Abstract
Shikonin, a botanical drug extracted from Lithospermum erythrorhizon, exhibits anti-cancer effects in various cancer cell lines. However, the mechanisms underlying these effects have not been completely elucidated yet. Here, we showed that Shikonin induces apoptosis and autophagy in A375 cells and inhibits their proliferation. Shikonin caused G2/M phase arrest through upregulation of p21 and downregulation of cyclin B1. Shikonin significantly triggered ER stress-mediated apoptosis by upregulating the expression of p-eIF2α, CHOP, and cleaved caspase-3. It also induced protective autophagy by activating the p38 pathway, followed by an increase in the levels of p-p38, LC3B-II, and Beclin 1. Upon suppression of autophagy by 3-methyladenine, Shikonin-induced apoptosis was enhanced in A375 cells. Moreover, after pretreatment with N-acetyl-cysteine, Shikonin increased the production of reactive oxygen species that are involved in regulating ER stress-mediated apoptosis and p38-activated autophagy, as evidenced by the reversion of cell viability and apoptosis and a decrease in p-eIF2α, CHOP, p-p38, LC3B-II, and Beclin 1 levels. Thus, we demonstrated that Shikonin induced apoptosis and autophagy in A375 cells via the activation of ROS-mediated ER stress and p38 pathways, indicating that Shikonin can serve as a potential agent for human melanoma therapy.
Introduction
Human melanoma is the most lethal form of skin cancer, showing increasing incidence and poor treatment and prognosis worldwide [Citation1,Citation2]. Presently, surgical removal of the tumour, radiotherapy, chemotherapy, and immune and molecular-targeted therapies are used to treat melanoma [Citation3]. Surgical clearance followed by adjuvant chemotherapy remains the best option among all therapies. Unfortunately, most patients develop primary or acquired resistance to chemotherapy [Citation4]. Thus, exploration of new therapeutic agents against melanoma progression is an urgent need for these patients.
Shikonin is an active component that is extracted from the roots of Lithospermum erythrorhizon (), a traditional medicinal Chinese herb, which has been used for treating various diseases including macular eruptions, burns, sore throats, and HIV-1 infection [Citation5,Citation6]. Recently, many studies have illustrated that Shikonin exerts anti-cancer effects through the suppression of proliferation and induction of apoptosis and autophagy in different cancer cells. Previous results have demonstrated that Shikonin induces apoptosis in osteosarcoma cells through increased reactive oxygen species (ROS) generation and ERK activation [Citation7]. Shikonin has also been reported to cause accumulation of ROS and p-ERK, and thus induce autophagy in human hepatocellular carcinoma [Citation8]. Moreover, Shikonin-induced apoptosis was caused by ROS-mediated activation of Akt/ASK1/p38-MAPK and downregulation of p21 in several cancer cells [Citation9]. In addition, Gara et al. [Citation10] reported that Shikonin promotes apoptosis by activating the ER stress pathway and the mitochondrial apoptotic pathway in human prostate cancer cells. However, the exact mechanism underlying the anti-cancer effect of Shikonin in human melanoma cells has not been thoroughly investigated.
Figure 1. Inhibitory effects of Shikonin on A375 cells. (A) Chemical structure of Shikonin. (B) A375 cells were treated with different concentrations of Shikonin (0–16 μM) for 24 h. (C) A375 cells were treated with the indicated concentration of Shikonin for 24 h, and morphological changes were visualized under the inverted microscopy. A representative result from three separate experiments is shown. ****p < .0001 compared with the control group.
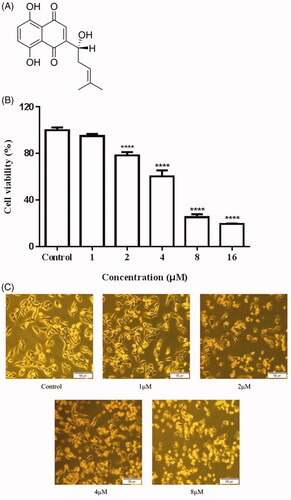
In the present study, we aimed to demonstrate the link among ROS, ER stress, and the p38 pathway, and focus on their roles in the regulation of Shikonin-induced apoptosis and autophagy in human melanoma A375 cells.
Materials and methods
Cells, reagents, and antibodies
Human melanoma A375 cells were obtained from Cell Bank, Chinese Academy of Sciences (Shanghai, China). The cells were cultured in Dulbecco's Modified Eagle Medium (DMEM), supplemented with heat inactivated 10% foetal bovine serum (FBS) and 1% penicillin/streptomycin at 37 °C in a humidified atmosphere with 5% CO2. Shikonin with purity greater than 98%, 3-methyladenine (3-MA), N-acetyl cysteine (NAC), and SB203580 were purchased from Sigma Aldrich (St. Louis, MO). The anti-p38, anti-p-p38, anti-Caspase-3, anti-cyclin B1, and anti-p-eIF2α, anti-CHOP, anti-LC3B, anti-Beclin 1, and β-actin antibodies were purchased from Cell Signalling Technology (Danvers, MA). The anti-p21 antibody was purchased from R&D Systems (Minneapolis, MN).
Cell viability assay
Cell viability was evaluated using the cell counting kit (CCK)-8 assay. A375 cells were seeded at a density of 2 × 104 cells/well into 96-well plates and stabilized at 37 °C for 24 h. The cells were then incubated with different concentrations of Shikonin (0–16 μM) for 24 h. After incubation, CCK-8 was added to the wells for another 2 h. Absorbance at 450 nm was measured using a microplate reader. Cell viability relative to the control was calculated as follows: survival ratio (%) = (A treatment/A control) × 100. Additionally, morphological changes in A375 cells treated with different concentrations of Shikonin for 24 h were also monitored using an inverted microscope.
Cell-cycle analysis
Cell-cycle distribution was examined by flow cytometry. A375 cells (1 × 106 cells/well) were seeded in 6-well plates and incubated with different concentrations of Shikonin (0, 1, 2, and 4 μM) for 24 h. The cells were then collected by centrifugation (1000 rpm, 5 min), washed with ice-cold phosphate-buffered saline (PBS), and fixed overnight with 70% ethanol at 4 °C. Prior to detection, the cells were washed twice with PBS, incubated with 100 μL RNase A for 30 min at 37 °C, and stained with 400 μL propidium iodide (PI) for another 30 min at 4 °C in the dark using Cell Cycle Detection Kit (keyGEN BioTECH, Nanjing, China). Finally, samples were analyzed on a flow cytometer (FACSCalibur, BD Biosciences, San Jose, CA), using DNA Modelling Software to determine the population of cells in each cycle phase.
Detection of apoptosis by flow cytometry and Hoechst 33258 staining
First, Hoechst 33258 staining was performed to assess apoptosis. A375 cells were seeded in 6-well plates and cultured overnight. After exposure to a medium containing Shikonin (0, 1, 2, and 4 μM) for 24 h, cells were fixed in methanol for 30 min and washed thrice with PBS. Next, the cells were incubated with Hoechst 33258 dye at room temperature in the dark for 10 min. The cells were then washed thrice with PBS and observed immediately under an IX53 fluorescence microscope (Olympus, Tokyo, Japan).
For the detection of apoptosis by flow cytometry, A375 cells were plated at a density of 1 × 106 cells/well on 6-well plates and grown overnight. The cells were then treated with increasing Shikonin concentrations (0, 1, 2, and 4 μM) for 24 h. The treated cells were collected by centrifugation, washed twice with ice-cold PBS, and resuspended at 2 × 105 cells/mL in 500 μL binding buffer to which 5 μL of Annexin V fluorescein isothiocyanate (FITC) was added and mixed well following Apoptosis Detection Kit (keyGEN BioTECH, Nanjing, China). Then, 5 μL of PI was added and cells were incubated for 15 min at room temperature in the dark. After staining, samples were analyzed by flow cytometry (FACSCalibur, BD Biosciences, San Jose, CA) within 1 h. Annexin V+/PI ± were considered apoptotic cells. The proportion of apoptotic cells was expressed as a percentage of the total cell number acquired, excluding debris, and was analyzed using FlowJo 7.6 software (SPSS Inc., Chicago, IL).
Reactive oxygen species (ROS) detection
Intracellular ROS production was measured using an ROS assay kit (Jiancheng Bioengineering Institute, Nanjing, China) and flow cytometry. A375 cells were plated on 6-well plates, at 1 × 106 cells/well. The following day, cells were treated with serial dilutions of Shikonin (0, 1, 2, and 4 μM) for 6 h. The cells were then incubated for 30 min in PBS containing 10 μM DCFH-DA. The stained cells were collected by centrifugation and washed twice with PBS. Cells resuspended in PBS were analyzed immediately by flow cytometry (FACSCalibur, BD Biosciences, San Jose, CA). The mean fluorescence intensity was analyzed using FlowJo 7.6 software (SPSS Inc., Chicago, IL).
Western blotting analysis
A375 cells were grown in 35 mm dishes at a density of 2 × 106 cells/dish with 2 ml complete DMEM medium for 24 h and were then treated with 2 μM of Shikonin for 3, 6, and 12 h. Cells were then lysed in a RIPA lysis buffer (150 mmol/L NaCl, 50 mmol/L Tris–HCl, pH 7.4, 1% Triton X-100, 1% sodium deoxycholate, 0.1% SDS) with 1 mM sodium orthovanadate, 1 mM PMSF, and 1% cocktail of protease inhibitors (Sigma, St. Louis, MO). Equal amounts of protein were separated by 8–15% SDS-PAGE and transferred onto nitrocellulose membranes (Millipore, Billerica, MA). After blocking with 5% skim milk for 2 h, the membrane was probed overnight with the primary antibodies (1:1000) against caspase-3, p38, p-p38, cyclin B1, p-eIF2α, CHOP, LC3B, Beclin-1, p21 and β-actin at 4 °C, followed by incubation with the corresponding secondary antibodies (1:20,000) for 2 h at room temperature. β-Actin was used as the loading control. The signal was detected with SuperSignalTM ELISA Femto Maximum Sensitivity Substrate (Thermo Scientific, Waltham, MA).
Statistical analysis
All data were expressed as the mean ± SD (standard division). One-way ANOVA with Dunnett’s post hoc test and GraphPad PRISM (Graph Pad Software, San Diego, CA) were used to determine the statistical significance of observed differences. All p values less than .05 were considered statistically significant.
Results
Inhibitory effects of Shikonin on A375 cells
The A375 cells were incubated with different concentrations of Shikonin (0, 1, 2, 4, 8, and 16 μM) for 24 h and cell viability was determined using the CCK-8 assay. As shown in , Shikonin exhibited an anti-proliferative effect on A375 cells and significantly suppressed the growth of A375 cells in a dose-dependent manner. Morphological observation also showed that Shikonin dose-dependently decreases the percentage of surviving cells ().
Cell cycle was arrested at the G2/M phase and cell-cycle proteins were affected by Shikonin in A375 cells
The cell-cycle distribution was analyzed using PI staining and flow cytometry. As displayed in , a significant increase in the percentage of the G2/M phase was observed in A375 cells after the treatment with Shikonin compared with the control group (4.42 ± 1.08%). Moreover, Shikonin induced cell-cycle arrest of A375 cells in the G2/M phase in a dose-dependent manner, and the percentages of cells in the G2/M phase were 4.06 ± 1.88%, 11.62 ± 1.18%, and 23.08 ± 2.36% in the 1, 2, and 4 μM groups, respectively. Additionally, the expression of the cell cycle-related proteins p21 and cyclin B1 (plays an important role in DNA replication during the G2/M phase) was changed dramatically in A375 cells after Shikonin treatment in a time-dependent manner. As shown in , cyclin B1 expression was attenuated in Shikonin-treated A375 cells, whereas p21, an important cyclin-dependent kinase inhibitor, was obviously increased. These results suggested that Shikonin induces cell-cycle arrest at the G2/M phase in A375 cells by downregulating cyclin B1 and upregulating p21.
Figure 2. Effects of Shikonin on cell-cycle distribution and cycle-associated proteins in A375 cells. (A) A375 cells were incubated with different concentrations of Shikonin for 24 h. The percentage of A375 cells in G0/G1, S, and G2/M phase was measured by flow cytometry. (B) A375 cells treated with Shikonin (2 μM) for 3, 6, and 12 h. Western blotting was performed for the detection of cyclin B1 and p21. β-Actin was used as a loading control. A representative result from three separate experiments is shown. ** p < .01, **** p < .0001 compared with the control group.
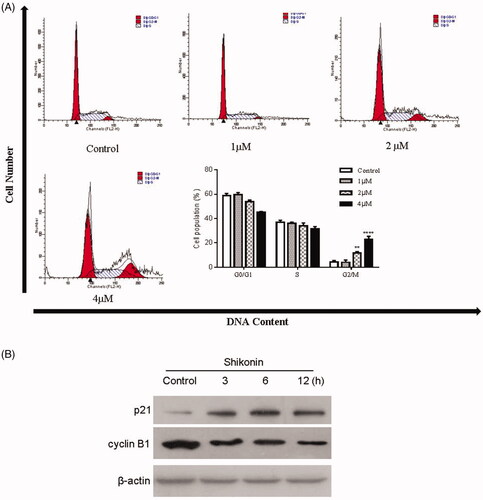
Shikonin induced apoptosis of A375 cells
Changes in the A375 cell nucleus were observed under a fluorescence microscope after staining the cells with Hoechst 33258. As shown in , Shikonin treatment resulted in typical apoptotic nuclear changes such as enhanced blue fluorescence. Flow cytometry was used to detect the percentage of apoptosis in A375 cells treated with Shikonin (0, 1, 2, and 4 μM) for 24 h. The proportion of apoptotic A375 cells was increased dramatically in a dose-dependent manner. The percentage of apoptotic cells was 5.58 ± 0.90%, 8.04 ± 1.33%, 18.83 ± 2.99%, and 38.85 ± 3.44% in the 0, 1, 2, and 4 μM groups, respectively (). Western blot analysis was used to detect the expression of related proteins in the apoptotic pathway. As shown in , Shikonin significantly increased the level of cleaved caspase-3 in a time-dependent manner. These results indicated that Shikonin induced apoptosis by increasing the level of cleaved caspase-3 in A375 cells.
Figure 3. Shikonin induces apoptosis in A375 cells. (A) After treated with Shikonin (0, 1, 2, and 4 μM) for 24 h, the A375 cells were incubated with Hoechst 33258 staining. Apoptotic cells exhibited karyopyknosis. (B) After treatment with increased doses of Shikonin (0, 1, 2, and 4 μM) for 24 h, induction of apoptosis in A375 cells measured by Annexin V/PI double-staining assay followed by flow cytometry analysis. (C) A375 cells treated with Shikonin (2 μM) for 3, 6, and 12 h. Effects of Shikonin on the expression of apoptosis-related proteins. β-Actin was measured as an internal control. The image shown is representative of three separate experiments. ***p < .01, ****p < .0001 compared with the control group.
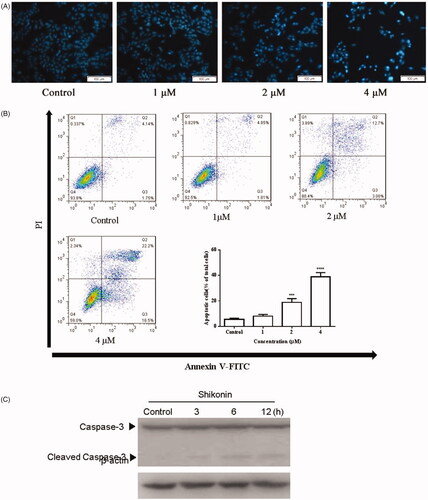
Shikonin induced apoptosis mediated by ROS generation
Shikonin has been reported to enhance cisplatin-induced apoptosis by inducing ROS generation in colon cancer cells [Citation11]. Therefore, we investigated the intracellular ROS production in A375 cells using DCFH-DA staining followed by flow cytometry. As shown in , ROS generation was increased in A375 cells treated with Shikonin in a dose-dependent manner. Next, the treatment of A375 cells with NAC, an ROS scavenger, was used to explore the role of ROS in Shikonin-induced apoptosis. A375 cells were pre-incubated with 8 mM NAC for 1 h, followed by Shikonin treatment for 24 h to detect the effect of ROS on apoptosis. The results showed that NAC pretreatment significantly inhibits Shikonin-induced apoptosis (). These findings demonstrated the role of ROS as a potential initiator to induce apoptosis in A375 cells.
Figure 4. Shikonin induces apoptosis mediated by ROS generation in A375 cells. (A) A375 cells were treated with different concentrations of Shikonin for 6 h. Flow cytometry was used to detect the levels of ROS. (B) A375 cells were pretreated with NAC (8 mM) for 1 h, and then Shikonin (2 μM) was added to the cells for 24 h. Flow cytometry was used to detect the change of apoptosis in A375 cells. A representative result from three separate experiments is shown. **p < .01, ****p < .0001 compared with the control group.
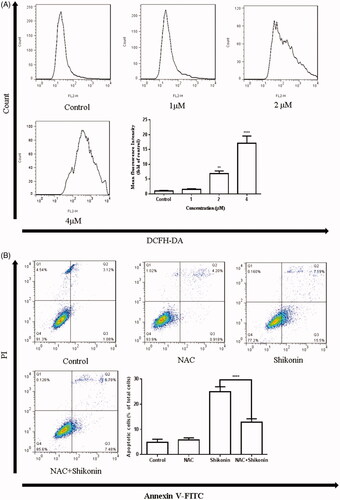
Shikonin induced apoptosis of A375 cells via ROS-mediated ER stress signal pathway
Emerging evidence suggests that ER stress plays an important role in apoptosis-related pathways. Recently, studies confirmed that Shikonin can cause ER stress involved in apoptosis of human prostate cancer cells [Citation10]. In this study, ER stress-related proteins were assessed by western blot analysis. The expression of p-eIF2α and CHOP was elevated in Shikonin-treated cells (). The results showed that Shikonin treatment significantly activates ER stress in a time-dependent manner in A375 cells. A previous study showed that Shikonin induces ER-stress-mediated apoptosis, and this activity could be regulated by ROS generation [Citation10]. Therefore, NAC was used to explore the role of ROS in Shikonin-induced ER stress in A375 cells. These cells were pre-incubated with NAC for 1 h, and then treated with Shikonin. Western blot analysis revealed that pre-treatment with NAC decreased the expression of p-eIF2α and CHOP (). These results indicated that Shikonin induces apoptosis in A375 cells through ER stress that is mediated by ROS generation.
Figure 5. Shikonin induces apoptosis of A375 cells via ROS-mediated ER stress signalling pathway. (A) A375 cells were treated with Shikonin (2 μM) for 3, 6, and 12 h. Western blotting was used to detect the levels of protein associated with ER stress activation. (B) A375 cells were pretreated with NAC (8 mM) for 1 h, and then Shikonin (2 μM) was added to the cells for 6 h. Western blotting was used to detect the changes of p-eIF2α and CHOP. β-Actin was measured as an internal control. The image shown is representative of three separate experiments. **p < .01, *** p < .001, ****p < .0001 compared with the control group.
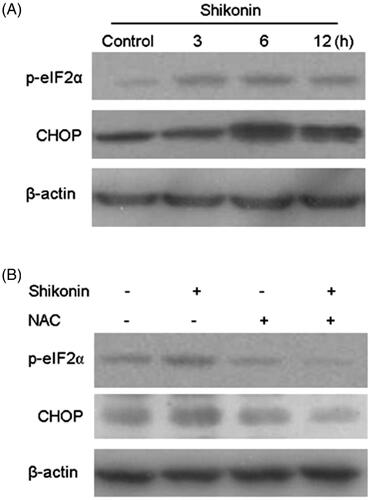
Shikonin induced cytoprotective autophagy in A375 cells via the ROS/p38 signal pathway
Western blot analysis was performed to investigate the expression levels of autophagy-related proteins. As shown in , an obvious increase in the expression of LC3B-II and Beclin 1 was observed after A375 cells were treated with 2 μM of Shikonin for different time spans. These results show that Shikonin could induce autophagy in A375 cells. To further confirm whether autophagy affected Shikonin-induced apoptosis, cells were pretreated with 3-MA, a specific autophagy inhibitor (preventing autophagy at an early stage), along with Shikonin. There was a decrease in cells viability and an increase in apoptosis triggered by Shikonin ()). These results indicate that inhibition of autophagy further reduced the Shikonin-inhibited growth and enhanced the apoptosis induced by Shikonin. These data suggest that Shikonin-induced autophagy protects against apoptosis in A375 cells.
Figure 6. Shikonin induces ROS/p38-mediated protective autophagy to against apoptosis in A375 cells. (A) Western blot was performed to analyze the expression of autophagic markers after incubating A375 cells with 2 μM Shikonin for 3, 6, and 12 h. After pretreatment with 3-MA (5 mM) 1 h, and then incubated with 2 μM Shikonin for 24 h, the changes of cell viability (B) and apoptosis (C) were detected by CCK-8 assay and flow cytometry in A375 cells. (D) Western blot analysis of p-p38 after incubating A375 cells with 2 μM Shikonin for 3, 6, and 12 h. (E) The cell viability of A375 cells was determined by CCK-8 assay after pretreated with SB203580 (5 μM) for 1 h followed by 2 μM Shikonin for 24 h. (F) A375 cells were pretreated with NAC (8 mM) for 1 h followed by 2 μM Shikonin for 6 h to detect the effects of ROS on p38-mediated autophagy. β-Actin was measured as an internal control. The image shown is representative of three separate experiments. ***p < .001, ****p < .0001 compared with the control group.
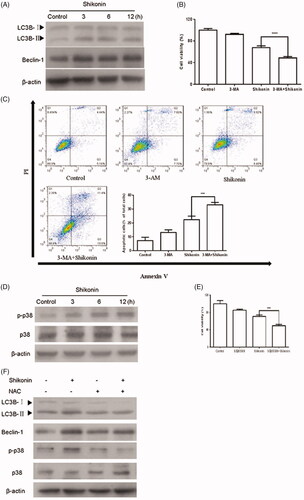
SB203580 (a specific inhibitor of the p38 pathway) was used to investigate the effect of p38 on Shikonin-induced apoptosis. As shown in , phosphorylation of p38 was significantly increased in A375 cells upon Shikonin treatment. Furthermore, Shikonin-induced growth inhibition was enhanced significantly after pretreatment with SB203580 (). The results showed that SB203580 increases the sensitivity of A375 cells to Shikonin. Moreover, Shikonin has been reported to induce p38-mediated autophagy through ROS generation in HT1080 cells [Citation12]. Thus, NAC was used to explore the role of ROS in p38-mediated autophagy in A375 cells. As shown in , NAC treatment significantly decreased the expression of p-p38, LC3B-II, and Beclin 1. Together, these results suggested that Shikonin induces autophagy in human melanoma A375 cells through ROS-mediated activation of the p38 signal pathway.
Discussion
A number of natural products extracted from plants, and showing low toxicity, including taxol, quercetin, curcumin, and shikonin, have been shown to induce or suppress complicated signaling pathways to inhibit cancer cell growth in vitro [Citation13,Citation14]. Shikonin, a natural naphthoquinone pigment, suppresses the growth of various cancer cells including those of lung cancer [Citation15], pancreatic cancer [Citation16], and gastric cancer [Citation17]. However, the exact molecular mechanism of Shikonin-induced toxicity remains incompletely elucidated in human melanoma A375 cells. In the present study, we demonstrated that Shikonin inhibits proliferation in A375 cells via G2/M cell-cycle arrest, induction of apoptosis and autophagy through regulation of the ROS/ER stress apoptotic pathway and the ROS/p38 signal pathway, respectively.
Dysregulation of the cell cycle results in uncontrolled cell proliferation, genomic instability, and tumorigenesis [Citation18]. Cell-cycle progression is controlled by the cyclin/cyclin-dependent kinase (CDK) complex, CDK activating kinase, and CDK inhibitors [Citation19]. Previous studies have demonstrated that Shikonin inhibits cell proliferation through induction of G0/G1 phase arrest or G2/M phase arrest in different cancer cells [Citation20,Citation21]. Our results demonstrated that Shikonin induces G2/M phase arrest in A375 cells. The CDK inhibitor p21 inhibits the cyclin/CDK complex and promotes cell cycle arrest in response to various stimuli [Citation22]. Additionally, it has been reported that cyclin B1 expression is associated with G2/M cell cycle progression [Citation21,Citation23]. To explore the mechanisms by which Shikonin induced a G2/M phase arrest in A375 cells, proteins related to cell cycle regulation were assessed using western blot analysis. The results showed that Shikonin treatment decreased the expression of cyclin B1 and increased the expression of p21. These data indicate that Shikonin induced G2/M phase arrest by downregulating cyclin B1 and upregulating p21.
Defects in programmed cell death (apoptosis) mechanisms play important roles in tumour pathogenesis [Citation24]. Numerous substances with anticancer activity cause apoptosis through the mitochondrial apoptosis pathway initiated by the loss of mitochondrial membrane potential and release of pro-apoptotic proteins including Cyto-c, leading to the sequential activation of Caspase-3 and PARP, which eventually results in apoptosis [Citation25,Citation26]. Shikonin-induced apoptosis was associated with activation of caspase-3 and PARP cleavage in MCF-7 cells and HeLa cells [Citation27]. In the current study, based on the results of Hoechst 33268 staining, flow cytometry analysis, and measurement of caspase-3 activity, it was concluded that Shikonin induces apoptosis in A375 cells.
ROS influences the fate of cancer cells by regulating various signaling pathways including apoptosis and autophagy [Citation28,Citation29]. Shikonin-induced apoptosis in gastric cancer cells was reported to be mediated by ROS generation [Citation30]. Here, we found that Shikonin elevated ROS generation and that NAC pretreatment reversed apoptosis induced by Shikonin in A375 cells, suggesting that Shikonin-triggered apoptosis was regulated by ROS. ER stress is shaped to re-establish ER homeostasis through activation of the unfolded protein response (UPR). However, when ER stress is too severe or prolonged, the pro-survival function of the UPR turns into a toxic signal that is predominantly executed by mitochondrial apoptosis [Citation31]. It has been reported that Shikonin induces apoptosis in human prostate cancer cells through ER stress [Citation10,Citation32]. We found that Shikonin induced ER stress-mediated apoptosis by elevating the expression of p-eIF2α and CHOP in A375 cells. Numerous studies have shown that ROS is one of the important stimuli that trigger ER stress-mediated apoptosis. ROS-mediated ER stress is involved in Auranofin-induced apoptosis in gastric cancer [Citation33]. Our results demonstrated that ROS are involved in regulating ER stress-mediated apoptosis induced by Shikonin in A375 cells, as evidenced by the change in p-eIF2α and CHOP levels after pretreatment with NAC. Together, these results indicate that Shikonin induces apoptosis human melanoma A375 cells by activating the ROS-mediated ER stress pathway.
Autophagy, a downstream signal of ROS, plays an important role in regulating apoptosis induced by anti-cancer drugs [Citation34]. Growing evidences have shown that autophagy induction in tumour cells has a cytoprotective or cytotoxic function, depending on different cell types and stimuli [Citation35,Citation36]. In addition, Shikonin has been reported to activate autophagy in BXPC-3 human pancreatic cancer cells [Citation37]. In this study, we demonstrated that Shikonin activated autophagy by increasing the expression of LC3B-II and Beclin-1. To further explore the role of Shikonin-induced autophagy in apoptosis, A375 cells were pretreated with 3-MA and then exposed to Shikonin. We found that blockage of autophagy by 3-MA increased the Shikonin-triggered apoptosis in A375 cells, suggesting that Shikonin-induced autophagy might act as a protective mechanism to promote A375 cell survival. Accumulating studies have confirmed that multiple signaling pathways are involved in activating autophagy. Shikonin has been reported to activate autophagy mediated by ROS in human hepatocellular carcinoma [Citation8]. A study on HT1080 human fibrosarcoma cells showed that Silibinin induces autophagy through the activation of ROS-p38 [Citation12]. Kim et al. [Citation38] also confirmed that Salinomycin induced autophagy through regulation of the ROS-mediated p38-MAPK signaling pathway in human prostate cancer cells. Ahn et al. [Citation9] reported that Shikonin could activate the p38 MAPK pathway. Therefore, we investigated whether the ROS/p38 pathway was involved in Shikonin-induced autophagy. In this study, we found that Shikonin increased the p-p38 level and that Shikonin-induced cell growth inhibition was significantly enhanced by SB203580. In addition, NAC pretreatment attenuated the increased levels of p-p38, LC3B-II, and Beclin-1 induced by Shikonin. These data indicate that Shikonin induced autophagy in human melanoma A375 cells by activating the ROS-mediated p38 pathway.
Taken together, these results suggest that Shikonin effectively inhibits A375 cell growth by inducing G2/M phase arrest and induces apoptosis and protective autophagy via activation of ER stress and p38 pathways, which are modulated by ROS generation. These findings support the potential of Shikonin as a potential drug for the treatment of human melanoma and indicate that the reasonable inhibition of autophagy in A375 cells may be a promising strategy for Shikonin induced apoptosis to be used as adjuvant chemotherapy for human melanoma.
Disclosure statement
No potential conflict of interest was reported by the authors.
Additional information
Funding
References
- Eggermont AMM, Robert C. Melanoma: Smart therapeutic strategies in immuno-oncology. Nature. 2014;515(7527):S109.
- Linares MA, Zakaria A, Nizran P. Skin cancer. Primary Care. 2015;42:645–659.
- Homet B, Ribas A. New drug targets in metastatic melanoma. J Pathol. 2014;232:134–141.
- Johnson DB, Pollack MH, Sosman JA. Emerging targeted therapies for melanoma. Expert Opin Emerg Drugs. 2016;21:195–207.
- Lu L, Qin A, Huang H, et al. Shikonin extracted from medicinal Chinese herbs exerts anti-inflammatory effect via proteasome inhibition. Eur J Pharmacol. 2011;658:242–247.
- Li W, Zhang C, Ren A, et al. Shikonin suppresses skin carcinogenesis via inhibiting cell proliferation. PLoS One. 2015;10:e0126459.
- Chang IC, Huang YJ, Chiang TI, et al. Shikonin induces apoptosis through reactive oxygen species/extracellular signal-regulated kinase pathway in osteosarcoma cells. Biol Pharm Bull. 2010;33:816–824.
- Gong K, Zhang Z, Chen Y, et al. Extracellular signal-regulated kinase, receptor interacting protein, and reactive oxygen species regulate shikonin-induced autophagy in human hepatocellular carcinoma. Eur J Pharmacol. 2014;738:142.
- Ahn J, Won M, Choi JH, et al. Reactive oxygen species-mediated activation of the Akt/ASK1/p38 signaling cascade and p21(Cip1) downregulation are required for shikonin-induced apoptosis. apoptosis: Int J Programmed Cell Death. 2013;18:870–881.
- Gara RK, Srivastava VK, Duggal S, et al. Shikonin selectively induces apoptosis in human prostate cancer cells through the endoplasmic reticulum stress and mitochondrial apoptotic pathway. J Biomed Sci. 2015;22:26.
- He G, He G, Zhou R, et al. Enhancement of cisplatin-induced colon cancer cells apoptosis by shikonin, a natural inducer of ROS in vitro and in vivo. Biochem Biophys Res Commun. 2016;469:1075–1082.
- Duan WJ, Li QS, Xia MY, et al. Silibinin activated ROS-p38-NF-kappaB positive feedback and induced autophagic death in human fibrosarcoma HT1080 cells. J Asian Nat Prod Res. 2011;13:27–35.
- Ouyang L, Luo Y, Tian M, et al. Plant natural products: from traditional compounds to new emerging drugs in cancer therapy. Cell Prolif. 2014;47:506–515.
- van Ginkel PR, Yan MB, Bhattacharya S, et al. Natural products induce a G protein-mediated calcium pathway activating p53 in cancer cells. Toxicol Appl Pharmacol. 2015;288:453–462.
- Yeh YC, Liu TJ, Lai HC. Shikonin induces apoptosis, necrosis, and premature senescence of human A549 lung cancer cells through upregulation of p53 expression. Evid Based Complement Alternat Med: eCAM. 2015;2015:1.
- Wang Y, Zhou Y, Jia G, et al. Shikonin suppresses tumor growth and synergizes with gemcitabine in a pancreatic cancer xenograft model: involvement of NF-kappaB signaling pathway. Biochem Pharmacol. 2014;88:322–333.
- Liu JP, Liu D, Gu JF, et al. Shikonin inhibits the cell viability, adhesion, invasion and migration of the human gastric cancer cell line MGC-803 via the Toll-like receptor 2/nuclear factor-kappa B pathway. J Pharm Pharmacol. 2015;67:1143–1155.
- Chen CF, Dou XW, Liang YK, et al. Notch3 overexpression causes arrest of cell cycle progression by inducing Cdh1 expression in human breast cancer cells. Cell Cycle (Georgetown, Tex). 2016;15:432–440.
- Diaz-Moralli S, Tarrado-Castellarnau M, Miranda A, et al. Targeting cell cycle regulation in cancer therapy. Pharmacol Ther. 2013;138:255–271.
- Zhai T, Hei Z, Ma Q, et al. Shikonin induces apoptosis and G0/G1 phase arrest of gallbladder cancer cells via the JNK signaling pathway. Oncol Rep. 2017;38:3473–3480.
- Kim SJ, Kim JM, Shim SH, et al. Shikonin induces cell cycle arrest in human gastric cancer (AGS) by early growth response 1 (Egr1)-mediated p21 gene expression. J Ethnopharmacol. 2014;151:1064–1071.
- Abbas T, Dutta A. p21 in cancer: intricate networks and multiple activities. Nat Rev Cancer. 2009;9:400–414.
- Lohberger B, Leithner A, Stuendl N, et al. Diacerein retards cell growth of chondrosarcoma cells at the G2/M cell cycle checkpoint via cyclin B1/CDK1 and CDK2 downregulation. BMC Cancer. 2015;15:891.
- Hassan M, Watari H, AbuAlmaaty A, et al. Apoptosis and molecular targeting therapy in cancer. BioMed Res Int. 2014;2014:1.
- Jiang C, Yuan Y, Hu F, et al. Cadmium induces PC12 cells apoptosis via an extracellular signal-regulated kinase and c-Jun N-terminal kinase-mediated mitochondrial apoptotic pathway. Biol Trace Elem Res. 2014;158:249–258.
- Brentnall M, Rodriguez-Menocal L, De Guevara RL, et al. Caspase-9, caspase-3 and caspase-7 have distinct roles during intrinsic apoptosis. BMC Cell Biol. 2013;14:32.
- Jang SY, Hong D, Jeong SY, et al. Shikonin causes apoptosis by up-regulating p73 and down-regulating ICBP90 in human cancer cells. Biochem Biophys Res Commun. 2015;465:71–76.
- Su Z, Yang Z, Xu Y, et al. Apoptosis, autophagy, necroptosis, and cancer metastasis. Mol Cancer. 2015;14:48.
- Dixon SJ, Stockwell BR. The role of iron and reactive oxygen species in cell death. Nat Chem Biol. 2014;10:9–17.
- Lee MJ, Kao SH, Hunag JE, et al. Shikonin time-dependently induced necrosis or apoptosis in gastric cancer cells via generation of reactive oxygen species. Chem Biol Interact. 2014;211:44–53.
- Fernandez A, Ordonez R, Reiter RJ, et al. Melatonin and endoplasmic reticulum stress: relation to autophagy and apoptosis. J Pineal Res. 2015;59:292–307.
- Han X, Kang KA, Piao MJ, et al. Shikonin exerts cytotoxic effects in human colon cancers by inducing apoptotic cell death via the endoplasmic reticulum and mitochondria-mediated pathways. Biomol Therapeut. 2019;27:41–47.
- Zou P, Chen M, Ji J, et al. Auranofin induces apoptosis by ROS-mediated ER stress and mitochondrial dysfunction and displayed synergistic lethality with piperlongumine in gastric cancer. Oncotarget. 2015;6:36505–36521.
- Kaminskyy VO, Zhivotovsky B. Free radicals in cross talk between autophagy and apoptosis. Antioxid Redox Signal. 2014;21:86–102.
- Sharma K, Le N, Alotaibi M, et al. Cytotoxic autophagy in cancer therapy. IJMS. 2014;15:10034–10051.
- Gordy C, He YW. The crosstalk between autophagy and apoptosis: where does this lead? Protein Cell. 2012;3:17–27.
- Shi S, Cao H. Shikonin promotes autophagy in BXPC-3 human pancreatic cancer cells through the PI3K/Akt signaling pathway. Oncol Lett. 2014;8:1087–1089.
- Kim KY, Park KI, Kim SH, et al. Inhibition of autophagy promotes salinomycin-induced apoptosis via reactive oxygen species-mediated PI3K/AKT/mTOR and ERK/p38 MAPK-dependent signaling in human prostate cancer cells. Int J Mol Sci. 2017;18:1088.