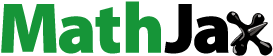
Abstract
The objective of this study was to assess the antibacterial activity of Rifampicin-loaded solid lipid nanoparticles on Brucella abortus 544. Rifampicin-loaded solid lipid nanoparticles were prepared by a modified microemulsion/sonication method and characterized. The results showed the average size about 319.7 nm, PI about 0.20 and zeta potential about 18.4 mv, encapsulation efficacy and drug-loading were equal to 95.78 and 34.2%, respectively, with a spherical shape. Drug release lasted for 5 days. The antibacterial activity was statistically significant with p < .05 in bacterial and cell culture media compared to free Rifampicin. It can be concluded that solid lipid nanoparticles can be considered as a promising delivery system for improving the antibacterial activity of Rifampicin against Brucella abortus.
Introduction
Brucellosis is a systemic infection observed in two forms including acute and chronic forms that can affect any organ or tissue in the body [Citation1]. Most chronic cases are caused by B. abortus [Citation2]. Brucella abortus is an intracellular bacterium that mononuclear-phagocytic system plays an essential role in coping with it [Citation3–5]. Some of these bacteria survive inside the macrophages, thus they will be protected against the immune system and antibiotics in the extracellular environment [Citation6] resulting in chronic and disease recurrence after treatment, which has been reported in 5–30% of patients [Citation2,Citation5]. Rifampicin is one of the antibiotics that are commonly used to treat brucellosis, which is administered along with Gentamicin or Streptomycin or Doxycycline for 2–3 weeks [Citation3–5].
Low permeability and instability in the intracellular medium due to lysosomal enzymes has resulted in the ineffectiveness of this antibiotic against intracellular Brucella [Citation6–8]. Rifampicin as one of the main drugs used for brucellosis treatment despite its extensive antibacterial activity, requires a replacement system/carrier system due to slight solubility and low absorption so that it can perform the endocytosis effectively by phagocytic cells and release its contents in the vicinity of bacteria [Citation9–11].
Therefore, it seems that drug-delivery using nanocarriers can be able to overcome these difficulties [Citation8,Citation12]. Solid lipid nanoparticles (SLNs) as a biodegradable carrier-system has been studied for many purposes [Citation8,Citation12,Citation13]. These nanoparticles can be harvested by phagocytic cells naturally (reticuloendothelial system or macrophage system) and release their contents within the target cells [Citation14,Citation15]. Therefore, the aim of this study was to evaluate the efficacy of Rifampicin-loaded SLNs (Rif-SLNs) on B. abortus 544 in vitro in order to develop a new therapeutic approach for the treatment of brucellosis.
Materials and methods
Stearic acid, Poloxamer 407 were purchased from Bio Basic Inc. (Markham, Canada), Lipoid S-100 was provided as a gift from the Lipoid Company (Ludwigshafen, Germany), Rifampicin powder was purchased from Sigma-Aldrich Company (Schnelldorf, Germany), and super pure distilled water (Q-water) was purchased from Kowsar Company (Alborz, Iran).
Preparation method
SLNs were prepared using a modified homogenization/sonication method. In summary, the optimal formulation was contained of 2% of stearic acid (400 mg, w/v) as matrix lipid, 0.75% of Poloxamer 407, (150 mg, w/v) as main surfactant, 0.25% of Lipoid S-100, (50 mg, w/v) as co-surfactant, Rifampicin (10 mg, w/v) and water (Q-water) at 20 ml volume. At first, Rifampicin was added to the melting lipid in the water bath at 75 °C and was mixed. Then, at the same temperature aqueous phase (surfactants mixture) it was added to the lipid phase suddenly. The obtained suspension was homogenized under mechanical stirrer (magnetic heater) at 70 °C with 600 rpm (rounds per minute) for 15 min. Afterward, it was subjected under a Sonication probe immediately (Bandelin, UV3100, Germany) at a medium frequency (amplitude, 50%) for 3-min and 4-cycles (30 s on and 15 s off). After sonication, SLN dispersion was placed at room temperature for 1 h to form nanoparticles. Same conditions were done exactly for preparation of blank SLN (without drug). The optimal formulation was lyophilized at 48–72 h by Freeze Dryer (Christ, BETA1-8LDPLUS, Germany) [Citation16–19]. Some formulations are presented in .
Table 1. Physicochemical properties of some formulations.
Size, PDI and zeta potential
The average particle size and polydispersity index (PDI) of SLNs and Rif-SLNs was measured at 25 °C by a Zeta Seizer Nano (ZS90, Malvern Instruments, Malvern, UK) (). Zeta potential (ZP) was measured using the same instrument in zeta mode (). Before measuring, SLN dispersion was diluted by water (Q-water) 1:30. Results which were obtained here were an average of measurements done for three times (triplicate) [Citation9,Citation16,Citation20,Citation21].
ʎ Max
Rifampicin (5 mg) was weighed and dissolved in 5 ml of methanol (Merck Company, Kenilworth, NJ). In order to prepare a stock solution (100 μg/ml), 1 ml of this solution was added to 9 ml of double-distilled water (DDW). Absorbance rate was measured by UV–vis spectrophotometry (UV-2100, Spectrum Technologies, Fort Worth, TX) at wavelengths from 200 to 400 at 5 nm intervals. The maximum Rifampicin wavelength was determined at 331 nm [Citation22].
Encapsulation efficacy (EE) and drug loading (DL)
HPLC method was used to measure the encapsulation efficacy (EE) and drug loading (DL). Using the lyophilized sample, 10 mg of Rif-SLNs, was dissolved in an equal ratio of methanol:acetonitrile (1:1, v/v). This solution was stirred vigorously for 3 min, followed by subjecting it under a sonication probe for 5 min. Then, it was centrifuged at 15,000 rpm for 10 min at 4 °C, after that, the supernatant was filtered (0.22 mm). In preparing internal standard, 5 mg/ml of Rifampicin (HPLC grade, Sigma) was prepared in the same ratio as described above and serial dilutions were prepared. Standards and samples were analyzed by HPLC instrument (SY-8100 model, China’s Birl Company, Fenghua). Rifampicin was detected in samples using a C18 chromatography column (250 mm × 4.6 mm, 5 μm) in a flow rate of 1.0 ml/min, at 331 nm wavelength by SY-8200 Multi-Wavelength UV Detector. The sample peak area ratio and internal standard were used for quantitative analysis. The regression and linearity equation which were equal to y = 1865/43x−5, 121/17 and 0.98 (r2), respectively showed the specificity of the method. The internal standard, DDW, and all other chemicals and solvents were selected with a high analytic degree. The (EE) and (DL) were calculated using the following equations [Citation23–26]:
(1)
(1)
(2)
(2)
Differential scanning calorimetry (DSC)
The behavior of the Rif-SLNs and its components was evaluated by differential scanning calorimetry (DSC) (Mettler Toledo, DSC1, Brazil). A small amount of Rif-SLNs lyophilized powder (5–10 mg) and each ingredients was contained of free Rifampicin, stearic acid and a physical mixture of drug-lipid, was heated in the range of 20–400 °C (5 k/min) and was subjected under the nitrogen gas (80 ml/min) [Citation25,Citation27,Citation28].
Fourier-transform infrared spectroscopy (FTIR)
In order to investigate the samples by spectroscopy, a small amount of each SLNs ingredients and Rif-SLNs, were mixed separately and homogeneously with a small amount of KBr (potassium bromide) and was converted into compact discs by a hydraulic compressor. Spectroscopy was performed by using FTIR spectroscopy (Perkin Elmer, Waltham, MA, Spectrum 400) in middle range IR (4000–400 cm−1) [Citation9].
Morphology
Morphology of RIF-SLNs was investigated by Field Emission-Scanning Electron Microscopy (FE-SEM) (MIRA3, TESCAN Company, Brno, Czech Republic). An amount (1–2 mg) of Rif-SLNs lyophilized was dispersed in DDW. Then, the imaging was carried out at 30 kV voltage [Citation29].
Release
The release test was performed using a dialysis bag (Sigma-Aldrich) with 2.5 nm pore and molecular weight cutoff about (12,000–14,000 Daltons). The membrane was soaked in DDW 12 h before use. Phosphate buffer (pH 7.4, 37 °C) was used as a release medium. Rif-SLNs (10 mg) lyophilized powder was dispersed in 2 ml of DDW and was placed in dialysis bag. Both ends of the bag were sealed and put in 60 ml of release medium (soaked), and then it was located in a shaker incubator (37 °C, 100 rpm). At constant intervals (0, 1, 2, 4, 8, 12, 24, 48, 72, 96 and 120 h), 2 ml of the medium was withdrawn and was substituted immediately with the same amount of PBS (pH 7.4) to keep the sink conditions. The same method was used for free Rifampicin. Aliquots of dialysis fluid (dialysate) were withdrawn from the sample at predetermined times and then were measured by UV-spectrophotometry at 331 nm [Citation16,Citation22,Citation24,Citation27].
Stability
SLN dispersion stability was performed for short-term (1 month at 25 °C) and long-term (6–9 months at 4 °C). In short-term, SLN dispersion was inspected visually to produce any possible form. In long-term, size, PDI, ZP and physical appearance was evaluated according to zero time [Citation16,Citation19,Citation24].
Antibacterial activity
Agar Well Diffusion was used to evaluate the antibacterial activity of free Rifampicin and Rif-SLNs. Brucella abortus biovar 3 strain 544 (Iran, Karaj, Brucellosis Section) was used as a strain to be tested. An aqueous solution of Rifampicin (100 μg/ml), Rif-SLNs (3 mg/ml based on loading ratio), blank SLNs and Rifampicin antibiotic disk (5 µg, Patan-Teb Company, Tehran, Iran) was prepared. The microbial suspension which had grown on Brucella agar at condition (48 h, 37 °C, 5% CO2) was adjusted in comparison to opacity 0.5 McFarland. Then, using sterile swabs, they were cultured on Mueller-Hinton agar plates. Next, wells (diameter 8 mm) were created in culture media by sterile Pasteur pipette. The volume containing 80 μl of each of the samples was added to wells. Bacterial growth around the wells was examined after 72 h incubation (37 °C, 5% CO2) () [Citation23,Citation27,Citation30].
Minimum inhibition concentration (MIC) assay
Microdilution method was used to perform minimum inhibition concentration (MIC) test. First, serial dilutions of Rif-SLNs lyophilized equivalent to loading ratio (512 μg/ml) and free Rifampicin (512 μg/ml) in Muller–Hinton broth were prepared in 96-well ELISA Microplates to reach concentrations of 256–0.0625 μg/ml. Then, 100 μl of bacterial suspension called B. abortus (grown on brucella agar for 48 h, 37 °C, 5% CO2) was added to each well to make an optimum concentration of 5 × 106 CFU/ml. First, absorbance was measured at 546 nm. Then, they were incubated at conditions (37 °C, CO2 5%). After 24, 48 and 72 h, absorbance was read at the same wavelength. Also, after 24 and 72 h the culture was carried out (1, 2 and 4). Results were validated using statistical analysis at p values <.05 [Citation31].
MTT assay
Cytotoxicity test was performed using MTT assay kit [3-(4,5-dimethylthiazol-2-yl)-2,5-diphenyltetrazolium bromide]. Mouse monocyte-macrophage cells J774A.1 (ATCC TIB-67; BALB/c Mouse, hematopoietic, macrophage-like; Pasteur Institute, Tehran, Iran), In DMEM (Gibco Product, Carlsbad, CA) were enriched by 10% of FBS and 1% of penicillin–streptomycin antibiotics, in 96-wells (Becton Dickinson Labware, Franklin Lakes, NJ) and were cultured at the concentration of 1 × 105 cells/wells at 37 °C and 5% of CO2 for 24 h. Different concentrations (double dilutions) of free Rifampicin (500–37.5 μg/ml) and Rif-SLNs (5–0.375 mg/ml) were added to wells containing DMEM. After 24 h, 10 μl of MTT was added, and after 3–4 h of incubation, the culture medium was removed and replaced with 200 µl of dimethyl sulfoxide (DMSO, Molecular & Cell Culture Grade) which was poured to each well. Then, it was shaken for about 20 min at 100 rpm to dissolve formazan crystals. Cell viability percentage was calculated by dividing the absorbance values of treated cells into untreated cells (control). IC50 of each sample was calculated using a software program (Graph Pad Prism 8.0.0, Inc., San Diego, CA) [Citation23,Citation27,Citation30].
In vitro cellular infection
In brief, before infection, J774A.1 cells were cultured in 10% of FBS-enriched DMEM in conditions (37 °C and CO2 5%) at 105/wells in 24-cell plates (Corning Inc., Corning, NY). After 24 h, cells were infected with B. abortus 544 in exponential phase (48 h culture in Brucella agar, 37 °C, CO2 5%) at a ratio of 1:100. Then, the culture medium was removed and cells were washed three times by DMEM containing 50 μg/ml of Gentamycin (Sigma Product Co.) to remove non-phagocyted, and bacterial attachment to the cell wall. Then, serial concentrations of free Rifampicin and Rif-SLNs (64–6.0625 µg/ml) were prepared below of the toxicity threshold and were added to DMEM (FBS 10%, without antibiotic) to compare the control wells (not treated cells). After 24 h incubation, DMEM medium was removed and cells were washed once by PBS and the second time by normal saline. Then, using Triton X-100 0.2% in PBS, lysed and serial dilutions and 10 samples of lysate were cultured on Brucella agar plates. After 96 h of incubation (37 °C, CO2 5%), the efficacy of Rif-SLNs was investigated regarding intracellular B. abortus 544 by colony counting compared to free Rifampicin [Citation9,Citation32].
Statistical analysis
Statistical analyses were performed using IBM SPSS Version 22.0 (IBM SPSS Statistics, Armonk, NY). Independent samples test and paired samples test were used for analyzing the effect of free Rifampicin and Rif-SLNs on MIC and colony counting in bacterial and cell culture media. P values of < .05 were considered statistically significant.
Results
Size, PDI and ZP
Using more than 400 mg of stearic acid in the formulation resulted in an increase in the viscosity, size and PDI. Using 200 mg and lower of stearic acid led to an increase in size and PDI, instability, drug leakage and sedimentation. At least 100 mg of Poloxamer 407 was required for stability, but increasing its amount, led to an increase in size (above 500 nm), PDI and a reflection on particle surface (interference in the Zetasizer). By contrast, using less amount of it led to drug leakage (data not shown). Adding 50 mg of Lipoid S-100 to formulations resulted in the decrease in size and PDI, with a spherical shape, preventing light reflection during measurement by Zetasizer, and also led to an increase in ZP, DL and EE, and stability. It was also noteworthy that, particles size was higher than 1-micron without using a Sonication probe. Amplitude intensity higher than 50 mV caused an increase in PDI and production foam in the SLNs dispersion. Conversely, at amplitude intensity below 50 mV desirable results were not achieved, due to the longer sonication time. Sonication time and cycles number had an effect on size, PDI and drug-leakage (data not shown). These conditions were optimized for 3 min (four cycles: 30 s on and 15 s off). Adding Rifampicin (10 mg) to the preparation process reduced ZP (−16 to −22 mV) compared to blank SLNs (−40 to −50 mV) ().
EE and DL
The retention time of Rif-SLNs and the internal standard curve are shown in ( and ), respectively. The retention time of Rifampicin was equal to 6.8 min. EE and DL calculated were equal to 95.78 and 34.2%, respectively. Due to the high solubility of Rifampicin in Stearic acid, EE was high enough for optimum formulation.
DSC
Thermograms of Rif-SLNs, Stearic acid, physicochemical drug-lipid and free Rifampicin are shown in . The stearic acid thermogram displays an endothermic peak at 56.43 °C. Rifampicin thermogram shows exothermic peaks about 75–94 °C and an exothermic peak at 250 °C, representing polymorphic purity of the drug. The physicochemical mixture of drug-lipid only shows the two exothermic peaks at 55 and 248 °C. In Rif-SLNs thermogram, endothermic peak related to Stearic acid was detected easily, while peaks related to Rifampicin were disappeared.
FTIR
Results of spectroscopy on Rif-SLNs, stearic acid, Poloxamer 407, Lipoid S-100 and free Rifampicin are shown in . In , the spectral numbers of each substance and Rif-SLNs are shown. According to , it can be observed that Rif-SLNs spectral numbers are consistent with four primary materials (matrix, drug, surfactant and co-surfactant), and new spectral numbers were not observed indicating a new functional group or bond.
Table 2. Spectral numbers of each substance and Rif-SLNs.
FE-SEM
The FE-SEM results showed that Rif-SLNs are in the nano range, with spherical to oval form with a smooth surface. The particle size was matched with Zetasizer data ().
Release
Release test performed during the 120-h period (5 days at PBS, pH 7.4) showed a double pattern. A rapid release was observed about 5% in the first 4 h. After that, the sustained release was started at a constant speed. The perfect release was observed in Rif-SLNs and free Rifampicin at 120 h and 24 h end, respectively [Citation25,Citation27,Citation33] ().
Stability
The optimum formulation was considered stable under conditions (for 1 month at 25 °C and for 9 months in 4 °C) along with an increase in size which was <10 and 20%, respectively. Sedimentation, drug-leakage and aggregation were not observed in both conditions ().
Table 3. Rif-SLNs dispersion stability trend in different times and temperatures based on size, PDI, ZP and appearance form.
Antibacterial activity
Due to the high amount of EE, a significant improvement was observed regarding the anti-brucella activity of Rif-SLNs against free Rifampicin in this study. It was found that MIC for free Rifampicin at 24, 48 and 72 h was equal to 8, 64 and 256 μg/ml, respectively. While MIC for Rif-SLNs at 24–48 h after testing, was equal to 256 μg/ml, and 72 h later, was decreased to 4 μg/ml. Free Rifampicin solution with MIC (8 μg/ml), and against Rif-SLNs (4 μg/ml), inhibited bacterial growth. These results showed that the improvement of antibacterial activity in Rif-SLNs was twice more than free Rifampicin.
MTT and cell culture
IC50 values for Rif-SLNs and free Rifampicin were obtained equal to 161.3 μg/ml and 4.66 mg/ml (87/89 μg/ml), respectively ( and ). Comparing the efficacy of free Rifampicin and Rif-SLNs on cells infected with B. abortus 544, in all concentrations, a significant reduction was observed in colony counting for cells treated with Rif-SLNs against free Rifampicin (p<.05) ().
Discussion
Method
SLN preparation technique was selected based on particle size, PDI and EE. Homogenization or ultrasonic technique is a simple method for producing nanoparticles in large-scale without the need for organic solvents and complex equipment [Citation11]. In this study, a Magnetic Stirrer (Magnetic Heater) was used instead of a high shear homogenizer to prevent metal contamination and to increase PDI [Citation34,Citation35]. This method is a suitable structural model for encapsulating lipophilic drugs such as Rifampicin in SLNs regarding purposes for which it needs to be protected from deactivation and therefore it can be released slowly.
Size, PDI and ZP
Stearic acid is a fatty acid (molecular weighing 284.48) with a medium-chain and negative charge at neutral pH, compared to the characteristics of any solid lipid, it is clear that stearic acid is the best choice for Rifampicin due to its good solubility in this lipid and the higher drug-loading potential [Citation21,Citation35–38]. In the optimal formulation, 2% of stearic acid was used that particle size was between 300 and 400 nm, but in the formulation of more than 2.5%, particle size was reached to more than 500 nm. Also, an increase in viscosity, gelation (occurs due to the possibility of high particle collision and increased contact between particles and vial surfaces), and aggregation were observed that is probably due to a decrease in homogenization efficacy at the high-lipid content [Citation11]. Therefore, using stearic acid at <1% leads to instability and an increase in size and PDI.
Poloxamer 407 is a non-ionic surfactant that due to produce smaller size and less toxicity than Poloxamer 188 it prevents from the lipid matrix destruction at in vivo by controlling lipase/co-lipase complex [Citation39].
In this study using Poloxamer 407 solely increased size, PDI and drug-leakage, rather than; co-surfactant which was used to solve this problem.
Lipoid S-100 (derived from soy lecithin), which was used in this study as a co-surfactant resulted in the smaller size, an increase in stability (due to its capability to produce the film strength) and drug-loading [Citation9,Citation40–42]. Soy lecithin is a biodegradable phospholipid, non-toxic, metabolize-like natural lipid, which is able to emulsify lipids and lipophilic drugs to create water-in-oil or oil-in-water emulsions, it has been reported useful in different nanocarriers [Citation39,Citation43]. The present study confirmed that without using a Lipoid S-100, the size of nanoparticles was higher than 700 nm, but using the Lipoid S-100, size was decreased to 300–400 nm. By adding 50 mg of Lipoid S-100 to the formulation, ZP was increased to −12 mV when used only from the Poloxamer 407. This property helps to prepare a more stable formulation [Citation32].
Another parameter in nanoparticles production is to optimize the time, temperature and homogenization speed of the magnetic stirrer (magnetic heater). Increasing homogenization time from 5 to 15 min at 600 rpm and 70 °C leads to making a homogeneous microemulsion, and also a decrease in PDI and obtaining a uniform size. Homogenization conditions had critical effects on size and its distribution. When homogenization occurs at the lipid melting point during the homogenization process, the softened lipid can be scattered easily, which results in more uniformity of product () [Citation39]. It is also noteworthy that, after homogenization, the obtained microemulsion was subjected under sonication immediately, otherwise desired result could not be achieved. Then, setting time of sonication, amplitude intensity and cycle’s number of the Sonication probe are other important factors in the production of nanoparticles. Reducing sonication amplitude intensity from 80% (caused induction foam and an increase in size and PDI) to 50% (optimal amplitude), sonication time from 10 min (drug-leakage and instability) to 3 min (optimal time) are suitable parameters for production smaller size and uniform PDI. After sonication, SLN dispersion should be cooled at room temperature for at least 1 h and fast cooling or shaking during cooling should be avoided [Citation39]. ZP is a critical factor in SLNs stability, which should be at least 15 mV [Citation35]. Rifampicin has a positive charge partially [Citation20] but stearic acid has a negative charge [Citation21,Citation35,Citation37,Citation38]. When Rifampicin is incorporated in Stearic acid, the ZP of stearic acid was reduced from −40 to −50 mV to less than −20 mV. In the present study, the charge of nanoparticles was measured (−16 to −22 mV); which is an indicator of drug-loading. This result has also been reported by others, which implies drug incorporating into the lipid matrix [Citation19,Citation32,Citation38] (). In general, SLN dispersion with low ZP (±10 mV) is not stable physically due to the quick accumulation of the particles [Citation20].
EE and DL
Due to Rifampicin lipophilicity and its high solubility in stearic acid is an excellent choice for loading Rifampicin in drug delivery, so it is expected to have drug molecules well-incorporated in the lipid matrix and providing higher encapsulation percentage [Citation25,Citation32]. The best encapsulation percentage depends on the amount and quality of drug, surfactant and co-surfactant ratio, type and amount of lipid, water quality, time and amplitude of sonication, and used method [Citation32].
DSC
Usually, the melting point of stearic acid is equal to 69 °C. According to obtained result, Stearic acid melting and freezing points are not the same exactly, depending on the product purity and the manufacturer but is usually about 55–70 °C [Citation44–46]. Peaks produced in this study are attributed to Palmitic acid content <10% w/w, as a result, it’s melting point has displaced from 54.7 to 59.9 °C [Citation47,Citation48]. Melting and crystallization peaks of Stearic acid are variable which has at least four polymorphic forms [Citation27]. Stearic acid has many amounts of α form which tend to disappear under thermal pressure; as a result, high thermodynamic instability appears. For this reason, in order to increase its long-term emulsion stability, it seems necessary to use a suitable surfactant/co-surfactant compound [Citation45]. According to the previous studies, Rifampicin has endothermic and exothermic characteristics from 190 to 260 °C [Citation44]. Disappearing Rifampicin melting peak in the thermogram of physical mixture indicates the interaction of Rifampicin with lipid because the lipid melting tends to solubilize Rifampicin. In the case of Rif-SLNs, Rifampicin peak shift to a lower temperature confirms its incorporation into the SLNs [Citation41]. Considering that Rif-SLNs thermogram does not show Rifampicin melting peak in the 190 °C range, indicates that probably Rifampicin is incorporated completely in the lipid network of nanoparticles and is probably safe against destruction [Citation42]. These factors indicate the molecular dispersion of Rifampicin in the lipid matrix of nanoparticles and confirm its presence in an amorphous form [Citation44]. Rifampicin seems to cause disorders in the crystalline structure of SLNs. Furthermore, incorporation of Rifampicin into nanoparticles, leads to the decrease in melting temperature and enthalpy in stearic acid [Citation49].
FTIR
In the FTIR study, it was observed that there was no functional peak or interaction between the nanoparticles components. The difference in spectral values of the raw materials with the Rif-SLNs is in peak intensity, indicating the similarity between the functional groups and the Rif-SLNs to raw materials, as well as the difference in the quantitative amount of each bond or functional group. Therefore, this information indicates that no chemical bond was formed between nanocarrier and drug. These findings, in turn, show the stability of the drug structure in the preparation process [Citation9].
FE-SEM
The image taken with FE-SEM confirmed the spherical and small size of nanoparticles. The relatively small difference which was observed between Zetasizer and FE-SEM may be due to the specific structure of stearic acid, which differentiates slightly.
The size range obtained in this study from 80 to 1000 nm in the spherical shape with a smooth surface seems to be desirable for entering macrophages. Spherical shape indicates that drug-loading has not led to morphological changes. Spherical nanoparticles contribute to the controlled release of the entrapped drug because the spherical shape has the longest route for moving drug and the lowest contact level with the aqueous medium (disperse phase) than other forms of nanoparticles [Citation13,Citation16].
Release
In in-vitro conditions, drug release from Rif-SLNs showed biphasic behavior. At first time interval of up to 4 h, there is a rapid release in the Rif -SLNs, which is attributed to not trapped free Rifampicin or to detached drug from the outer layer that has been adsorbed on the surface of SLNs and it should not be considered [Citation49]. Rifampicin is a high-solubility drug in lipid, and therefore the Rifampicin incorporated in the lipid matrix is responsible for the second sustained release phase, which starts from 4 h and goes up to 120 h (stable release for hours) [Citation9]. Continuous release (120 h) indicates that Rif-SLNs can prevent explosive release. Lipid matrix nature, surfactant and co-surfactant ratio (3:1) and parameters involved in production nanoparticles are effective factors on drug-release from SLNs [Citation16]. The more the drug loaded on the surface particles leads to faster release, on the contrary, the incorporated drug in the lipid matrix leads to the slower release of drug [Citation16].
Stability
The size of nanoparticles is the best indicator of stability assessment [Citation32]. Considering the initial volumes in each period, the mean measurement size, PDI and ZP, showed that Rif-SLNs is stable without a significant increase in physical parameters (). Stability studies showed that Rif-SLNs have good stability, which can be due to the correct selection of surfactants (Poloxamer407) and Lipoid S-100 in proper ratio (3:1) (). Poloxamer 407 provides the high stability and Lipoid S-100, creates a layer on the level of nanoparticles which prevents the particles from colliding [Citation9,Citation39,Citation40].
Antibacterial activity
Variations in two groups (free Rifampicin and Rif-SLNs) was statistically significant (p = .003). Mean response in both groups was different statistically (). Variations process at 24, 48 and 72 h was statistically significant in two groups (free Rifampicin and Rif- SLNs) with p = 0.001 (). Namely, type of drug and Nano-drug, and dose rate have had a different effect on response variation at 24, 48 and 72 h (p < .01). Improving the anti-bacterial effect of Rif-SLNs in Muller–Hinton broth was associated with drug-solubility, sustained release and drug protection against inactivation [Citation32]. MIC test showed that Rifampicin in free form is only active for 1–2 days. As a result, the antibacterial activity of free Rifampicin was decreased which is confirmed by a higher number of colony counting in the culture medium (. In contrast, Rif-SLNs due to drug encapsulation prevents it from being inactivated and along with its controlled release over time, acts more efficiently than free Rifampicin on bacteria [Citation40] (.
Table 4. Comparing results at different times (24, 48 and 72 h) in two groups (free Rifampicin and Rif-SLNs).
Table 5. Variations process at different times (24, 48 and 72 h) in two groups (free Rifampicin and Rif-SLNs).
Cell culture
For the first time in this study, the efficacy of Rif-SLNs on B. abortus 544 was investigated in cell culture. Compared to free drug, reduction in the number of intracellular bacteria treated with Rif-SLNs was statistically significant with a p < .05 versus free Rifampicin as a predictable result, confirmed therapeutic efficacy of Rif-SLNs strongly. Studies have shown that nanoparticles greater than 200 nm are suitable for phagocytosis done by macrophages [Citation11,Citation19,Citation35,Citation46]. Improving the efficacy of Rif-SLNs on cells infected with B. abortus 544 can be due to phagocytosis occurred by target cells, continuous release, and drug protection against inactivation [Citation50]. Antibiotic-loaded nanoparticles can reach to the site or niche of bacteria in phagocytic cells, where they are able to release their contents near their targets. Therefore, using SLN as Rifampicin carrier leads to controlled drug release and a decrease in long-term treatment. Rifampicin is widely used to treat brucellosis and a wide range of infectious diseases, including TB [Citation40].
Reduced number of bacteria in brucellosis under treatment with free Rifampicin is due to the killing of some extracellular bacteria and those transmitted through life cycles to other cells. Although no investigator has ever stated that nano-based antibiotic therapy has resulted in complete elimination of intracellular bacteria, it has been reported that loading antibiotics into SLNs can lead to an increase in drug solubility, a decrease in cellular toxicity and finally, it will improve the treatment. In the present study, acceptable results were obtained regarding the efficacy improvement of Rifampicin on intracellular B. abortus 544, although further studies are needed to confirm these findings. The results of this study and previous studies [Citation50] showed that the value of this work has a promising approach.
Conclusion
The preparation of Rif-SLNs using 2% of stearic acid, 0.75% of poloxamer 407 and 0.25% of Lipoid S-100 by a Homogenization/Sonication modified method, produced desirable formulation. In the MIC test, Rif-SLNs were more effective several times more than free Rifampicin regarding inhibiting the number of bacteria in cell cultures, its anti-brucella efficacy was improved significantly.
Based on the results of this study, it seems that SLNs can be considered as a suitable carrier for Rifampicin against B. abortus 544. Currently, there are very limited reports regarding the field of application and nano-antibiotics toxicity. Therefore, further studies knowledge and interdisciplinary tools are required for developing effective and targeted treatment of infectious diseases using nano-antibiotics. Nonetheless, in the case of confirming the efficacy of Rif-SLNs on B. abortus 544, further studies are recommended. Overall results in this study indicate that Rif-SLNs provide a promising strategy for drug delivery and represent a promising proof-of-concept against one of the infectious diseases of the world.
Acknowledgements
We would like to thank, Andrea Schumacher for her kind support regarding providing material (Lipoid S-100, Lipoid Company, Germany), Dr. Mohammad Yousef Alikhani, Administrator of Microbiology Department and Brucellosis Centre for inter-institutional coordination. We also appreciate Dr. Abbas Farmani, Manager of Comprehensive Research Labs for preparing necessary types of equipment, and Ms. Samira Ataei, in charge of the Science and Technology Park for providing required equipment in making nanoparticles.
Disclosure statement
No potential conflict of interest was reported by the authors.
Additional information
Funding
References
- Farazi A, Zarrinfar N, Didgar F, et al. Risk factors for failure of treatment and relapse of brucellosis. Arak Uni Med Sci. 2014;17(4):47–53.
- Díaz R, Gamazo C. Protective effect of liposomal gentamicin against systemic acute murine brucellosis. Chemotherapy 1997;43:204–210.
- de Figueiredo P, Ficht TA, Rice-Ficht A, et al. Pathogenesis and immunobiology of brucellosis: review of Brucella-host interactions. Am J Pathol. 2015;185:1505–1517.
- Seleem MN, Jain N, Pothayee N, et al. Targeting Brucella melitensis with polymeric nanoparticles containing streptomycin and doxycycline. FEMS Microbiol Lett. 2009;294:24–31.
- Roushan MRH, Moulana Z, Afshar ZM, et al. Risk factors for relapse of human brucellosis. Global J Health Sci. 2016;8:77.
- Imbuluzqueta E, Gamazo C, Ariza J, et al. Drug delivery systems for potential treatment of intracellular bacterial infections. Front Biosci. 2010;65:1215–1223.
- Kunjachan S, Jose S, Thomas CA, et al. Physicochemical and biological aspects of macrophage‐mediated drug targeting in anti‐microbial therapy. Fundam Clin Pharmacol. 2012;26:63–71.
- Briones E, Colino CI, Lanao JM. Delivery systems to increase the selectivity of antibiotics in phagocytic cells. J Control Release. 2008;125:210–227.
- Farnia P, Velayati AA, Mollaei S, et al. Modified rifampin nanoparticles: increased solubility with slow release rate. Int J Mycobacteriol. 2017;6:171.
- Gelperina S, Kisich K, Iseman MD, et al. The potential advantages of nanoparticle drug delivery systems in chemotherapy of tuberculosis. Am J Respir Crit Care Med. 2005;172:1487–1490.
- Lopes CPA. Development and characterization of lipid nanoparticles prepared by miniemulsion technique [dissertation]. Portugal: Technico Lisboa; 2014.
- Pei Y, Yeo Y. Drug delivery to macrophages: challenges and opportunities. J Control Release. 2016;240:202–211.
- Ekambaram P, Sathali AAH, Priyanka K. Solid lipid nanoparticles: a review. Sci Rev Chem Commun. 2012;2:80–102.
- Xiong M-H, Bao Y, Yang X-Z, et al. Delivery of antibiotics with polymeric particles. Adv Drug Deliv Rev. 2014;78:63–76.
- Kharaji MH, Doroud D, Taheri T, et al. Drug targeting to macrophages with solid lipid nanoparticles harboring paromomycin: an in vitro evaluation against L. major and L. tropica. AAPS PharmSciTech. 2016;17:1110–1119.
- Kalhapure RS, Mocktar C, Sikwal DR, et al. Ion pairing with linoleic acid simultaneously enhances encapsulation efficiency and antibacterial activity of vancomycin in solid lipid nanoparticles. Coll Surf B Biointer. 2014;117:303–311.
- Vitthal KU, Pillai M, Kininge P. Study of solid lipid nanoparticles as a carrier for bacoside. Int J Pharma BioSci. 2013;3:414–426.
- Ekambaram P, Sathali A. Formulation and evaluation of solid lipid nanoparticles of ramipril. J Young Pharm. 2011;3:216–220.
- Kumar M, Kakkar V, Mishra AK, et al. Intranasal delivery of streptomycin sulfate (STRS) loaded solid lipid nanoparticles to brain and blood. Int J Pharm. 2014;461:223–233.
- Li M, Zahi MR, Yuan Q, et al. Preparation and stability of astaxanthin solid lipid nanoparticles based on stearic acid. Eur J Lipid Sci Technol. 2016;118:592–602.
- Siddiqui A, Alayoubi A, El-Malah Y, et al. Modeling the effect of sonication parameters on size and dispersion temperature of solid lipid nanoparticles (SLNs) by response surface methodology (RSM). Pharm Dev Technol. 2014;19:342–346.
- Sivadasan D, Madavan B, Penmatsa SD, et al. Formulation and characterization of solid lipid nanoparticles of rifampicin. Erciyes Med J. 2012;35:1–5.
- Pandey R, Sharma S, Khuller G. Oral solid lipid nanoparticle-based antitubercular chemotherapy. Tuberculosis. 2005;85:415–420.
- Xu XM, Wang YS, Chen RY, et al. Formulation and pharmacokinetic evaluation of tetracycline-loaded solid lipid nanoparticles for subcutaneous injection in mice. Chem Pharm Bull. 2011;59:260–265.
- Singh H, Bhandari R, Kaur IP. Encapsulation of Rifampicin in a solid lipid nanoparticulate system to limit its degradation and interaction with Isoniazid at acidic pH. Int J Pharm. 2013;446:106–111.
- Lim WH, Tan YJ, Lee CS, et al. Preparation and optimization of palm-based lipid nanoparticles loaded with griseofulvin. Iran J Pharm Res. 2017;16:451.
- Aboutaleb E, Noori M, Gandomi N, et al. Improved antimycobacterial activity of rifampin using solid lipid nanoparticles. Int Nano Lett. 2012;2:33.
- Kakkar V, Kaur IP. Preparation, characterization and scale-up of sesamol loaded solid lipid nanoparticles. Nanotechnol Dev. 2012;2:8.
- Mehnert W, Mäder K. Solid lipid nanoparticles: production, characterization and applications. Adv Drug Deliv Rev. 2012;64:83–101.
- Kazemi D, Salouti M, Rostamizadeh K, et al. Development of gentamicin-loaded solid lipid nanoparticles: evaluation of drug release kinetic and antibacterial activity against Staphylococcus aureus. Int J Pharm Res Innov. 2014;7:1–6.
- Ghaffari S, Varshosaz J, Saadat A, et al. Stability and antimicrobial effect of amikacin-loaded solid lipid nanoparticles. Int J Nanomed. 2011;6:35.
- Jain D, Banerjee R. Comparison of ciprofloxacin hydrochloride‐loaded protein, lipid, and chitosan nanoparticles for drug delivery. J Biomed Mater Res. 2008;86:105–112.
- Hu F-Q, Jiang S-P, Du Y-Z, et al. Preparation and characterization of stearic acid nanostructured lipid carriers by solvent diffusion method in an aqueous system. Coll Surf B: Biointer. 2005;45:167–173.
- Nikam S, Chavan M, Sharma PH. Solid lipid nanoparticles: a lipid based drug delivery. Nanotechnology. 2014;1:5.
- Gupta S, Kesarla R, Chotai N, et al. Systematic approach for the formulation and optimization of solid lipid nanoparticles of efavirenz by high pressure homogenization using design of experiments for brain targeting and enhanced bioavailability. BioMed Res Int. 2017;2017:1.
- Lalan M, Baweja J, Misra A. Atopic dermatitis: drug delivery approaches in disease management. Crit Rev Ther Drug Carr Syst. 2015;32:323–361.
- Wang R, Li L, Wang B, et al. FK506-loaded solid lipid nanoparticles: preparation, characterization and in vitro transdermal drug delivery. Afr J Pharm Pharmacol. 2012;6:904–913.
- Sharma HS, Sharma A. Conference scene: nanoneuroprotection and nanoneurotoxicity: recent progress and future perspectives. Nanomedicine 2010;5:533–537.
- Hippalgaonkar K, Majumdar S, Kansara V. Injectable lipid emulsions—advancements, opportunities and challenges. AAPS PharmSciTech. 2010;11:1526–1540.
- Tan ME, He CH, Jiang W, et al. Development of solid lipid nanoparticles containing total flavonoid extract from Dracocephalum moldavica L. and their therapeutic effect against myocardial ischemia–reperfusion injury in rats. Int J Nanomed. 2017;12:3253.
- Singh H, Jindal S, Singh M, et al. Nano-formulation of rifampicin with enhanced bioavailability: development, characterization and in-vivo safety. Int J Pharm. 2015;485:138–151.
- Wissing S, Müller R. The influence of the crystallinity of lipid nanoparticles on their occlusive properties. Int J Pharm. 2002;242:377–379.
- Singh RP, Gangadharappa H, Mruthunjaya K. Phospholipids: unique carriers for drug delivery systems. J Drug Deliv Sci Technol. 2017;39:166–179.
- Du Y, Zhai Y, Zhang J, et al. Development and evaluation of taste-masked dry suspension of cefuroxime axetil for enhancement of oral bioavailability. Asian J Pharm Sci. 2013;8:287–294.
- Severino P, Pinho SC, Souto EB, et al. Polymorphism, crystallinity and hydrophilic-lipophilic balance of stearic acid and stearic acid-capric/caprylic triglyceride matrices for production of stable nanoparticles. Coll Surf B Biointer. 2011;86:125–130.
- Chen D-B, Yang T-Z, Lu W-L, et al. In vitro and in vivo study of two types of long-circulating solid lipid nanoparticles containing paclitaxel. Chem Pharm Bull. 2001;49:1444–1447.
- Ebrahimi HA, Javadzadeh Y, Hamidi M, et al. Repaglinide-loaded solid lipid nanoparticles: effect of using different surfactants/stabilizers on physicochemical properties of nanoparticles. DARU J Pharm Sci. 2015;23:46.
- Bibi S, Bremner DH, Macdougall-Heasman M, et al. A preliminary investigation to group disparate batches of licit and illicit diazepam tablets using differential scanning calorimetry. Anal Methods. 2015;7:8597–8604.
- Vieira AC, Magalhães J, Rocha S, et al. Targeted macrophages delivery of rifampicin-loaded lipid nanoparticles to improve tuberculosis treatment. Nanomedicine 2017;12:2721–2736.
- Bodaghabadi N, Hajigholami S, Malekshahi ZV, et al. Preparation and evaluation of rifampicin and co-trimoxazole-loaded nanocarrier against Brucella melitensis infection. Iran Biomed J. 2018;22:275.