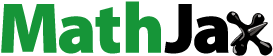
Abstract
Elicited plant in vitro cultures are gaining more interest worldwide for their potential in the uniform production of industrially important secondary metabolites. In the present study, different ratios of silver nanoparticles (AgNPs) and plant growth regulators (PGRs) were supplemented to in vitro cultures for the sustainable production of biomass and antioxidant secondary metabolites through callus cultures of Caralluma tuberculata. Results indicated that various concentrations of AgNPs significantly affected the callus proliferation and substantially increased the callus biomass, when combined with PGRs in the MS (Murashige and Skoog) media. The highest fresh (0.78 g/l) and dry (0.051 g/l) biomass accumulation of callus was observed in the cultures raised in vitro at 60 µg/l AgNPs in combination with 0.5 mg/l 2,4-D plus 3.0 mg/l BA. Phytochemical analysis of the callus cultures showed higher production of phenolics (TPC:3.0 mg), flavonoids (TFC:1.8 mg), phenylalanine ammonialyase activity (PAL: 5.8 U/mg) and antioxidant activity (90%), respectively, in the callus cultures established on MS media in the presence of 90 ug/l AgNPs. Moreover, enhanced activities of antioxidant enzymes such as superoxide dismutase (SOD: 4.8 U/mg), peroxidase (POD: 3.3 U/mg), catalase (CAT: 2.5 U/mg) and ascorbate peroxidase (APX: 1.9 U/mg) were detected at higher level (90 ug/l) of AgNPs tested alone for callus proliferation in the MS media. It may be concluded that the AgNPs can be effectively utilized for the enhancement of bioactive antioxidants in the callus cultures of C. tuberculata, a highly medicinal and threatened plant. This protocol can be scaled up for the industrial production of plant biomass and pharmacologically potent metabolites in C. tuberculata.
Introduction
Nanomaterials are the small size (1–100 nm) substances with unique physiochemical properties. Different varieties of nanomaterials including silver, gold, zinc, copper, titanium, silicon and magnesium nano particles are commercially available, having diverse applications in biology, agriculture and medicine [Citation1]. Among the different types of nanomaterials, silver nanoparticles (AgNPs) have received global attraction due to their tremendous physiological properties including higher anti-microbial potential. Besides, AgNPs have been used widely for their pertinent potential in influencing plant cell growth, biomass production and production of notable secondary metabolites in plant cell cultures [Citation2]. Using AgNPs either solitary in the growth media or in augmentation with plant growth regulators (PGRs), such α-naphthalene acetic acid (NAA) profoundly influenced the callus growth and antioxidants potential in P.vulgaris [Citation3]. Caralluma tuberculata belongs to the family Asclepediaceae is a leafless, fleshy, important medicinal and edible plant. Its stem is angular and grows up to 15 cm. The branches are terminate with a flower of dark purple petals [Citation4]. C. tuberculata is locally known as chung, boteri, marmut, marmootk, aputag and pamanky in different regions of the world [Citation5]. Caralluma tuberculata contains a variety of bioactive metabolites, having higher antioxidant potential. The plant is mostly used in treatment against diabetes, inflammation, asthma, paralysis, obesity, joints pains and fever. Nonetheless, the plant extracts have shown positive results through in vitro assays, against human carcinoma cell lines [Citation6,Citation7]. Due to the ruthless collection and overexploitation for pharmaceutical applications, C. tuberculata plant species have drastically disappeared in their natural habitat in different localities of the world. According to the criteria devised by IUCN (International Union for the Conservation of Nature) red list categories, C. tuberculata is described as a critically endangered species. In Pakistan, two species of Caralluma, that is C. tuberculata and C. edulis, grow wild in the hilly areas of Sulaiman and the Hindu Kush region in the Khyber Pakhtunkhwa province [Citation8]. Due to less viability of C. tuberculata seeds, the rate of germination is very less and the plant is mainly propagated through stem cutting which is a time-consuming method. The plant needs alternative methods for propagation, production of biomass and conservation. Plant cell cultures offer promising technology for the rapid propagation and secondary metabolites production in plants in short period of time. Besides, the plant cell culture technology may ensure the conservation (ex-situ) of those plants which are threatened or at the risk of extinction in their natural habitat [Citation9]. Among the various plant cell culture approaches, callus culture is the suitable method for feasible production of healthy plant material and bio active phytochemicals. Callus can be manipulated further for the in vitro regeneration of entire plants [Citation10,Citation11]. Till date, no previous research article is available in literature to show the effects of AgNPs on callus biomass formation and production of antioxidants in callus cultures of C. tuberculata. Therefore, the overall objective of the current study was to evaluate the possible effects of AgNPs on callus induction, biomass formation and production of desirable levels of metabolites including phenolics and flavonoids in C. tuberculata. Moreover, free radical scavenging activity and the antioxidant enzyme activities were also determined in the callus cultures.
Materials and methods
Establishment of callus cultures
Growth room potted plantlets of C. tuberculata were used to harvest the shoot explants and to develop callus cultures. Practically, 0.5 cm explants (fresh apical shoot tips) were inoculated on the solid surface of Murashige and Skoog [Citation12] media, initially supplemented with 0.5 mg/l 2,4-D + 3.0 mg/l BA. Further, 30 g/l sucrose as carbon source and 8 g/l agar (Oxoid, England) were added to the MS media. Then, pH of the MS media containing plant growth regulators (PGRs) was attuned to 5.7 (pH 510, Eutech Instruments, Singapore). The flasks containing media were placed in autoclave and were sterilized at 121 °C for 20 min (Systec, Germany). The cultures were then placed under light intensity of 40–50 µmol m−2 s−1 at 25 °C in the growth chamber. The photoperiod of the chamber was set as 16/8 h for callus culture development.
Application of AgNPs on callus proliferation and biomass formation
To evaluate the effects of AgNPs and PGRs on callus proliferation, the calli established on MS media augmented with 2,4-D (0.5 mg/l) + BA (3.0 mg/l) were derived from cultures after 49 days of culture period. The calli were cut into small pieces using sterile forceps and surgical blade, placed on sterile filter paper and were subsequently cultured on MS media comprised of AgNPs lonely or in mix with PGRs. AgNPs were purchased from Sigma (Aldrich) with catalogue number 730807. The characterization data of AgNPs revealed the size of nanoparticles as 40 nm. All the details of the characterization of AgNPs can be seen in . Stock solution of AgNPs was prepared by following the protocol of Rahman et al. [Citation13].
Figure 1. Synthesis and characterization of AgNPs. (A) 40 nm silver nanoparticles, (B) representative spectra of silver nanoparticle dispersion, 10–200 nm, (C) TEM of 40 nm silver nanoparticles. Representative TEM, batch-to-batch variation may be expected.
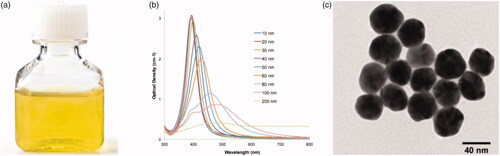
Varying concentrations (30, 60 and 90 μg/l) of AgNPs are either tested alone or in combination with 2,4-D (0.5 mg/l) + BA (3.0 mg/l) in the MS media. The MS media augmented with 2,4-D (0.5 mg/l) + BA (3.0 mg/l) was used as a control treatment. Further, sucrose (30 g/l) was used in the culture of MS media, which was solidified with agar (8.0 g/l). The pH of the prepared media was set to 5.7 and then all the media were autoclaved at 121 °C for 20 min. For sterilization of AgNPs solution, micro filter was used; after filtration, the AgNPs at different concentrations were added in autoclaved MS media under aseptic condition. Finally, autoclaved test tubes were filled with almost 9 ± 1 ml medium in each tube and sealed with a cotton plug. All the cultures were maintained in the growth chamber for callus proliferation at 25 ± 1 °C under a 16/8 h photoperiod. Data were collected after 49 days of culture incubation time to evaluate the influence of various concentrations of AgNPs with PGRs on fresh weight (FW), dry weight (DW), callus moisture content and callus morphological characteristics. For the determination of FW, the calli harvested from all the respective treatments were washed with sterilized distilled water. After that, each callus was kept on filter paper, pressed by using forceps to remove the extra water, and then finally weighed. For DW, each callus was kept in an oven (50 °C) for 24 h and then finally weighed. The data of FW and DW were calculated as g/l. Percent moisture content in callus cultures was determines using the FW and DW of callus using the following formula reported by Rashmi and Trivedi [Citation14]:
where A = weight of empty petri dish, B = weight of petri dish with fresh callus, C = weight of petri dish with dried callus.
Phytochemical analysis of the callus cultures raised in vitro in the presence of AgNPs and PGRs
Quantification of total phenolics, total flavonoids, phenylalanine ammonia lyase and DPPH free radical scavenging activity
About 300 mg of callus sample was utilized for the phytochemical analysis. The crushed powder was mixed in 10 ml of 50% methanol and the solution was placed on shaker at 24 rpm for 24 h at 25–30 °C, then sonicated for 30 min and vortexed for 30 min, and again sonicated for 15 min [Citation15]. The mixture was then subjected to centrifugation at 6500 rpm for 10 min. After that, the supernatant was collected, syringe was filtered and shifted to fresh Eppendorf tubes. The extract was diluted to a final concentration of 10.0 (mg/ml) for uniformity and was kept at 4 °C for the phytochemical analysis.
Total phenolic content (TPC) was determined by following the protocol of Velioglu et al [Citation16]. For this assay, 20 µl supernatant of sample from 10 mg/ml sample of each treatment was taken and put into the wells on the 96-well plate. Then, 90 µl from the 10× diluted Folin–Ciocalteu reagent was added to each well and then placed for 5 min at room temperature. Further, 90 µl of sodium carbonate was mixed to make up the mixture volume to 200 µl. In this procedure, the methanol (20 µl) and gallic acid (1 mg/ml) were used as negative and positive control, respectively. Incubating the reaction for 90 min, the absorbance was taken at 630 nm. Data were taken and expressed in standard unit as mg gallic acid equivalent (GAE/g).
For the determination of TFC, the protocol of Chang et al. [Citation17] was used. In this assay, 20 µl of sample was added to a well on the micro plate for the reaction and then 10 µl of AlCl3 (Aluminum chloride: 10 g/l) and 10 µl of CH3CO2K (Potassium acetate: 98.15 g/l) were stepwise supplemented to the reaction mixture. In this procedure, the methanol (20 µl) and quercitin (1.0 mg/mL) were used as negative and positive control, respectively. After that, 160 µl of distilled water (D H2O) was added to adjust the final volume to 200 µl. After 30 min of incubation of the reagents for the complete chemical reaction, the absorbance was measured at 450 nm. The results were expressed as mg quercitin equivalent (QE) per gram.
For the determination of PAL activity in the selected callus samples, the procedure of Khan et al. [Citation15] was adopted. For this purpose, about 80–100 mg of each sample was first homogenized using ice-cold buffer of potassium borate (BK3O3) at a concentration of 100 mM, maintained at 8.8 pH in combo with 2 mM mercaptoethanol and finally centrifuged at 12,000 rpm for 10 min at 4◦C. Thereafter, the obtained supernatant was employed for the PAL assay. The reaction mixture was adjusted by the addition of 0.5 ml of the extract of each selected sample in a tube already filled up with 0.5 ml of phenylalanine (con: 4.0 mM−1) and 1.0 ml of potassium borate (BK3O3) buffer (con: 100 mM; pH 8.8). Exactly 2.0 ml of the reaction mixture was incubated at 30 °C and was supplemented with 0.2 ml of 6 M HCl. After half an hour (30 min) the absorbance was taken at 290 nm. Absorbance of the reaction mixture was considered as function of the formation of enzyme product. It was proposed that, one unit of the phenylalanine ammonia lyase activity (U) is the amount of absorbance variation of 0.01.
To evaluate the DPPH free radical scavenging activity, the procedure of Abbasi et al. [Citation18] was employed. In this assay, different amounts, i.e. 10 μl, 5 μl, 2.5 μl and 1 μl of the sample, negative control (methanol) and positive control (ascorbic acid) were added to the 96-well plate. The volume of each sample were adjusted to 1000, 750, 500 and 250 μg/ml by adding 190 μl, 195 μl, 197.5 μl and 199 μl of DPPH0 solution (4.8 mg/50 ml). These samples were taken for the determination of IC50 value. The absorbance was taken at 515 nm. Results as (IC50) were calculated by using positive control for ascorbic acid as equivalent µg/mg of extract. The following equation was used for free radical scavenging activity as percentage of DPPH discoloration.
AE is the absorbance of solution when a particular concentration of extract is added and AD is the absorbance of the DPPH solution when nothing was added to this solution.
Activities and quantification of the antioxidant enzymes
For the preparation of extracts of selected callus samples for the quantification of antioxidant enzymes the method of Khan et al. [Citation15] was used with some modification. Briefly, the dried callus (0.2 g) of each sample was powdered using pestle and mortar by adding 2 ml of phosphate buffer (50 mM, 1% PVP 0.1 mM EDTA and pH 7.8) to develop a homogenate. For homogenation of the samples in the reaction buffer, centrifugation at 12, 000 rpm was repeated twice, each time for 15 min at 4 °C. The obtained supernatants from all the selected callus samples were used for the quantification of antioxidant enzymes. Superoxide dismutase (SOD; EC 1.15.1.1) and peroxidase (POD; EC 1.11.1.7) were determined using the method of Giannopolitis and Ries [Citation19], and Abeles and Biles [Citation20], respectively, whereas catalase (CAT; EC 1.11.1.6) and ascorbate peroxidase (APx; EC 1.11.1.11) were determined using the methods developed by Arrigoni et al. [Citation21] and Miyake et al. [Citation22], respectively.
Experimental design and statistical analysis
All the experiments were conducted in completely randomized design. Each treatment consisted of three replicates, repeated three times and the data were analysed statistically by one-way analysis of variance (ANOVA) using SPSS ver. 16.0 software (Chicago, IL). The mean values and significant differences in the data were calculated using DMRT at p < .05.
Results and discussion
Effects of AgNPs and PGRs on callus proliferation and biomass formation in C. tuberculata
Depending on the size and concentration, interaction of nanomaterials with plant cell influences many physiological and developmental changes during plant cellular growth [Citation23]. In this study, applications of AgNPs significantly affected the callus growth, proliferation and biosynthesis of precious antioxidant compounds. AgNPs at different doses were employed in vitro on apical shoot explants in the MS media in combination with 0.5 mg/l 2, 4-D + 3.0 mg/l BA. Individually the various concentrations of AgNPs, when tested alone did not significantly affect the callus proliferation. Compared with the control treatment (0.5 mg/l 2, 4-D + 3.0 mg/l BA) at which 0.143 g/l FW and 0.140 g/l DW of the callus biomass was recorded, AgNPs at individual levels produced less biomass, wherein the maximum value (0.106 g/l FW and 0.0113 g/l DW) was observed at higher dose (90 μg/l). Morphologically the calli established were fragile and yellow-brownish in colour ( and ). However, when applied in combination with 0.5 mg/l 2, 4-D + 3.0 mg/l BA, a significant increase in the callus biomass was observed. The highest FW (0.780 g/l) and DW (0.051 g/l) in total biomass accumulation of callus was observed in cultures in response to 60 μg/l AgNPs in combination with 0.5 mg/l 2,4-D + 3.0 mg/l BA (; . Further, the calli were visually observed as compact and greenish in colour (). Likewise, in vitro supplementation by AgNPs is reported for the positive role of AgNPs on callus induction and growth in Lycopersicum esculentum [Citation24]. Whereas, profound production of callus was observed in the MS culture media under the effects of 8.0 mg/l Ag NPs in combo with 5.0 mg/l BA and 3.0 mg/l NAA. However, contrary to our data, different culture characteristics in context of callus morphology and colour were noted in the cultures of Lycopersicum esculentum supplemented with AgNPs. In another study, different concentrations of AgNPs showed significantly positive effects on the growth of shoot and root in Zea mays [Citation25].
Figure 2. Pictorial presentation of the in vitro cultures in Caralluma tuberculata (A). Explant inoculated on culture media (B). Callus initiation from explant (C). Callus induction on MS media with 2,4-D (0.5 mg/L) + BA (3 mg/L) (D). Sub-culturing of induced callus (E). Callus proliferated on MS media with 2,4-D (0.5 mg/L) + BA (3 mg/L) (F). Callus proliferated on MS media with 2,4-D (0.5 mg/L) + BA (3 mg/L) + AgNPs 30 μg/L (G). Callus proliferated on MS media with 2,4-D (0.5 mg/L) + BA (3 mg/L) + AgNPs 60 μg/L (H). Callus proliferated on MS media with 2,4-D (0.5 mg/L) + BA (3 mg/L) + AgNPs 90 μg/L (I).
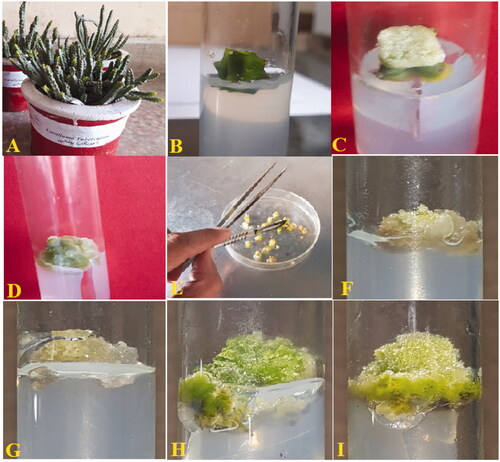
Table 1. Effect of AgNPs either alone or in combination with 2,4-D + BA on callus proliferation in Caralluma tuberculata.
Table 2. Effect of AgNPs either alone or in combination with 2,4-D + BA on callus morphology in Caralluma tuberculata.
In this study, higher level of AgNPs (90 μg/l) beyond an optimal level (60 μg/l) in combination with PGRs resulted in a significant decline in callus biomass (). Determining the optimal concentration of any nano particles, at which the plant cell can attain maximum and healthy growth, is very much crucial in order to prevent the toxicity issues related to application of nanomaterial on plants. For instance, when the explants of Phaseolus vulgaris were treated with different levels of AgNPs, maximum growth parameters during callus growth such as the percent callus induction and biomass were observed at the optimum concentration (50 mg/ml) of AgNPs [Citation26]. Literature regarding the behaviour of nanoparticles to the plant cell and tissue culture development is scare. The mechanism that how AgNPs along with PGRs manage the callus cultures is yet to be explored in medicinal plants including C. tuberculata. Currently, the reports about the absorption, translocation, accumulation, biotransformation and toxicity of NPs are mostly contradictory [Citation27]. The nature of plant species, age and types of tissues and physiological state are among the parameters affected by NPs [Citation28]. Though the exact mechanism of the action of AgNPs in plant cell growth is not yet known, it may be speculated that AgNPs can enhance the plant cell’s nutrient and water uptake from the culture media by mutilating the cell wall. Compared with animal cell, the presence of cell wall in plant cell acts as an important structure for the uptake of nutrients [Citation29].
In this study, the treatments at which the callus cultures were found for accumulation of maximum biomass (FW and DW) also contained maximum moisture content as compared to calli making lowest FW and DW. It was observed that, moisture content varied from 88% to 94% in all the applied treatments (). The variation between FW and DW is equal to the moisture content of the callus. Water content is an essential factor for the optimal photosynthetic activity because it plays a key role in the formation of sugars. Further, the information of the water content in a plant tissue is important to know the metabolic and physiological status responsible for the healthy growth of plant tissues [Citation14].
Effects of AgNPs and PGRs on total phenolic content, total flavonoid content, phenylalanine ammonia lyase activity and DPPH free radical scavenging activity in callus cultures
Several research articles have been reported on the evaluation of polyphenols, flavonoids and antioxidant activities in Caralluma species [Citation7,Citation30,Citation31]. Yet, there was a need for sustainable and standardized method for maximum production of flavonoids and phenolics with higher antioxidant capacity. For this reason, callus of C. tuberculata was subjected to optimum stress condition by incubation in the presence of AgNPs at different concentrations in the MS media.
Total phenolic content (TPC), total flavonoid contents (TFC), phenylalanine ammonia lyase (PAL) and DPPH free radical scavenging activity were evaluated in the callus cultures, established under the effects of different AgNPs treatments. Unlike data on the callus growth, AgNPs were found to boost the production of higher levels of secondary metabolites, when tested alone.
Wherein, highest amount of TPC (3.0 mg), TFC (1.8 mg), PAL (5.8 U/mg) and DPPH free radical activity (90%) were observed in the calli established at 90 μg/l AgNPs, added alone in the MS media (). No significant increase in the quantities of these metabolites was observed in the callus samples produced at the same concentration (90 μg/l) of AgNPs in the MS media augmented with PGRs. The antioxidant potential in a plant tissue is by virtue of the presence of phenolics and flavonoids. Manipulation in the in vitro micro propagation methods through changing physical factors, i.e. light, temperature and most importantly the utilization of different nano materials as elicitor compounds in the culture media can significantly enhance the antioxidant potential [Citation32]. During unfavourable environment, reactive free radicals are produced in higher quantities, which form complex chain reactions, which eventually effect cells and tissues and their processes [Citation33]. In such circumstances, plants defence system release polyphonic contents (phenol and flavonoid) as natural antioxidants [Citation32]. These natural antioxidants reduced the activity of free radicals or inhibit it completely from formation of ROS, which directly fluctuate the activities of lipids, proteins and synthesis of DNA molecule [Citation34]. That is why the antioxidant potential in plant-based products is due to the polyphenolics contents.
Figure 3. Assessment of the non-enzymatic antioxidant elements in the callus cultures of Caralluma tuberculata established in response to different concentration of AgNPs treatments. Data represent the mean values of triplicates with ± standard error. Columns data with different letter/s differ significantly at p ≤ .05 (A): total phenolic content (TPC), (B) total flavonoid content (TFC), (C) phenylalanine ammonia lyase (PAL) activity, (D) free radical scavenging activity.
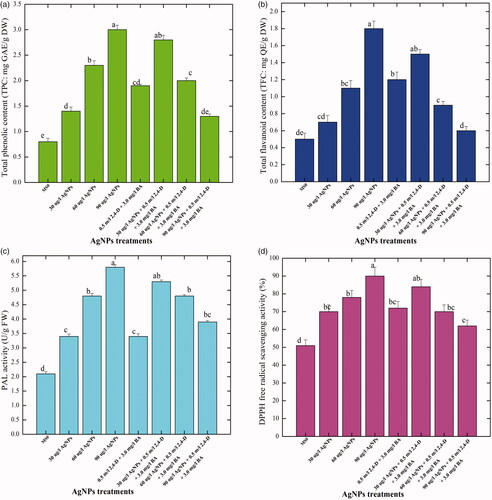
In the current research work, considerable levels of TPC (2.8 mg), TFC (1.5 mg), PAL (5.3 U/mg) and DPPH free radical activity (84%), respectively were detected in the callus samples in response to 30 μg/l AgNPs in combination with 0.5 mg/l 2,4-D + 3.0 mg/l BA. Further, a significant decrease in the production of secondary metabolites was observed, when higher concentrations of AgNPs were combined with PGRs in the media (). Higher concentration (90 μg/l) of AgNPs augmented with PGRs showed lower values for TPC, TFC, PAL and DPPH activity (1.3 mg, 0.6 mg, 3.9 U/mg and 62%) in the callus cultures, as compared with the production of the metabolites by AgNPs at the same concentration without PGRs. Thus, it is clear from the results that AgNPs alone were more supportive for the enhancement of TPC, TFC, PAL and DPPH activity in the callus cultures (). Nanoparticles are known to greatly affect the callus physiology and secondary metabolism by producing a situation of an oxidative stress in the callus tissue, which eventually activate plant metabolic responses to mitigate the effects of oxidative outburst through production of different types of phytochemicals [Citation35]. The augmentation of AgNPs to solid culture media was found encouraging for production of polyphenolics content in callus cells of C. tuberculata. The information on polyphenolics content in in vitro-based materials of Caralluma are still absent in the literature cited; however, various medicinal plant species are exploited for the production of polyphenolics content [Citation36–38]. Khan et al. [Citation39] and Saeed et al. [Citation40] observed that elicitors and PGRs are not only involved in the process of morphogenesis but also fluctuate the pathways of secondary metabolism to release higher contents. Ali et al. [Citation29] observed maximum accumulation of phenolics in callus cultures than other tissues in A. bracteosa. In accordance to our findings, a similar pattern in the production of secondary metabolites was also observed by Ghasemzadeh et al. [Citation41] in two varieties of Zingiber officinale. These results suggest that NPs-induced synthesis of polyphenolics content is a valid approach for industrial production of these products. Furthermore, these AgNPs have a potential for improvement of physiological and morphological characteristics of plants.
Effects of AgNPs and PGRs on antioxidant enzyme activities in callus cultures
Both biotic and abiotic stresses delay the cell differentiation during callogenesis by the unwanted production of reactive oxygen species (ROS) and produce toxic metabolites that can directly injure the cells [Citation42]. To fight against such oxidative stress resulted ROS, plants produce specific enzymes, i.e. SOD, POD, CAT, and APX which provide a strong defence system and scavenge the toxic-free radicals during cell division [Citation43]. In the literature cited, still there is inadequate information’s regarding the effect of AgNPs on stress enzymes in C. tuberculata and other medicinal plants callus cultures.
In the present research investigation, activities of the most essential antioxidant enzymes, i.e. SOD, POD, CAT and APx were evaluated in the callus cultures produced in response to different concentration of AgNPs either alone or in combination with 0.5 mg/l 2, 4-D + 3.0 mg/l BA. Among all the applied treatments, AgNPs at a concentration of 90 μg/l without PGRs resulted in higher activities of SOD, POD, CAT and APx (4.8, 3.3, 2.5 and 1.9 U/mg protein, respectively) in the callus cultures (). No considerable increase in the activities of antioxidant enzymes was detected in the callus cultures established under the effects of AgNPs in combination with PGRs. Compared with control treatment, at higher doses of AgNPs (90 μg/l) in combination with PGRs (0.5 mg/l 2, 4-D + 3.0 mg/l BA), lower values in the activities of antioxidant enzymes (SOD:1.2 U/mg, POD: 1.2 U/mg, CAT: 0.6 U/mg and APx: 0.5 U/mg), respectively, were observed in the callus samples (). It means that AgNPs alone at higher concentrations were found much efficient for the production of antioxidant enzymes. In a similar study, Sharma et al.[Citation44] evaluated the impact of various ratios of AgNPs on the enzymatic activity of Brassica juncea callus. They concluded that the augmentation of AgNPs in culture media enhanced the antioxidant enzymes activities in B. juncea. Our results demonstrated that at higher concentrations AgNPs enhanced the activity of SOD in callus cultures, when compared with the lower doses. Similarly, Faisal et al. [Citation45] reported that when tomato plants were treated with higher doses of NPs, significantly higher activation of SOD was observed. On the contrary, Ma et al. [Citation46] concluded lower SOD activity in Oryza sativa and Allium cepa in response to higher concentration of NPs. The specific antioxidant enzymes activity in callus improved with the increase of AgNPs concentration in culture media. Lu et al. [Citation47] suggested that when soybean seeds treated with a combination of nano TiO2 and nano SiO2 enhances the activities of SOD, POD, CAT and APx. NPs might have improved the specific enzymes activities SOD, APx and CAT [Citation48]. In another research study, when seeds of Ricinus communis were exposed to AgNPs, a significant increase in the activities of antioxidant enzymes (POD and SOD) was observed in comparison to non-treated seeds [Citation49]. SOD is widely known for its pivotal role in management of oxidative stress for defence mechanism [Citation38]. Several authors have been reported that the enhancement of SOD activity is correlated by increasing the tolerance of plants against ecological stresses [Citation46]. Assessment of the influence of AgNPs on plant antioxidant enzymatic system such as CAT, POD, SOD and APx and non-enzymatic antioxidant elements such as TPC and TFC is crucial to better understand the cellular mechanisms underlying the plant–nano materials interaction. It is proposed that NPs might act as signal compounds, those like in nature to the chemical elicitors and influence cellular growth and secondary metabolism. Upon exposure to application of NPs, plant cell goes through a series of events in a cascade manner, resulting in oxidative outburst and generation of singlet oxygen species (ROS) in the surrounding environment of the plant cell. The ROS in turn can damage cell membrane and nuclei. To cope with the intense stress situation and to scavenge the ROS, plants activate their metabolic pathways including the notable mitogen-activated protein kinase (MAPK) pathway (. MAPK activation propels the plant antioxidant elements to come in contact with ROS in a cascade fashion. The plant secondary metabolites scavenge the ROS and as a result the cellular responses such as cell division and differentiation are regulated to attain the normal growth and development of plant cell [Citation50]. Among the antioxidant enzymes, CAT and APX can scavenge substantially the ROS scavenger and play a key role to mitigate the oxidative stress [Citation51]. Several research articles have been reported to evaluate the effects of NPs on secondary metabolism in in plants. It was found that CeO2 NPs treatment positively affected the activities of CAT, SOD, POD and APX in A. thaliana [Citation46].
Figure 4. Assessment of the enzymatic antioxidant elements in the callus cultures of Caralluma tuberculata established in response to different concentration of AgNPs treatments. Data represent the mean values of triplicates with ± standard error. Columns data with different letter/s differ significantly at p ≤ .05 (A): superoxide dismutase (SOD) activit, B: peroxidase (POD) activity, C: catalase (CAT) activity, D: ascorbate peroxidase (APx) activity.
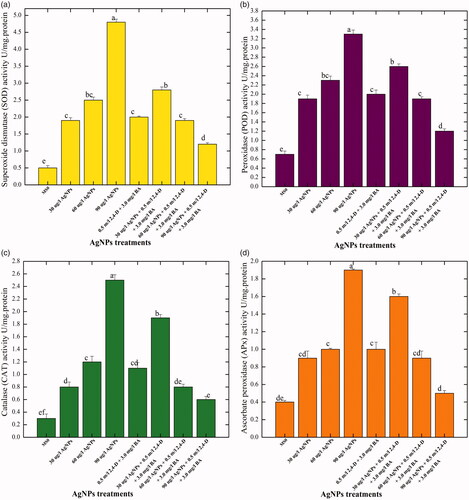
Conclusions
Caralluma tuberculata is an important medicinal herb and needs sustainable means for enhancement of biomass production and medicinal metabolites. In the present study, for the first time a feasible protocol for callus formation was standardized. AgNPs supplementation in vitro in combination with PGRs resulted in the proliferation of callus. However, solitary application of AgNPs was found inductive in the biosynthesis of higher amounts of different antioxidant secondary metabolites. This is the first practical application of nanotechnology in the bioprocess of plant callus cultures. But, this study need further research to understand the molecular mechanism of these nanoparticles in cell developmental processes and secondary metabolism. It further needs to understand their movement and chemistry for future health challenges.
Disclosure statement
The authors declare that they have no conflict of interest regarding this publication.
Data availability
All the required data, which is related to current study, are embedded in this manuscript.
References
- Ruttkay-Nedecky B, Krystofova O, Nejdl L, et al. Nanoparticles based on essential metals and their phytotoxicity. J Nanobiotechnol. 2017;15:33.
- Elechiguerra JL, Burt JL, Morones JR, et al. Interaction of silver nanoparticles with HIV-1. J Nanobiotechnol. 2005;3:6.
- Fazal H, Abbasi BH, Ahmad N, et al. Elicitation of medicinally important antioxidant secondary metabolites with silver and gold nanoparticles in callus cultures of Prunella vulgaris L. Appl Biochem Biotechnol. 2016;180:1076–1092.
- Collenette S. Wildflowers of Saudi Arabia. Riyadh, Kingdom of Saudi Arabia: National Commission for Wild life Conservation and Development; 1999.
- Rizwani GH. Phytochemical and biological studies on medicinal herbs, Caralluma tuberculata and Caralluma edulis. Karachi: University of Karachi; 1991.
- Ansari N, Houlihan L, Hussain B, et al. Antioxidant activity of five vegetables traditionally consumed by south‐Asian migrants in Bradford, Yorkshire, UK. Phytother Res. 2005;19:907–911.
- Rauf A, Jan M, Rehman W, et al. Phytochemical, phytotoxic and antioxidant profile of Caralluma tuberculata NE Brown, Wudpecker. J Pharmacy Pharmacol. 2013;2:21–25.
- Abdul-Aziz Al-Yahya M, Abdel-Sattar E, Guittet E. Pregnane glycosides from Caralluma russeliana. J Nat Prod. 2000;63:1451–1453.
- Anis M, Faisal M. In vitro regeneration and mass multiplication of Psoralea corylifolia—an endangered medicinal plant. Indian J Biotechnol. 2005;4:261–264.
- Manjkhola S, Dhar U, Joshi M. Organogenesis, embryogenesis, and synthetic seed production in Arnebia euchroma—a critically endangered medicinal plant of the Himalaya. In Vitro Cell Dev Biol Plant. 2005;41:244–248.
- Rani R, Khan MA, Kayani WK, et al. Metabolic signatures altered by in vitro temperature stress in Ajuga bracteosa Wall. ex. Benth. Acta Physiologiae Plantarum. 2017;39:97.
- Murashige T, Skoog F. A revised medium for rapid growth and bio assays with tobacco tissue cultures. Physiol Plant. 1962;15:473–497.
- Rahman L-u, Qureshi R, Yasinzai MM, et al. Synthesis and spectroscopic characterization of Ag–Cu alloy nanoparticles prepared in various ratios. Comptes Rendus Chimie. 2012;15:533–538.
- Rashmi R, Trivedi MP. Callus induction and callogenic response of Rauvolfia serpentina and Catharanthus roseus by using various growth hormone concentrations singly and in combination. Int J Sci Eng Res. 2014;5(1):300.
- Khan MA, Abbasi BH, Ahmed N, et al. Effects of light regimes on in vitro seed germination and silymarin content in Silybum marianum. Industr Crops Prod. 2013;46:105–110.
- Velioglu Y, Mazza G, Gao L, et al. Antioxidant activity and total phenolics in selected fruits, vegetables, and grain products. J Agric Food Chem. 1998;46:4113–4117.
- Chang C-C, Yang M-H, Wen H-M, et al. Estimation of total flavonoid content in propolis by two complementary colorimetric methods. J Food Drug Anal. 2002;10:178–182.
- Abbasi BH, Khan MA, Mahmood T, et al. Shoot regeneration and free-radical scavenging activity in Silybum marianum L. Plant Cell Tiss Organ Cult. 2010;101:371–376.
- Giannopolitis CN, Ries SK. Superoxide dismutases: I. Occurrence in higher plants. Plant Physiol. 1977;59:309–314.
- Abeles FB, Biles CL. Characterization of peroxidases in lignifying peach fruit endocarp. Plant Physiol. 1991;95:269–273.
- Arrigoni O, De Gara L, Tommasi F, et al. Changes in the ascorbate system during seed development of Vicia faba L. Plant Physiol. 1992;99:235–238.
- Miyake C, Shinzaki Y, Nishioka M, et al. Photoinactivation of ascorbate peroxidase in isolated tobacco chloroplasts: Galdieria partita APX maintains the electron flux through the water–water cycle in transplastomic tobacco plants. Plant Cell Physiol. 2006;47:200–210.
- Khodakovskaya MV, De Silva K, Biris AS, et al. Carbon nanotubes induce growth enhancement of tobacco cells. ACS Nano. 2012;6:2128–2135.
- Ewais E, Desouky S, Eshazly E. Studies on callus induction, phytochemical constituents and antimicrobial activity of Solanum nigrum L.(Solanaceae). Nature Sci. 2015;13:133–138.
- Salama HM. Effects of silver nanoparticles in some crop plants, common bean (Phaseolus vulgaris L.) and corn (Zea mays L.). Int Res J Biotechnol. 2012;3:190–197.
- Mustafa HS, Oraibi AG, Ibrahim KM, et al. Influence of silver and copper nanoparticles on physiological characteristics of Phaseolus vulgaris L. in vitro and in vivo. Int J Curr Microbiol Appl Sci. 2017;6:834–843.
- Ma X, Geiser-Lee J, Deng Y, et al. Interactions between engineered nanoparticles (ENPs) and plants: phytotoxicity, uptake and accumulation. Sci Total Environ. 2010;408:3053–3061.
- Vannini C, Domingo G, Onelli E, et al. Morphological and proteomic responses of Eruca sativa exposed to silver nanoparticles or silver nitrate. PLoS One. 2013;8:e68752
- Ali H, Khan MA, Ullah N, et al. Impacts of hormonal elicitors and photoperiod regimes on elicitation of bioactive secondary volatiles in cell cultures of Ajuga bracteosa. J Photochem Photobiol B. 2018;183:242–250.
- Ch S. Preliminary phytochemical screening, total phenol content and invtro antioxidant activity of Caralluma umbellata Haw. JGTPS 2014;5:1603–1611.
- Karthishwaran K, Shamisi SOSOA, Kurup SS, et al. Free-radical-scavenging and antioxidant capacities with special emphasis on enzyme activities and in vitro studies in Caralluma flava NE Br. Biotechnol Biotechnolog Equip. 2018;32:156–162.
- Hong Y, Lin S, Jiang Y, et al. Variation in contents of total phenolics and flavonoids and antioxidant activities in the leaves of 11 Eriobotrya species. Plant Foods Hum Nutr. 2008;63:200
- Sreelatha S, Padma P. Antioxidant activity and total phenolic content of Moringa oleifera leaves in two stages of maturity. Plant Foods Hum Nutr. 2009;64:303.
- Koşar M, Göger F, Başer KHC. In vitro antioxidant properties and phenolic composition of Salvia halophila Hedge from Turkey. Food Chem. 2011;129:374–379.
- Choi O, Hu Z. Size dependent and reactive oxygen species related nanosilver toxicity to nitrifying bacteria. Environ Sci Technol. 2008;42:4583–4588.
- Khan MA, Abbasi BH, Shah NA, et al. Analysis of metabolic variations throughout growth and development of adventitious roots in Silybum marianum L.(Milk thistle), a medicinal plant. Plant Cell Tiss Organ Cult. 2015;123:501–510.
- Abbasi BH, Ali H, Yücesan B, et al. Evaluation of biochemical markers during somatic embryogenesis in Silybum marianum L. Biotechnology. 2016;6:71.
- Ali H, Khan MA, Kayani WK, et al. Thidiazuron regulated growth, secondary metabolism and essential oil profiles in shoot cultures of Ajuga bracteosa. Industr Crops Prod. 2018;121:418–427.
- Khan T, Abbasi BH, Khan MA. The interplay between light, plant growth regulators and elicitors on growth and secondary metabolism in cell cultures of Fagonia indica. J Photochem Photobiol B. 2018;185:153–160.
- Saeed S, Ali H, Khan T, et al. Impacts of methyl jasmonate and phenyl acetic acid on biomass accumulation and antioxidant potential in adventitious roots of Ajuga bracteosa Wall ex Benth., a high valued endangered medicinal plant. Physiol Mol Biol Plants. 2017;23:229–237.
- Ghasemzadeh A, Jaafar HZ, Rahmat A, et al. Effect of different light intensities on total phenolics and flavonoids synthesis and anti-oxidant activities in young ginger varieties (Zingiber officinale Roscoe). IJMS. 2010;11:3885–3897.
- Srinivasan K. Black pepper and its pungent principle-piperine: a review of diverse physiological effects. Crit Rev Food Sci Nutr. 2007;47:735–748.
- Abbasi BH, Khan M, Guo B, et al. Efficient regeneration and antioxidative enzyme activities in Brassica rapa var. turnip. Plant Cell Tiss Organ Cult. 2011;105:337–344.
- Sharma P, Gupta S, Arora S. Effect of silver nanoparticles on antioxidant status of Brassica juncea callus. 2014;8:8–18.
- Faisal M, Saquib Q, Alatar AA, et al. Phytotoxic hazards of NiO-nanoparticles in tomato: a study on mechanism of cell death. J Hazardous Mater. 2013;250:318–332.
- Ma C, Liu H, Guo H, et al. Defense mechanisms and nutrient displacement in Arabidopsis thaliana upon exposure to CeO2 and In2O3 nanoparticles. Environ Sci Nano. 2016;3:1369–1379.
- Lu C, Zhang C, Wen J, et al. Research of the effect of nanometer materials on germination and growth enhancement of Glycine max and its mechanism. Soybean Sci. 2002;21:168–171.
- Lei Z, Mingyu S, Xiao W, et al. Antioxidant stress is promoted by nano-anatase in spinach chloroplasts under UV-B radiation. Biol Trace Elem Res. 2008;121:69–79.
- Yasur J, Rani PU. Environmental effects of nanosilver: impact on castor seed germination, seedling growth, and plant physiology. Environ Sci Pollut Res. 2013;20:8636–8648.
- Sinha AK, Jaggi M, Raghuram B, et al. Mitogen-activated protein kinase signaling in plants under abiotic stress. Plant Signal Behav. 2011;6:196–203.
- Garg N, Manchanda G. ROS generation in plants: boon or bane?. Plant Biosyst. 2009;143:81–96.