Abstract
Osteoarthritis (OA) is a major age-related disease, which may be caused by the accumulation of advanced glycation end-products (AGEs). Excessive degradation of type II collagen and aggrecan by matrix metalloproteinases (MMPs) and a disintegrin and metalloproteinase with thrombospondin type 1 motif (ADAMTS) induced by AGEs is a pivotal event in the pathogenesis of osteoarthritis. In addition, activation of the nuclear factor-κB (NF-κB) pathway induces the expression of a cascade of proinflammatory cytokines, such as interleukin (IL)-1β and tumor necrosis factor-α (TNF-α). In the present study, we investigated the effects of salicin, one of the main constituents of aspirin and a derivative of Alangium chinense, on AGE-induced degradation of the articular extracellular matrix in SW1353 human chondrocytes. Our findings reveal a novel beneficial role of salicin in rescuing degradation of type II collagen and aggrecan, reducing oxidative stress, attenuating expression of proinflammatory cytokines, and inhibiting activation of the NF-κB proinflammatory signaling pathway in chondrocytes stimulated with AGEs. Salicin may thus have potential as a safe and effective therapy against the development and progression of OA.
Introduction
Salicin is a prodrug of acetylsalicylic acid, a main constituent of aspirin and can be derived from the stems and roots of Alangium chinense (Lour.) Harms. (Alangiaceae), a common evergreen shrub native to east and southeast Asia [Citation1]. Alangium chinense has long been used in traditional Chinese medicine (TCM) to treat rheumatic disease, snake bites, circulatory issues, hemostasis, and toxicity, it is also used as a contraceptive or analgesic and to promote wound healing [Citation2,Citation3]. Records of the use of willow bark, another common source of salicylic acid, have been found dating back to the 3rd century B.C. [Citation4]. Numerous substances can be derived from the rhizome, roots, stems, leaves, or flowers of Alangium chinense such as alkaloids, sugars, saponins, steroids, triterpenes, anthraquinones, and glycosides including salicin [Citation2,Citation5,Citation6] Recently, research has focused on methods of extraction of salicin and its potential therapeutic applications [Citation6–8] As a prodrug, salicin is hydrolyzed to salicyl alcohol and then oxidized to salicylic acid in the gut [Citation9]. As a metabolite of salicin, salicylic acid inhibits the activity of cyclo-oxygenase (COX), which plays a major role in regulating pain, fever, and inflammation by metabolizing arachidonic acid, a prostaglandin precursor [Citation10]. However, to the best of our knowledge, this is the first time that the potential effects of salicin have been explored in osteoarthritis (OA). OA is characterized by chronic inflammation and irreversible cartilage destruction, which takes a massive toll on patients’ quality of life and mobility. The main risk factor for OA is age, in part due to the accumulation of advanced glycation end-products (AGEs). AGEs came to exist in the body as a byproduct of the innate process of non-enzymatic glycation as well as via dietary intake, as AGEs are used as a food preservative owing to their high resilience to degradation [Citation11,Citation12]. Some of the factors involved in the development and progression of OA include oxidative stress, secretion of proinflammatory cytokines, recruitment of immune cells, degradation of cartilage and activation of proinflammatory signaling pathways, all of which are demonstrated to be triggered by exposure to AGEs.
The cartilage extracellular matrix (ECM) is primarily composed of type II collagen and aggrecan. Excessive degradation of type II collagen resulting from overexpression of proteolytic enzymes including matrix metalloproteinases (MMPs) by chondrocytes is a major event in the pathogenesis of OA. Of these, collagenases (MMP-1 and MMP-13) cleave the type II collagen triple helix at the site between residues 776 and 778, while stromelysin 1 (MMP-3) cleaves denatured collagen within the telopeptides [Citation13]. Exposure to AGEs has been shown to increase the expression of MMP-1, MMP-3, and MMP-13 in human osteoarthritic chondrocytes [Citation14]. Aggrecan is degraded by a disintegrin and metalloproteinase with thrombospondin motifs (ADAMTS), of which ADMATS-4 and ADAMTS-5 have been shown to play a major role in osteoarthritic joint deterioration [Citation15]. In the present study, we demonstrated the effects of salicin on AGE-induced oxidative stress, secretion of the proinflammatory cytokines interleukin 1β (IL-1β) and tumor necrosis factor alpha (TNF-α), expression of the chemokine monocyte chemoattractant 1 (MCP-1) and the non-histone chromosomal protein high-mobility group protein 1 (HMGB-1), as well as degradation of components of the articular ECM and activation of the NF-κB signaling pathway. Our results demonstrate the promise of salicin as a novel therapy for the treatment and prevention of OA.
Materials and methods
Cell culture and treatment
Human SW1353 cells were purchased from ATCC (Manassas, WV, USA). Cells were maintained in DMEM (Gibco, Grand Island, NY, CA) supplemented with 10% FBS (Gibco, Grand Island, NY, CA, USA) and 1% penicillin-streptomycin (P/S, Sigma-Aldrich, St Louis, MO, USA). Cells were treated with 100 μg/ml AGEs in the presence or absence of 50 and 100 μM salicin for 48 h.
Real-time PCR analysis
Total RNA from human SW1353 cells was isolated using Qiazol reagent (Qiagen , Germany). The quality and concentration of isolated RNA were assessed using a UV spectrophotometer (Thermo Fisher Scientific, Waltham, MA, USA). cDNA was generated through the reverse transcription PCR (RT-PCR) with 1 µg mRNA and a commercial RT-PCR kit (Bio-Rad, Hercules, CA, USA). Real-time PCR analysis was performed to determine the expression of target genes using an SYBR Green real-time PCR Kit on a 7500 Real-time PCR analysis (Waltham, MA, USA). The relative units were calculated by a comparative Ct method normalized to GAPDH. The following primers were used in this study: TNF-α: forward, 5′-GATGGACTCACCAGGTGAG-3′, reverse, 5′-CTCATGGTGTCCTTTCCAGG-3′);
IL-1β: forward, 5′-TACCTGTCCTGCGTGTTGAA-3′; reverse, 5′-TCTTTGGGTAATTTTTGGGATCT-3′;
MCP-1: forward, 5′-AGCCAGATGCAGTTAATGCCC-3′; reverse, 5′-ACACCTGCTGCTGGTGATTCTC-3′;
MMP-1: forward, 5’-CTG AAG GTG ATG AAG CAG CC-3’, reverse, 5′-AGTCCA AGA AATGGCCGAG-3′;
MMP-3: forward, 5′-CCTCTATGGACCTCCCACAGAATC-3′, reverse, 5′-GGTGCTGACTGCATCGAAGGACAAA-3′;
MMP-13: forward, 5′-CTGGCCTGCTGGCTCATGCTT-3′, reverse, 5′-CCTCAGAAAGAGCAGCATCGATATG-3′;
ADAMTS-4: forward, 5′-ACACTGAGGACTGCCCAAC-3’; reverse, 5′-GGTGAGTTTGCACTGGTCCT-3′;
ADAMTS-5: forward: 5′-GCAGAACATCGACCAACTCTACTC-3′; reverse, 5′-CCAGCAATGCCCACCGAAC-3′.
GAPDH: forward, 5′-ACTGGCGTCTTCACCACCAT-3′, reverse, 5’-AAG GCC ATG CCA GTG AGC TT-3’.
Western blot analysis
Human SW1353 cells were treated with 100 μg/ml AGEs in the presence or absence of 50 and 100 μM salicin for 48 h. Cells were harvested and lysed with the RIPA buffer containing protease and phosphatase inhibitors. The specimens from each test and control group were electrophoresed on 10% SDS-polyacrylamide gels. The separated proteins on the gels were then transferred to PVDF membranes, which were then blocked with 5% non-fat milk at room temperature (RT) for 2 h. After that, membranes were probed with each primary antibody at 4 °C overnight. Membranes were then washed three times and incubated with horseradish peroxidase (HRP)-labeled secondary antibody for 2 h at RT. Subsequently, blots were visualized using an enhanced chemiluminescence kit (GE Healthcare , Boston, MA, USA). The following antibodies were used in this study: mouse monoclonal antibody (mAb) against type II collagen (1:1000, #MAB8887, Chemicon, Billerica, MA, USA); Rabbit mAb against Lamin B (1:5000, #12255, Cell Signaling Technology, Danvers, MA, USA); Mouse mAb against β-actin (1:10000, #3700, Cell Signaling Technology, USA); Mouse mAb against aggrecan (1:1000, #ab3778, Abcam , Cambridge, MA, USA); Rabbit monoclonal antibody against p65 (1:3000, #4767, Cell Signaling Technology, USA); Anti-rabbit IgG, HRP-linked secondary antibody (1:3000, #7074, Cell signaling technology, USA); Anti-mouse IgG, HRP-linked antibody (1:3000, #7076, Cell signaling technology, USA).
Reactive oxygen species (ROS) measurement
Intracellular ROS was determined by DCFH-DA staining to reflect oxidative stress in human SW1353 chondrosarcoma cells. Human SW1353 cells were treated with 100 μg/ml AGEs in the presence or absence of 50 and 100 μM salicin for 48 h. After three washes, cells were then loaded with DCFH-DA for 30 min in the dark. Fluorescence was recorded with a fluorescent microscope at an excitation wavelength of 488 nm. Fluorescent intensity was determined by the Image J software (NIH, USA).
Protein carbonyl assay
To investigate oxidative stress in human SW1353 cells, a commercial protein carbonyl ELISA kit (#STA-310, Cell Biolabs, San Diego, CA, USA) was used to measure protein carbonyls. Cells were treated with 100 μg/ml AGEs in the presence or absence of 50 and 100 μM salicin for 48 h. Protein lysates were prepared and added to an ELISA plate to react with dinitrophenylhydrazine (DNPH). Wells of the ELISA plate were then washed 3 times and sequentially probed with an anti-DNPH antibody for 3 h and a horseradish peroxidase-conjugated second antibody for 1 h at RT. The total level of protein carbonyls was calibrated by oxidized BSA.
ELISA
The release of pro-inflammatory cytokines including IL-1β, TNF-α, MCP-1, MMP-1, MMP-3, MMP-13, ADAMTS-4, and ADAMTS-5 were measured by the ELISA assay using commercial kits from R&D Systems in accordance with the manufacturer’s instructions. Secretions of HMGB-1 were measured using the HMGB-1 ELISA Kit (Shino Test Corporation, Tokyo, Japan) according to the manufacturer’s protocols. Results were recorded using a 96-plate reader spectrometry.
Luciferase activity assay
A luciferase assay was used to determine the transcriptional capacity of NF-κB. Briefly, cells were co-transfected with the NF-κB promoter and a firefly luciferase promoter vectors using the Lipofectamine 2000 (#11668027, Invitrogen, Carlsbad, CA, USA). 24 hours after transfection, cells were treated with 100 μg/ml AGEs in the presence or absence of 50 and 100 μM salicin for 48 h. The dual luciferase activity of renilla and firefly luciferase was measured with a dual luciferase reporter assay system kit (Promega, Madison, WI, USA).
Statistical analysis
Results are shown as the mean ± standard deviation (SD). Differences among different treatment groups were analyzed by one-way analysis of variance (ANOVA). p < .05 was considered as statistically significant differences.
Results
Salicin reduces oxidative stress in SW1353 cells
Cellular oxidative stress is a result of the accumulation of AGEs and can be measured based on cellular production of reactive oxygen species (ROS) and oxidative protein carbonylation [Citation16]. Here, we investigated the effects of treatment with salicin on SW1353 cells exposed to 100 ng/ml AGEs. As shown in 00 ng/ml AGEs gave rise to more than 3-fold production of ROS by SW1353 cells, while treatment with 50 and 100 µM salicin significantly reduced the level of ROS in a dose-dependent manner to only roughly 2.2- and 1.3-fold, respectively. Concordantly, exposure to 100 ng/ml AGEs increased the level of carbonylated protein by roughly 3-fold, which was reduced to approximately 2.2- and 1.2-fold by treatment with 50 and 100 µM salicin in a dose-dependent manner (). These findings demonstrate the ability of salicin to ameliorate markers of oxidative stress induced by AGEs in SW1353 human chondrocytes.
Figure 1. Salicin treatment suppressed AGE-induced oxidative stress in human SW1353 chondrosarcoma cells. Human SW1353 cells were treated with 100 μg/ml AGEs in the presence or absence of 50 and 100 μM salicin for 48 h. (A). Intracellular ROS was determined by the DCFH-DA assay; (B). The protein carbonyl content was determined by the 2, 4-dinitrophenyl-hydrazine (DNPH) assay (a, b, c, p < .01 vs. previous column group).
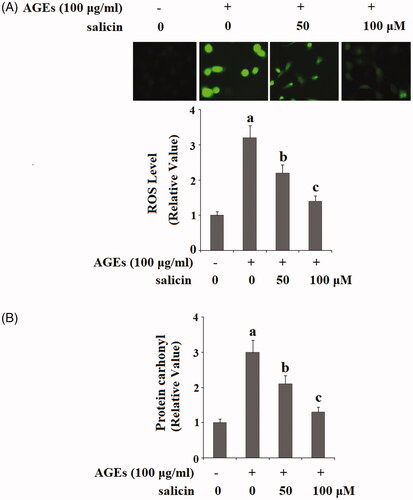
Salicin attenuates secretion of proinflammatory cytokines and chemokines
Proinflammatory cytokines and chemokines play a major role in the inflammatory response in OA. Expression of IL-1β induces production of the proinflammatory cytokine TNF-α and has been shown to be increased in OA [Citation17]. In addition, expression of the proinflammatory chemokine MCP-1 perpetuates inflammation by recruiting macrophages to joint tissues and has been shown to play a unique role in the pathology of OA [Citation18]. In the present study, we measured the effect of salicin on AGE-induced expression of IL-1β, TNF-α, and MCP-1 in SW1353 human chondrocytes. Briefly, chondrocytes were exposed to 100 ng/ml AGEs in the presence or absence of 50 and 100 µM salicin for 48 h. As demonstrated by the results of real-time PCR and ELISA analysis in , respectively, exposure to AGEs increased the mRNA and protein levels of all three of these factors by roughly 4- and 3-fold. However, salicin caused a significant reduction in the expression of IL-1β, TNF-α, and MCP-1 at both the mRNA and protein levels in a dose-dependent manner, with 100 µM salicin returning protein levels of the three factors to close to baseline ().
Figure 2. Salicin treatment suppressed the expression and secretions of pro-inflammatory cytokines. Human SW1353 cells were treated with 100 μg/ml AGEs in the presence or absence of 50 and 100 μM salicin for 48 h. (A). Expressions of IL-1β, TNF-α, and MCP-1 at the mRNA levels were determined by real time PCR analysis; (B). Secretion of IL-1β, TNF-α, and MCP-1 was determined by ELISA.
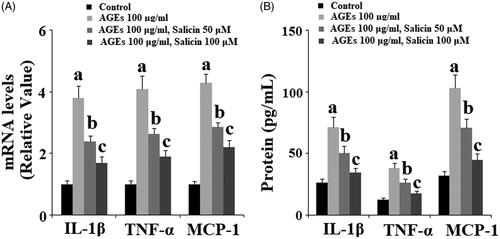
High-mobility group protein 1 (HMGB-1) has been shown to play a role in regulating inflammation in diverse tissues and disease states by activating cells via RAGE, among other surface receptors [Citation19–21]. Here, we endeavored to determine the effects of salicin on the expression of HMGB-1 in SW1353 cells. As demonstrated by the results of ELISA analysis in , exposure to 100 ng/ml AGEs induced roughly 3-fold expression of HMGB-1, which was ameliorated by treatment with 50 and 100 µM salicin in a dose-dependent manner.
Figure 3. Salicin treatment inhibited the secretion of high-mobility group protein 1 (HMGB-1). Human SW1353 cells were treated with 100 μg/ml AGEs in the presence or absence of 50 and 100 μM salicin for 48 h. Secretion of HMGB-1 was determined by the ELISA assay (a, b, c, p < .01 vs. previous column group).
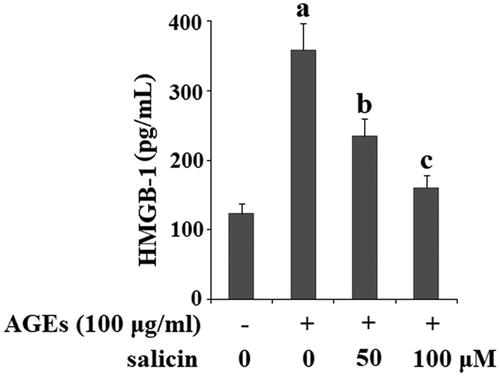
Salicin prevents degradation of type II collagen by MMP-1/3/13
Inhibition of degradation of type II collagen by MMPs including MMP-1, MMP-3, and MMP-13 is a popular target for the prevention and treatment of OA. Here, we endeavored to determine the effects of salicin on AGE-induced degradation of type II collagen by MMPs. Briefly, using β-actin as a positive control, human SW1353 chondrocytes were exposed to 100 ng/ml AGEs in the presence or absence of 50 and 100 µM salicin for 48 h. As demonstrated by the results of Western blot analysis in , exposure to AGEs significantly increased degradation of type II collagen which was ameliorated by 50 and 100 µM salicin in a dose-dependent manner. Concordantly, the results of real-time PCR and ELISA in , respectively, demonstrate that salicin successfully rescued AGE-induced increased expression of MMP-1, MMP-3, and MMP-13 in a dose-dependent manner.
Figure 4. Salicin treatment ameliorated AGEs-induced degradation of type II collagen in human SW1353 cells. Human SW1353 cells were treated with 100 μg/ml AGEs in the presence or absence of 50 and 100 μM salicin for 48 h. Expression of type II collagen was determined by western blot analysis (a, b, c, p < .01 vs. previous column group).
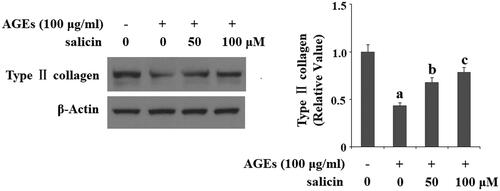
Figure 5. Salicin treatment ameliorated AGEs-induced expression of MMP-1, MMP-3, and MMP-13 in human SW1353 cells. Human SW1353 cells were treated with 100 μg/ml AGEs in the presence or absence of 50 and 100 μM salicin for 48 h. (A). Expression of MMP-1, MMP-3, and MMP-13 at the gene levels was determined by real time PCR analysis; (B). Expression of MMP-1, MMP-3, and MMP-13 at the protein levels was determined by ELISA (a, b, c, p < .01 vs. previous column group).
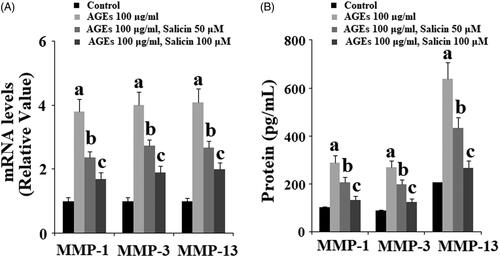
Salicin prevents degradation of aggrecan by ADAMTS-4/5
To further confirm the beneficial effect of salicin against AGE-induced degradation of articular ECM, we measured the degradation of aggrecan using β-actin as a control. As demonstrated by the results of Western blot analysis in , exposure of SW1353 chondrocytes to 100 ng/ml AGEs for 48 h resulted in a significant increase in degradation of aggrecan, reducing the level of aggrecan by roughly 50%, which was prevented by treatment with 50 and 100 µM salicin in a dose-dependent manner. Concordantly, the results of real-time PCR and ELISA analysis shown in , respectively, demonstrate that exposure to 100 ng/ml AGEs for 48 h also significantly increased levels of ADAMTS-4 and ADAMTS-5 in SW1353 chondrocytes. However, treatment with 50 and 100 µM salicin rescued degradation of aggrecan in a dose-dependent manner, with the higher dose of salicin returning levels of aggrecan to near baseline.
Figure 6. Salicin treatment ameliorated AGEs-induced degradation of aggrecan in human SW1353 cells. Human SW1353 cells were treated with 100 μg/ml AGEs in the presence or absence of 50 and 100 μM salicin for 48 h. Expression of Aggrecan was determined by Western blot analysis (a, b, c, p < .01 vs. previous column group).
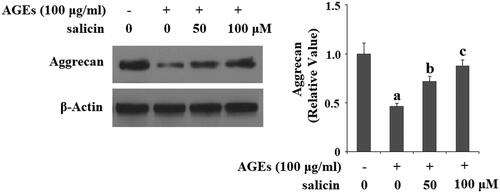
Figure 7. Salicin treatment ameliorated AGEs-induced expressions of ADAMTS-4 and ADAMTS-5 in human SW1353 cells. Human SW1353 cells were treated with 100 μg/ml AGEs in the presence or absence of 50 and 100 μM salicin for 48 h. (A). Expression of ADAMTS-4 and ADAMTS-5 at the gene levels was determined by real time PCR analysis; (B). Expression of ADAMTS-4 and ADAMTS-5 at the protein levels was determined by ELISA (a, b, c, p < .01 vs. previous column group).
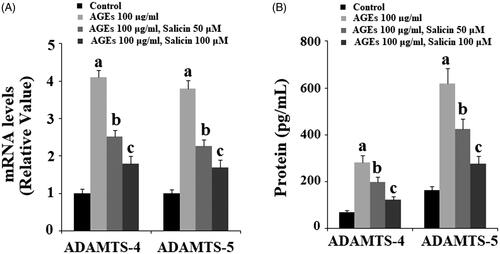
Salicin inhibits activation of the NF-κB proinflammatory pathway
Lastly, we set out to determine whether treatment of chondrocytes with salicin can prevent AGE-induced activation of the NF-κB proinflammatory signaling pathway. Briefly, SW1353 human chondrocytes were exposed to 100 ng/ml AGEs in the presence or absence of 50 and 100 µM salicin for 48 h, followed by an assessment of nuclear levels of p65 protein and NF-κB luciferase activity. As demonstrated by the results of Western blot analysis in , exposure to AGEs caused an approximate 3-fold increase in nuclear translocation of p65, the precursor of NF-κB activation, which was attenuated by treatment with salicin in a dose-dependent manner. Lamin B was used as a positive control in this experiment. Consistently, the results of NF-κB luciferase reporter assay show that exposure to AGEs increased activation of NF-κB by roughly 30-fold, which was significantly reduced by treatment with 50 and 100 µM salicin in a dose-dependent manner. These findings demonstrate the potent ability of salicin to inhibit activation of NF-κB signaling induced by AGEs.
Figure 8. Salicin treatment inhibits AGEs-induced activation of NF-κB in human SW1353 cells. Human SW1353 cells were treated with 100 μg/ml AGEs in the presence or absence of 50 and 100 μM salicin for 48 h. (A). Nuclear translocation of p65; Lamin B was used as a positive control; (B). Luciferase activity of NF-κB was determined (a, b, c, p < .01 vs. previous column group).
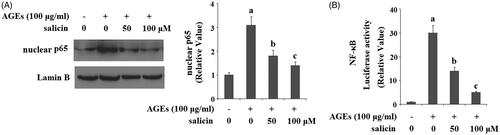
4. Discussion
Up to now, effective non-invasive therapies against the development and progression of OA have eluded researchers. However, by employing methods such as real-time PCR, ELISA and luciferase assay analyses, we can explore the potential of existing drugs as novel inhibitors of OA progression. In the present study, we employed these methods using SW1353 human chondrocytes exposed to insult from AGEs and determined the potential benefit of the widely used anti-inflammatory agent salicin. Our results indicate that salicin has a significant influence on AGE-induced factors of OA including oxidative stress, recruitment of immune cells, cartilage degradation, and activation of the NF-κB proinflammatory pathway.
Accumulation of AGEs triggers a significant increase in ROS and carbonylated protein (). Oxidative stress and overproduction of ROS disrupts the balance between pro-oxidants and antioxidants, which has been shown to exert a myriad of deleterious effects in OA, including senescence and apoptosis of chondrocytes, increased cartilage degradation, decreased matrix synthesis, synovial inflammation, and activation of proinflammatory signaling pathways, including the mitogen-activated protein kinase (MAPK), activator protein (AP)-1 and phosphatidylinositol-3-kinase (PI3K)/Akt pathways, and importantly, the nuclear factor (NF)-κB pathway [Citation22,Citation23]. Carbonylation of protein is an indicator of irreversible loss of protein function resulting from a high degree of oxidative damage [Citation24]. The results of our study indicate that salicin significantly reduced AGE-induced increased levels of ROS and carbonylated protein at both the mRNA and protein levels, thus demonstrating the potential value of salicin in down-regulating oxidative stress.
Expression of proinflammatory cytokines and chemokines is a major event in the pathogenesis of OA. We confirmed that exposure to AGEs significantly upregulated expression of IL-1β, TNF-α, MCP-1, and HMGB-1 in chondrocytes. Previous research has shown IL-1β and TNF-α to play important roles OA due to their ability to promote degradation of type II collagen and aggrecan through up-regulating expression of MMPs and ADAMTS and to perpetuate the inflammatory response by triggering expression of downstream cytokines and chemokines, such as MCP-1 [Citation25–27]. In addition, HMGB-1 has been shown to increase the levels of IL-1β and TNF-α in OA chondrocytes [Citation28,Citation29]. Importantly, our results show that 100 µM salicin significantly attenuates AGE-induced expression of Il-1β, TNF-α, MCP-1, and HMGB-1, returning the levels of all of these factors to near basal levels ( and ). This remarkable inhibition of proinflammatory cytokines and chemokines suggests that salicin may be applicable for the treatment of numerous inflammatory diseases including OA.
Degradation of the articular ECM is a hallmark of OA and is a major target of up and coming OA therapies. Here, we confirmed that exposure to AGEs significantly increased degradation of type II collagen and aggrecan by up-regulating the expression of MMP-1, MMP-3, MMP-13, ADAMTS-4, and ADAMTS-5. Decreasing expression of MMPs is an important target in OA as type II collagen has an extremely slow rate of synthesis, and therefore, excessive degradation of type II collagen is virtually irreversible [Citation30]. Our results indicate that treatment with salicin greatly reduced expression of MMP-1, MMP-3, and MMP-13, which was further evidenced by partial preservation of type II collagen by salicin even during an assault from AGEs ( and ). Furthermore, we demonstrate that salicin also significantly rescued aggrecan from degradation due to increased expression of ADAMTS-4 and ADAMTS-5 induced by AGEs ( and ). These findings indicate a novel role of salicin in preserving cartilage integrity by rescuing type II collagen and aggrecan from AGE-induced degradation.
Lastly, we investigated the effect of salicin on activation of the NF-κB proinflammatory signaling pathway in chondrocytes exposed to insult from AGEs. Activation of NF-κB has been widely explored in a plethora of diseases and has been shown to play a pivotal role in OA by acting as a master regulator of inflammation [Citation31]. Activation of NF-κB induces expression of a myriad of proinflammatory molecules that trigger the destruction of the joints and the onset of OA. Under normal conditions, NF-κB is sequestered in the cytoplasm by its inhibitor IκBα, but upon phosphorylation of IκBα triggered by the accumulation of AGEs, the p65 subunit translocates to the nucleus, where it triggers activation of NF-κB and a cascade of deleterious effects [Citation32]. In the present study, we measured the effects of salicin on nuclear accumulation of p65 protein and luciferase activity of NF-κB. Our results indicate significant activity of salicin against AGE-induced nuclear translocation of p65 and subsequent activation of NF-κB ().
Altogether, our findings indicate that salicin derived from Alangium chinense exerts powerful antioxidant, anti-inflammatory, and anti-degradative effects in AGE-induced OA chondrocytes. As a safe and long-trusted therapy, salicin treatment shows great potential as an effective strategy to slow cartilage degradation and combat inflammation in OA and other chronic inflammatory diseases. Further research using primary chondrocytes and animal models is necessary to better understand the unique mechanisms behind the beneficial effects of salicin.
Disclosure statement
The authors of this article declare that they have no conflicts of interest requiring disclosure.
References
- Zhang Y, Liu YB, Li Y, et al. Sesquiterpenes and alkaloids from the roots of Alangium chinense. J Nat Prod. 2013;76:1058–1063.
- Gong NB, Du LD, Lu Y. Anabasine. In Natural small molecule drugs from plants. Singapore: Springer; 2018. p. 353–356.
- Lin C, Cai J, Xu Y, et al. Effect of Alangium chinense on CYP450 isoforms activity of rats. Int J Clin Exp Med. 2016;9:3097–3103.
- Aboelsoud N. Herbal medicine in ancient Egypt. J Med Plants Res. 2010;4:82–86.
- Duan H, Zhai KF, Gao GZ, et al. Macroporous resin adsorption for purification of salicin from Alangium chinense (Lour.) Harms. Food Sci. 2012;33:99–102.
- Chen F, Mo K, Liu Z, et al. Ionic liquid-based vacuum microwave-assisted extraction followed by macroporous resin enrichment for the separation of the three glycosides salicin, hyperin and rutin from Populus bark. Molecules 2014; 19:9689–9711.
- Zhang Y, Liu YB, Li Y, et al. Terpenoids from the roots of Alangium chinense. J Asian Nat Prod Res. 2015;17:1025–1038.
- Zabihi NA, Mahmoudabady M, Soukhtanloo M, et al. Salix alba attenuated oxidative stress in the heart and kidney of hypercholesterolemic rabbits. Avicenna J Phytomed. 2018;8:63.
- Schmid B, Kötter I, Heide L. Pharmacokinetics of salicin after oral administration of a standardised willow bark extract. Eur J Clin Pharmacol. 2001;57:387–391.
- Klessig DF, Tian M, Choi HW. Multiple targets of salicylic acid and its derivatives in plants and animals. Front Immunol. 2016;7:206.
- Verzijl N, DeGroot J, Zaken CB, et al. Crosslinking by advanced glycation end products increases the stiffness of the collagen network in human articular cartilage: a possible mechanism through which age is a risk factor for osteoarthritis. Arthritis Rheum. 2002;46:114–123.
- Qu H, Li J, Wu L, et al. Trichostatin A increases the TIMP-1/MMP ratio to protect against osteoarthritis in an animal model of the disease. Mol Med Rep.2016;14:2423–2430.
- P. Garnero. Chapter 8 – biochemical markers of osteoarthritis. In Sharma L, Berenbaum F. Osteoarthritis. Mosby; 2007. p. 113–130.
- Nah SS, Choi IY, Yoo B, et al. Advanced glycation end products increases matrix metalloproteinase-1, -3, and -13, and TNF-alpha in human osteoarthritic chondrocytes. FEBS Lett. 2007;581:1928–1932.
- Verma P, Dalal K. ADAMTS-4 and ADAMTS-5: key enzymes in osteoarthritis. J Cell Biochem. 2011;112:3507–3514.
- Dalle-Donne I, Giustarini D, Colombo R, et al. Protein carbonylation in human diseases. Trends Mol Med. 2003;9:169–176.
- Liu X, Fang H, Zeng C, et al. Myricetin attenuates osteoarthritis by blockade of the IL-1β/MAPK pathway. Osteoarthritis Cartilage. 2018;26:S84.
- Xu YK, Ke Y, Wang B, et al. The role of MCP-1-CCR2 ligand-receptor axis in chondrocyte degradation and disease progress in knee osteoarthritis. Biol Res. 2015;48:64.
- Amin AR, Islam AB. Genomic analysis and differential expression of HMG and S100A family in human arthritis: upregulated expression of chemokines, IL-8 and nitric oxide by HMGB1. DNA Cell Biol. 2014;33:550–565.
- Yang HP, Lundbäck P, Ottoson L, et al. Redox modification of cysteine residues regulates the cytokine activity of high mobility group box-1 (HMGB1). Mol Med. 2012;18:250–259.
- Harris HE, Andersson U, Pisetsky DS. HMGB1: a multifunctional alarmin driving autoimmune and inflammatory disease. Nat Rev Rheumatol. 2012;8:195–202.
- Lepetsos P, Papavassiliou AG. ROS/oxidative stress signaling in osteoarthritis. Biochim Biophys Acta. 2016;1862:576–591.
- Ahmadinejad F, Geir Møller S, Hashemzadeh-Chaleshtori M, et al. Molecular mechanisms behind free radical scavengers function against oxidative stress. Antioxidants. 2017;6:51.
- Dalle-Donne I, Aldini G, Carini M, et al. Protein carbonylation, cellular dysfunction, and disease progression. J Cell Mol Med. 2006;10:389–406.
- Kapoor M, Martel-Pelletier J, Lajeunesse D, et al. Role of proinflammatory cytokines in the pathophysiology of osteoarthritis. Nat Rev Rheumatol. 2011;7:33.
- Wang J, Markova D, Anderson DG, et al. TNF-α and IL-1β promote a disintegrin-like and metalloprotease with thrombospondin type I motifs (ADAMTS)-5 mediated aggrecan degradation through syndecan-4 in intervertebral disc. J Biol Chem. 2011;286:39738–39749.
- Roman-Blas JA, Jimenez SA. NF-κB as a potential therapeutic target in osteoarthritis and rheumatoid arthritis. Osteoarthritis Cartilage. 2006;14:839–848.
- Yamada S, Maruyama I. HMGB1, a novel inflammatory cytokine. Clin Chim Acta. 2007;375:36–42.
- Pisetsky DS, Erlandsson-Harris H, Andersson U. High-mobility group box protein 1 (HMGB1): an alarmin mediating the pathogenesis of rheumatic disease. Arthritis Res Ther. 2008;10:209.
- Goldring MB, Otero M. Inflammation in osteoarthritis. Curr Opin Rheumatol. 2011;23:471–478.
- Roshak AK, Callahan JF, Blake SM. Small-molecule inhibitors of NF-κB for the treatment of inflammatory joint disease. Curr Opin Pharmacol. 2002;2:316–321.
- Rigoglou S, Papavassiliou AG. The NF-κB signalling pathway in osteoarthritis. Int J Biochem Cell Biol. 2013;45:2580–2584.