirmation of melamine residues in catfish, trout, tilapia, salmon, and shrimp by liquid chromatography with tandem mass spectrometry. J Agric Food Chem. 2008;56:4340–4347.
- Ingelfinger JR. Melamine and the global implications of food contamination. N Engl J Med. 2008;359:2745–2748.
- Hau A, Kwan TH, Li P. Melamine toxicity and the kidney. J Am Soc Nephrol. 2009;20:245–250.
- Finete V, Gouvêa M, Margues F, et al. Characterization of newfound natural luminescent properties of melamine, and development and validation of a method of high performance liquid chromatography with fluorescence detection for its determination in kitchen plastic ware. Talanta. 2014;123:128–134.
- Li Y, Xu J, Sun C. Chemical sensors and biosensors for the detection of melamine. RSC Adv. 2015;5:1125–1147.
- Rovina K, Siddiquee S. A review of recent advances in melamine detection techniques. J Food Compost Anal. 2015;43:25–38.
- Wang Y, Liu B. ATP detection using a label-free DNA aptamer and a cationic tetrahedralfluorene. Analyst. 2008;133:1593. 1598
- Zhang L, Cai QY, Li J. A label-free method for detecting biothiols based on poly(thymine)-templated copper nanoparticles. Biosens Bioelectrons. 2015;93:293–297.
- Lee CY, Park KS, Jung YK, et al. A label-free fluorescent assay for deoxyribonuclease I activity based on DNA-templated silver nanocluster/graphene oxide nanocomposite. Biosens. Bioelectrons. 2017;93:293–297.
- Filigenzi MS, Puschner B, Aston LS, et al. Diagnostic determination of melamine and related compounds in kidney tissue by liquid chromatography/tandem mass spectrometry. J Agric Food Chem. 2008;56:7593–7599.
- Zhu L, Gamez G, Chen H, et al. Rapid detection of melamine in untreated milk and wheat gluten by ultrasound-assisted extractive electrospray ionization mass spectrometry. Chem Comm. 2009;559–561.
- Lachenmeier DW, Humpfer E, Fang F, et al. NMR-spectroscopy for nontargeted screening and simultaneous quantification of health-relevant compounds in foods: the example of melamine. J Agric Food Chem. 2009;57:7194–7199.
- Diez I, Ras RHA. Fluorescent silver nanoclusters. Nanoscale. 2011;3:1963–1970.
- Xu H, Suslick KS. Water-soluble fluorescent silver nanoclusters. Adv Mater Weinheim. 2010;22:1078–1082.
- Lan GY, Chen WY, Chang HT. Characterization and application to the detection of single-stranded DNA binding protein of fluorescent DNA-templated copper/silver nanoclusters. Analyst. 2011;136:3623. 3628
- Kim H, Kang S, Park KS, et al. Enzyme-free and label-free miRNA detection based on target-triggered catalytic hairpin assembly and fluorescence enhancement of DNA-silver nanoclusters. Sensor Actuat B-Chem. 2018;260:140–145.
- Han S, Zhu S, Liu Z, et al. Oligonucleotide-stabilized fluorescent silver nanoclusters for turn-on detection of melamine. Biosens Bioelectrons. 2012;36:267–270.
- Xie P, Zhan Y, Wu M, et al. The detection of melamine base on a turn-on fluorescence of DNA-Ag nanoclusters. J Lumin. 2017;186:103–108.
- Dai H, Shi Y, Wang Y, et al. Label-free turn-on fluorescent detection of melamine based on the anti-quenching ability of Hg2+ to gold nanoclusters. Biosens Bioelectron. 2014;53:76–81.
- Du J, Yin S, Jiang L, et al. A colorimetric logic gate based on free gold nanoparticles and the coordination strategy between melamine and mercury ions. Chem Commun. 2013;49:4196.
- Ding N, Yan N, Ren C, et al. Colorimetric determination of melamine in dairy products by Fe3O4 magnetic nanoparticles-H2O2-ABTS detection system. Anal Chem. 2010;82:5897–5899.
- Gao F, Ye Q, Cui P, et al. Efficient fluorescence energy transfer system between CdTe-doped silica nanoparticles and gold nanoparticles for turn-on fluorescence detection of melamine. J Agric Food Chem. 2012;60:4550–4558.