Abstract
Circular RNAs (circRNAs) have been revealed to play important roles in modulating gene expression and are involved in several pathological processes. However, a large number of the circRNAs in bladder cancer progression remain undetermined. In this study, the expression level of circCDYL was detected by quantitative real-time PCR in bladder cancer tissues. The circCDYL over-expression plasmid was constructed and transfected into bladder cancer cell lines. The cell migration was detected by using transwell migration assay and wound healing assay, and cell cycles were detected by flow cytometry. The relative protein expression was detected by Western blotting. It was found that circCDYL was low expressed in bladder cancer tissues and cell lines. Functionally, over-expression of circCDYL inhibited cell growth and migration. Importantly, over-expression of circCDYL down-regulated the protein level of C-MYC in both EJ and T24T cells, while the mRNA level of C-MYC was not significantly reduced. Furthermore, over-expression of C-MYC could partly reverse the G0/G1 phase cell cycle arrest induced by circCDYL in bladder cancer cells. Our findings suggest that circCDYL functions as a tumor suppressor in bladder cancer by down-regulating the expression of C-MYC, and this circular RNA might be used as a new target for bladder cancer therapy.
Introduction
As one of the most commonly occurring urological malignancy, bladder cancer is also the eleventh most frequently diagnosed cancer worldwide [Citation1]. Bladder cancer is diagnosed in over 430,000 patients and causes about 165,000 deaths every year in the world. Despite considerable progress being made in surgical techniques [Citation2], the 5-year survival rate of bladder tumor is still at the same level [Citation3]. In order to improve the treatment of bladder cancer, we need a better understanding of detailed mechanisms activated during the growth and progress of cancer.
Circular RNA (circRNA) is an important class of non-coding RNA and it was first found in 1976 [Citation4,Citation5]. Later, Hsu et al. discovered the existence of a circRNA in the cytoplasm of monkey renal CV-1 cells in 1979 by using electron microscopy [Citation6]. Recently, owing to the advent of RNA-Seq technology, numerous circRNAs were identified from various cancer types, such as breast cancer, ovarian cancer, laryngeal cancer, gastric cancer and bladder cancers [Citation7–10]. Up to now, more than 30,000 circRNAs have been reported in different mammalian cells [Citation11]. CircRNA is a kind of RNA that forms a covalently closed continuous loop [Citation12]. Theoretically, circRNAs may be beneficial from its circularization that it protects them from exonucleases and could impose structural constraints on the RNA in order to increase proper folding. The abundance and diversity of circRNAs suggest that some circRNAs will have important biological functions. For example, some studies have reported that circRNAs are closely related to Alzheimer’s disease, myotonic dystrophy, neoplasm and so on [Citation13–15].
In our previous study, thousands of distinct circRNAs had been identified between bladder cancer tissues and normal bladder tissues [Citation3]. In this study, we found that circCDYL is low expressed in bladder cancer. The previous study has reported that circCDYL can be used as a prognostic biomarker for patients diagnosed with early-stage bladder cancer [Citation16]. However, the role of circCDYL in bladder cancer is still unknown. Thus, in this study, we sought to explore the function and underlying mechanism of circCDYL in bladder cancer.
Materials and methods
Cell lines and treatment
The human bladder cancer cell line EJ and human immortalized uroepithelium cell line SV-HUC-1 were obtained from the American Type Culture Collection (ATCC, USA). The human bladder cancer cell line T24T was provided by Dr. Dan Theodorescu (Department of Urology, University of Virginia, USA) in 2010, which is a lineage-related lung metastatic variant of invasive bladder cancer cell line T24. All cells were cultured in RPMI-1640 medium (Gibico, USA) containing 10% FBS, at 37 °C supplied with 5% CO2.
Human tissue specimens
Thirty pairs of bladder cancer tissues and paired surrounding normal bladder tissues were collected from patients who underwent radical cystectomy at the Department of Urology of the Union Hospital of Tong Medical College between 2015 and 2018. All samples were immediately snap-frozen in liquid nitrogen after surgical removal. Histological and pathological diagnoses were assessed by at least two pathologists. There are no satellite tumors detected in surrounding normal bladder tissues. All specimens were classified according to the 2004 World Health Organization Consensus classification and staging system. Approval for the research was received from the Institutional Review Board of Tongji Medical College of Huazhong University of Science and Technology. Informed consent was obtained from the corresponding patients before surgery.
RNA extraction and real-time PCR assays
The nuclear and cytoplasmic RNAs were isolated according to the manufacturer’s instructions [Citation17]. Complementary DNA was synthesized using random primers and the reverse transcription kit Prime Script™ RT reagent Kit (Takara Biomedical Technology, China). Quantitative real-time PCR (qRT-PCR) analysis was carried out using the SYBR® Premix Ex Taq™ kit (Takara Biomedical Technology, China). The primer set for β-actin was 5′-TAGTTGCGTTACACCCTTTCTTG-3′ (forward) and 5’- TGTCACCTTCACCGTTCCAGT-3′ (reverse). The primer set for circCDYL was 5’ -CTTAGCTGTTAACGGGAAA-3′ (forward) and 5′-CTGTTGAAGTCGTGGATGT-3′ (reverse). The primer set for C-MYC was 5′-AGGGATCGCGCTGAGTATAA-3′ (forward) and 5′-TGCCTCTCGCTGGAATTACT-3′ (reverse). The primer set for GAPDH was 5′-TCAAGAAGGTGGTGAAGCAG-3′ (forward) and 5′-CGTCAAAGGTGGAGGAGTG-3′ (reverse). The primer set for Human U1 was 5′-ACTTACCTGGCAGGGGAGATACC-3′ (forward) and 5′-CCACTACCACAAATTATGCAGTCG-3′ (reverse). The qRT-PCR procedure was performed for 42 cycles of 95 °C for 5 s, 60 °C for 30 s, and the melting curve reaction was performed at the end. We used the Step One Plus Real-Time PCR System (Applied Biosystems, USA) for all data analyses. The ΔΔCT method was used to assess the relative expression of different candidate genes. All results were standardized to the expression of GAPDH, β–actin or U1 respectively.
CircRNA plasmids construction and transfection
To construct circCDYL over-expression plasmids, human circCDYL cDNA was synthesized by TSINGKE (China) and cloned into pcDNA3.1 (+) CircRNA Mini vector (Addgene, USA). The plasmids were transfected into cells lines by using Lipofectamine2000 (Life Technologies, USA) followed the manufacturer’s instructions. EJ and T24T cells were treated with the corresponding Plasmid or vectors and screened with G418 (Life Technologies) for 4–6 weeks.
Transwell migration assays
The migration assays were conducted by using a transwell chamber according to the manufacturer’s protocol (BD Science, Bedford, MA, USA). The homogeneous single cell suspensions (5 × 10 4 cells/well) were added to the upper chambers and incubated for 24 h. At least three random fields were calculated to quantify the migration rates.
Wound healing assay
EJ and T24T cells were cultured in six-well plates and collected with the fine end of 200 μl pipette tips (time 0 h). Cell migration was photographed at 0 and 24 h after injury, by using 10 high-power fields. Remodeling was measured as diminishing distance across the induced injury, standardized to the 0 h control, and recorded as relative migration.
CCK8 assays
Cells were cultured in 96-well plates coated with 10 μL of Cell Counting Kit-8 (Dojindo, Japan) per well and incubated at 37 °C supplied with 5% CO2 for 4 h. Next, the optical density was measured at a wavelength of 450 nm.
Flow cytometry assays for the cell cycle
EJ and T24T cells were treated with the corresponding Plasmid or vectors. The cells transfected circCDYL were screened with G418 (Life Technologies) for 4–6 weeks. After that, cells were harvested and stained with propidium iodide buffer (Sigma) for cell-cycle analysis according to the manufacturer’s instructions. The ModFit LT software was applied to analyze the results.
Western blotting analysis
Protein lysates were prepared with RIPA buffer (1×) (Thermo Scientific) containing a protease inhibitor cocktail (Roche, USA). A bicinchoninic acid (BCA) protein assay kit (Pierce, Thermo Scientific) was used to determine the concentration of protein samples. The lysates were separated by 12% SDS-PAGE gels and then were transferred to PVDF membranes. Tris-buffered saline (TBS) containing 5% nonfat milk was used to block the membranes for 1.5 h. And then probed with primary antibodies at 4 °C for 12 h. Primary antibodies included β-actin (Cat. No: 60008–1-Ig, 1:1000 dilution, Proteintech, USA), C-MYC (ab39688, 1:1000 dilution, Abcam), cyclin D1 (Cat. No: 60186–1-Ig, 1:1000 dilution, Proteintech, USA), p21 (Cat. No: 10355–1-AP, 1:1000 dilution, Proteintech, USA), p27 (Cat. No: 25614–1-AP, 1:1000 dilution, Proteintech, USA). After that, the concentration of protein samples was incubated with HRP-conjugated secondary goat anti-rabbit (Cat. No: SA00001-2) or anti-mouse (Cat. No: SA00001-1) antibodies (1:4000 dilution, Proteintech, USA) (Beyotime) for 2 h. Image J Software was used for data analysis after developing with the ECL kit (Beyotime Institute of Biotechnology).
Statistical analysis
All results were performed with SPSS 18.0 software (IBM, NY, USA), and with GraphPad Prism Version 7 (GraphPad Software). All data are shown as the mean ± standard error of the mean (SEM). Statistical significance was defined when p < .05.
Results
CircCDYL is derived from the exon 4 of CDYL and formed by a back-splice event
Firstly, in this study, we investigated the mechanism by which circCDYL is formed. Through an analysis of the CDYL gene, we found that the flanking introns of CDYL exon4 showed highly complementary Alu repeats, and two primate-specific Alu elements were in an inverted orientation (). The inverted repeated Alu elements (IRAlus) were highly reverse complementary (84% identity over 300 nt) (). Next, by using RT–PCR, we found that a single, distinct product of the expected size was amplified from EJ and T24T cells with specially designed divergent primers to circCDYL (). Subsequently, we confirmed the head-to-tail splicing in the RT–PCR product of circCDYL with expected size by Sanger sequencing (). Therefore, we can infer that circCDYL comes from the exon4 spliceosome-mediated pre-mRNA splicing where a downstream 5’ splice site (splice donor) is joined to an upstream 3’ splice site (splice acceptor).
Figure 1. CircCDYL is derived from the exon 4 (667 bp) of CDYL and formed by a back-splice event. (A) Schematic illustration showed the sequence of CDYL gene, and two primate-specific Alu elements were in an inverted orientation in the flanking introns of CDYL exon4. (B) Blast alignment showed the highly reverse complement of the inverted repeated Alu elements. (C) The existence of circCDYL was validated in two bladder cancer Cell lines EJ and T24T by RT–PCR. β-actin was used as negative control. (D) The head-to-tail splicing in the RT–PCR products of circCDYL with expected size were confirmed by Sanger sequencing. Red arrow represents ‘head-to-tail’ splicing sites of circCDYL.
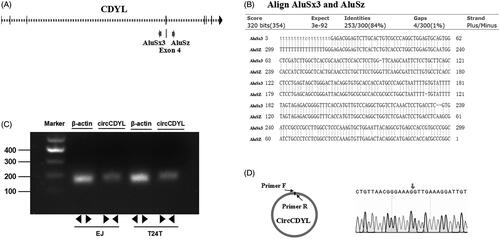
CircCDYL is lowly expressed in bladder cancer tissues and cell lines, and mainly localized in cytoplasm
Some studies reported that human circCDYL could be detected in the serum from tumor-bearing mice and was correlated with tumor mass [Citation18]. Besides, it can be used as a prognostic biomarker for patients diagnosed with early-stage bladder cancer [Citation16]. Firstly, in this study, the expression level of circCDYL was detected in 30 pairs of bladder cancer tissues and normal bladder tissues as well as bladder cancer cell lines by using quantitative RT–PCR, and the expression of circCDYL was obviously decreased in 70% of the bladder cancer tissues as compared with that in the normal bladder tissues (), and was negatively correlated with bladder cancer Pathological stage (p < .05, ). Down-regulation of circCDYL was also found in human muscle-invasive bladder cancer cells EJ and T24T compared with human immortalized uroepithelium cells SV-HUC-1 (). These results revealed a potential role of circCDYL in suppressing bladder cancer progression. By using the separation of nuclear and cytoplasmic RNA isolation, we demonstrated that circCDYL predominately localized in the cytoplasm both in EJ and T24T cell lines ().
Figure 2. The expression and subcellular location of circCDYL in human bladder cancer tissues and cell lines. (A) The expression of circCDYL was detected by quantitative real-time PCR in 30 pairs of bladder cancer tissues and normal bladder tissues. GAPDH was used as a negative control. Data are mean ± SEM, n = 3. **p < .05 (Student’s t-test). (B) The expression of circCDYL was tested by quantitative real-time PCR in SV-HUC-1, EJ and T24T cells. GAPDH was used as internal control. Data are mean ± SEM, n = 3. **p < .05 (Student’s t-test). (C,D) We detectec the isolation of nuclear and cytoplasmic RNA, circCDYL was mainly localized in the cytoplasm in both EJ and T24T cell lines. GAPDH and U1 were used as a negative control, respectively.
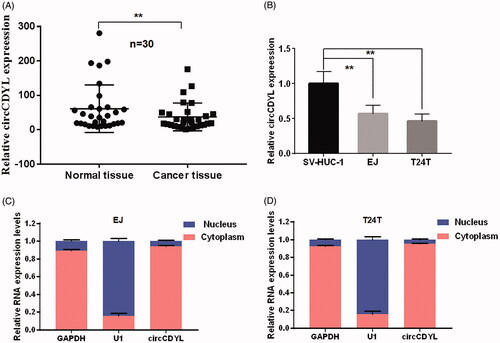
Table 1. Clinicopathological features of 30 bladder cancer patients and the expression of circCDYL.
Over-expression of circCDYL suppresses cell growth and migration in bladder cancer
Next, to explore the biological functions of circCDYL in bladder cancer cells, the circCDYL expression construct was transfected into EJ and T24T cells and the stable transfectants were established. The expression of circCDYL was significantly increased in these stable transfection cells (). CCK8 assay showed that over-expression of circCDYL significantly decelerated the growth rate of both EJ and T24T cells, especially after 72 h (). Besides, flow cytometry assay showed that enforced expression of circCDYL induced cell cycle arrest at G0–G1 phase in both EJ and T24T cells compared with negative control (). Moreover, cell migration abilities of EJ and T24T cells transfected with circCDYL were evaluated by wound healing assay and transwell migration assay, and the results indicated that circCDYL could suppress cell migration of bladder cancer cells ().
Figure 3. Over-expression of circCDYL inhibits cell growth in bladder cancer cell lines. (A) The expression levels of circCDYL in EJ and T24T cells after stable transfection of circCDYL or vector plasmids were detected by quantitative real-time PCR. Data are mean ± SEM, n = 3. **p < .05(Student’s t-test). (B,C) Stable transfection of circCDYL in EJ and T24T cellssignificantly decrease the proliferation. Data are mean ± SEM, n = 3. **p < .05, ***p < .01 (Student’s t-test). (D) Flow cytometry showed that circCDYL induced cell cycle arrest at G0–G1 phase in both EJ and T24T cells compared with negative control.
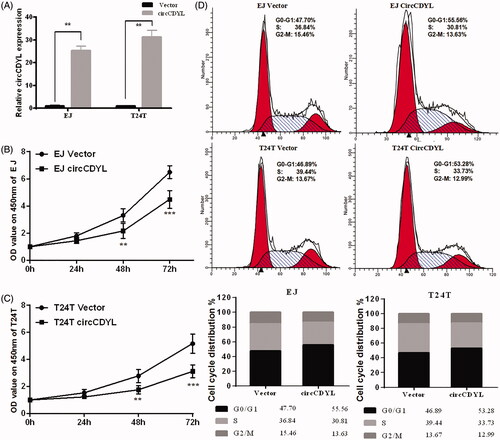
Figure 4. Over-expression of circCDYL inhibits cell migration in bladder cancer cell lines. (A) In wound healing assay, the migration of EJ and T24T cells transfected with circCDYL were significantly decreased. Data are mean ± SEM, n = 3. **p < .05 (Student’s t-test). Scale bar: 200 μm. (B) Transwell migration assays demonstrated that circCDYL decreased the invasion ability of EJ and T24T cells. Data are mean ± SEM, n = 3. **p < .05 (Student’s t-test). Scale bar: 100 μm.
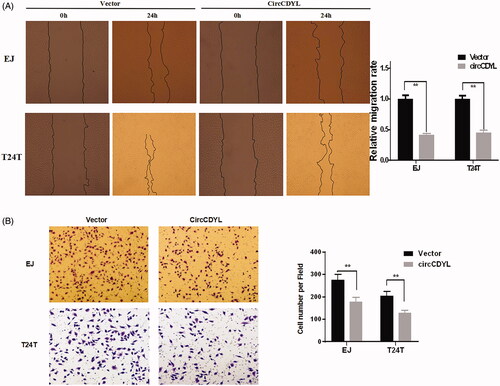
CircCDYL inhibits cell growth by down-regulating the protein level of C-MYC in bladder cancer
Next, we explored the underlying mechanism in circCDYL-regulated cell cycle arrest of bladder cancer cells. We found that the protein levels of C-MYC were decreased in both EJ and T24T cells that transfected with circCDYL, while P21, P27, CyclinD1 were not affected, as evaluated by Western blot analysis (). Meanwhile, the results of quantitative RT-PCR indicated that the mRNA levels of C-MYC were not changed (). As a key regulator of cellular proliferation, the transcription factor C-MYC [Citation19] was found upregulated in bladder cancer in our previous study [Citation20]. To address the role of C-MYC in circCDYL-regulated cell cycle arrest, we co-transfected C-MYC and circCDYL expression constructs into bladder cancer cells. It showed that the expression of C-MYC was significantly increased in the bladder cancer cells co-transfected with circCDYL and C-MYC plasmids, compared with the cells transfected with circCDYL alone (). Meanwhile, the cell-cycle assay indicated that over-expression of C-MYC could promote cell-cycle from G0–G1 phase to S phase, and co-transfected with C-MYC and circCDYL could partly reverse the G0/G1 phase cell cycle arrest induced by circCDYL in both EJ and T24T cells (). Taken together, these data suggested that circCDYL suppressed cell growth through inhibiting the protein expression of C-MYC.
Figure 5. CircCDYL inhibits cell growth by down-regulating the protein level of C-MYC in bladder cancer. (A) Western blot indicating that over-expression of circCDYL down-regulated the expression of C-MYC in EJ and T24T cells, while the expression of P27, P21 and CyclinD1 were not changed. (B) Quantitative real-time PCR indicating that the mRNA levels of C-MYC were not changed by circCDYL transfected both in EJ and T24T. (C,D) Western blot indicating that the expression of C-MYC was significantly increased in the bladder cancer cells co-transfected with circCDYL plasmids and C-MYC, compared with the cells transfected with circCDYL alone. (E) The cell-cycle assay showed that compared with empty vector group, over-expression of C-MYC could promote cell-cycle from G0–G1 phase to S phase, and co-transfected with C-MYC and circCDYL could partly reversed the G0/G1 phase cell cycle arrest induced by circCDYL in both EJ and T24T cells. Data are mean ± SEM, *p < .05 (Student’s t-test).
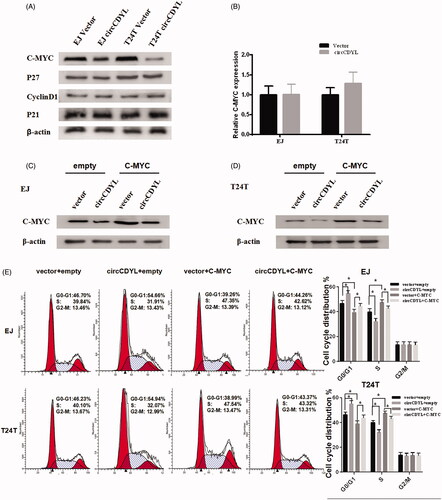
Discussion
Though it has been about 40 years since circRNAs in the cytoplasm of eukaryotic cells were observed [Citation6], it is not yet apparent what biological role the majority of circRNAs play. They were long considered as an accidental by-product, resulting from errors of posttranscriptional processing [Citation21]. However, increasing pieces of evidence indicated that circRNAs were mainly generated by a process called “backsplicing” [Citation7,Citation22,Citation23], and most of them were formed by circularization of exons [Citation24]. In addition, other studies identified another new class of intron-derived circular RNAs [Citation15]. For the biogenesis of exonic circRNAs, two main mechanisms involving the canonical spliceosome have been reported [Citation7,Citation25]. One is called that “direct backsplicing”, for which the 3’-end of the downstream exon as splice donor pairs with the 5’-end of the unspliced upstream exon as splice acceptor. After that, circRNA is generated by intervening RNA. The other is called “exon skipping”, where a lariat containing an exon is created by exon skipping. After that, the intron is removed and the exon is circularized [Citation10]. It has long been said that large exons were prone to be circularized because they were sterically easier for 30 to 50 splicing at canonical splice sites [Citation26]. The size of exon 4 of CDYL gene is 667 bp and blast alignment shows the flanking introns contain highly complementary Alu repeats to further promote its circularization. All these features indicate that circCDYL is formed by “direct backsplicing”.
As the most frequent amplified oncogene in human cancers, all three MYC family members (C-MYC, MYCN and MYCL1) have been the subjects of intense research for over 30 years involved in the etiology of human cancers [Citation27], which including lung, breast and prostate cancer [Citation28]. The C-MYC-induced genes play important roles in cell growth, cell cycle control, energy production, anabolic metabolism and DNA replication [Citation29]. Our previous study found that C-MYC was upregulated in bladder cancer cells [Citation20]. In this study, we found that circCDYL inhibited the bladder cancer cell growth by down-regulating the protein level of C-MYC, while the mRNA level of C-MYC was not significantly reduced. In view of C-MYC, rapidly degrading protein with a half-life is 20–30 min, a variety of different factors could interact with C-MYC to control its stability and transcriptional activity [Citation30], circCDYL may downregulate the protein level of C-MYC through promoting its degradation. However, the detailed mechanism involved in circCDYL-induced down-regulation of the protein level of C-MYC in bladder cancer still needs further study.
Some studies have shown that circRNAs contain microRNAs (miRNAs) response elements (MREs) and can sponge miRNAs that regulating target gene function [Citation10,Citation13,Citation24,Citation31,Citation32]. For example, it has been clearly demonstrated for CiRS-7, which function as miR-7 sponges to regulate the expression of miR-7 target mRNAs [Citation13]. Recently, it has been reported that circBIRC6 acts as the sponge of miR-34a and miR-145 to regulate target genes that maintain pluripotency [Citation33]. CircRNAs which act as a miRNA sponge have some common characteristics. Firstly, they derive from one or more exons of known protein-coding genes and formed by a back-splice event [Citation34]. Secondly, these circRNAs are predominantly localized in the cytoplasm, which occupies the same space of miRNA [Citation35]. In the present study, we found that circCDYL is derived from the exon 4 of CDYL through back-splicing and mainly localized in the cytoplasm. These findings suggest that circCDYL may act as a miRNA sponge to indirectly regulate the expression of C-MYC. However, not all circRNAs can act as “miRNA sponges” [Citation26]. Small sized circRNAs are too small to act as miRNA sponges, but they can be transported into exosomes and function as a bioindicator for cancer diagnosis [Citation10]. With little enrichment for miRNA target sites, intronic circRNAs and exon-intron RNAs, which mainly localize in the nucleus, have been reported to modulate their parental genes expression through specific RNA–protein interaction [Citation15,Citation36]. In addition, some circRNAs, such as cricFmn, circMbl and circDMD, can finally lead to the reduction in protein expression by binding to congeneric linear transcripts to segregate mRNA from transcription [Citation14,Citation26]. This process is also termed as “mRNA trap”. Thus, differentially expressed circRNAs in bladder cancer may have various functions and the detailed mechanism still needs further study beyond “miRNAs sponges”.
Taken together, we found that CircCDYL was derived from the exon 4 of CDYL and formed by a back-splice event in this study. Functionally, circCDYL could inhibit cell growth and migration in bladder cancer. Mechanistically, circCDYL induced cell cycle arrest through down-regulating the protein level of C-MYC in bladder cancer cells. Our findings provide a better understanding of detailed mechanisms activated during the growth and progress of bladder cancer.
Disclosure statement
The authors declare that they have no conflict interest that can inappropriately influence this work.
Additional information
Funding
References
- Parkin DM, Pisani P, Ferlay J. Global cancer statistics. Ca Cancer J Clin. 1999;49:33–64.
- Kaufman DS, Shipley WU, Feldman AS. Bladder cancer. Lancet 2009;374:239–249.
- Li B, Xie F, Zheng FX, et al. Overexpression of CircRNA BCRC4 regulates cell apoptosis and MicroRNA-101/EZH2 signaling in bladder cancer. Curr Med Sci. 2017;37:886–890.
- Kolakofsky D. Isolation and characterization of Sendai virus DI-RNAs. Cell 1976;8:547–555.
- Sanger HL, Klotz G, Riesner D, et al. Viroids are single-stranded covalently closed circular RNA molecules existing as highly base-paired rod-like structures. Proc Natl Acad Sci USA. 1976;73:3852–3856.
- Hsu MT, Coca-Prados M. Electron microscopic evidence for the circular form of RNA in the cytoplasm of eukaryotic cells. Nature 1979;280:339–340.
- Stefan S, Isabelle J, Oliver R, et al. Exon circularization requires canonical splice signals. Cell Reports 2015;10:103–111.
- Zhang Y, Liu H, Li W, et al. CircRNA_100269 is downregulated in gastric cancer and suppresses tumor cell growth by targeting miR-630. Aging 2017;9:1585.
- Ahmed I, Karedath T, Andrews SS, et al. Altered expression pattern of circular RNAs in primary and metastatic sites of epithelial ovarian carcinoma. Oncotarget 2016;7:36366–36381.
- Li Y, Zheng F, Xiao X, et al. CircHIPK3 sponges miR-558 to suppress heparanase expression in bladder cancer cells. Embo Rep. 2017;18:1646.
- Wang Y, Mo Y, Gong Z, et al. Circular RNAs in human cancer. Mol Cancer. 2017;16:25.
- Jingqiu L, Jie Y, Ping Z, et al. Circular RNAs in cancer: novel insights into origins, properties, functions and implications. Am J Cancer Res. 2015;5:472–480.
- Hansen TB, Jensen TI, Clausen BH, et al. Natural RNA circles function as efficient microRNA sponges. Nature 2013;495:384–388.
- Ashwal-Fluss R, Meyer M, Pamudurti NR, et al. circRNA biogenesis competes with pre-mRNA splicing. Mol Cell. 2014;56:55–66.
- Zhang Y, Zhang XO, Chen T, et al. Circular intronic long noncoding RNAs. Mol Cell. 2013;51:792–806.
- Okholm TLH, Nielsen MM, Hamilton MP, et al. Circular RNA expression is abundant and correlated to aggressiveness in early-stage bladder cancer. Npj Genom Med. 2017;2:36.
- Rio DC, Ares M, Hannon GJ, et al. Preparation of cytoplasmic and nuclear RNA from tissue culture cells. Cold Spring Harb Protoc. 2010;2010:pdb.prot5441.
- Gohji K, Okamoto M, Kitazawa S, et al. Heparanase protein and gene expression in bladder cancer. J Urol. 2001;166:1286–1290.
- Rahl PB, Lin CY, Seila AC, et al. C-MYC regulates transcriptional pause release. Cell 2010;141:432–445.
- Wu X, Liu D, Tao D, et al. BRD4 regulates EZH2 transcription through upregulation of C-MYC and represents a novel therapeutic target in bladder cancer. Mol Cancer Ther. 2016;15:1029–1042.
- Cocquerelle C, Mascrez B, Hetuin D, et al. Mis-splicing yields circular RNA molecules. Faseb J. 1993;7:155–160.
- Zhang XO, Wang HB, Zhang Y, et al. Complementary sequence-mediated exon circularization. Cell 2014;159:134–147.
- Yang W, Zefeng W. Efficient backsplicing produces translatable circular mRNAs. Rna-a Publication of the Rna Society 2015;21:172–179.
- Memczak S, Jens M, Elefsinioti A, et al. Circular RNAs are a large class of animal RNAs with regulatory potency. Nature 2013;495:333–338.
- Sonja P, Sabine M. RNA circularization strategies in vivo and in vitro. Nucl Acids Res. 2015;43:2454.
- Jeck WR, Sharpless NE. Detecting and characterizing circular RNAs. Nat Biotechnol. 2014;32:453–461.
- Conacci-Sorrell M, McFerrin L, Eisenman RN. An overview of MYC and its interactome. Cold Spring Harb Perspect Med. 2014;4:a014357.
- Beroukhim R, Mermel CH, Porter D, et al. The landscape of somatic copy-number alteration across human cancers. Nature 2010;463:899–905.
- Kress TR, Arianna S, Bruno A. MYC: connecting selective transcriptional control to global RNA production. Nat Rev Cancer. 2015;15:593.
- Herbst A, Salghetti SE, Kim SY, et al. Multiple cell-type-specific elements regulate Myc protein stability. Oncogene 2004;23:3863–3871.
- Han D, Li J, Wang H, et al. Circular RNA circMTO1 acts as the sponge of microRNA-9 to suppress hepatocellular carcinoma progression. Hepatology. 2017;66:1151.
- Xie F, Li Y, Wang M, et al. Circular RNA BCRC-3 suppresses bladder cancer proliferation through miR-182-5p/p27 axis. Molecular Cancer 2018;17(1):144.
- Yu CY, Li TC, Wu YY, et al. The circular RNA circBIRC6 participates in the molecular circuitry controlling human pluripotency. Nat Commun. 2017;8:1149.
- Yang C, Yuan W, Yang X, et al. Circular RNA circ-ITCH inhibits bladder cancer progression by sponging miR-17/miR-224 and regulating p21, PTEN expression. Molecular Cancer 2018;17:19.
- Zeng K, Chen X, Xu M, et al. CircHIPK3 promotes colorectal cancer growth and metastasis by sponging miR-7. Cell Death Dis. 2018;9:417.
- Li Z, Huang C, Bao C, et al. Exon-intron circular RNAs regulate transcription in the nucleus. Nat Struct Mol Biol. 2015;22:256–264.