Abstract
Mir-10b has been reported as a key regulator of metastasis in many human tumours. Moreover, it has also been regarded as a prognostic marker and therapeutic target of colorectal cancer (CRC). Whether miR-10b could affect the metastasis and proliferation of CRC is unclear. MiR-10b expression was detected by qPCR in human CRC tissues and cell line, Luciferase activity was employed for miR-10b binding to the 3`UTR of KLF4, Genes expression were examined by western blot, and mRNA by qPCR. PI and Annexin V staining were used to evaluate the cell cycle and apoptosis. Cell proliferation was detected with MTT, and cell migration and invasion were performed with Transwell assay. We found that miR-10b expression was up-regulated in metastatic CRC tissues and cell lines. Inhibition of miR-10b prevented cancer cell metastasis and growth by inducing cell-cycle arrest and apoptosis in vitro. Moreover, we found that KLF4 was a direct target of miR-10b. MiR-10b inhibitor led to the up-regulation of E-cadherin expression and the down-regulation of cyclin D1, which were partly abrogated after silencing KLF4.
Introduction
Colorectal cancer (CRC) is one of the most frequent malignancies in the world and is the third most common cancer in men and the second most common in women, it is almost 10% of the annual global cancer incidence and is increasing with the economic development. Moreover, the 5-year survival rate decreases with lower levels of income, with rates reaching 60% in high-income countries but falling to 30% or less in low–income countries[Citation1,Citation2]. The low survival rate is due to the metastasis of primary colorectal cancer, which usually moves to the liver, lung and other organs. Despite new methods for the diagnosis and treatment of metastasis, CRC was still associated with high mortality. The molecular mechanisms underlying the metastatic phenotype and progression of CRC have not been well elucidated.
MicroRNAs (miRNAs), a newly discovered class of non-protein-coding small RNAs, had been shown to play critical roles in physiological and pathological conditions, such as cell differentiation, proliferation, and more recently apoptosis [Citation2,Citation3]. Moreover, the aberrant expression of miRNAs had been linked to several human cancers. Increasing evidence had uncovered their functions as oncogenes and tumour suppressor genes [Citation4]. Recent work had revealed that a large set of miRNAs were overexpressed in human tumours as compared to normal tissues [Citation5,Citation6]. Certain miRNAs that function as oncogenes had been shown to promote carcinogenesis by targeting tumour suppressors, cell cycle regulators and proapoptotic genes, such as miR-543 [Citation5,Citation6]. Among all miRNAs, miR-10b was the first to be associated with metastasis of breast cancer, because it regulated extracellular matrix remodeling by targeting HOXD10 (Homeobox D10) in breast cancer cells [Citation7]. On the other hand, miR-10b could suppress migration of breast cancer cells by silencing TIAM1 (T-cell lymphoma invasion and metastasis [Citation8]. Furthermore, miR-10b also inhibited the expression of neurofibromin and subsequently inactivates the RAS signalling pathway [Citation9]. Together, miR-10b acted as an oncogene to regulate the function of cancer cells by targeting different genes in various cancers.
Krüppel-like factor 4 (KLF4; also called gut-enriched Krüppel-like factor) was a zinc finger-containing transcription factor that plays an important role in cell cycle, apoptosis, and differentiation [Citation10,Citation11]. KLF4 expression decreased in the multiple type intestinal adenoma and colonic adenomas of familial adenomatous polyposis patients and restoration of the KLF4 expression could inhibit the metastasis of lung cancer [Citation12,Citation13]. Moreover, KLF4 inhibited cell proliferation by inducing cell cycle arrest of CRC depending on targeting of the cdx gene [Citation14], and regulated oesophageal carcinogenesis by modulating proliferation, apoptosis, and cell invasion [Citation13,Citation15]. KLF4 was regarded as a target of miR-10b in oesophageal cancer to regulate migration and invasion of cancer cell [Citation15]. However, the molecular regulated mechanism of KLF4 in CRC is not known.
In this study, we applied a loss-of-function approach to identify the functional role of miR-10b in CRC migration, invasion, and proliferation. Additionally, we have identified KLF4 mRNA as a direct target of miR-10b in CRC. Our data supported that miR-10b was a global regulator of CRC cell proliferation and metastasis, and suggested that miR-10b may be a novel therapeutic target for the treatment of human CRC.
Materials and methods
Cells
The human CRC cell lines FHC (ATCC® CRL-1831), SW620 (ATCC® CCL-227), SW480 (ATCC® CCL-228), HT 29 (ATCC® HTB-38), HCT116 (ATCC® CCL-247), LoVo (ATCC® CCL-229), and Colo205 (ATCC® CCL-222) were purchased from American Type Culture Collection (ATCC) and cultured in RPMI-1640 (Invitrogen, CA, USA) with 10% fetal bovine serum (Gibco, CA, USA). Human tissue samples (16 non-metastasis and 14 metastasis colorectal cancer) were obtained from the Nanfang Hospital in Southern Medical university in 2013–2014. Our project was approved by the ethics committee of the Nanfang Hospital and written informed consents were obtained from all the patients.
Transfection
miR-10b inhibitor and KLF4 siRNA were purchased from Genepharma (Shanghai, China). The pcDNA3.1 (referred as “vector”) and pcDNA3.1-KLF4 (over-expression of KLF4, referred as “KLF4”) were generous gifts from Professor Zhihua Liu at the Cancer Institute of the Chinese Academy of Medical Sciences (Beijing, China) [Citation16].
RNA isolation and quantitative real-time PCR
Total RNA was extracted using Trizol reagent (Invitrogen, CA, USA). RNA was reversely transcribed to cDNA using Thermoscript RT system reagent (Invitrogen, CA, USA) following the instructions.
qRT-PCR was performed to quantitate RNA levels in CRC tissues and cells. miR-10b expression was detected using a Hairpin-itTM miRNAs qPCR kit (Genepharma, Shanghai, China), with RNU6B as an endogenous control. KLF4 expression was measured with SYBR green qPCR assay (Takara, Dalian, China) and GAPDH was detected as an internal control. The sequences of the primers are as follows: miR-10b forward: 5′-GGATACCCTGTAGAACCGAA-3′, miR-10b reverse: 5′-CAGTGCGTGTCGTGGAGT-3′, KLF4 forward: 5′-CGAA CCCACACAGGTG-AGAA-3′, KLF4 reverse: 5′-TACGGTAGTGCCTGGTCAGTTC-3′, GAPDH forward: 5′-GACCACAGTCCATGCCATCAC-3′, GAPDH reverse: 5′-CCACCA-CCCTGTTGCTGTA-3’.
Cell invasion and migration assays
The invasive and migratory potentials of cells were evaluated using Transwell inserts with 8 μm pores (Corning, NY, USA) according to the instructions. 48 h after transfection, three random fields at 100× magnification for each insert were counted. Inserts were conducted in triplicate in three separate experiments.
Cell proliferation assay
Cell proliferation was determined using the Cell Counting Kit-8 (CCK-8) (Beyotime, Hangzhou, China) according to the manufacturer’s instructions. Briefly, 2 × 103 cells/well were seeded in 96-well plates. Subsequently, 48 h after transfection, 10 μl CCK-8 solutions were added to each well. The cells were then incubated at 37 °C and the absorbance was determined at 450 nm using a spectrophotometer (MDC, USA) at 2.0 h.
Cell cycle and apoptosis analysis
The cells were trypsinized and pelleted by centrifugation. After washing the pellet with PBS, the cells were counted and stained according to the instructions of Annexin V-FITC Kit (BD, USA) and Cell Cycle Detection Kit (KeyGen, China), respectively. The suspended cells were analyzed for apoptosis and cell cycle using FACS Calibur Flow Cytometer (BD, USA).
Western blot
The cells were washed twice with ice-cold PBS, then were lysed in the lysis buffer (Beyotime Inst Biotech) which contained 1 mM phenylmethylsulfonyl fluoride (PMSF) (Sigma, USA). Equal aliquots of total cell lysates (20–30 μg) were separated by SDS-PAGE and then transferred to PVDF membranes (Millipore, Bedford, MA). The membranes were blocked in 5% non-fat milk in TBS-T containing 0.05% Tween-20. The membranes were then probed with the appropriate primary antibodies, followed by a secondary horseradish peroxidase-conjugated antibody. The protein bands were developed with ECL solution (Beyotime Inst Biotech). The following antibodies were used: primary antibodies against E-cadherin and cyclin D1 were purchased from Santa Cruz Biotechnology (USA); primary antibodies against p53 and GAPDH were purchased from Cell Signaling Technology (USA); the primary antibody against KLF4 was purchased from Abcam (Cambridge, UK).
Luciferase reporter assay
The cells were transiently cotransfected in 96-well plates with a luciferase reporter vector containing 3’-UTR variants [Citation15] and either control or miR-10b inhibitors. After 48 h, luciferase activity was measured with Dual-Glo Luciferase Assay System (Promega, WI, USA), and Renilla luciferase activity was normalized to Firefly luciferase activity.
Statistical analysis
All data from three independent experiments were expressed as mean ± SD and processed using SPSS 13.0 (Armonk, NY, USA). The difference between the two groups or among more than two groups was estimated by Student’s t-test or 1-way ANOVA, respectively. A P-value of <.05 was considered statistically significant.
Results
miR-10b is highly expressed in metastatic CRC tissues and cell lines
Compared with non-metastatic CRC samples, the level of miR-10b was much higher in the metastatic samples (P < .001) (). In the six cell lines and normal mucus cells, miR-10b levels varied considerably. Generally, compared with the normal mucus cells, the metastatic cells SW620, colo205 and HT29 expressed notably higher levels of miR-10b (P < .05, ).
Figure 1. Expression of miR-10b in both CRC tissues and CRC cell lines. (A) miR-10b was overexpressed in metastatic CRC samples (n = 14), compared with non-metastatic ones (n = 16). (B) The expression of miR-10b in 6 CRC cell lines and normal mucosa cells was different from each other. Cell results shown are representative of three independent experiments. Data are given as means ± SD.
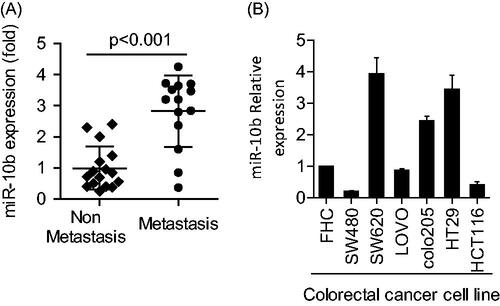
miR-10b inhibition prevents the invasion, migration and proliferation of CRC cells
We selected high expression of miR-10b in colon cancer cells, SW620 and HT29, with transfection of miR-10b inhibitor Compared with control cells, silencing miR-10b increased the expression of E-cadherin, but decreased the expression of Cyclin D1 and Snail (). Furthermore, silencing miR-10b led to significant reduction in invasion and migration of SW620 cells, and suppressed cell proliferation (). On the contrary, overexpression of miR-10b promoted the migration, invasion and proliferation in SW480 (Supplementary Figure 1(A–C)). These results indicated the promoting role of miR-10b in the metastasis and proliferation of CRC cells.
Figure 2. Effects of miR-10b on metastasis, proliferation, apoptosis and cell-cycle of SW620 cells. (A) the expression of E-cadherin increased after treatment of miR-10b inhibitor, but the expression of cyclin D1 and Snail protein decreased. (B,C) Downregulation of miR-10b notably inhibited cell migration and invasion of SW620 cells (100× magnification). (D) Transfecting miR-10b inhibitors significantly reduced proliferation of SW620 cells (*p < .05). (E) miR-10b inhibition leads to cell-cycle arrest in SW620 cells. Cell cycle was stained with PI and analyzed by flow cytometer after 2 days following transfections in SW620 cells. (F) Inhibition of miR-10b expression enhanced apoptosis. Cells were stained with PI and Annexin V staining, and analyzed by flow cytometer after 2 days following transfections. Cell results shown are representative of three independent experiments. Data are given as means ± SD.
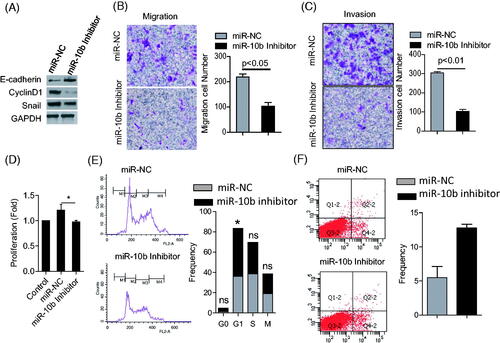
miR-10b inhibition induces cell cycle arrest and apoptosis
To further validate the effect of miR-10b on proliferation in CRC cells, we performed cell cycle and apoptosis analyses. Flow cytometry showed that SW620 cells underwent cell cycle arrest at the G1 phase following miR-10b inhibition. Compared to the control group, the proportion of cells that were positive for Annexin V was much higher after silencing miR-10b (). Therefore, miR-10b inhibition blocks the progression of cell cycle in CRC and induces programmed cell death, implying the involvement of this miRNA in proliferation.
KLF4 is a direct target of miR-10b
Like the previous report, microRNA needed to be combined with the targeting gene, which helps to effectively play on its function. Thus, to explore the mechanism of miR-10b in CRC, we utilized PicTar as our bioinformatic target prediction algorithm to identify new targets. We were particularly interested in targets that belonged to cell cycle- and apoptosis-related gene ontology (GO) terms, and focused on KLF4, as its expression was found to be progressively lost in CRC [Citation17], as well as in oesophageal cancer. We constructed Renilla luciferase reporters containing either wild type or mutated 3’-UTR of the KLF4 gene to validate whether KLF4 is a direct target of miR-10b (). MiR-10b inhibitor induced the expression of KLF4 through both transcriptional and translational regulation (). The reporter containing the wild-type 3’-UTR showed notable derepression in response to inhibition of miR-10b (), indicating that miR-10b regulates KLF4 expression through its 3’-UTR. Mutations within the miR-10b binding site of KLF4 3’-UTR partially abrogated the responsiveness of the corresponding reporters to the miR-10b inhibitor. We also performed this assay in HEK293 cell line by cotransfecting with miR-10b mimic and KLF4 3’-UTR or KLF4 3’-UTR-mut plasmid. The results showed that miR-10b mimic obviously inhibited the luciferase activity in KLF4 3’-UTR plasmid but not in KLF4 3’-UTR-mut plasmid ().
Figure 3. The KLF4 3’-UTR is a target of miR-10b. (A,B) KLF4-encoded mRNA contains a 3`- UTR which perfectly matches to the seed region of miR-10b. Plasmid with the full-length KLF4-3’- UTR insert and plasmid with a mutant KLF4-3`- UTR (KLF4-3`UTR-mut). (B) klf4 mRNA was detected by qPCR after the treatment of miR-10b inhibitor in SW620. (C) KLF4 expression was detected by western blot after the treatment of miR-10b inhibitor in SW620 and HT29. (D) The luciferase activity was measured with transfection of miR-10b inhibitor and KLF4-3`UTR or KLF4-3`UTR-mut plasmid in HT29. (E) The luciferase activity was measured with transfection of miR-10b mimic and KLF4-3`UTR or KLF4-3`UTR-mut plasmid in HEK293T cell line. Renilla luciferase activity was normalized to Firefly luciferase activity. Cell results shown are representative of three independent experiments. Data are given as means ± SD.
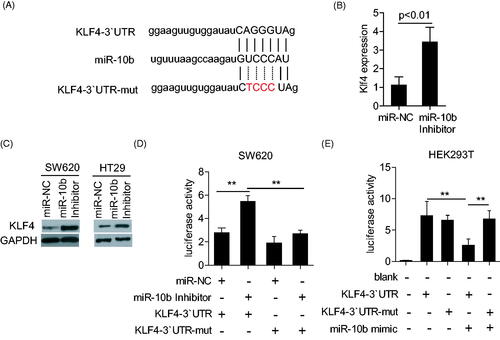
KLF4 inhibition partially abolishes the regulatory function of miR-10b on metastasis and proliferation of CRC cells
The results above showed that miR-10b inhibition increased the KLF4 level, thus we investigated the effect of overexpressing KLF4 on the metastasis and proliferation of SW620 and HT29 cells. Overexpression of KLF4 resulted in a significant inhibition in cell proliferation, migration and invasion in SW620 and HT29 cells (; Supplementary Figure 2(A–C)), which demonstrated that KLF4 is a negative regulator of proliferation and metastasis of CRC cells.
Figure 4. Effects of KLF4 on metastasis, proliferation, apoptosis and cell cycle of SW620 cell lines. (A) Over-expression of KLF4 greatly inhibited cell migration and invasion of SW620 cells (100× magnification). (B) Transfecting KLF4 plasmids significantly reduced proliferation of SW620 cells (*P < .05). (C) Over-expression of KLF4 leads to cell-cycle arrest in SW620 cells. (D) Over-expression of KLF4 enhanced apoptosis. SW620 cells were analyzed with PI and Annexin V staining by flow cytometer after 3 days following transfections. Cell results shown are representative of three independent experiments. Data are given as means ± SD.
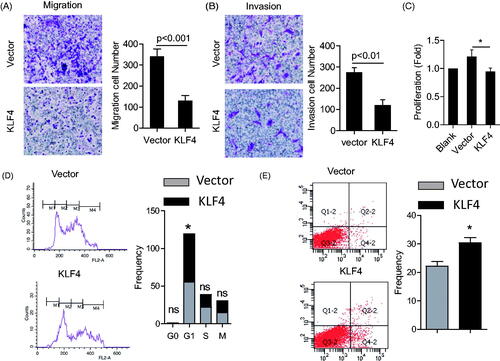
KLF4 is necessary of the regulatory of miR-10b to the metastatic and proliferation in colon cancer cell
To further confirm the relationship between miR-10b and KLF4, we tested whether KLF4 was required for the function of miR-10b by co-transfecting KLF4 siRNA and miR-10b inhibitor into SW620 cells. Silencing miR-10b increased the expression of E-cadherin, and decreased the expression of Cyclin D1 and Snail, but co-transfecting KLF4 siRNA rescued the expression of E-cadherin, cyclin D1 and Snail (). Surprisingly, the inhibition of metastasis, proliferation () and the induction of cell cycle arrest and apoptosis () resulting from miR-10b inhibitior were partly attenuated by silencing KLF4. These results indicate that KLF4 may serve as a target of miR-10b, contributing to the effect of miR-10b on colon cancer cell metastasis, cell proliferation, cell cycle arrest and apoptosis.
Figure 5. KLF4 Partly attenuated the function of miR-10b. SW620 cells were transfected with siRNA for KLF4 mRNA and miR-10b inhibitor. (A) KLF4, E-cadherin, cyclinD1 and Snail expression were detected by western blot. (B–D) Then migration and invasion assays were evaluated by Transwell and the proliferation was detected by MTT. Cell results shown are representative of three independent experiments. Data are given as means ± SD.
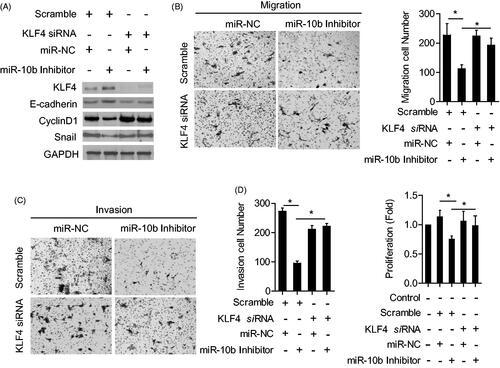
Figure 6. KLF4 Partly attenuated the function of miR-10b on cell cycle and apoptosis of CRC cells. SW620 cells were transfected with siRNA for KLF4 mRNA and miR-10b inhibitor. Then migration and invasion assays were performed to evaluate the effect of KLF4 on the function of miR-10b. (A,B) cell cycle and (C,D) apoptosis assays were also conducted. Cell results shown are representative of three independent experiments. Data are given as means ± SD.
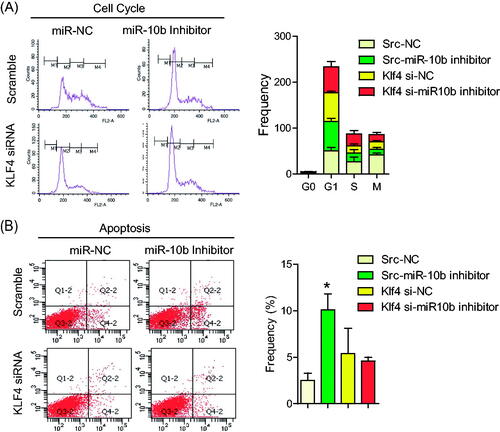
Discussion
In this study, we have shown, for the first time, that miR-10b is highly expressed in metastatic CRC tissue and cell lines. Its inhibition attenuates metastasis and reduces cell growth. Additionally, we have identified KLF4 mRNA as a direct and functional target of miR-10b. Finally, we revealed that miR-10b appears to modulate several independent signalling pathways that involve EMT, cell cycle and apoptosis by targeting KLF4.
Recent researches have identified many targets that are regulated by miR-10b. Among them, HOXD10 and RhoC, which function in metastasis, have been studied extensively [Citation7]. Moreover, serial evidence has indicated that KLF4 plays an inhibitory role in metastasis, proliferation and cell cycle progression of CRC. Taken together, these observations demonstrate that KLF4 acts as a suppressor in metastasis and proliferation of cancer cells, which is opposite to the promoting role of miR-10b. Like oesophageal cancer or others, the 3’-UTR of KLF4 mRNA perfectly matches the seed region of miR-10b and assisted in the miR-10 function.
We further explain the mechanism of miR-10b to cell proliferation in CRC. Cyclin D1 is one of the most important regulators of the cell cycle, because its degradation leads to G1 cell cycle arrest and inhibits cell growth [Citation18,Citation19]. Our data showed that the inhibition of miR-10b was sufficient to cause the down-regulation of cyclin D1 expression, and this reflects the potential of miR-10b in promoting cell cycle through KLF4 mediated signalling pathway as described in .
Then, the effect of miR-10 on the metastasis of CRC is to be known. As the initial events for cancer metastasis, EMT can promote the hallmark features of carcinoma associated with poor histologic differentiation, destruction of tissue integrity and metastasis [Citation20]. E-cadherin has been regarded as a master regulator of the epithelial/mesenchymal phenotype switch during EMT. Repression of E-cadherin is sufficient to induce and complete EMT. As reported, the expression of miR-10b was induced by the transcription factor Twist [Citation7], a factor that promoted epithelial-mesenchymal transition (EMT) [Citation21]. It meant that miR-10b could play a vital role in colorectal cancer metastasis. In our study, after alone or/and together transfecting with miR-10b inhibitor and KLF4 siRNA, the changes of E-cadherin, and Snail were all attenuated. At the same time, cell metastasis ability was also receded. Collectively, miR-10b/KLF4 signalling pathway was regarded to be involved in regulating the EMT of CRC by alerting the expression of E-cadherin and Snail.
In conclusion, these results demonstrate the pivotal role of miR-10b in the metastasis and proliferation of CRC cells, and support that miR-10b may be a novel therapeutic target for human colorectal cancer.
ABBREVIATIONS | ||
CRC | = | colorectal cancer |
KLF4 | = | Krüppel-like factor 4 |
HODX10 | = | Homeobox D10 |
TIAM1 | = | T-cell lymphoma invasion and metastasis 1 |
PMSF | = | phenylsulfony1 fluoride |
CCK-8 | = | Cell count kit-8 |
EMT | = | mesenchymal-epithelial transition |
EMT | = | epithelial-mesenchymal transition |
qPCR | = | Quantitative real-time polymerase chain reaction |
PI | = | Propidine iodide |
Acknowledgements
We are grateful to Professor Zhihua Liu (Cancer Institute of the Chinese Academy of Medical Sciences, Beijing, China) for providing pcDNA3.1 and pcDNA3.1-KLF4 plasmids. We thank Dr. Qunying Ma and Dr. Wenjing Zhang from our laboratory for technical assistance in cell invasion and migration.
Disclosure statement
No potential conflict of interest was reported by the authors.
Additional information
Funding
Reference
- Arnold M, Sierra MS, Laversanne M, et al. Global patterns and trends in colorectal cancer incidence and mortality. Gut 2017;66:683–691.
- Ferlay J, Colombet M, Soerjomataram I, et al. Cancer incidence and mortality patterns in Europe: estimates for 40 countries and 25 major cancers in 2018. Euro J Cancer. 2018;103:356–387.
- Bartel DP. MicroRNAs: genomics, biogenesis, mechanism, and function. Cell 2004;116:281–297.
- Baffa R, Fassan M, Volinia S, et al. MicroRNA expression profiling of human metastatic cancers identifies cancer gene targets. J Pathol. 2009;219:214–221.
- Kabekkodu SP, Shukla V, Varghese VK, et al. Clustered miRNAs and their role in biological functions and diseases. Biol Rev. 2018;93:1955–1986.
- Zhai F, Cao C, Zhang L, et al. miR-543 promotes colorectal cancer proliferation and metastasis by targeting KLF4. Oncotarget 2017;8:59246–59256.
- Ma L, Teruya-Feldstein J, Weinberg RA. Tumour invasion and metastasis initiated by microRNA-10b in breast cancer. Nature 2007;449:682–688.
- Moriarty CH, Pursell B, Mercurio AM. miR-10b targets Tiam1: implications for Rac activation and carcinoma migration. J Biol Chem. 2010;285:20541.
- Chai G, Liu N, Ma J, et al. MicroRNA-10b regulates tumourigenesis in neurofibromatosis type 1. Cancer Sci. 2010;101:1997–2004.
- Ghaleb AM, McConnell BB, Kaestner KH, et al. Altered intestinal epithelial homeostasis in mice with intestine-specific deletion of the Kruppel-like factor 4 gene. Develop Biol. 2011;349:310–320. PubMed PMID: 21070761;
- Yu T, Chen X, Zhang W, et al. Kruppel-like factor 4 regulates intestinal epithelial cell morphology and polarity. PloS One. 2012;7:e32492.
- Li S, Huang L, Gu J, et al. Restoration of KLF4 Inhibits Invasion and Metastases of Lung Adenocarcinoma through Suppressing MMP2. J Cancer. 2017;8:3480–3489.
- Dang DT, Bachman KE, Mahatan CS, et al. Decreased expression of the gut-enriched Kruppel-like factor gene in intestinal adenomas of multiple intestinal neoplasia mice and in colonic adenomas of familial adenomatous polyposis patients. FEBS Lett. 2000;476:203–207.
- Dang DT, Mahatan CS, Dang LH, et al. Expression of the gut-enriched Kruppel-like factor (Kruppel-like factor 4) gene in the human colon cancer cell line RKO is dependent on CDX2. Oncogene 2001;20:4884–4890.
- Tian Y, Luo A, Cai Y, et al. MicroRNA-10b promotes migration and invasion through KLF4 in human esophageal cancer cell lines. J Biol Chem. 2010;285:7986–7994.
- Zhou Q, Hong Y, Zhan Q, et al. Role for Kruppel-like factor 4 in determining the outcome of p53 response to DNA damage. Cancer Res. 2009;69:8284–8292.
- Zhao W, Hisamuddin IM, Nandan MO, et al. Identification of Kruppel-like factor 4 as a potential tumour suppressor gene in colorectal cancer. Oncogene 2004;23:395–402.
- Hayakawa Y, Hirata Y, Nakagawa H, et al. Apoptosis signal-regulating kinase 1 and cyclin D1 compose a positive feedback loop contributing to tumour growth in gastric cancer. Proc Nat Acad Sci USA. 2011;108:780–785.
- Legge DN, Shephard AP, Collard TJ, et al. BCL-3 promotes a cancer stem cell phenotype by enhancing beta-catenin signalling in colorectal tumour cells. Disease Models & Mechanisms 2019;12:dmm037697.
- Lino-Silva LS, Salcedo-Hernandez RA, Gamboa-Dominguez A. Tumour budding in rectal cancer. A comprehensive review. Contemp Oncol. 2018;22:61–74.
- Yang J, Mani SA, Donaher JL, et al. Twist, a master regulator of morphogenesis, plays an essential role in tumour metastasis. Cell 2004;117:927–939.