Abstract
Long noncoding RNA lung cancer associated transcript 1 (LUCAT1) has been shown to be a lncRNA that facilitates the development and progression of several tumours. However, the evidence of LUCAT1 modulating the growth and metastasis of cervical cancer (CC) were still lacking. The present study aimed to explore the expression pattern, biological function and potential mechanism of LUCAT1 in CC. In this study, we, first, confirmed that LUCAT1 acted as an up-regulated lncRNA by analyzing the data from GCTA dataset and RT-PCR in both CC tissues and cell lines. We also showed that TINCR overexpression is induced by nuclear transcription factor SP1. Then, clinical assays showed that LUCAT1 was associated with advanced clinical progression and poor prognosis of CC patients. Importantly, multivariate Cox model confirmed that LUCAT1 expression was an independent prognostic factor for both 5-year overall survival in CC. Then, lost-function assays revealed that knockdown of LUCAT1 significantly suppressed CC cells proliferation, colony formation, migration, invasion and EMT by a series of cells experiments. Mechanistically, Bioinformatic tools predicted that miR-181a may target LUCAT1, which was confirmed using luciferase reporter assay and RNA immunoprecipitation (RIP) assays. Overall, our findings showed that SP1-activated LUCAT1 exerts an oncogenic function in CC by binding to miR-181a, suggesting that miR-181a may be a ponderable and promising therapeutic target for CC.
Introduction
Cervical cancer (CC) is the second leading cause of cancer mortality in women worldwide with more than 0.5 million new cases diagnosed annually [Citation1,Citation2]. The mechanisms of CC include multi-step processes. Growing evidence have revealed that high-risk human papillomavirus (HPV) infection is a necessary factor for CC tumorigenesis [Citation3]. However, HPV does not develop CC. With the development in screening CC, more and more new cases were diagnosed [Citation4]. Unfortunately, there are still a growing number of advanced diseases, especially in developing countries, such as China [Citation5]. Although increasing diagnostic techniques and therapeutic strategies for CC have improved, the CC mortality rate remains high [Citation6]. Tumour invasion and metastasis remain formidable obstacles for favourable treatment of CC. Therefore, it is essential to reveal the molecular mechanisms of the development of CC.
Previous researches have shown that a substantial portion of the human genome can be transcribed into ncRNAs which were previously regarded as “noise” or “junk” RNAs lacking protein-coding potential [Citation7]. Long noncoding RNA (lncRNAs) are a class of non-coding RNA molecules longer than 200 nt [Citation8]. Recent advancements have revealed that lncRNAs act as important regulators in cellular development, differentiation, migration, apoptosis and several other biological processes [Citation9,Citation10]. Increasing number of lncRNAs has been identified to be involved in the tumorigenesis by acting as tumour suppressor or oncogenes according to the cells conditions [Citation11–13]. In addition, more and more lncRNAs have been identified to be dysregulated [Citation14,Citation15]. However, only very few of them have been well-studied and have clear biological functions and molecule mechanisms so far.
LncRNA lung cancer associated transcript 1 (LUCAT1), also named SCAL1 and located on chromosome 5, has been previously identified to be a tumour-related lncRNA and is induced by cigarette smoke and elevated in lung cancer cell lines [Citation16,Citation17]. Recently, several studies frequently reported that LUCAT1 was abnormally expressed in some different tumours by analyzing online microarray data and performing RT-PCR in tumour tissues. Generally, LUCAT1 may act as a tumour promoter which was demonstrated by in vitro and in vivo experiments [Citation18–20]. However, to our best knowledge, whether LUCAT1 expression was dysregulated in CC and its potential biological, as well as molecule mechanism, remained unclear. In this study, using TCGA datasets, we preliminarily confirmed LUCAT1 as an up-regulated lncRNA in CC, which was further confirmed by following RT-PCR in CC tissues and cell lines. Subsequently, we explored the potential mechanism by which LUCAT1 expression was modulated and the biological function via lost-function assays. Our present study provided a better understanding of the development and progression of CC.
Materials and methods
Patients and tissue samples
Fresh CC and matched adjacent normal tissue specimens were collected from 125 patients between May 2010 and August 2013 in the Cangzhou Central Hospital. None of the patients had received chemotherapy or radiotherapy before surgery. All specimens were immediately frozen in liquid nitrogen and stored at −70 °C until use. The stage of tumours was defined according to the 2009 FIGO criteria. Follow-up information was collected every 3 months via telephone or by mail. The clinical information was shown in . The study was approved by the Research Ethics Committee of Cangzhou Central Hospital. Informed consent was obtained from all patients.
Cell lines and cell transfection
The expression levels of LUCAT1 were examined in six human CC cell lines (HeLa, C33A, SiHa, Caski, SW756 and ME-180) and one non-carcinoma cervical epithelial cell line (Ect1/E6E7). The cells which were cultured using RPMI-1640 complete medium (10% FBS, 1% streptomycin-penicillin antibiotic solution) were all obtained from FENGHUI Biotechnology Co., Ltd. (Changsha, Hunan, China).
We used NanoEnter transfection reagent (NCM Biotech, Suzhou, Jiangsu, Chian) to transfected plasmids, siRNAs or miRNA mimics into CC cells. The small interfering RNAs (siRNAs) against LUCAT1 (LUCAT1-siRNA#1 and LUCAT1-siRNA#2), negative control siRNA for LUCAT1 (NC siRNA), SP1 (si-SP1#1 and si-SP1#2), negative control siRNA for SP1 (si-NC), miR-181a mimic and negative control mimic (Control mimic) were all obtained from Promoterbio Co., Ltd. (Taizhou, Jiangsu, China). The LUCAT1 overexpressing plasmid, pcDNA3.1-LUCAT1, was constructed by Vigene Biosciences Co., Ltd. (Jinan, Shandong, China).
Quantitative real-time PCR (qRT-PCR)
An Eastep Super Total RNA purification kit (Lab Biotech, Nankai, Tianjin, China) was applied to extract the RNA of CC cells. Then, we applied Plexor One-Step qRT-PCR System (Jingke Biotech, Changsha, Hunan, China) to detect the expression levels of SP1 or LUCAT1 using a Quant Studio 6 qRT-PCR system (Thermo Fisher Scientific, Pudong, Shanghai, China). The primers were all summarized in . Thereafter, a miRNA fluorescent quantitative PCR detection kit (BaiAoLaiBo, Haidian, Beijing) was employed to examine miR-181a in HeLa and SiHa cells. The expression levels of SP1 or LUCAT1 were normalized to GAPDH, and the expression levels of miR-181a were normalized to U6. The results of qRT-PCR were expressed relative to threshold cycle (CT) values and subsequently converted to fold changes.
Table 1. The Primer sequences included in this study.
Cell counting kit-8 (CCK-8) and colony formation assays
After the HeLa and SiHa cells were transfected with LUCAT1 or SP1 siRNAs, miRNA mimics or corresponding plasmids, the cells at a density of 2000 cells per well were plated into 96-well plates and continued to be cultured for 24 h. Subsequently, 10 μl CCK-8 solution (Enogene, Nanjing, Jiangsu, China) were added into each well, followed by incubating at 37 °C for an additional 1 h. Then, a CLARIOstar microreader system (BMG Labtech, Offenburg, Germany) was applied to evaluate the absorbance (450 nm) values.
For cell colony formation assays, about 500 treated HeLa or SiHa cells were seeded into the 6-well plates, culturing at 37 °C for two weeks. Then, 0.1% crystal violet dye (J & L Biotech, Wuhan, Hubei, China) was employed to stain the cell colonies. Finally, an XSP-22C microscope (Huxing, Pudong, Shanghai, China) was utilized to take photographs.
Western blot assays
A total protein extraction kit (GeneLife, Mentougou, Beijing, China) was applied to lyse the HeLa and SiHa cells. Then, 20 μg of protein was loaded onto the SDS-PAGE and sequentially transferred to a polyvinyl fluoride membrane. After being blocked with 5% non-fat milk, the membranes were probed with primary and secondary antibodies. Finally, an ECL Western Blotting Substrate kit (ZeYe Biotech, Jinshan, Shanghai, China) and a ChemiScope 6000 system (CLiNX, Baoshan, Shanghai, China) were utilized to detect the protein bands. The primary antibodies against caspase-3, vimentin and caspase-9 were purchased from Sino Biological Inc. (Yizhuang, Beijing, China). The primary antibodies targeting N-Cadherin and GAPDH were obtained from Boster Biotechnology Co., Ltd. (Wuhan, Hubei, China).
Chromatin immunoprecipitation (ChIP) assays
A ChIP assay kit (BersinBio, Guangzhou, Guangdong, China) was employed to conduct ChIP assays. Briefly, the treated HeLa and SiHa cells were first fixed using 1% formaldehyde and sequentially incubated with glycine (125 nM) for 5 min. Afterwards, DNA fragments ranging from 200 to 300 bp were obtained by sonicating the chromatin. Then, anti-SP1 antibody (KangLang, Minhang, Shanghai, China) or anti-IgG antibody (Huijia Biotech, Xiamen, Fujian, China) were applied to immunoprecipitate the chromatin. Finally, qPCR was utilized to analyze the precipitated DNA.
RNA immunoprecipitation (RIP) assays
For RIP experiments, we used a Research Science RIP assay kit (R&S Biotechnology, Pudong, Shanghai, China). In short, the HeLa and SiHa cells were first transfected with pcDNA3.1-LUCAT1 or miR-181a mimics. After 48 h, the cells were added with RIP lysis buffer. Subsequently, anti-Argonaute-2 (Ago2) antibodies (Abcam, Pudong, Shanghai, China), which were conjunct with magnetic beads, were added into the cell lysates. Finally, the co-precipitated RNAs were examined by qRT-PCR assays.
Apoptosis assays
HeLa and SiHa cells transfected with LUCAT1 siRNAs were collected using trypsin. Afterwards, an Annexin V/PI apoptosis assay kit (Berke Biotech, Nanjing, Jiangsu, China) was used to conduct the cell apoptosis analysis and sequentially analyzed using a MoFlo XDP flow cytometer (Beckman-Coulter Inc., Fullerton, CA, USA).
Dual-luciferase reporter assays
The full promoter sequence of LUCAT1 (PF), E1 site and E3 site sequences of LUCAT1 (P1), E2 site sequence of LUCAT1 (P2) were constructed into pGL3 reporter plasmids by Transduction Bio Co., Ltd. (Wuhan, Hubei, China). The wild type LUCAT1 (wt LUCAT1) and mutant LUCAT1 (mut LUCAT1) were constructed by Syngentech Co., Ltd. (Changping, Beijing, China). After transfecting with corresponding plasmids, the luciferase activities in HeLa and SiHa cells were examined using a Dual-Lucy Assay kit (VigorousBio, Haidian, Beijing, China).
Wound healing assays
The wound healing assays were carried out using an Ibidi 3.5 cm µ-dish (Koster Scientific, Guangzhou, Guangdong, China) with an insert containing two reservoirs. Briefly, HeLa and SiHa cells were first transfected with LUCAT1 siRNAs and then added into the inserts (70 μl, 5 × 105 cells/ml). Finally, an XSP-22C microscope (Huxing, Pudong, Shanghai, China) was applied to observe the migration of HeLa and SiHa cells when the inserts were removed using sterilized tweezers.
Transwell invasion assays
In brief, the HeLa and SiHa cells transfected with LUCAT1 siRNAs were first resuspended in RPMI-1640 medium without FBS. Then, the HeLa and SiHa cells were placed into the upper chamber of the transwell inserts which was pre-treated with Matrigel (70 μl). In addition, complete medium (400 μl, 15% FBS) was added into the lower chambers, and the cells were maintained for 24 h. After staining with 0.1% crystal violet dye (RenoldBio, Suzhou, Jiangsu, China), an XSP-22C microscope (Huxing, Pudong, Shanghai, China) was applied to take images.
Statistical analysis
Data in the present study were analyzed using two-tailed Student T-test (differences between groups), or one-way ANOVA (differences between more than two groups). The associations between LUCAT1 expression and clinical-pathological parameters were evaluated using chi-square tests. Patient survival and their differences were determined using the Kaplan–Meier method and log–rank test. The influence of each variable on survival was examined by the Cox multivariate regression analysis. A p-value <.05 was considered significant.
Results
LUCAT1 was highly expressed in CC tissues and cell lines
First, the expression level of abnormally expressed lncRNAs in human CC samples was evaluated using TCGA RNA-seq data. The differently expressed lncRNA was screened, and 135 mostly significantly dysregulated lncRNA was shown in the heatmap and volcano plot (). Furthermore, the expression of LUCAT1 was significantly up-regulated in CC tissues compared to normal cervical tissues in TCGA datasets (p < .01). Then, we further performed RT-PCR to measure the expression pattern of LUCAT1 in our own cohort, including 125 pairs of HCC tissues and adjacent noncancerous cervical tissues, finding that LUCAT1 was highly expressed in CC tissues compared to normal tissues (p < .01). In addition, the expression of LUCAT1 was also detected in CC cell lines and E6E7, and the results showed that the expression of LUCAT1 was high in CC cells compared with that of normal cervical epithelial cells. Overall, our data indicated that up-regulation may play a critical role in CC progression.
Figure 1. Relative LUCAT1 expression in CC tissues and its clinical significance. (a) Unsupervised clustering of patients based on the expression pattern of differentially expressed lncRNAs using TCGA datasets. (b) Volcano Plot visualizing the differentially expressed lncRNAs using TCGA datasets. The vertical lines demark the fold-change value. (c) TCGA cohort analysis of the expression levels of LUCAT1 in CC samples as well as in the normal bone tissues. (d) LUCAT1 expression was examined by qRT-PCR in 125 paired CC tissues and adjacent non-tumour tissues. (e) qRT-PCR analysis determined the expression of LUCAT1 in six CC cell lines and E6E7. (f) Kaplan–Meier curves for survival time in patients with CC divided according to LUCAT1 expression. *p < .05, **p < .01.
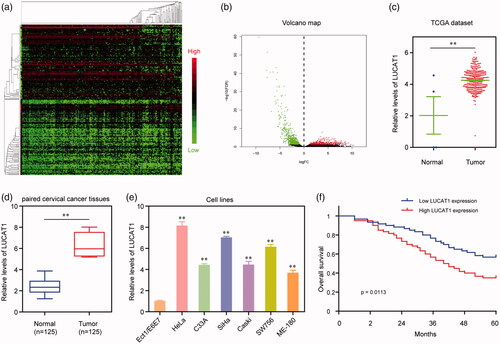
Prognostic value of LUCAT1 in CC
In order to explore the clinical significance of LUCAT1 in CC, the 125 patients with CC were divided into two groups according to the median value of LUCAT1expression level, including high-expression group (n = 65) and low-expression group (n = 60), respectively. As shown in , we found that high LUCAT1 was associated with histologic grade (p = .003), FIGO stage (p = .022), lymph node metastasis (p = .021) and depth of cervical invasion (p = .017). However, there was no significance between LUCAT1 expression and other clinicopathological factors. Then, we further explore the influence of LUCAT1 on prognosis of CC patients. Kaplan–Meier analysis manifested that patients with high-LUCAT1 expression had a shorter overall survival time than those with low-LUCAT1 expression (p = .0113). Moreover, univariate analysis identified four prognostic factors: Histologic grade, FIGO stage, Lymph node metastasis, depth of cervical invasion and LUCAT1 expression (). More importantly, multivariate Cox regression analysis confirmed that LUCAT1 expression (HR = 3.014, 95% CI: 1.328–4.465; p = .008) was an independent prognostic marker for overall survival of CC patients ().
Table 2. Clinicopathological features associated with LUCAT1 expression in 125 CC patients.
Table 3. Univariate and multivariate analysis of clinicopathological factors for overall survival in 125 patients with CC.
SP1 stimulated LUCAT1 overexpression in human CC cells
We next aimed to discover the upstream molecular mechanisms which caused the high expression of LUCAT1 in CC cells. Hence, JASPAR (http://jaspar.genereg.net/) was then applied to predict the potential transcription factors (TFs) which could bind to the promoter regions of LUCAT1. The results indicated that there were several SP1 binding sites in the promoter of LUCAT1, and we next focused on three SP1 binding sites (E1: −1121 to −1111, tccctgcccat; E2: −787 to −777, gctccacctcc; E3: −455 to −445, acccctccttg) with the highest scores (). Furthermore, the results of qRT-PCR assays revealed that transfection of LUCAT1 siRNAs (si-SP1#1 and si-SP1#2) significantly reduced the expression of SP1, whereas transfecting pcDNA3.1-SP1 plasmid remarkably increased the expression levels of SP1 in HeLa and SiHa cells (). In addition, overexpression of SP1 dramatically enhanced the expression of LUCAT1 in HeLa and SiHa cells, whereas knockdown of SP1 led to a notably decreased expression of LUCAT1 (). Moreover, ChIP assays confirmed that there was a marked SP1-binding activity in the E2 site of LUCAT1 promoter (). Besides, we next carried out dual-luciferase reporter assays to validate that E2 site was the exact binding region of SP1. First, we constructed three luciferase report plasmids which contained full promoter (PF), E1 as well as E3 regions (P1) or E2 region alone (P2), respectively (). Afterwards, these plasmids were respectively transfected into HeLa or SiHa cells. The data suggested that co-transfection of PF or P2 with pcDNA3.1-SP1 plasmids resulted significantly increased luciferase activities of HeLa and SiHa cells, whereas co-transfection of P1 with pcDNA3.1-SP1 plasmids had no effects on the luciferase activities of HeLa and SiHa cells (). Taken together, these data demonstrated that LUCAT1 overexpression in CC was mediated by SP1.
Figure 2. The transcription factor SP1 was involved in LUCAT1 upregulation. (a) The predicted sites of putative SP1 binding motif in human LUCAT1 promoter. (b) The relative mRNA levels of SP1 in HeLa and SiHa cells after transfection of SP1 siRNAs. (c) Transfection of SP1 overexpressing plasmids, pcDNA3.1-SP1, increased the mRNA levels of SP1. (d) The relative expression levels of LUCAT1 in HeLa and SiHa cells after transfection of SP1 siRNAs or pcDNA3.1-SP1. (e) ChIP assays analyzed the SP1 binding sites. (f) The schematic diagram of constructed luciferase reporter plasmids. (g) The relative luciferase activities examined by Dual luciferase reporter assays. *p < .05, **p < .01.
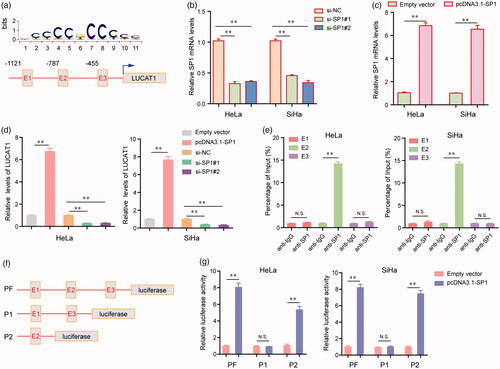
Depression of LUCAT1 suppressed the proliferation of CC cells and induced cell apoptosis
Given LUCAT1 was highly expressed in CC, we next asked whether LUCAT1 played important roles in regulating the proliferation and apoptosis of HeLa and SiHa cells. The results of qRT-PCR assays demonstrated that the expression levels of LUCAT1 in HeLa and SiHa cells were significantly inhibited by LUCAT1 siRNAs (). The growth curves assessed by CCK-8 assays indicated that LUCAT1 knockdown remarkably impaired HeLa and SiHa cells growth (). In addition, the silence of LUCAT1 notably suppressed the colony formation abilities of HeLa and SiHa cells, which was consistent with the CCK-8 assay results (). Besides, we next conducted flow cytometry to examine the apoptosis of HeLa and SiHa cells transfected with LUCAT1 siRNAs. As the data presented in , repressing the expression of LUCAT1 obviously accelerated the cell apoptosis. Furthermore, the results of western blot analysis revealed that transfection of LUCAT1 siRNAs significantly promoted the protein levels of caspase-3 and caspase-9 in HeLa and SiHa cells (). Collectively, these data indicated that LUCAT1 played essential roles in the development of CC.
Figure 3. LUCAT1 affected the proliferation and apoptosis of HeLa and SiHa cells. (a) The relative expression levels of LUCAT1 in HeLa and SiHa cells after transfection of LUCAT1 siRNAs (LUCAT1-siRNA#1 and LUCAT1-siRNA#2) or negative control (NC) siRNAs. (b) The growth curves of HeLa and SiHa cells after transfection of LUCAT1 siRNAs or NC siRNAs. (c) Transfection of LUCAT1 siRNAs reduced the colony formation abilities of HeLa and SiHa cells. (d) Silence of LUCAT1 accelerated the cell apoptosis. (e) Western blot assays detected the protein levels of caspase-3 and caspase-9. *p < .05, **p < .01.
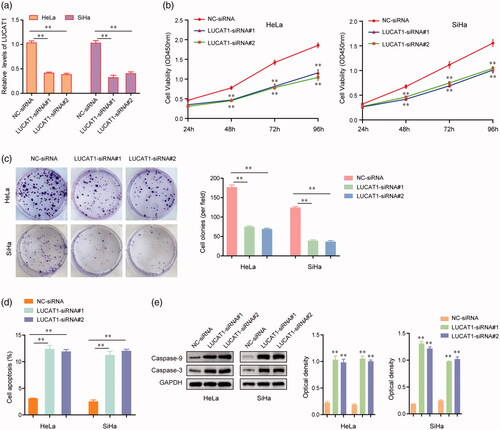
Knockdown of LUCAT1 impaired the migration and invasion of CC cells
We next assessed the function of LUCAT1 on the migration and invasion of CC cells. The wound healing assays demonstrated that the migratory abilities of HeLa and SiHa cells were markedly reduced after they were transfected with LUCAT1 siRNAs (). Similarly, after the transfection of LUCAT1 siRNAs, a remarkably decreased number of HeLa and SiHa cells were observed to invade through the Martrigel-treated membranes compared with controls (). Considering the migratory and invasive capacities of CC cells were affected by LUCAT1, we next examined the alteration of epithelial-mesenchymal transition related molecules, such as N-cadherin and vimentin, in HeLa and SiHa cells using western blot assays. The data confirmed that transfection of LUCAT1 siRNAs dramatically reduced the protein levels of both N-cadherin and vimentin (). Overall, these results provided evidence that LUCAT1 affected the metastasis potentials of CC cells.
Figure 4. LUCAT1 affected the invasion and migration of HeLa and SiHa cells. (a,b) Transfection of LUCAT1 siRNAs reduced the migratory abilities of HeLa and SiHa cells. (c) Knockdown of LUCAT1 reduced the invasion of HeLa and SiHa cells. (d,e) Silence of LUCAT1 siRNAs significantly decreased the protein levels of N-cadherin and vimentin. *p < .05, **p < .01.
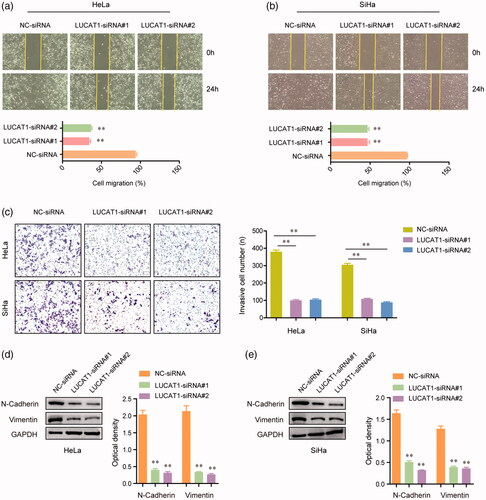
LUCAT1 directly interacted with miR-181a in CC cells
Accumulating evidence had indicated that lncRNAs could exert their functions by “sponge” specific miRNAs. Therefore, the “starbase” (http://starbase.sysu.edu.cn/) was used to predict the potential miRNAs which could act as the target of LUCAT1. The data suggested that miR-181a was possibly directly interacted with LUCAT1 (). To further validate this, we next conducted dual-luciferase reporter assays. The results demonstrated that co-transfection with wild type LUCAT1 (wt LUCAT1) plasmids and miR-181a mimics remarkably reduced the luciferase activities of HeLa and SiHa cells, whereas the luciferase activities were not changed when the cells were co-transfected with mutant LUCAT1 (mut LUCAT1) plasmids as well as miR-181a mimics (). In addition, LUCAT1 and miR-181a were markedly enriched in Ago2-containing beads when compared with the input group, which was evaluated by RIP assays (). Moreover, the relative expression of miR-181a was significantly decreased in HeLa and SiHa cells when they were transfected with pcDNA3.1-LUCAT1 plasmids, whereas silence of LUCAT1 resulted in notably increased expression of miR-181a (). Therefore, these data suggested that miR-181a acted as a target of LUCAT1, and LUCAT1 exerted its functions in the development and progression of CC via modulating miR-181a.
Figure 5. miR-181a was a direct target of LUCAT1. (a) The binding site of miR-181a was predicted by “starbase”. (b) The luciferase activities of HeLa and SiHa cells. (c) RIP assays examined the enrichment of LUCAT1 and miR-181a Ago2-containing beads. (d) The relative expression of miR-181a in HeLa and SiHa cells. *p < .05, **p < .01.
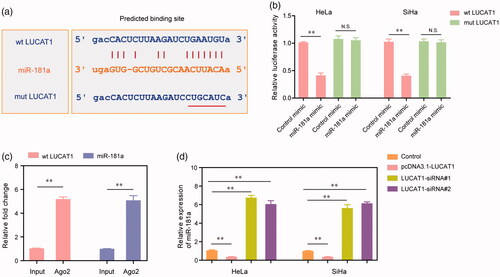
Discussion
Although the great efforts have been made in the research of CC, CC remains to be a serious burden not only in the worldwide but also in China [Citation21]. The overall survivals of CC diagnosed at an early stage have developed in recent years. However, the prognosis of metastasis CC remains very poor [Citation22]. The identification of diagnostic and prognostic biomarkers which can be effectively used to guide treatment decision is very important in the individuation management of CC patients [Citation23,Citation24]. Recently, the potential of lncRNAs used as novel biomarker attracted increasing attention because of its critical regulation roles and the development of high throughput sequencing [Citation25]. In this study, our attention focused on a novel lncRNA, LUCAT1 which was found to be up-regulated in CC tissues from TCGA datasets. In addition, the results of RT-PCR in 125 CC patients from our hospital confirmed LUCAT1 was highly expressed in CC. Then, we explored the association between LUCAT1 expression and clinicopathological variables, finding that its high expression was observably associated with higher histologic grade, advanced FIGO stage, positive lymph node metastasis and depth of cervical invasion. Further clinical assays indicated that high LUCAT1 expression was significantly associated with poor overall survival of CC patients. More importantly, multivariate Cox regression analysis confirmed that LUCAT1 was an independent prognostic marker for overall survival of CC patients. Overall, our data provided important evidence that LUCAT1 was highly expressed in CC and may be used as a potential prognostic biomarker for CC patients.
Up to date, although more and more lncRNAs were confirmed to be abnormally expressed in various tumours, including CC, the critical factors involved in their altered expression pattern in tumours are still not well understood. Recently, growing data indicated that the levels of lncRNAs can be modulated by transcription factors and epigenetic regulators [Citation26,Citation27]. For instance, SP1-induced overexpression of lncRNA SNHG14 could contribute to the progression of clear cell renal cell carcinoma [Citation28]. SP1 activates lncRNA UCA1 transcription to promote gastric cancer cells proliferation via recruiting EZH2 [Citation29]. In this study, via analyzing the JASPAR CORE database, we focused on SP1 transcription factor because there are several SP1 binding sites in the promoter region of LUCAT1 and the roles of SP1 have been studied in CC. Then, we down-regulated the expression of SP1 and performed luciferase reporter assays and ChIP, confirming that SP1 could physically interact with the promoter of LUCAT1. In addition, we found that overexpression of SP1 promoted LUCAT1 expression, whereas down-regulation of SP1 suppressed LUCAT1 expression. Thus, our findings indicated that the expression of LUCAT1 may be modulated by SP1.
Recently, dysregulation of LUCAT1 and its potential function have been reported in several tumours. For instance, Yoon et al. [Citation30] reported that LUCAT1 was highly expressed in oesophagal squamous cell carcinoma and its knockdown suppressed metastasis of oesophagal squamous cell carcinoma by through DNA methylation. Gao et al. found that LUCAT1 exerted oncogenic activity in glioma because its knockdown significantly inhibited the proliferation and invasion of glioma cells by sponging miR-375 [Citation31]. Sun et al. showed that LUCAT1 was up-regulated in lung cancer and associated with advanced clinical stages and poor prognosis; moreover, they revealed that LUCAT1 was correlated with enhancer of EZH2 by repressing the expression of p21 and p57 [Citation32]. Besides, Liu et al. had demonstrated that increased EZH2 expression was relevance with the proliferation and progression of CC [Citation33]. Therefore, based on these findings, we wondered whether LUCAT1 also exerted oncogenic roles in CC progression. In order to demonstrate our hypothesis, we performed lost-function assays, finding that knockdown of LUCAT1 significantly suppressed CC cells proliferation, migration, invasion and EMT. It has been confirmed that lncRNAs can modulate multiple physiopathologic mechanisms in various tumours via ceRNA and miRNA “sponge”. In order to explore the potential mechanism by which LUCAT1 contributed to the progression of CC, the online software was used for the prediction of miRNA target sites. The results showed that miR-181a was predicted as a target of LUCAT1, and luciferase assays and RIP assays confirmed that LUCAT1 was a direct target of miR-181a. Previously, miR-181a has been reported to be lowly expressed in various tumours, including CC, and its tumour-suppressive roles were also confirmed by multiple experiments in CC [Citation34–37]. Our data showed that overexpression of LUCAT1 could suppress the expression of miR-181a, indicating that LUCAT1 may display its oncogenic roles by modulating miR-181a.
In conclusion, our findings indicate that LUCAT1 functions as an oncogene in CC and its high levels are associated with CC progression and unfavourable prognosis of CC patients. First, we reported that SP1 could bind directly to the LUCAT1 promoter region and activate its transcription. Moreover, knockdown of LUCAT1 inhibited CC cells proliferation, migration and invasion although directly binding to miR-181a. Based on our findings, LUCAT1 has now emerged as a novel prognostic biomarker and a potential new target for CC treatment.
Acknowledgements
We thank all donors for their donations. We thank Prof Liang Yuan and Prof Tiantian Xue from Department of molecular biology teaching and research, Peking University for their technical support. We thank the Union Hospital, Fujian Medical University, Fuzhou, for their generous help.
Disclosure statement
No potential conflict of interest was reported by the authors.
References
- Torre LA, Bray F, Siegel RL, et al. Global cancer statistics, 2012. CA Cancer J Clin. 2015;65:87–108.
- Miller KD, Siegel RL, Lin CC, et al. Cancer treatment and survivorship statistics, 2016. CA Cancer J Clin. 2016;66:271–289.
- Goodman A. HPV testing as a screen for cervical cancer. BMJ. 2015;350:h2372.
- Peirson L, Fitzpatrick-Lewis D, Ciliska D, et al. Screening for cervical cancer: a systematic review and meta-analysis. Syst Rev. 2013;2:35.
- Zhang X, Zhang L, Tian C, et al. Genetic variants and risk of cervical cancer: epidemiological evidence, meta-analysis and research review. BJOG: Int J Obstet Gy. 2014;121:664–674.
- du Toit GC, Kidd M. Prospective quality of life study of South African women undergoing treatment for advanced-stage cervical cancer. Clin Ther. 2015;37:2324–2331.
- Pircher A, Gebetsberger J, Polacek N. Ribosome-associated ncRNAs: an emerging class of translation regulators. RNA Biol. 2014;11:1335–1339.
- Yoon JH, Kim J, Gorospe M. Long noncoding RNA turnover. Biochimie. 2015;117:15–21.
- Yang L, Froberg JE, Lee JT. Long noncoding RNAs: fresh perspectives into the RNA world. Trends Biochem Sci. 2014;39:35–43.
- Booton R, Lindsay MA. Emerging role of MicroRNAs and long noncoding RNAs in respiratory disease. Chest. 2014;146:193–204.
- Martens-Uzunova ES, Bottcher R, Croce CM, et al. Long noncoding RNA in prostate, bladder, and kidney cancer. Eur Urol. 2014;65:1140–1151.
- Luo D, Deng B, Weng M, et al. A prognostic 4-lncRNA expression signature for lung squamous cell carcinoma. Artif Cells Nanomed Biotechnol. 2018;46:1207–1214.
- Liu C, Tian X, Zhang J, et al. Long non-coding RNA DLEU1 promotes proliferation and invasion by interacting with miR-381 and enhancing HOXA13 expression in cervical cancer. Front Genet. 2018;9:629.
- Kim HJ, Kim LK, Lee SH, et al. Expression levels of the long noncoding RNA steroid receptor activator promote cell proliferation and invasion and predict patient prognosis in human cervical cancer. Oncol Lett. 2018;16:5410–5418.
- Sharma S, Munger K. Expression of the cervical carcinoma expressed PCNA regulatory (CCEPR) long noncoding RNA is driven by the human papillomavirus E6 protein and modulates cell proliferation independent of PCNA. Virology. 2018;518:8–13.
- Xiao H, Bao L, Xiao W, et al. Long non-coding RNA Lucat1 is a poor prognostic factor and demonstrates malignant biological behavior in clear cell renal cell carcinoma. Oncotarget. 2017;8:113622–113634.
- Thai P, Statt S, Chen CH, et al. Characterization of a novel long noncoding RNA, SCAL1, induced by cigarette smoke and elevated in lung cancer cell lines. Am J Respir Cell Mol Biol. 2013;49:204–211.
- Han Z, Shi L. Long non-coding RNA LUCAT1 modulates methotrexate resistance in osteosarcoma via miR-200c/ABCB1 axis. Biochem Biophys Res Commun. 2018;495:947–953.
- Haque SU, Niu L, Kuhnell D, et al. Differential expression and prognostic value of long non-coding RNA in HPV-negative head and neck squamous cell carcinoma. Head Neck. 2018;40:1555–1564.
- Wang LN, Zhu XQ, Song XS, et al. Long noncoding RNA lung cancer associated transcript 1 promotes proliferation and invasion of clear cell renal cell carcinoma cells by negatively regulating miR-495-3p. J Cell Biochem. 2018;119:7599–7609.
- Bikhof Torbati M, Ebrahimian M, Yousefi M, et al. GO-PEG as a drug nanocarrier and its antiproliferative effect on human cervical cancer cell line. Artif Cells Nanomed Biotechnol. 2017;45:568–573.
- Dasari S, Wudayagiri R, Valluru L. Cervical cancer: biomarkers for diagnosis and treatment. Clin Chim Acta. 2015;445:7–11.
- Somashekhar SP, Ashwin KR. Management of early stage cervical cancer. Rev Recent Clin Trials. 2015;10:302–308.
- Cong J, Liu R, Wang X, et al. MiR-634 decreases cell proliferation and induces apoptosis by targeting mTOR signaling pathway in cervical cancer cells. Artif Cells Nanomed Biotechnol. 2016;44:1694–1701.
- Cheng WS, Tao H, Hu EP, et al. Both genes and lncRNAs can be used as biomarkers of prostate cancer by using high throughput sequencing data. Eur Rev Med Pharmacol Sci. 2014;18:3504–3510.
- Jiang Q, Wang J, Wang Y, et al. TF2LncRNA: identifying common transcription factors for a list of lncRNA genes from ChIP-Seq data. Biomed Res Int. 2014;2014:1.
- Jiang H, Huang G, Zhao N, et al. Long non-coding RNA TPT1-AS1 promotes cell growth and metastasis in cervical cancer via acting AS a sponge for miR-324-5p. J Exp Clin Cancer Res. 2018;37:169.
- Liu G, Ye Z, Zhao X, et al. SP1-induced up-regulation of lncRNA SNHG14 as a ceRNA promotes migration and invasion of clear cell renal cell carcinoma by regulating N-WASP. Am J Cancer Res. 2017;7:2515–2525.
- Wang ZQ, Cai Q, Hu L, et al. Long noncoding RNA UCA1 induced by SP1 promotes cell proliferation via recruiting EZH2 and activating AKT pathway in gastric cancer. Cell Death Dis. 2017;8:e2839.
- Yoon JH, You BH, Park CH, et al. The long noncoding RNA LUCAT1 promotes tumorigenesis by controlling ubiquitination and stability of DNA methyltransferase 1 in esophageal squamous cell carcinoma. Cancer Lett. 2018;417:47–57. Mar 28
- Gao YS, Liu XZ, Zhang YG, et al. Knockdown of long noncoding RNA LUCAT1 inhibits cell viability and invasion by regulating miR-375 in glioma. Oncol Res. 2018;26:307–313.
- Sun Y, Jin SD, Zhu Q, et al. Long non-coding RNA LUCAT1 is associated with poor prognosis in human non-small lung cancer and regulates cell proliferation via epigenetically repressing p21 and p57 expression. Oncotarget. 2017;8:28297–28311.
- Liu Y, Liu T, Bao X, et al. Increased EZH2 expression is associated with proliferation and progression of cervical cancer and indicates a poor prognosis. Int J Gynecol Pathol. 2014;33:218–224.
- Danza K, Silvestris N, Simone G, et al. Role of miR-27a, miR-181a and miR-20b in gastric cancer hypoxia-induced chemoresistance. Cancer Biol Ther. 2016;17:400–406.
- Azumi J, Tsubota T, Sakabe T, et al. miR-181a induces sorafenib resistance of hepatocellular carcinoma cells through downregulation of RASSF1 expression. Cancer Sci. 2016;107:1256–1262.
- Luo C, Qiu J. miR-181a inhibits cervical cancer development via downregulating GRP78. Oncol Res. 2017;25:1341–1348.
- Chen Y, Ke G, Han D, et al. MicroRNA-181a enhances the chemoresistance of human cervical squamous cell carcinoma to cisplatin by targeting PRKCD. Exp Cell Res. 2014;320:12–20.