Abstract
Today, metal-organic frameworks (MOFs) for the release of drugs have been studied extensively. In this research, we have investigated the novel magnetic framework including Fe3O4@PAA@ZIF-8 for the delivery of ciprofloxacin (CIP) antibiotic. Moreover, the antibacterial activity of Fe3O4@PAA@ZIF-8 and Fe3O4@PAA@ZIF-8@CIP screened against Staphylococcus aureus (S. aureus) and Escherichia coli (E. coli) bacteria. The structure of the nano carrier, as well as the encapsulation of the drug in the framework was confirmed by the Fourier-transform infrared spectroscopy (FT-IR), Ultraviolet–visible spectroscopy (UV–Vis), Energy-dispersive X-ray spectroscopy (EDX), X-ray crystallography (XRD), Scanning electron microscope (SEM) and Brunauer–Emmett–Teller (BET) analysis. The results of this study showed that 93% of the CIP was loaded on the synthesized framework. Drug release was done at pH: 7.4 and pH: 5 within 2 d, resulting about 73% release of the drug.
Introduction
The traditional drug delivery system does not meet the medical needs of the modern world as a result modern drug delivery systems have emerged today. In modern drug delivery systems, three factors can be controlled in contrast to the traditional drug delivery system which is including 1: time, 2: place and 3: speed drug release. For this reason, the system is called a targeted drug delivery system. The basis of this system is the insertion of some of the desired drug into the carrier, which carries the drug into the target tissue and begins to release after it is placed in the intended place [Citation1–3]. Over the past few years, numerous compounds have been investigated, such as micelles [Citation4], liposomes [Citation5], grapheme [Citation6] and hydrogels [Citation7] as drug carriers, which have some disadvantages and advantages.
In recent years, new porous compounds called metal-organic frameworks (MOFs) have been noticed as the carrier of drugs.
The MOFs are composed of metal ions and organic ligands. Metal structures such as Zr2+, Zn2+, Fe2+ and Cu2+ and ligands such as terephthalic acid and 2-methylimidazole (2-Melm) are used to construct the MOFs, that can have a one, two and three-dimensional structure. They have special features such as high porosity, adjustable size and high loading capacity, which make it possible to use these frameworks in therapeutic projects such as drug delivery. Different methods such as temperature response, response to pressure and response to pH are used to release the drug depending on the framework [Citation8,Citation9]. The first framework which used as a carrier for medication was MIL-100 that was used as a carrier for ibuprofen release [Citation10]. Recently, other frameworks such as UiO-66 [Citation11], MIL-53 [Citation12] and ZIF-8 [Citation13] were made for carrying anti-cancer and anti-inflammatory drugs such as doxorubicin [Citation14], 5-fluorouracil [Citation15] and ibuprofen [Citation16]. In a number of studies, the level of MOFs and other carriers has been modified using natural and synthetic polymers, including polyacrylic acid (PAA) [Citation17] and poly dopamine [Citation18], which has shown good results for drug delivery.
Zeolithicimidazole frameworks (ZIFs) are a group of highly porous and three-dimensional crystalline frameworks with good properties, including high levels and thermal stability and is a pH-sensitive framework that is ideal for transferring the drug [Citation19].
MOFs that contain paramagnetic metal ions are used as nuclei in their structure for magnetic imaging and targeted magnetic transfer, which improves the efficiency of the treatment. Iron oxide nanoparticles (Fe3O4) are often used as nuclei in structures that improve drug loading and framework compatibility [Citation20,Citation21].
Ciprofloxacin (CIP) is a fluoroquinolone antibiotic used to treat skin infections, bone and joint infections and respiratory infections (. CIP, after penetrating into the cell’s membrane, stops DNA synthesis by preventing the effect of DNA gyrase in the bacteria. As a result, when the DNA opens for replication, it will lack the enzyme to rotate and screw again and it will disappear [Citation22].
Considering the importance of the above-mentioned researches related to the need of modern targeted drug delivery and in continuation of our studies in order to synthesis biologically interesting products using nanostructures [Citation23–26], herein we were able to report a novel and effective metal-organic framework Fe3O4@PAA@ZIF-8 as an astonishing carrier for the drug delivery of CIP. In addition, antibacterial properties of drug-loaded nanostructures were compared with pure CIP against Escherichia coli and Staphylococcus aureus bacteria by disk diffusion method and showed excellent and significant results (Scheme 1).
Experimental section
Materials
2-Melm, zinc nitrate hexahydrate (Zn (NO3)2.6H2O), ferric chloride (FeCl3.6H2O), PAA (Mw ≈ 3000), sodium acetate (NaAc) and ethylene glycol (EG) are purchased from Merck (Kenilworth, NJ).
Characterization
FT-IR spectra were recorded on Magna-IR spectrometer 550. Powder X-ray diffraction (XRD) was carried out on a Philips diffractometer of X’pert Company with mono chromatized Cu Kα radiation (λ = 1.5406 Å). Microscopic morphology of products was visualized by scanning electron microscopy (SEM) (LEO 1455VP) . The compositional analysis was done by energy dispersive analysis of X-ray (EDX, Kevex (Newark, DE) Delta Class I). Nitrogen adsorption–desorption isotherms were measured at 196 °C using a Belsorp mini automatic adsorption instrument after degassing the samples at 150 °C for 5 h. The approximate sample weight was 10 mg in TG experiment with 10 °C/min heating rate. Absorption spectra were recorded in the range 200–800 nm on a Shimadzu model 1601 PC UV–visible spectrophotometer (Shimadzu, Tokyo, Japan).
Preparation of Fe3O4-PAA
Fe3O4-PAA was prepared according to the previous literature by Jin et al. with some modifications [Citation27]. In a typical procedure, to a mixture of FeCl3.6H2O (0.75 g) in ethylene glycol (40 ml) was added PAA (0.5 g). The solution was stirred for 30 min, after this time, 2 g of NaAc was added to the solution and the mixture was transferred to 50 ml teflon-lined stainless-steel autoclave for a period of 24 h at 220 °C. The obtained Fe3O4-PAA was washed three times with deionized water and ethanol and finally was dispersed in 40 ml of ethanol.
Synthesis of ZIF-8 functionalized Fe3O4@PAA
Briefly, 0.5 g of Zn(NO3)2.6H2O and 0.25 g of 2-Melm were dissolved in ethanol (30 ml). Then, 10 ml of the prepared Fe3O4@PAA solution was added to it. This solution was stirred at 80 °C for 2 h and finally, it was washed with ethanol and dried at 100 °C.
Loading CIP on the Fe3O4@PAA@ZIF-8
Drug loading of ZIF-8 functionalized Fe3O4@PAA was done as follows: 0.1 g of CIP was dissolved in 5 ml of deionized water which continued by addition of Fe3O4@PAA@ZIF-8 (0.05 g). This solution was stirred at room temperature for 5 d. The final product was washed three times with methanol to remove unloaded drugs and then it was dried at 60 °C.
Drug release from Fe3O4@PAA@ZIF-8
The release of the drug was performed in two buffers, buffer acetate (pH: 5) and phosphate buffer saline (pH: 7.4). In this procedure, 0.02 g of Fe3O4@PAA@ZIF-8@CIP was added to 50 ml of each buffer separately. Then, the solution was stirred at 37 °C for 3 d. Each time, 5 ml of the solution were removed and quickly replaced with the same amounts of fresh buffer. The amount of drug released from MOF was investigated by UV–Vis spectrometer at 323 nm.
Antibacterial activity
S. aureus and E. coli were used as standard strains in this study. To determine the susceptibility of the microorganisms, antibiotics were used from Muller–Hill Agar culture media [Citation28]. Initially, a concentration of 10 μg/ml of the substance was prepared in 0.01 mol/ml of HCl. After culturing bacteria, the plates were placed at 37 °C for one night. The disks are impregnated in a volume of 10 μl of MOF, MOF@CIP and CIP. Finally, the discs were placed in the medium and placed in an incubator for one night at 35 °C.
Results and discussion
The FT-IR spectra of Fe3O4@PAA, Fe3O4@PAA@ZIF-8 and Fe3O4@PAA@ZIF-8@CIP are shown in . In FT-IR spectrum of Fe3O4@PAA (), the absorption band of 515 cm−1 is related to the stretching vibration of Fe-O. The peaks which observed at 1566 cm−1 and 1412 cm−1 are related to stretching vibration of C=O and C-O of PAA, respectively. Furthermore, the stretching vibration of OH group of PAA has appeared at 3460 cm−1. FT-IR spectrum of Fe3O4@PAA@ZIF-8 shows 2 peaks at 3128 cm−1 and 2925 cm−1 corresponding to the stretching vibration of C-H aromatic and aliphatic bonds [Citation29] (). Moreover, the peak at 1574 cm−1 is related to the stretching vibration of the C=N bond of imidazole ring [Citation30]. The available peak in 553 cm−1 is also for the Fe-O vibrating bond. The stretching vibration of Zn-N bond is appeared at 426 cm−1. The spectrum is shown in (Fe3O4@PAA@ZIF-8@CIP), confirms the presence of CIP in the MOF. The peak of the OH group of CIP carboxylic acid has appeared in the range of 2565–3425 cm−1. The carboxylate group of CIP shows a medium band at 1385 cm−1, and the strong peak at 1628 cm−1 refers to the stretching vibration of the carbonyl group of the drug.
The XRD patterns of Fe3O4@PAA@ZIF-8 and Fe3O4@PAA@ZIF-8@CIP confirmed the formation and maintenance of crystallite structure of magnetite nanoparticles (. The diffraction peaks in , almost confirms the crystallinity of the Fe3O4 after functionalization with PAA and ZIF-8. The obtained results are approximately the same as pure Fe3O4 peaks [Citation31]. Also, after loading the drug in the framework, the crystalline structure of Fe3O4 is preserved ().
By using SEM, the morphology of the particles can be determined. SEM images for the nanocarrier before and after drug loading are shown in . shows the image of the drug CIP in which the drug particles have a rod shape. SEM image of Fe3O4@PAA@ZIF-8 shows Fe3O4 nanoparticles with spherical shapes on the surfaces of PAA@ZIF-8 (). In , the structure of the drug rod is observed, and it has also been shown that the spherical structure of MOF is maintained.
Elements in ZIF-8 immobilized Fe3O4@PAA were investigated by EDX analysis. shows the elements including O, Fe, C, N and Zn for the Fe3O4@PAA@ZIF-8 nanocomposite. The EDX spectrum of Fe3O4@PAA@ZIF-8@Cip indicated the elements are O, Fe, C, N, Zn and also F element for the nanostructure which is the element of the drug CIP.
The BET method is absorption based on which the porosity of the material can be measured. The BET analysis for the nanoparticle shows that available surface area before the loading was 13.79 m2g−1 and after loading the drug, the area has fallen to 2.57 m2g−1. The volume of cavities in the Fe3O4@PAA@ZIF-8 is 3.16 cm3g−1, which, after loading the drug, reached 0.5919 cm3g−1, which is a reduction in the volume of cavities indicating the loading of the drug in the cage of metal-organic framework (). The Barrett–Joyner–Halenda (BJH) analysis also shows that the average diameter of the nanocarrier is 1.88 nm, which indicates the presence of porosity in the nanoscale ().
Figure 6. (a) BET Fe3O4@PAA@ZIF-8 analysis, (b) BET of Fe3O4@PAA@ZIF-8@CIP, (c) BJH of Fe3O4@PAA@ZIF-8 and (d) BJH Fe3O4@PAA@ZIF-8@CIP.
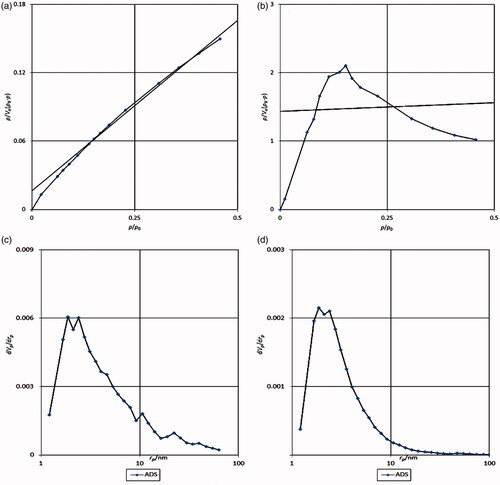
Study on drug release
Before the release, different concentrations of the drug were prepared in two buffer solutions and the absorption was achieved by a UV–Vis device at a wavelength of 323 nm, then their calibration curve was plotted. The release diagram is shown in . Drug release was performed at pH: 5 and pH: 7.4. The framework Fe3O4@PAA@ZIF-8 is unstable in acidic conditions (pH: 5) and degraded very rapidly. For this reason, drug release at pH: 5 is much faster than pH: 7.4. At pH: 5 in the first 2 h, about 20%, 3 h later the percentage of drug release reached about 64%. Degradation of metal organic framework in acidic solution cause too much release rate of the CIP. The release of the drug at physiological pH (pH: 7.4) of the body was about 74% in 24 h and then fixed to be.
Release analysis based on kinetic equations
The release of CIP from nanocomposite was studied with different kinetic models, zero order, first order diffusion, Higuchi equation and Korsmeyer–Peppas model equations (). Regarding the results, it was found that in order to describe the release of CIP in pH: 5 and pH: 7.4, the Papas and Hig models are satisfactory.
Table 1. Kinetic parameters of ciprofloxacin release.
The result of the antimicrobial test
Antimicrobial activity of Fe3O4@PAA@ZIF-8@CIP on two standard strains including E. coli and S. aureus was performed. The MOF structure is gradually degraded, and with the release of CIP, the cell wall of the bacteria is destroyed. The use of Fe3O4@PAA@ZIF-8@CIP showed excellent results for E. coli and S bacteria, as shown in . The halo created on E. coli was 15 mm for the CIP drug and the 32 mm for the drug loaded on the MOF, Also, for the aureus bacterium, it was 0 and 15 mm, respectively (). The results show that the framework makes the antibacterial effect of the drug much higher.
Figure 8. Results of antibacterial test (a) disk containing Escherichia coli bacteria and (b) disk containing Staphylococcus aureus bacteria.
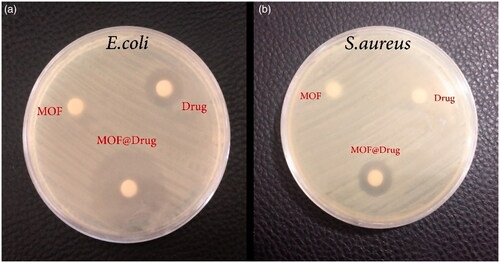
Table 2. Antibacterial activity of CIP, Fe3O4@PAA@ZIF-8 and Fe3O4@PAA@ZIF-8@CIP.
Conclusions
In summary, a novel nano-structure including Fe3O4@PAA@ZIF-8 was prepared and its structure was confirmed by FT-IR, SEM, EDX, XRD and BET techniques after approving nanocomposite’s construction, anti-inflammation drug (CIP) has loaded on the framework and with using spectroscopy analyses, drug loading in the cages of the metal-organic framework has approved. In the next level drug releasing from framework in 2pH is done, which showed framework that built has the capability of shipped and drug releasing in the physiological condition of the body. Finally, E. coli and S. aureus were used for antibacterial testing, and the results showed that the Cipro drug loaded on the framework further inhibited the growth of the bacterium. As a result, Fe3O4@PAA@ZIF-8@CIP has a stronger antibacterial property than pure CIP. It can be concluded that the use of Fe3O4@PAA@ZIF-8 as a carrier, in addition to slow release in physiological conditions of the body, can also improve the antibacterial properties of the drug.
Disclosure statement
No potential conflict of interest was reported by the authors.
References
- Wang NX, Von-Recum HA. Affinity-based drug delivery. Macromol Biosci. 2011;11:321–332.
- Tiwari G, Tiwari R, Sriwastawa B. Drug delivery systems: an updated review. Int J Pharm Investig. 2012;2:2–11.
- Farshbaf M, Davaran S, Zarebkohan A. Significant role of cationic polymers in drug delivery systems. Artif Cells Nanomed Biotechnol. 2017;46:1872–1892.
- Croy SR, Kwon GS. Polymeric micelles for drug delivery. Curr Pharm Des. 2006;12:4669–4684.
- Sharma A, Sharma U. Liposome in drug delivery: progress and limitations. Int J Pharm. 1997;154:123–140.
- Kazempour M, Namazi H, Akbarzadeh A, et al. Synthesis and characterization of PEG-functionalized graphene oxide as an effective pH-sensitive drug carrier. Artif Cells Nanomed Biotechnol. 2019;47:90–94.
- Qiu Y, Park K. Environment-sensitive hydrogels for drug delivery . Adv Drug Deliv Rev. 2001;53:321–339.
- Batten S, Champness N, Chen X, et al. Terminology of metal–organic frameworks and coordination polymers (IUPAC recommendations 2013). Pure Appl Chem. 2013;85:1715–1724.
- James S. Metal-organic frameworks. Chem Soc Rev. 2003;32:276–288.
- Horcajada P, Serre C, Vallet-Regi M, et al. Metal-organic frameworks as efficient materials for drug delivery. Angew Chem Int Ed. 2006;45:5974–5978.
- Zhu X, Wang Y, Gu J, et al. Inherent anchorages in Uio-66 nanoparticles for efficient capture of alendronate and its mediated release. Chem Commun (Camb). 2014;50:8779–8782.
- Horcajada P, Serre C, Maurin G, et al. Flexible porous metal-organic frameworks for a controlled drug delivery . J Am Chem Soc. 2008;130:6774–6780.
- Zhuang J, Kuo CH, Chou LY, et al. Opimized metal-organic framework nanospheres for drug delivery: evaluation of small-molecule encapsulation. ACS Nano. 2014;8:2812–2819.
- Zheng H, Zhang Y, Wan W, et al. One-pot synthesis of metal-organic frameworks with encapsulated target molecules and their applications for controlled drug delivery. J Am Chem Soc. 2016;138:962–968.
- Tan L, Song N, Zhang SX, et al. Ca2+, pH and thermo triple-responsive mechanized Zr-based MOFs for on command drug release in bone diseases. J Mater Chem B. 2016;4:135–140.
- Kazemi N, shojaosadati S, Morsali A. In situ synthesis of a drug-loaded MOF at room temperature. Microporous Mesoporous Mater. 2014;186:73–79.
- Zhang L, Chen Y, Li Z, et al. Tailored synthesis of octopus-type Janus nanoparticles for synergistic actively-targeted and chemo-photothermal therapy. Angew Chem Int Ed. 2016;55:2118–2121.
- Zhang M, Zhang L, Chen Y, et al. Precise synthesis of unique polydopamine/mesoporous calcium phosphate hollow Janus nanoparticles for imaging-guided chemo-photothermal synergistic therapy. Chem Sci. 2017;8:8067–8077.
- Huang XC, Lin YY, Zhang JP, et al. Ligand-directed strategy for zeolite-type metal-organic frameworks: zinc(II) imidazolates with unusual zeolitic topologies. Angew Chem Int Ed Engl. 2006;45:1557–1559.
- Hergt R, Dutz S, Muller R, et al. Magnetic particle hyperthermia: nanoparticle magnetism and materials development for cancer therapy. J Phys Condens Matter. 2006;18:2919–2934.
- Jurgons R, Seliger C, Hilpert A, et al. Drug loaded magnetic nanoparticles for cancer therapy. J Phys Condens Matter. 2006;18:2893–2903.
- Zhanet G, Fontaine S, Schurek K, et al. A review of new fluoroquinolones: focus on their use. Treat Respir Med. 2006;5:437–465.
- Ghasemzadeh MA, Mirhosseini-Eshkevari B, Abdollahi BMH. MIL‐53 (Fe) metal-organic frameworks (MOFs) as an efficient and reusable catalyst for the one‐pot four‐component synthesis of pyrano [2, 3‐c]‐pyrazoles. Appl Organometal Chem. 2019;33:e4679.
- Ghasemzadeh MA, Abdollahi-Basir MH, Mirhosseini-Eshkevari B. Multi-component synthesis of spiro [diindeno [1, 2-b: 2′, 1′-e] pyridine-11, 3′-indoline]-triones using zinc terephthalate metal-organic frameworks. Green Chem Lett Rev. 2018;11:47–53.
- Ghasemzadeh MA, Abdollahi-Basir MH, Elyasi Z. Synthesis of some novel imidazoles catalyzed by Co3O4 nanoparticles and evaluation of their antibacterial activities. Comb Chem High Throughput Screen. 2018;21:271–280.
- Farhadi S, Ghasemzadeh MA, Aghaei SS. NiCo2O4@Ni(BDC) nano-porous metal-organic framework as a novel catalyst for the synthesis of spiro[indene[1,2-d]pyrimidine-ones and investigation of their antimicrobial activities. Chem Select. 2019;4:729–736.
- Jin T, Yang Q, Meng C, et al. Promoting desulfurization capacity and separation efficiency simultaneously by the novel magnetic Fe3O4@PAA@MOF-199. RSC Adv. 2014;4:41902–41909.
- Aguado S, Quirós J, Canivet J, et al. Antimicrobial activity of cobalt imidazolate metal-organic frameworks. Chemosphere. 2014;113:188–192.
- Jian M, Liu B, Zhang G, et al. Adsorptive removal of arsenic from aqueous solution by zeolitic imidazolate framework-8 (ZIF-8) nanoparticles. Colloids Surf A. 2015;465:67–76.
- Ethiraj J, Bonino F, Lamberti C, et al. H2S interaction with HKUST-1 and ZIF-8 MOFs: a multitechnique study. Micropor Mesopor Mat. 2015;207:90–94.
- Ghasemzadeh MA, Safaei-Ghomi J, Molaei H. Fe3O4 nanoparticles: as an efficient, green and magnetically reusable catalyst for the one-pot synthesis of 1,8-dioxo-decahydroacridine derivatives under solvent-free conditions. C R Chim. 2012;15:969–974.