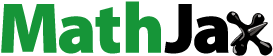
Abstract
Cancer therapies are aimed at eliminating the rapidly growing tumor cells by surgery and radiotherapy. The present therapies are only fruitful in early identified cases. The present study involves the preparation and characterization of eudragit S100-coated mini-capsules filled with chitosan nanoparticles-unconjugated and folic acid (FA)-conjugated encapsulating caspase 3 activator (7-hydroxystaurosporine). The formulated nanoparticles were compared for the cancer targeting and curing ability of the same by pre-investigation through drug release in organ-imitated fluids and ex vivo studies (cell viability, DNA fragmentation, caspase 3 activity), and then its confirmation through in vivo studies (tumor regression and distribution). The prepared nanoparticles were nearly spherical in shape, having positive zeta potential. From the cell line studies, it can be concluded that both the conjugated formulations showed better uptake, apoptosis, caspase 3 activation and DNA fragmentation. Stability study was performed according to ICH guidelines and formulation stored at 5° ± 3 °C was found to be most stable. The in vivo studies also supported the findings and showed better comprehensive residence time (23.61 ± 1.75 h), tumor distribution profile than UCN 01 alone. The results of in vitro, ex vivo and in vivo studies lead to the conclusion that the coated minicapsules specifically deliver the drug in the colon showing high therapeutic value and low side effects.
Introduction
Over the past two decades, many scientists have clinched the challenge of targeting active pharmaceutical ingredients (API) specifically to the colonic region. Scientific venture in this area has primarily been driven by the need to improve the treatment of pathologies of the colon ranging from diarrhoea, constipation, ulcers, colorectal cancer, etc. Cancer was known in relic, and these days it remains a worldwide escalating health predicament. Surgery in combination with chemotherapy and radiation are widely used. When the process of natural death occurs in proper schedule in cells, the unwanted ones which should be excreted remain and subsequently grow as tumour [Citation1]. Cancer cells grow at a much faster pace than the normal cells. The chemotherapy targets this property and radiation helps in killing these specific cells. The advances in the treatment of cancer are progressing quickly both in terms of new drugs and new methods of delivering the new API [Citation2].
Death is a predictable fact of life for all organisms. The homeostasis of tissues remains until the cells keep on renewing itself through the balance between apoptosis and cell growth. Many efforts have been put forward to explicate the process of cell termination at the molecular level. But, still the recognition and amputation of apoptotic bodies have gained less attention. Moreover, apoptotic cells create self-antigens leading to autoimmune responses in the body. Thus, the thought of tissue balance depends not only on the mitosis and apoptosis equilibrium, but also on the apotosis rate in relation to the clearance of dead cells from the body [Citation3]. Imbalance in enzymes (caspases) may lead to one of the deadly disease, cancer. Carcinogenesis mainly occurs due to the gene mutation. This mutation leads to disturbance in apoptotic signals of cells causing cancer development [Citation4]. But, the same can be beneficial in cancer treatment and management. Caspase-3 belongs to the family of cysteine proteases. Caspases, a proteases regulates apoptotic features like DNA fragmentation and formation of apoptotic bodies [Citation5]. The carcinoma of the colorectal region has been associated with depletion of apoptotic signals [Citation6], indicating a major functionality in tumorigenesis. The hunt for new therapeutic means for the management of colon cancers at last stages have led to the development of intentionized carriers. Also, this helps in elucidating the molecular mechanisms related to the progress of cancer.
To promote selectivity and intended association with the target (tumour), which are anatomically accessible, the carrier must be able to recognize the cell (target); should bind to them and if required, penetrate into their interior. Similarly, surface modification of the carriers with particular ligands like antibodies, peptides and sugars, which can bind to the specific receptors at the target site, could impart a specific targeting potential to the carrier. These include ligands like antibodies, glycolipids, glycoproteins, polysaccharides, proteins and immune regulatory molecules. Among these folate receptors (which recognizes folic acid as ligand) are the ones which are largely found in almost all types of cancer [Citation2].
Herein, eudragit-coated minicapsules filled with 7 hydroxy staurosporine (UCN 01), entrapped chitosan nanoparticles were prepared with the aim to protect UCN 01 from degrading in the environment of stomach and also releases it specifically in the colonic region for tumour targeting, wherein the nanoparticles being mucoadhesive will attach to the mucosa and release the drug. The present work deals with surmounting the constraint of a highly persuasive anticancer drug which could be easily used if it is victoriously targeted.
Experimental section
Materials
Alomone labs, Israel, gifted UCN 01 for research work. Acridine orange chitosan, ethidium bromide and folic acid were purchased from Sigma Aldrich. Sodium tri-polyphosphate, sorbitan monooleate, 1-ethyl-3-(3-dimethylaminopro-pyl)carbodiimide (EDC), anhydrous dimethylsulfoxide, Triton X 100, l-tris-hydrochloride, PEG 4000, RNase A (2-(3-aminopropanoylamino)-3-(3H-imidazol-4-yl) propanoic acid), agarose TBE were obtained from HiMediaPvt. Ltd. Mumbai, India. Eudragit S100was purchased from Evonik Roehm Pharma polymers. The water used in all experimental procedures was triple-distilled water obtained from MilliQ Plus, USA. All the materials were of AR grade. No purification was done before usage.
Conjugation of FA with chitosan. FA was conjugated with chitosan using the previously reported method [Citation7] using 1-ethyl‐3‐(3‐dimethylamino)propyl carbodiimide, hydrochloride (EDC) and anhydrous dimethylsulfoxide (DMSO) (molar ratio was 1:1) as conjugating agents. The final precipitate was purified by intermittent dialysis for 3 days against phosphate-buffered saline (pH 7.4) and for 4 days against water. Final conjugate was lyophilized and stored in nitrogen-flushed vials for further studies.
Preparation of chitosan and FA-conjugated nanoparticles. Chitosan (CH) nanoparticles were prepared by ionotropic gelation of CH with sodium tripolyphosphate (TPP). The method is reported by Khatik et al. [Citation8]. Briefly, TPP aqueous solution was added to the CH solution (in 1% v/v acetic acid) kept in a beaker. The solution was stirred (400 rpm) at room temperature for 30 min. The UCN 01 solution (1 mg/mL) was added into the CH solution in order to get drug-loaded chitosan nanoparticles. The nanoparticles were formed spontaneously upon the addition of an aqueous TPP solution to chitosan solution under mechanical stirring. The prepared CH NP was separated by centrifugation (Remi, Mumbai) at 16,000g for 30 min at 4°C to remove the free unentrapped drug. The supernatant of dispersion was discarded, NPs were washed with 10% methanol, lyophilized (HetoDrywinner) and preserved for further studies. FA-conjugated chitosan nanoparticles were prepared according to the same method using FA-conjugated chitosan (FA-CH) in place of chitosan.
Preparation of mini-capsules
Preparation of Eudragit solution. 5 g of Eudragit S100 and 1.25 g of PEG 4000 were dissolved by continuous stirring in a mixture of 86.25 g ethanol (96%) and distilled water (7.5 g).
Preparation of nanoparticle-filled enteric-coated minicapsules. PC caps mini-hard gelatin capsules #9 obtained from Capsule having a width of cap 5.02 mm × 15.0 mm were utilized to prepare required capsules of 9.5 ± 0.5 mm length. A small funnel was used for filling the capsules with lyophilized nanoparticles [Citation9].
Enteric coating of mini capsules. Six successive coatings were done to obtain the properly coated capsules. The formulation formed was coded as E-U-CH NP and E-U-FA-CH NP.
Physicochemical characterization. Deionized water at 1:9 w/v was used to dilute the NPs and measured for size and potential by Zetasizer (DTS Ver. 4.10, Malvern Instruments, Malvern, England). The shape of the nanoparticles was viewed using a transmission electron microscope (TEM) (Morgagni 268, FEI electron microscope, the Netherlands). The routine staining process was followed by utilising aqueous phosphotungstic acid [Citation10]. The FTIR spectrum of chitosan, FA-CH and FA were recorded by FTIR Spectrophotometer (Perkin Elmer, Beaconsfield, United Kingdom) equipped with spectrum v3.02 software to confirm the conjugation.
Ex-vivo studies. Maintenance of colon cancer Caco 2 cell line: Dulbecco’s Modified Eagle’s (DME) growth medium with add-on 100 µg/mL Streptomycin heat-inactivated fetal bovine serum (FBS, 10%), 100 U/mL penicillin and l-glutamine (2 mM) at 37 °C in 5% CO2 environment were used to maintain the cells. 1 × 105 cells/well were seeded for 24 h before the experiment.
Colorimetric caspase3 assay. The caspase 3 activity was done using the ELISA microplate reader at a wavelength of 405 nm following the procedure described earlier [Citation10,Citation11].
DNA cell cycle analysis. Flow cytometer (FACH, Becton-Dickinson), with pre-installed software “Cell Quest” was used for the experiment. The fixing and staining of cells was done by ethanol (75%) and propidium iodide (40 µg/mL), respectively, the method reported earlier were referred for the study [Citation8,Citation10,Citation11].
Cytotoxicity assay. In vitro cytotoxicity of UCN 01, CH NP, U-CH NP and U-FA-CH NP were analyzed by (3-(4,5-dimethylthiazol-2-yl)-2,5-diphenyl tetrazolium bromide) MTT assay [Citation12]. For the comparison normal colon cell lines, NCM 460 were used. Cell cytotoxicity was determined by staining the viable cells using MTT (0.5 mg/mL). The effect of treatment was observed after 3 h. The optical density values were determined at 540 nm. The data have been reported as a mean of three experiments [Citation13].
In vivo studies. The in vivo studies were done on C57BL Balb/c mice 5–6 weeks/80–100 g of either sex after getting approval from the Institutional Animal Ethical Committee (379/01/ab/CPCSEA) of Dr. H. S. Gour University, Sagar, India. Permission No. 19: Animal Eth. Comm./08/387 [Citation1]. Food and water were allowed ad libitum.
Tumor induction and biodistribution study. Caco 2 cancer lines were maintained by serial transplantation in C57BL/6 mice. Tumor induction was carried out according to the method [Citation7] with slight modifications. Briefly, 2 × 106 trypsinized cells/mouse were injected into the mucosa of the ascending colon of anesthetized mice. The tumor was allowed to grow to a mean volume of 100 mm3. These tumor-bearing mice were used for further propagation of tumor.
Tumor distribution profile. The amount of UCN 01 in tumor was determined by the HPLC method reported in the previous section. The pharmacokinetic analysis was performed on the raw data expressing concentration of drug in tumor vs time profile for all the administered formulations and the parameters such as area under the curve (AUC), half-life (t1/2), mean residence time (MRT) were calculated using Kinetic version 5.0. The results of organ distribution studies show that very low concentration of drug is reaching colon; therefore, the uncoated formulation was not analyzed for tumor distribution profile.
Tumor regression study. C57BL/6 tumor-bearing mice having 100 mm3 size of tumor and distributed in 4 × 6 groups were used to analyze the regression in tumor growth. The first/control group was given 0.2 ml (0.9% w/v) normal saline solution. The 2nd group was given UCN 01^ (The symbol ^ is used to differentiate animal group names (in vivo) in mini- capsules from those of ex vivo cell line) , 3rd group E-U-CH NP and 4th group E-U-FA-CH NP. The antitumor activity was evaluated at dose of 0.35 mg UCN 01/kg body weight for 5 days. The uncoated formulations were not examined for tumor regression analysis due to limitation in permission from institutional animal ethical committee. Vernier calliper with digital display was used to measure the tumor volume and converted into percentage. The length and width obtained were fitted in the following formula:
where L is the longest diameter of the tumor (length) and W is the diameter perpendicular to the width [Citation14]. The survival probability of treated tumor-bearing mice was calculated by Kaplan–Meir Survival Analysis using MedCalc software. The log-rank test applied in the study resulted in p < .0001.
Preparation of tissue homogenates for biodistribution studies. The mice were sacrificed at 2, 4, 6 and 8 h after dosing for 5 days as given in the previous section, and the organs were collected. The adhering and unwanted tissue, fatty acid were removed. The organs were washed with 0.9% w/v NaCl. For HPLC analysis, the tissues were chopped and diluted with HPLC grade water, homogenized maintaining the temperature at 4 °C, centrifuged (Z36HK, HERMLE LaborTchnik GmbH, Germany) at 1000 × g for 10 min and the supernatant was separated. The deproteinization was done with 0.5 ml acetonitrile and homogenates. Finally, the sample was centrifuged for 10 min. At 1200 x g. The supernatant obtained was passed through 0.22 µm membrane filter to get a clear plasma. [Citation15].
Histopathological studies. The distribution of nanoparticles in various organs was performed by histopathological studies. Animals were killed 10 h after the administration of the formulations and the stomach, colon and small intestine were removed. The organs were kept in a fixative solution containing 10% formalin (10% formaldehyde in water) for tissue stabilization to prevent tissue decay until dispensation. Before processing, the tissues were cut into pieces, blotted and wiped with the tissue paper followed by successive dehydration through a graded series of alcohols (70%, 80%, 95%, and 100%), and finally with absolute alcohol:Toluene (1:1) and kept for 15 min. The tissue samples were then immersed for 30 min in molten wax scrapings and allowed to set for 24 h. Thin sections of 3–5 μm width were obtained by microtome using egg albumin as fixative and stained with hematoxylin/eosin for microscopic examination (Fluorescence Microscope, Leica, DMRBE, Herbrugg, Switzerland) [Citation16].
Statistical analysis
The data were analyzed statistically using Graph Pad In Stat 3.0 (Version 3, Graph Pad Software, San Diego, California, USA). All the experiment were done in triplicate. The values given are mean of these, p values of <.05 was considered significant unless otherwise indicated.
Result
Preparation and physicochemical characterization of NPs
The mean nanoparticle size of prepared U-CH NP, U-FA CH NP were found to be 156 ± 5.3 nm and 240 ± 3.5 nm, respectively, and average zeta potential of + 24.3 ± 1.4 mV and + 16.3 ± 2.2 mV, respectively.
The efficiency of drug entrapment was found to be 54.2 ± 1.2% and 62.3 ± 2.8% for U-CH NP, U-FA CH NP, respectively. The data have been reported in . The well-known property of CH NPsof being positively charged was seen. The zeta potential of all formulations (conjugated and unconjugated) were found to be nearly +20 mV. Both the developed formulations, i.e. U-CH NP and U-FA-CH NP were also characterized for their surface morphology, shape and particle size by transmission electron microscopy (TEM) and atomic force microscopy (AFM). TEM photographs shown in reveal that both the formulations were nearly spherical in shape with the denser regions appearing darker due to more electrons scattering. AFM images were also taken to provide substantiation of the spherical structure alleged by the TEM analyses.
Figure 1. Transmission electron microscopy of U-CH NP (A), (B) U-FA-CH NP, (C) atomic force microscopy of U-CH NP and (D) U-FA-CH NP.
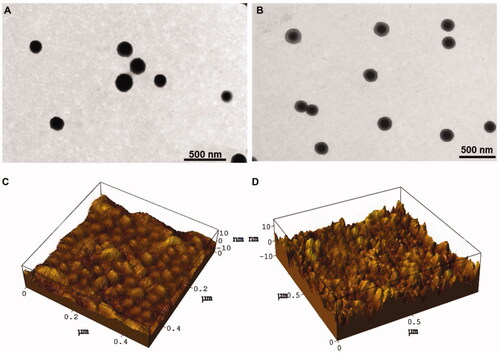
Table1. Particle size, zeta potential and entrapment efficiency (EE %) of U-CH-NP and U-FA-CH-NP.
3D AFM images of U-CH NP and U-FA-CH NPwere taken in a semi-contact mode. AFM topographical images of both the formulations showed non-aggregated, convex meniscus formed, evenly scattered particles (). The particle size of U-CH NP and U-FA-CH NP revealed by 3D AFM were 156 nm and 240 nm, respectively. Also, in semi-contact mode both the formulations exhibited spherical and regular morphology.
Ex vivo study
Colorimetric caspase3 assay
The caspase 3 activity (% of control) measured for all formulations and free drug is represented in . Significant increase as compared to control was seen in the case of caspase 3 activity of UCN 01, U-CH NP (p < .01), indicating an effective capsase 3 activation in cells. However, the caspase 3 activity in case of folate-coupled nanoparticles was much greater as compared to the control group (p < .001) due to greater uptake of folate-conjugated nanoparticles from the folate receptors reported to be over-expressed on Caco 2 cells surface. Propidium Iodide was used to detect apoptosis and the effect of formulations on the cell cycle. The DNA histogram () gives a static picture of the percentage of cells in different phases of the cell cycle. The percentage of cells undergoing apoptosis was also determined. Percentage of apoptotic cells was raised from 3.81% to 6.29%. On the contrary, in case of cells treated with free UCN 01, there is 78.49% increase in apoptosis, i.e. from 16.51% to 95.0%. Analyses of the data obtained in case of cells treated with U-CH NP and U-FA-CH NP () showed that the percentage of cells undergoing apoptosis is increased from 23.17% to 31.75% for U-CH NP and from 19.98% to 55.4% for U-FA-CH NP. Overall, the ex vivo studies reveal that U-CH NP and U-FA-CH NP are safe formulations which can be used for the enhanced delivery of chemotherapeutic agents to colon cancer cells. The therapeutic efficacy of the conjugated and unconjugated chitosan nanoparticles was assessed by the MTT method using Caco-2 cells. Surviving cells were assayed for the percentage of cell growth inhibition after 24 h incubation period. The IC50 values of free UCN 01 and its chitosan nanoparticles were estimated from the percentage cell viability at different concentrations. The percentage cell viability of free UCN 01 and various other formulations is shown in It shows that the free drug as well as encapsulated drug in various formulations have toxicity against the Caco-2 cells in a dose-dependent manner. The ligand-conjugated nanoaprticles showed significantly higher toxicity, i.e. lower viability (p < .01) effect than the unconjugated nanoparticle formulations. The IC50 values are shown in .
Figure 2. (A) Caspase 3 colorimetric assay. Significant differences were observed as compared to control (*p < .01), (B) percentage of cells in various phases of cell cycle 2 h and 12 h post treatment, (C) DNA Cell cycle analysis, Cells (in percentage) at G1, S, G2 phases of cell cycle and apoptosis at 2h and 12 h post-treatment, and (D) percentage cell viability of different formulations in Caco-2 cells.
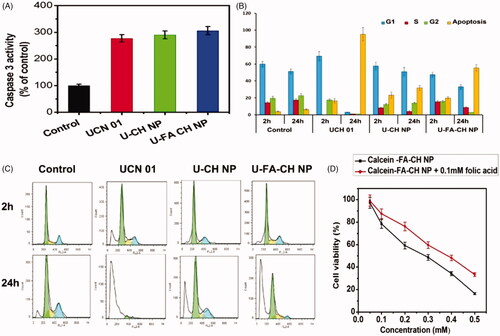
Table 2. IC50 Values of different formulation on Caco-2 cells.
In vivo study
In vivo studies were done on colon cancer-bearing C57BL/6 mice (30–35 g). The drug concentration in different parts of GIT was determined at 2 h, 4 h, 6 h and 8 h post administration of UCN 01^, U-CH NP^, U-FA-CH NP^, E-U-CH NP and E-U-FA-CH NP (. The results indicate that among all the formulations administered, 42.1 ± 3.4% of the drug was recovered from UCN 01^ after oral administration from stomach at 2 h, the percentage decreased in subsequent hours. 31.6 ± 3.2% of the drug was recovered at 4 h, 14.5 ± 1.5% at 6 h and 8.2 ± 0.6% at 8 h. However, in case of U-CH NP^ and U-FA-CH NP^ the percentage of drug recovered were increased from 13.2 ± 1.3% to 38.3 ± 1.3% and from 12.8 ± 1.1% to 34.3 ± 3.1% till 6 h, which then decreased to 4.4 ± 0.5% and 5.8 ± 0.8% till 8 h, respectively. No drug was detected in small intestine even after 4 h from eudragit S100-coated mini-capsules. 1.6 ± 0.8% drug release was observed from E-U-CH NP and 1.7 ± 0.06% from E-U-FA-CH NP in the small intestine after 6 h. The drug recovered from the formulations UCN 01^, U-CH NP^ and U-FA-CH NP^ increased from 28.3 ± 0.6%, 13.7 ± 1.2% and 18.2 ± 1.4% at 6 h to 34.5 ± 1.5%, 41.5 ± 2.1% and 44.2 ± 2.4% further in the colonic region at 8 h, respectively. The eudragit S100-coated capsules filled with nanoparticles showed almost no drug in upper GIT (till 2–4 h). Maximum drug was recovered after 8 h in colon, i.e. 66.5 ± 2.3% and 74.4 ± 5.2% from coated unconjugated and coated conjugated dosage forms.
Figure 3. In Vivo distribution profiles of various formulations at different time intervals. (A) Tumor drug concentrations after oral administration of different formulations, (B) effect of free UCN 01 and various nanoparticle formulations on tumor growth in mice as compared to control, (C) Kaplan–Meir survival plot, (D) histopathology of stomach, small intestine and colon 10 h after formulation administration and (E) stability assessment of U-CH NP and U-FA-CH NP.
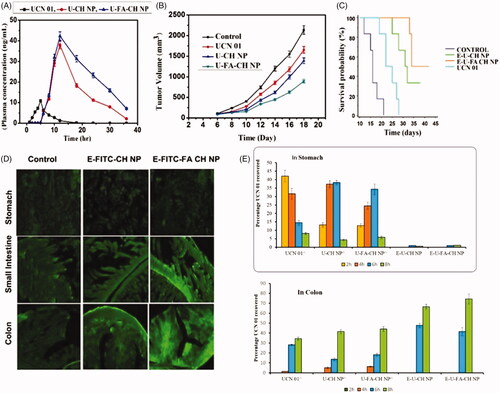
Tumor concentration profile of free UCN 01 and various formulations are given in and shown in . The concentration of UCN 01 in tumor in case of E-U-FA-CH NP (t½: 9.81 ± 2.01) was much higher (p < .01) than UCN 01^ and E-U-CH NP. Sustained distribution is considered as an imperative inevitability for therapeutic use of a targeted system [Citation17]. Eudragit coating of capsules (E-U-CH NP and E-U-FA CH NP) significantly enhance the half-life, mean residence time (MRT; 18.48 ± 1.24 h and 23.61 ± 1.75 h) and area under the curve (AUC; 484.81 ± 8.22 µg h/mL and 823.68 ± 6.32 µg h/mL) of UCN 01, respectively in tumor as compared to UCN 01^ (t1/2 1.97 ± 0.25h; MRT 6.46 ± 0.03h and AUC 53.39 ± 7.60 µg h/mL) (). The results of pharmacokinetic studies clearly reveal that E-U-CH NP and E-U-FA-CH NP both have prolonged residence time in tumor.
Table 3. Tumor distribution profile.
The antitumor activity of UCN01^, E-U-CH NP and E-U-FA-CH NP were evaluated in cancer-bearing mice at a dose of 0.35 mg UCN 01/kg body weight for 5 days. Both the coated formulations delayed the growth of the tumor as compared to the control group. FA-conjugated nanoparticles were observed to have higher tumor inhibition ability than the unconjugated one. This higher cancer curing worthiness of conjugated nanoparticles is consistent with the higher accumulation and MRT as compared to other groups (). Therefore, it can be said that the higher UCN 01 levels of E-U-FA-CH NP in tumor would result in higher therapeutic potency. The Kaplan–Meier survival plot was also studied (). E-U-FA-CH NP and E-U-CH NP exhibited higher survival rates than the untreated group (0.9% w/v normal saline) with median survival rates of 42 and 38 days, respectively, compared to 21 days (p < .05 and p < .01). A slight variation was observed between UCN 01^ and control group.
Histopathology studies
Ten hours postoral administration of FITC-loaded formulation sections of stomach, small intestine and colon were examined histopathologically. The control showed very less fluorescence (). The photomicrographs clearly show that the order of fluorescence in different organs from FITC-loaded folic acid-conjugated chitosan nanoparticles encapsulated in mini-capsules (E-FITC-FA CH NP) is colon > small intestine > stomach. Similar results are obtained for E-FITC-FA CH NP.
Discussion
Conjugation of CH with FA was confirmed by FTIR in Figure S1. The NPs were formed as a result of ionic gelation method. The formation of nanoparticles has been based on the interaction between the negative groups of the TPP and the positively charged amino group of the chitosan. In addition to simplicity, mild preparation conditions and small size nanoparticles, this technique also offers reversible physical cross-linking by electrostatic interaction, instead of chemical cross-linking, thereby avoiding the possible toxicity of reagent and other undesirable effects [Citation12].
The results of drug–polymer interaction by X-ray diffraction were in agreement with those reported by Trickler et al. [Citation18]. They reported that the powder X-ray diffraction pattern for the drug-loaded nanoparticles was not remarkably different from that of the powder X-ray pattern for blank nanoparticles, as shown in Figure S2. According to them, the lack of any remarkable diffraction pattern for the nanoparticles and blank nanoparticles were very similar, signifying the drug in the nanoparticles existed in non-crystalline state. The measurement of zeta potential is a useful method for assessing the stability of nanoparticles and the extent of CH-NP, and to check whether the drug is truly encapsulated within the NPs or simply adsorbed onto the surface. Zeta potential values of blank NPs and U-CH NP revealed that the surface of NPs is positively charged, the reason being residual amino group left after neutralized by TPP molecule. Also, Wang et al. [Citation19] proposed that the stretched amino groups obstruct the adsorption of anion, preventing the aggregation and rendering positive charge to particles. TEM and AFM images reveal that morphologically, U-CH NP seems to be round to oval in shape and have relatively smooth surface than U-FA CH NP.
The accelerated storage stability of U-CH NP, U-FA-CH NP was assessed by storing the formulations at various temperatures and humidity conditions. It is extremely important to predict both the physical and chemical stability of formulation/new product from accelerated storage data because of the considerable economic advantage gained in the market or clinical trials of a new product as soon as it is formulated. The storage stability of prepared formulations was accessed in two different ways. Firstly, the stability of enteric coated mini-capsules entrapping U-CH NP and U-FA CH NP were checked by determining the disintegration time in simulated fluids and then the in vitro release parameters. Secondly, the change in vesicular size and EE% were examined. Disintegration test is often used to study the time release of formulation placed in simulated body fluids. One of the extensively used techniques for controlled-release oral formulation, especially for colonic delayed release formulations is checking the integrity of enteric coating. The effect of different storage conditions on particle size and EE% for U-CH NP and U-FA CH NP are shown in Figure S4. The increase in the size of nanoparticles and the decrease in EE % might be due to the increasing tendency of particles to get aggregated (to reduce entropy) causing drug leakage. Thus, from the observations it can be concluded that all the formulations stored at 5° ± 3 °C are more stable. The refrigerated conditions for the storage of the prepared drug delivery system are explicitly recommended.
The pH variations faced by a colon-targeted delivery system in the body were impersonated while performing cumulative percentage release of UCN 01 from mini capsules. The same rat caecal content at 2% concentration indicating microbial degradation in large intestine was used for the in vitro drug release study. Greater drug release was noted when 2% rat ceacal content was present in the dissolution medium. This could be credited to the various anerobic bacteria (B. fragillis, B. ovatus, B. Variabilis, B. uniformis, B. distasonis and B. thetaiotaomicron) producing a vast number of enzymes like glucoronidase, xylosidase, arabinosidase, galactosidase and mannose present in caecal contents, out of which galactosidase may be responsible for the digestion of nanoparticles in the colon in order to release the drug [Citation20]. The results of the present study also prove that the UCN 01 was protected completely from the acid environment of stomach and enzymes in gastric juice through eudragit S100 coating. Eudragit polymer contains carboxyl groups that ionize from neutral to alkaline media. As the ionization takes place, the integrity of the film is disturbed and releases the drug. At pH 7.4 (small intestine), the membrane coating gets dissolved.
For cell uptake studies, calcein-loaded nanoparticles (unconjugated and conjugated) were prepared. Caco-2 cells were treated with Calcein-CH NP and Calcein-FA-CH NP for different time periods. The cell uptake was found to increases gradually with the increase in incubation time. However, charge-mediated endocytosis might be the reason for non-targeted uptake of the Calcein-CH NP and receptor-mediated for folate-conjugated particles. Thus, more calcein was accumulated in the cells exposed to Calcein-FA-CH NP than those treated with Calcein-CH NP. Caco-2 cells incubated with free calcein and Calcein-FA CH NP were analyzed for uptake through fluorescence micrographs taken at 2, 12, and 24 h (. A significant amount of cellular internalization seen as strong green fluorescence was visible only after 12 h of incubation for conjugated nanoparticles (Figure S5 12h) and at 24 h with free Calcein. Initially, nanoparticles attach to the membrane of cells containing over-expressed folate receptors and then the drug is released after its internalization [Citation21,Citation22]. DNA fragmentation study has been extensively used as an idiosyncratic sign of the apoptosis process, where fragmented DNA as ladder signifies apoptosis and continuous spectrum represents necrosis on samples of DNA when run on agarose gel [Citation23]. The results showed similarities with the present observation (Figure S6). Caspase 3 was the molecule of choice due to its essentiality as a convergence point for different signalling pathways in cell death. The caspase 3 commencement efficiency of UCN01-loaded nanoparticles (conjugated and unconjugated) in comparison with the free drug was done. The study of cell growth, differentiation and death is called as the cell cycle (). Cancer growth and metastasis leads to disturbance in this cycle [Citation19], and thus, restraining of the cell cycle can be a good target for tumor therapy [Citation23].
Based on different cell types and p53 level, UCN-01 has been reported to induce arrest in G2 M or G0 G1 phases of cell cycle [Citation24,Citation25]. UCN 01 is a potent modulator of the cyclin-dependent kinase system. The G1 phase arrest in human colon, pancreatic and blood cancer cell lines have been reported for UCN-01 [Citation26]. The results of our studies revealed that the UCN 01, U-CH NP and U-FA CH NP induced cell cycle block at G0/G1 phase in Caco-2 cells after the treatment of various formulations till 24 h in . This might possibly be due to the inhibition of protein kinase C leading to accumulation of dephosphorylated retinoblastoma protein.
The cytotoxicity and cell uptake studies were done to check the safety and efficacy of the developed formulations. The preliminary in vitro cytotoxicity study was done by MTT colorimetric assay. The free UCN 01 was found to be more cytotoxic as compared to both the formulations. The higher effectiveness of bare drug compared to formulations could be due to the difference in uptake of formulations. Free drug can simply diffuse across the plasma membrane of the cells whereas the macromolecular carriers are taken up by endocytosis, which is a more gradual process [Citation27]. The blank nanoparticles (without drug) showed no significant cytotoxicity in Caco-2 cells. It implies that the formulation constituents are non-toxic and that the cytotoxicity observed previously was due to UCN 01 encapsulation and release in cells. Therefore, it could be concluded that these formulations could be used as a drug carrier without any significant cytotoxic effect.
The hemolytic toxicity of all formulations was also determined. The data have been represented in (Table S4). Both formulations were less toxic than drug, possibly due to drug encapsulation in a bioattuned natural polymeric (chitosan) environment. Decreased hemolysis caused by FA conjugation to the CH might probably be due to reserving of free amine groups present on CH NP. It is clear from the hemolysis study that the encapsulation of drug in nanoparticles reduces the erythrocytes toxicity [Citation10].
Conventional dosage forms are administered with the aim of achieving systemic absorption for therapeutic action. However, some pathophysiological conditions require stagnation of released drug in the GI environment rather than systemic absorption. An example of such a system is colon-specific drug delivery, where delivery system needs to travel intact through the upper GIT and at the same time exhibit its therapeutic action on reaching the colon. The specificity of formulation is difficult to predict in vivo. In order to determine the fate of UCN 01-loaded nanoparticles in vivo, its biodistribution to various organs determined at 2 h, 4 h, 6 h and 8 h post administration was investigated (). The results indicate that among all the formulations administered, maximum percentage of drug from UCN 01^ after oral administration (42.1 ± 3.4%) was observed in the stomach at 2 h and then in subsequent hours; less amount of drug, i.e. 31.6 ± 3.2% at 4 h, 14.5 ± 1.5% at 6 h and 8.2 ± 0.6% at 8 h were recovered. However, in case of U-CH NP^ and U-FA-CH NP^, the percentage of drug recovered were increased from 13.2 ± 1.3% to 38.3 ± 1.3% and from 12.8 ± 1.1% to 34.3 ± 3.1% till 6 h which then decreased to 4.4 ± 0.5% and 5.8 ± 0.8% till 8 h, respectively. This might be due to the opening of uncoated mini-capsules and burst release of free drug from UCN 01^, and leaching of high amount of drug from U-CH NP^ and U-FA-CH NP^ due to shrinkage of CH nanoparticles in the acidic medium of stomach. On contrary, from E-U-CH NP and E-U-FA-CH NP no drug was recovered till 4 h and nearly 1% drug was found at 6 h and 8 h, the reason for hindered release may be the additional enteric coating of Eudragit S100, which does not allow the opening of mini-capsules in stomach till 2 h.
The drug released from the formulations UCN 01^, U-CH NP^ and U-FA-CH NP^ increased from 28.3 ± 0.6%, 13.7 ± 1.2% and 18.2 ± 1.4% at 6 h to 34.5 ± 1.5%, 41.5 ± 2.1% and 44.2 ± 2.4% further in the colonic region at 8 h, respectively. Greater amount was recovered in intestine and colonic region due to the presence of microbial population in small intestine which facilitates the release of drug. The release pattern of UCN 01 from eudragit-coated capsules can be understood by the protective nature of eudragit coating delaying/minimizing drug release in upper GIT and releasing drug only after reaching colonic region.
During chemotherapy, different pathological changes have been seen in various organs and hematological functions. Therefore, the formulations were further evaluated for their performance on animals for toxicity studies, including hematological, genotoxicity and biochemical aspects. The potential of drug delivery systems in reducing the toxicity of the anticancer drug (i.e. UCN 01) was assessed by hematological and toxicology assays. Since the drug UCN 01 is still under clinical trials, it is recommended that blood analysis should be performed on all patients receiving UCN 01. Significant (p < .05) decrease in blood cells for all the three parameters tested was noted in the case of UCN 01^ administration (Table S5 and S6). This might be due to the fast absorption of UCN 01 in upper GIT causing anemia and thrombocytopenia. In case of the administration of UCN 01-loaded eudragit-coated mini-capsules, no significant change was noted in all the parameters, which might be due to the sustained and targeted release of UCN 01.
Inflammation contributes to the pathogenesis and development of colorectal cancer (CRC). Cytokines levels have been reported to change during CRC [Citation28]. Cytokines are involved in amazingly broad array of biological activities including cancer, innate immunity, adaptive immunity, inflammation, and haematopoiesis. They can modify cancer development by intervening with cancer cells and macrophages. Interleukin (IL-12, IL-15) and interferon ɣ (IFN-γ) that contribute to cellular immune reactions are considered essential for an effective response against tumor cells, whereas tumor suppression might occur due to cytokines, e.g. IL-4, IL-6, IL-10 and IL-13 [Citation29]. In CRC, IL-6 has been reported to increase the propagation and capturing ability of cancer cells, promoting angiogenesis sometimes associated with pyrogenic effect [Citation30,Citation31]. The data have been shown in Table S7. Histopathology studies were used for the qualitative determination of polymer matrix in different parts of GIT. However, greater relative fluorescence was observed for E-FITC-FA CH NP than E-FITC CH NP. The absence of any fluorescence at the apical part of the stomach is expectedly due to eudragit coating. show the uptake of E-FITC-CH NP and E-FITC-FA CH NP in the sections of intestine. Furthermore, in sections of colon, fluorescence is visible in villi representing conjugated nanoparticles inside vascular structures. This reveals enhanced uptake in the colonic sections. Overall, it can be concluded that eudragit-coated FA-CH-conjugated nanoparticles specifically deliver the maximum amount of drug in the colon, which is beneficial for the targeting of colorectal cancer with high therapeutic value and low side effects.
Revised_Supporting.docx
Download ()Acknowledgements
One of the authors Dr. Aakanchha Jain is thankful to the Department of Science and Technology, India, for INSPIRE fellowship to pursue Ph.D. The authors are thankful to AIIMS, New Delhi, for Transmission Electron Microscopy and IIT, Indore, for Atomic Force Microscopy facility, Inter University Consortium, Indore, for X-ray Diffraction facility.
Disclosure statement
No potential conflict of interest was reported by the authors.
References
- Prabhudesai SG, Rekhraj S, Roberts G, et al. Apoptosis and chemo-resistance in colorectal cancer. J Surg Oncol. 2007;96:77–88.
- Brannon-Peppas L, Blanchette JO. Nanoparticle and targeted systems for cancer therapy. Adv Drug Deliv Rev. 2004;56:1649–1659.
- Fadeel B, Xue D, Kagan V. Programmed cell clearance: molecular regulation of the elimination of apoptotic cell corpses and its role in the resolution of inflammation. Biochem Biophys Res Commun. 2010;396:7–10.
- Wong RSY. Apoptosis in cancer: from pathogenesis to treatment. J Exp Clin Cancer Res. 2011;30:87.
- Vermeulen K, Van Bockstaele DR, Berneman ZN. Apoptosis: mechanisms and relevance in cancer. Ann Hematol. 2005;84:627–639.
- Hanahan D, Weinberg RA. Hallmarks of cancer: the next generation. Cell. 2011;144:646–674.
- Khatik R, Dwivedi P, Junnuthula VR, et al. Potential in vitro and in vivo colon specific anticancer activity in a HCT-116 xenograft nude mice model: targeted delivery using enteric coated folate modified nanoparticles. RSC Adv. 2015;5:16507–16520.
- Khatik R, Mishra R, Verma A, et al. Colon-specific delivery of curcumin by exploiting Eudragit-decorated chitosan nanoparticles in vitro and in vivo. J Nanoparticle Res. 2013;15:1893.
- Saphier S, Rosner A, Brandeis R, et al. Gastro-intestinal tracking and gastric emptying of solid dosage forms in rats using X-ray imaging. Int J Pharma. 2010;388:190–195.
- Jain A, Jain S, Jain R, et al. Coated chitosan nanoparticles encapsulating caspase 3 activator for effective treatment of colorectral cancer. Drug Deliv Transl Res. 2015;5:596–610.
- Sarkar J, Singh N, Meena S, et al. Staurosporine induces apoptosis in human papillomavirus positive oral cancer cells at G2/M phase by disrupting mitochondrial membrane potential and modulation of cell cytoskeleton. Oral Oncol. 2009;45:974–979.
- Agnihotri SA, Mallikarjuna NN, Aminabhavi TM. Recent advances on chitosan-based micro- and nanoparticles in drug delivery. J Control Release. 2004;100:5–28.
- Khatik R, Dwivedi P, Shukla A, et al. Development, characterization and toxicological evaluations of phospholipids complexes of curcumin for effective drug delivery in cancer chemotherapy. Drug Deliv. 2016;23:1067–1078.
- Vredenburg MR, Ojima I, Veith J, et al. Effects of orally active taxanes on P-glycoprotein modulation and colon and breast carcinoma drug resistance. J Natl Cancer Inst. 2001;93:1234–1245.
- Kurata N, Kuramitsu T, Tanii H, et al. Development of a highly sensitive high-performance liquid chromatographic method for measuring an anticancer drug, UCN-01, in human plasma or urine. J Chromatogr B. 1998;708:223–227.
- Jain A, Mehra NK, Nahar M, et al. Topical delivery of enoxaparin using nanostructured lipid carrier. J Microencapsul. 2013;30:709–715.
- Xu Z, Gu W, Huang J, et al. In vitro and in vivo evaluation of actively targetable nanoparticles for paclitaxel delivery. Int J Pharm. 2005;288:361–368.
- Trickler WJ, Nagvekar AA, Dash AK. A novel nanoparticle formulation for sustained paclitaxel delivery. AAPS PharmSciTech. 2008;9:486–493.
- Wang X, Chi N, Tang X. Preparation of estradiol chitosan nanoparticles for improving nasal absorption and brain targeting. Eur J Pharma Biopharma. 2008;70:735–740.
- Jain A, Jain SK, Ganesh N, et al. Design and development of ligand-appended polysaccharidic nanoparticles for the delivery of oxaliplatin in colorectal cancer. Nanomedicine. 2010;6:179–190.
- Yang SJ, Lin FH, Tsai KC, et al. Folic acid-conjugated chitosan nanoparticles enhanced protoporphyrin IX accumulation in colorectal cancer cells. Bioconjugate Chem. 2010;21:679–689.
- Sahu SK, Mallick SK, Santra S, et al. In vitro evaluation of folic acid modified carboxymethyl chitosan nanoparticles loaded with doxorubicin for targeted delivery. J Mater Sci: Mater Med. 2010;21:1587–1597.
- Ramasamy S, Abdul Wahab N, Zainal Abidin N, et al. Growth inhibition of human gynecologic and colon cancer cells by Phyllanthus watsonii through apoptosis induction. PLoS One. 2012;7:e34793.
- Akinaga S, Nomura K, Gomi K, et al. Effect of UCN-01, a selective inhibitor of protein kinase C, on the cell-cycle distribution of human epidermoid carcinoma, A431 cells. Cancer Chemother Pharmacol. 1994;33:273–280.
- Chen X, Lowe M, Keyomarsi K. UCN-01-mediated G1 arrest in normal but not tumor breast cells is pRb-dependent and p53-independent. Oncogene. 1999;18:5691–5702.
- Abe S, Kubota T, Otani Y, et al. UCN-01 (7-hydoxystaurosporine) inhibits in vivo growth of human cancer cells through selective perturbation of G1 phase checkpoint machinery. Jpn J Cancer Res. 2001;92:537–545.
- Wang W, Bu B, Xie M, et al. Neural cell cycle dysregulation and central nervous system diseases. Progr Neurobiol. 2009;89:1–17.
- Pages F, Galon J, Dieu-Nosjean MC, et al. Immune infiltration in human tumors: a prognostic factor that should not be ignored. Oncogene. 2010;29:1093–1102.
- Kantola T, Klintrup K, Vayrynen JP, et al. Stage-dependent alterations of the serum cytokine pattern in colorectal carcinoma'. Br J Cancer. 2013;108:1917–1918.
- Naugler WE, and Karin M. The wolf in sheep’s clothing: the role of interleukin-6 in immunity, inflammation and cancer. Trends in Molecular Medicine. 2008;14:109–119.
- Knupfer H, and Preiss R. Serum interleukin-6 levels in colorectal cancer patients -a summary of published results. International Journal of Colorectal Disease. 2010;25:135–140.