Abstract
Bacterial brown stripe (BBS) is one of the most economically important diseases of rice caused by Acidovorax oryzae (Ao). In order to ensure food security and safe consumption, the use of non-chemical approach is necessary. In this study, MgO and MnO2 were synthesized using chamomile flower extract. The synthesized MgO and MnO2 nanoparticles were characterized by UV–Visible spectroscopy, Fourier transform infrared spectroscopy, X-ray diffraction, transmission/scanning electron microscopy. The sizes were 18.2 and 16.5 nm for MgO and MnO2 nanoparticles, respectively. The MgO and MnO2 nanoparticles reduced the growth of Ao strain RS-2 by 62.9 and 71.3%, respectively. Also, the biofilm formation and swimming motility were significantly reduced compared to the control. The antibacterial mechanisms of MgO and MnO2 nanoparticles against RS-2 reveals that MgO and MnO2 nanoparticles penetrated the cells and destroyed the cell membrane leading to leakage of cytoplasmic content. Also, the flow cytometry observation reveals that the apoptotic cell ratio of RS-2 increased from 0.97% to 99.52 and 99.94% when treated with MgO and MnO2 nanoparticles, respectively. Altogether, the results suggest that the synthesized MgO and MnO2 nanoparticles could serve as an alternative approach method for the management of BBS.
Introduction
Plants are major sources of food which are essential for humans, animals and microorganisms existence and survival all over the world and in the ecosystem. In the process of feeding on plants, microorganisms cause various diseases to their host which leads to the loss in yield and economic losses of varying degrees. Bacterial brown stripe (BBS) is one of the most economically important diseases of rice caused by Acidovorax oryzae (Ao). This pathogen causes yield loss of economically important plant parts [Citation1,Citation2]. The disease incidence is prevalent in rice-growing regions of East Asia; China, South Korea and Japan [Citation3,Citation4]. Over time, these diseases have been managed through the use of chemicals which have posed concerns to people due to its hazardous effect on the environment and antibiotic-resistance development [Citation5]. Therefore, due to the growing population of man and a high demand for food, this disease has become a significant threat to global food security.
In order to overcome this global threat, researchers are delving into nanotechnology field to find biocompatible and eco-friendly nanomaterials that can effectively replace antibiotics in the antimicrobial application. Recent advances in nanotechnology have explored the use of carbon nanotubes, metal nanoparticles and metal oxide nanoparticles for its potential antimicrobial ability [Citation6,Citation7].
Magnesium oxide (MgO) nanoparticles have gained the interest of most scientist because of its low cost and eco-friendliness which has found usefulness as a catalyst for organic dyes degradation, photocatalytic, anticancer and antimicrobial activity due to its surface chemical activity [Citation8–11]. For manganese oxide nanoparticles, its electro-catalyst property, antimicrobial activity and its use as a fluorescence probe for glucose detection have been reported [Citation12–14].
In order to produce safe, eco-friendly and biocompatible nanomaterials green synthesis method which is gaining interest over the chemical method that is of high cost using toxic chemicals and harsh conditions for reduction is circumvented [Citation15]. Various metal oxide has been successfully green synthesized using plant extracts, bacterial, yeast, fungi and actinomycetes [Citation8,Citation16–21].
Chamomile is a perennial plant belonging to the Compositae family. In traditional medicine, it has been used over time to facilitate digestion, headache and toothache relief and as a sedative agent. Antioxidant, antimicrobial, anti-inflammatory and anti-arthritic properties of the plant have been reported [Citation22,Citation23]. Also, the plant extract has been reported to be rich in triterpenoids, phenols, tannins, flavonoids such as flavone, glycosides and flavonols [Citation24,Citation25].
The application of green synthesis to obtain MgO nanoparticles is still unexplored, and there is a dearth of information on green synthesis of manganese dioxide (MnO2) nanoparticles. Hence, this is the first report to synthesize MgO and MnO2 nanoparticles using flower extract of chamomile (Matricaria chamomilla L.) and its characterization. The MgO and MnO2 nanoparticles were tested for their antibacterial property against A. oryzae strain RS-2.
Materials and methods
Preparation of flower extract
Chamomile flower extract was prepared according to [Citation18,Citation26]. In brief, chamomile flowers, which consisted of the white petals and the yellow florets, were rinsed in double distilled water and air-dried which was followed by grinding of the flower into a fine powder. Two grams of the fine powdered was extracted in a water bath containing 200 mL of double distilled water at a temperature of 60–70 °C for 4 h. The extract was cooled to room temperature and filtered through Whatman filter paper No. 1, which was used for the synthesis of MgO and MnO2 nanoparticles.
Synthesis of MgO and MnO2 nanoparticles
Magnesium oxide and manganese dioxide were used for the synthesis of magnesium oxide and manganese dioxide nanoparticles according to [Citation27] with minor modifications. A 1:1 ratio of 1 M of magnesium oxide or manganese dioxide and the flower extracts were mixed in separate flasks, and the solutions were stirred at 100 rpm for 4 h. The resultant nanoparticles were purified by collecting the washed pellets after centrifugation at 10,000g for 20 min. The pellets were freeze-dried using Alpha 1–2 LDplus and stored at –80 °C for further characterization.
Characterization of MgO and MnO2 nanoparticles
Freeze-dried magnesium oxide and manganese dioxide nanopowder were resuspended to yield dilute suspension, and UV–Vis spectrum was generated using UV spectrophotometer (spectrophotometer Cary E 500) in the wavelength range of 200–800 nm. The Fourier transform infrared spectroscopy (FTIR) analysis of the freeze-dried powder of the reduced magnesium oxide and manganese dioxide nanoparticles were recorded under identical conditions in the range 400–4000 cm−1 at 4 cm−1 resolution using FTIR spectrophotometer vector 22, Bruker, Germany. The values of the peak observed represented the functional group responsible for the synthesized MgO and MnO2 nanoparticles.
TEM was performed using TEM (JEM-1230, JEOL, Akishima, Japan) to observe the structural characterization of MgO and MnO2 nanoparticles. SEM TM-1000, Hitachi, Japan was used to observe the structural morphology of MgO and MnO2 nanoparticles. The equipment was equipped with an energy dispersive spectrum (EDS) to evaluate the elemental composition of nanoparticles. The crystalline phase of the synthesized MgO and MnO2 nanoparticles was performed using X-ray diffractometer (XRD), XPert PRO diffractometer (Holland) with a voltage of 40 kV and a current of 30 mA using Cu-Kα radiation as an X-ray source. The recorded range of 2Ɵ was 20°–80° and the crystallite size of the nanoparticles was found using the Scherrer’s formula.
Antibacterial activity study
The minimum inhibitory concentration (MIC) which is the minimum dilution that gives complete inhibition of bacterial growth was determined by a broth dilution method assayed in 96-well microtiter plates (Corning-Costar Corp., Corning, NY, USA) according to [Citation28] with little modification. In brief, an overnight culture of Ao strain RS-2 was adjusted to 108 CFU/mL LB broth. A hundred µL of RS-2 culture was mixed with 100 µL of two-fold serial dilutions (4.0, 8.0 and 16.0 µg/mL) of nanoparticles which was incubated at 30 °C for 48 h. Bacterial culture without nanoparticles was maintained as the control. The optical density 600 was measured by a Scanning Microplate Spectrophotometer (Thermo Fisher Scientific Inc., Waltham, MA, USA).
Antagonist activity of the nanoparticles were measured on plate assay inoculated with RS-2 strain 108 CFU/mL by dropping 20 µL of different concentrations of MgO and MnO2 nanoparticles (4.0, 8.0 and 16.0 µg/mL) in their respective holes and incubated at 30 °C for 18 h. The inhibition zone was measured, and the experiment was repeated thrice.
Reduction of biofilm formation by MgO and MnO2 nanoparticles was performed in a 96-well plate by [Citation29] with minor modification. The overnight culture of Ao strain RS-2 (100 µL) was added into wells, and respective concentrations of MgO and MnO2 nanoparticles (4.0, 8.0 and 16.0 µg/mL) were added while wells without the addition of nanoparticles served as the control. After incubation for 48 h at 30 °C, the cultures were discarded and washed thrice with ddH2O to remove non-adhering cells and air-dried. An aqueous solution of crystal violet (1%, w/v) was added to the wells and left undisturbed for 30 min to stain the attached bacterial cells. A gentle wash of the wells with ddH2O was done after discarding the dye. The optical density 570 nm was recorded after the addition of 100 µL of 33% acetic acid. Three replicates were used for each treatment, and the experiment was repeated thrice.
The motility assays were carried out as previously described [Citation30] with slight modifications. A soft agar assay of 0.4% (wt/vol) agar mixed with MgO and MnO2 nanoparticles at 4.0, 8.0 and 16.0 µg/mL were poured over the plates and allowed to solidify. Ten microliters of 108 CFU/mL of Ao strain RS-2 were spotted at the centre of the plate and incubated at 30 °C for 24 h. Swimming motility was evaluated by measuring the colony diameter, and the experiment was repeated three times independently.
A. oryzae strain RS-2 was prepared for electron microscopy as previously described by [Citation31] with slight modification. Approximately, 108 bacterial of RS-2 was centrifuged at 11,000g for 10 min; the pellet was collected and resuspended in 16.0 µg/mL of MgO and MnO2 nanoparticles in their respective tubes. The suspension was incubated at 30 °C for 20 min followed by centrifugation at 11,000g for 10 min. The samples were then prepared following standard procedures for fixing and embedding. The samples were cut with a diamond knife, stained with saturated uranyl acetate and lead citrate. The grids were examined using a JEM-1230 transmission electron microscope (TEM) (JEOL, Tokyo, Japan) at an operating voltage of 75 kV.
Changes in the light scattering of Ao strain RS-2 after incubation with MgO and MnO2 nanoparticles was measured with a flow cytometer [Citation32]. The effect of MgO and MnO2 nanoparticles on Ao strain RS-2 was carried out according to [Citation33] with slight modification. Briefly, cells of overnight cultured Ao strain RS-2 was collected by centrifugation at 5,000g for 4 min and resuspended in double distilled water. The cells were then treated with 16.0 µg/mL of MgO and MnO2 nanoparticles for 4 h and immediately stained with propidium iodide (PI) in the dark for 30 min. The apoptosis in Ao strain RS-2 was detected using flow cytometry (Beckman Coulter, Gallios, Germany).
The efflux of the cytoplasmic material released as a result of the damaged cell membrane by the MgO and MnO2 nanoparticles was carried out as previously described [Citation33] with slight modification. The bacterial suspension with (4.0, 8.0 and 16.0 µg/mL) MgO and MnO2 nanoparticles were incubated at 30 °C for 4 h which was then filtered with 0.22 µm filter to remove the bacterial, MgO and MnO2 nanoparticles. The OD value was recorded at 260 nm ultraviolet light.
Statistical analysis
All results were expressed as mean ± SD (Standard Deviation) of experiments performed in triplicate. The ANOVA test was done using SAS software (SAS, Institute, Cary, NC, USA) and means were compared by the least significant difference (LSD) method at p < .05.
Results and discussion
Characterization of MgO and MnO2 nanoparticles
The synthesis of MgO and MnO2 nanoparticles using chamomile flower extract after 4 h of magnetic stirring gave an end product of yellow and brownish-black nanopowder after freeze-drying for MgO and MnO2, respectively (). In our previous studies, the presence of terpenes, flavonoids, tannins, glycosides/carbohydrates and alkaloids have been found as the main constituents of chamomile flower extract [Citation18]. Therefore, in this study, the extraction technique of [Citation18] was applied to avoid distortion of the phytochemical constituents. Also, double distilled water (polar solvent) and mild temperature of 60–70 °C was used for the extraction in order to prevent degradation of the flower’s molecular-chemical structure. The synergistic reaction of these phytochemical compounds present in chamomile flower extract act as both reducing and stabilizing agent, reducing both magnesium and manganese to its 0 valence state. The yellow and brownish-black end product of MgO and MnO2 nanopowder, respectively, was a preliminary indicator of the successful synthesis of the nanopowder ().
Figure 1. Schematic presentation of MgO and MnO2 nanoparticles synthesis: (A1) Chamomile plant (Matricaria chamomilla L.), (A2) Fresh flowers of chamomile (Matricaria chamomilla L.), (A3) Dried flowers of chamomile (Matricaria chamomilla L.), (A4) Ground dried flowers of chamomile (Matricaria chamomilla L.), (B) Filtered aqueous flower extract of chamomile (Matricaria chamomilla L.), (C) MgO solution, (D) MnO2 solution, (BC) Synthesized MgO nanoparticles, (BD) Synthesized MnO2 nanoparticles, (E) Dried MgO nanoparticles, (F) Dried MnO2 nanoparticles.
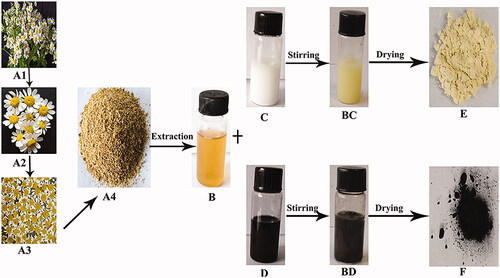
The existence of MgO and MnO2 nanoparticles was confirmed and measured by the UV spectrophotometer. The absorption spectra of MgO and MnO2 synthesized by chamomile flower extract is shown in . MgO and MnO2 nanoparticle exhibit a strong absorption band at 230 nm (). Similar results were obtained for Pisidium guvajava and Aloe vera aqueous leaf extracts mediated synthesis of MgO nanoparticles [Citation35].
Figure 2. UV–Vis spectra of nanomaterials synthesized by flower extract of chamomile (Matricaria chamomilla L.) *Mg4 - MgO nanoparticles, Mn4 - MnO2 nanoparticles.
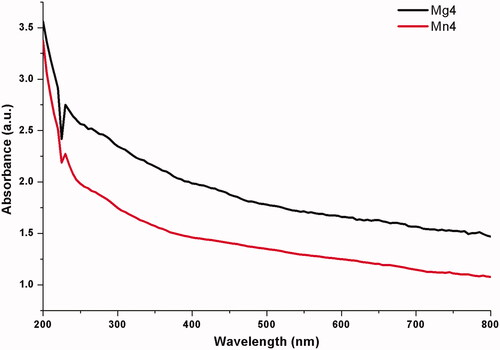
The functional group of MgO and MnO2 nanopowder was analyzed by FTIR spectrophotometer vector 22, Bruker, Germany in the range 400–4000 cm–1 (). The absorption bands for MgO nanopowder were 3697, 3646, 3405, 1633, 1557, 1395, 1059 and 436 cm–1. The three bands present at 3697, 3646 and 3405 cm–1 were due to the O–H bond stretching vibration. Band 1633 cm−1 is ascribed to the stretching vibration of C=C while the band at 1557 cm−1 is due to the stretching of C=C and bending of N–H bond. The band at 1395 cm−1 is ascribed to the bending vibration of the C–H bond. The band at 1059 cm−1, is ascribed to the stretching vibration of C–O bond and the peak at 436 cm−1 indicate the presence of MgO ().
Figure 3. Fourier transform infrared spectra of nanomaterials synthesized by flower extract of chamomile (Matricaria chamomilla L.) of (A) MgO nanoparticles, (B) MnO2 nanoparticles.
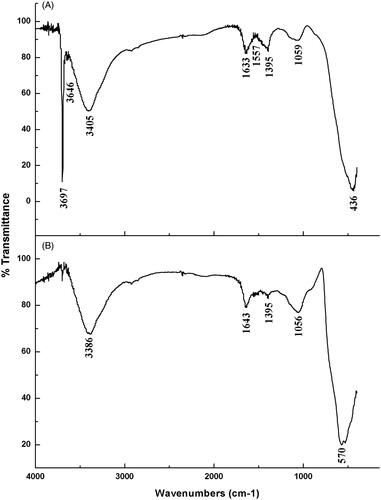
The absorption bands for MnO2 nanoparticles were 3386, 1643, 1395, 1056 and 570 cm−1. The band at 3386 cm−1 is ascribed to the broad stretching of the O–H and N–H bond. The band at 1643 cm−1 is ascribed to the stretching vibration of C=C and C=O bond. The peak at 1395 cm−1 is ascribed to the bending vibration of C–H bond. The band at 1056 cm−1 is ascribed to the stretching vibration of the C–O bond. The band at 570 cm−1 is the characteristic bands of the manganese dioxide nanoparticles ().
The FTIR spectroscopy is among the most important and widely used analytical method for obtaining information about the presence of specific functional groups in the synthesized nano-material [Citation36]. The FTIR spectra show the interaction between the MgO and MnO2 and the bioactive molecules, which was responsible for the synthesis and capping of MgO and MnO2 nanoparticles. The functional group of O–H present in the synthesized nanopowder is due to the interaction of water with the nanoparticles. The bands assigned to the C–O group is as a result of the presence of polyphenols in hydroxyl flavones while the N–H group is indicative of the protein contained in the chamomile flower extract. The C–H bond is as a result of the glycosides/carbohydrates. Previous studies mentioned that the presence of these functional groups is due to the compounds present in the plant extract which enhance the biological properties of metal oxides derived from plant extracts [Citation37].
shows the TEM micrographs obtained from the green synthesized MnO2 and MgO nanoparticles. TEM images show that MnO2 nanoparticles were irregularly spherical shaped while MgO were disc-shaped. Scanning electron microscope (SEM) gave a further understanding of the morphology and size details of MnO2 and MgO nanoparticles synthesized by chamomile flower extract. It was confirmed that MnO2 nanoparticles were irregularly spherical shaped with the size ranging from 36.6 to 112.0 nm while magnesium oxide nanoparticles had a size ranging from 8.87 to 15.2 nm (). Most syntheses of MnO2 using plant extracts have been reported to be spherical [Citation38,Citation39] which is consistent with our study. On the other hand, diverse shapes such as spherical and hexagonal were reported for the plant-mediated synthesis of MgO [Citation10,Citation40]. To the best of the author’s knowledge, this is the first report of disc-shaped MgO synthesized by plant extract.
Figure 4. Transmission electron micrographs of synthesized nanomaterials of (A) MgO nanoparticles (D) Manganese dioxide nanoparticles. Magnification 50,000X, Bar = 100 nm for A and 15,000X, Bar = 1 µm for D and Scanning electron micrographs of synthesized nanomaterials of (B and C) Magnesium oxide nanoparticles (E and F) Manganese dioxide nanoparticles.
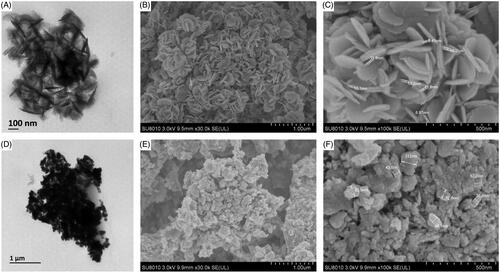
The existence of manganese and magnesium element in MnO2 and MgO nanoparticles was confirmed by SEM equipped with an EDS. The percentage elemental composition of MnO2 nanoparticles were 67.41% Mn and 32.59% O while MgO nanoparticles was composed of 47.89% Mg and 52.11% O (). The elemental percentage of MgO of this study is similar to the report [Citation41]. The nanoparticles had maximum intensity at 6.0 and 1.5 keV for Mn and Mg respectively which were of high purity, this confirms the reduction of magnesium and manganese ion to the zero valences (). The maximum intensity peak of 6.0 keV for Mn has been reported by other researchers [Citation12].
Figure 5. XRD spectra of synthesized nanomaterials (A) Magnesium oxide nanoparticles (D) Manganese dioxide nanoparticles and EDS profile of synthesized nanomaterials of (B and C) Magnesium oxide nanoparticles (E and F) Manganese dioxide nanoparticles.
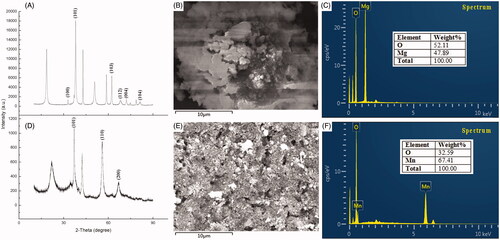
The crystalline nature of the synthesized MnO2 and MgO nanoparticles was examined by the XRD technique. The XRD analysis showed the diffraction peaks at 2Ɵ of MnO2 nanoparticles to be 37.04°, 55.9° and 66.97° which corresponds to crystal planes of (101), (110) and (200) while the diffraction patterns for MgO nanoparticles had peaks at 32.89°, 36.98°, 62.32°, 68.98°, 72.12° and 81.31° which corresponds to crystal planes of (100), (101), (103), (112), (004) and (104) (). The average crystallite size in the samples using Scherrer’s equation were 16.5 and 18.2 nm, respectively, for MnO2 and MgO nanoparticles ().
Antibacterial activity of MgO and MnO2 nanoparticles
The antibacterial activity of the synthesized nanoparticles using chamomile flower extract was tested against Ao strain RS-2. Antagonist reaction in the form of clearing zones from the spot of the nanoparticles application was observed after 18 h of incubation. MgO nanoparticles had an inhibition diameter of 1.8 cm at 16.0 µg/mL while MnO2 nanoparticles had an inhibition zone of 2.3 cm at 16.0 µg/mL. The inhibition diameter of the respective nanoparticles was significantly different from concentration 4.0 and 8.0 µg/mL (). The antibacterial behavior of magnesium oxide nanoparticles has been reported previously against Escherichia coli, Staphylococcus aureus and Bacillus subtilis var. niger [Citation42,Citation43]. The effect of MnO2 nanoparticles as an antibacterial agent has been reported on S. aureus, E coli, Klebsiella pneumonia, B. subtilis and Pseudomonas aeruginosa bacterial which had a varying diameter of inhibition zone [Citation12].
Figure 6. (A) Antibacterial activity of synthesized nanomaterials against Acidovorax oryzae strain RS-2. (B) Effect of synthesized nanomaterials on the growth of A. oryzae strain RS-2. Plates are showing inhibition zones of A. oryzae strain RS-2. *Mg + 4 magnesium oxide nanoparticles; Mn + 4 manganese dioxide nanoparticles. *Values are a mean ± standard error of three replicates and bars with the same letters are not significantly different in LSD test (p < .05).
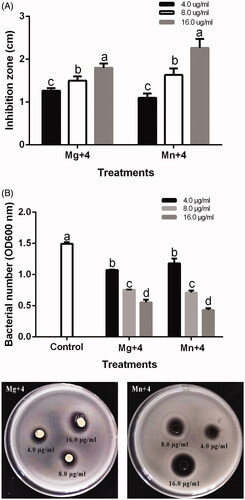
The minimum inhibitory concentration (MIC) of MgO and MnO2 nanoparticles on Ao strain RS-2 was carried out on a 96-well microtiter plate using concentrations of 4.0, 8.0 and 16.0 µg/mL. MgO and MnO2 nanoparticles maximally inhibited strain RS-2 at concentration 16.0 µg/mL at OD600 (). The bacterial number of RS-2 at OD600 was 1.49 which was significantly reduced at 16.0 µg/mL by 62.90% and 71.25% for MgO and MnO2 nanoparticles, respectively (). This result depicts that the efficiency of the MgO and MnO2 nanoparticles used in this study were concentration dependent which is in agreement with previous reports of other metal oxide nanoparticles [Citation34,Citation44,Citation45]. Also, it has been reported that the sizes of nanoparticles play a significant role in their antibacterial efficiency [Citation34,Citation45,Citation46]. This nanometer size have different shapes with a high surface area, charge, adsorption and high chemical reactivity which enables them to interact efficiently with biological systems, causing significant suppression [Citation47]. We, therefore, conclude that the nanometer sizes of MgO and MnO2 nanoparticles played a vital role in its antibacterial efficiency.
Biofilm formation and swimming motility of A. oryzae has been reported to be highly involved in bacterial virulence [Citation34] . In order to ascertain the efficacy of the nanoparticles in bacterial virulence, MgO and MnO2 nanoparticles effect on the biofilm formation and swimming motility experiment were carried out. The biofilm formed by RS-2 was significantly reduced by MgO and MnO2 nanoparticles. A biofilm reduction of 69.71% and 77.86% (OD 570) at 16.0 µg/mL was recorded for MgO and MnO2 nanoparticles, respectively (). The Ao strain RS-2 swam in the soft agar medium and had a diameter of 1.36 cm after 24 h of incubation (). Treatment of the soft agar medium with MgO and MnO2 nanoparticles significantly reduced its swimming motility. A significant reduction in the halo diameter of 43.63% and 53.43% at 16.0 µg/mL for MgO and MnO2 nanoparticles, respectively, were recorded (). Therefore, the MgO and MnO2 nanoparticles synthesized using chamomile extract used in this study are an efficient anti-biofilm agent.
Figure 7. (A) Effect of synthesized nanomaterials on biofilm formation of Acidovorax oryzae strain RS-2. (B) Effect of synthesized nanomaterials on the swimming motility of A. oryzae strain RS-2 of *Mg + 4 magnesium oxide nanoparticles; Mn + 4 manganese dioxide nanoparticles. *Values are a mean ± standard error of three replicates and bars with the same letters are not significantly different in LSD test (p < .05).
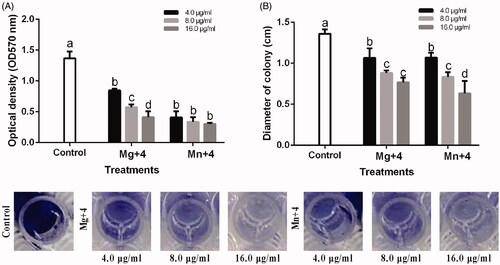
Biofilm formation has been attributed to contributing to the antibiotic resistance in bacterial [Citation48]. The loss of biofilm formation and swimming motility ability of RS-2 when treated with MgO and MnO2 nanoparticles indicates less virulence when invading and colonizing host tissue. The suppression of biofilm formation and swimming motility observed is consistent with the studies of [Citation33,Citation48] who reported a concentration-dependence on the ability to suppress biofilm formation and the motility of the bacteria.
The release of cell content after 4 h of incubation at 30 °C with MgO and MnO2 nanoparticles out of RS-2 is shown in . Treatment of RS-2 with double distilled water (control) at OD260 had a cell efflux of 0.05 while treatment with MgO and MnO2 nanoparticles at 16.0 µg/mL had an efflux of 0.26 and 0.30, respectively (). The efflux value recorded reveals that the cell membrane was severely destroyed, which was dependent on the concentration of MgO and MnO2 nanoparticles applied. Thus, proving the deformation and damage of cell observed under the transmission electron microscope [Citation33] also observed cellular injury after treating Ralstonia solanacearum with MgO nanoparticles.
Figure 8. (A) Effect of the nanomaterials on the efflux of cytoplasmic materials on Acidovorax oryzae strain RS-2. (B) TEM images of A. oryzae strain RS-2. Magnification 150,000X for control and Mg + 4; 200,000X for Mn + 4; Bar = 200 nm *Mg + 4 magnesium oxide nanoparticles; Mn + 4 manganese dioxide nanoparticles. *Values are a mean ± standard error of three replicates and bars with the same letters are not significantly different in LSD test (p < .05).
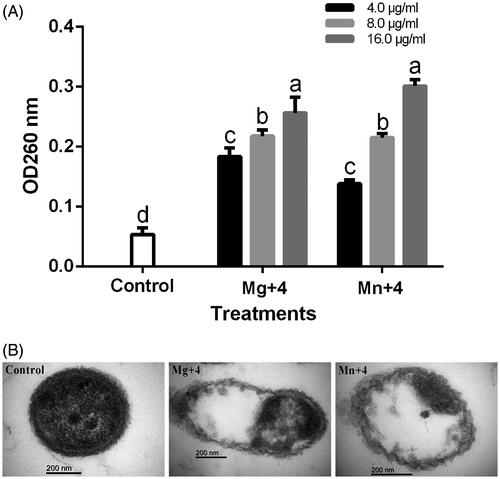
The effect of MgO and MnO2 nanoparticles on RS-2 cell were viewed under a transmission electron microscope (TEM). The RS-2 cell treated with MgO and MnO2 nanoparticles shows a disruption in the morphology of the cell indicating that the nanoparticles had successfully penetrated the cell thus damaging the cell membrane and causing leakage of cell content (). Contrary to this observation, the Ao strain RS-2 treated with double distilled water which served as the control maintained its well-defined cell membrane and having an evenly dispersed cell content which corresponds to the proteins, DNA and cytoplasmic content of the cell (). The small-sized nanoparticle of this study gave it an added advantage to be able to penetrate the bacterial membrane easily which lead to cell membrane leakage and ultimately death [Citation49]. The penetration of nanoparticles into the bacterial cell has been reported to be the primary mechanism for bacterial inhibition [Citation49].
Flow cytometry observation has been reported to allow for early detection of the internalization of nanoparticles in live bacterial [Citation32]. Propidium iodide (PI) is a dye that can only stain disrupted cell membranes which then intercalates into the double-stranded nucleic acids. The cellular structural damage caused by MgO and MnO2 nanoparticles to RS-2 cell was observed using flow cytometry in combination with PI (). The apoptotic cell ratio of RS-2 increased to 99.52% and 99.94% when treated with 16.0 µg/mL MgO and MnO2 nanoparticles, respectively, while the control had 0.97% (). The result revealed that the MgO and MnO2 nanoparticles increased the membrane permeability of RS-2 which resulted in cell injury and ultimately cell death. This result is similar to the reports of [Citation33] when R. solanacearum cells were treated with MgO nanoparticles.
Figure 9. Flow cytometry images of Acidovorax oryzae strain RS-2 after incubation with (A) double distilled water (Control) (B) magnesium oxide nanoparticles (C) manganese dioxide nanoparticles.
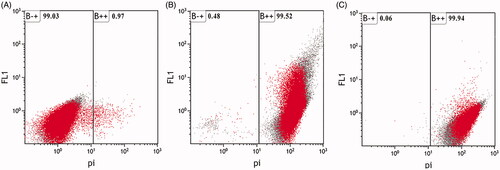
Nanoparticles toxicity has been reported to be highly dependent on the concentration used [Citation50, Citation51]. In the medical field, researchers mostly use manganese nanoparticles due to their non-toxic effect [Citation52–55]. Recently, Cai et al. [Citation33] reported that MgO nanoparticles had no toxic effect on the environment, plants and human health. It is reasonable to infer that the low concentration (4.0, 8.0 and 16.0 µg/mL) of metal oxide nanoparticles to combat BBS will have no toxic effect on the ecosystem.
Conclusions
The synthesized MgO and MnO2 nanoparticles by flower extract of chamomile (M. chamomilla L.) to the best of the author's knowledge is the first report of its characterization and antibacterial activity. The nanoparticles were characterized using UV–Visible spectroscopy, FTIR, XRD, SEM, TEM, and EDS. The MgO and MnO2 nanoparticles displayed strong antibacterial effects against A. oryzae strain RS-2 which was concentration-dependent. Overall, we expect that the MgO and MnO2 nanoparticles can be used as an alternative management approach in combatting Bacterial brown stripe (BBS) diseases by soaking the rice seeds in the nanoparticles solution.
Disclosure statement
The authors declare that the research was conducted in the absence of any commercial or financial relationships that could be construed as a potential conflict of interest.
Additional information
Funding
References
- Qu ZC, Liang DL, Harper G, et al. Comparison of sequences of RNAs 3 and 4 of rice stripe virus from China with those of Japanese isolates. Virus Genes. 1997;15:99–103.
- Wei TY, Yang JG, Liao FL, et al. Genetic diversity and population structure of rice stripe virus in China. J Gen Virol. 2009;90:1025–1034.
- Cho WK, Lian S, Kim SM, et al. Current insights into research on rice stripe virus. Plant Pathol J. 2013;29:223–233.
- Wu W, Zheng L, Chen H, et al. Nonstructural protein NS4 of rice stripe virus plays a critical role in viral spread in the body of vector insects. PLos One. 2014;9:1–11.
- Yoshii A, Moriyama H, Fukuhara T. The novel kasugamycin 2'-N-acetyltransferase gene aac(2')-IIa, carried by the IncP Island, confers kasugamycin resistance to rice-pathogenic bacteria. Appl Environ Microbiol. 2012;78:5555–5564.
- EI-Argawy E, Rahhal MMH, EI-Korany A, et al. Efficacy of some nanoparticles to control damping-off and root rot of sugar beet in EI-Behiera governorate. Asian J Plant Pathol. 2017;11:35–47.
- Zhong L, Liu H, Samal M, et al. Synthesis of ZnO nanoparticles-decorated spindle-shaped graphene oxide for application in synergistic antibacterial activity. J Photochem Photobiol B Biol. 2018;183:293–301.
- Abdallah Y, Ogunyemi SO, Abdelazez A, et al. The green synthesis of MgO nano-flowers using Rosmarinus officinalis L. (Rosemary) and the antibacterial activities against Xanthomonas oryzae pv. oryzae. BioMed Res Int. 2019;2019:1–8.
- Salehifar N, Zarghami Z, Ramezani M. A facile, novel and low-temperature synthesis of MgO nanorods via thermal decomposition using new starting reagent and its photocatalytic activity evaluation. Mater Lett. 2016;167:226–229.
- Suresh J, Pradheesh G, Alexramani V, et al. Green synthesis and characterization of hexagonal shaped MgO nanoparticles using insulin plant (Costus pictus D. Don) leave extract and its antimicrobial as well as anticancer activity. Adv Powder Technol. 2018;29:1685–1694.
- Zhang W, Tay HL, Lim SS, et al. Supported cobalt oxide on MgO: highly efficient catalysts for degradation of organic dyes in dilute solutions. Appl Catal B Environ. 2010;95:93–99.
- Cherian E, Rajan A, Baskar G. Synthesis of manganese dioxide nanoparticles using co-precipitation method and its antimicrobial activity. Int J Modern Sci Technol. 2016;1:17–22.
- Kang B, Jin X, Oh SM, et al. An effective way to improve bifunctional electrocatalyst activity of manganese oxide via control of bond competition. Appl Catal B Environ. 2018;236:107–116.
- Shen SL, Jia MM, Tang ZH, et al. Preparation and application of Mn-doped Zn0.5Cd0.5S @ ZnS nanorods with high quantum yield as sensitive fluorescence probe for detection of glucose. Mater Res Bull. 2018;106:471–477.
- Mason C, Vivekanandhan S, Misra M, et al. Switchgrass (Panicum virgatum) extract mediated green synthesis of silver nanoparticles. WJNSE. 2012;02:47–52.
- Elegbede JA, Lateef A, Azeez MA, et al. Fungal xylanases-mediated synthesis of silver nanoparticles for catalytic and biomedical applications. IET Nanobiotechnol. 2018;12:857–863.
- Fouad H, Li HJ, Ding YM, et al. Synthesis and characterization of silver nanoparticles using Bacillus amyloliquefaciens and Bacillus subtilis to control filarial vector Culex pipiens pallens and its antimicrobial activity. Artif Cells Nanomed Biotechnol. 2017;45:1369–1378.
- Ogunyemi SO, Abdallah Y, Zhang M, et al. Green synthesis of zinc oxide nanoparticles using different plant extracts and their antibacterial activity against Xanthomonas oryzae pv. oryzae. Artif Cell Nanomed Biotechnol. 2019a;47:341–352.
- Saravanakumar K, Chelliah R, Shanmugam S, et al. Green synthesis and characterization of biologically active nanosilver from seed extract of Gardenia jasminoides Ellis. J Photochem Photobiol B Biol. 2018;185:126–135.
- Shanmugasundaram T, Balagurunathan R. Bio-directed synthesis, structural characterisation of platinum based metal nanocomposites (Pt/Ag, Pt/Au, Pt/Ag/Au) and their biomedical applications. Mater Res Express. 2018;5:1–9.
- Wypij M, Czarnecka J, Swiecimska M, et al. Synthesis, characterization and evaluation of antimicrobial and cytotoxic activities of biogenic silver nanoparticles synthesized from Streptomyces xinghaiensis OF1 strain. World J Microbiol Biotechnol. 2018;34:1–13.
- Munir N, Iqbal AS, Altaf I, et al. Evaluation of antioxidant and antimicrobial potential of two endangered plant species Atropa belladonna and Matricaria chamomilla. Afr J Trad Compl Alt Med. 2014;11:111–117.
- Singh KG, Sonia S, Konsoor N. In-vitro and ex-vivo studies on the antioxidant, anti-inflammatory and antiarthritic properties of Camellia sinensis, Hibiscus rosa sinensis, Matricaria chamomilla, Rosa SP., Zingiber officinale tea extracts. Int J Pharm Sci Res. 2018;9:3543–3551.
- Harbourne N, Jacquier JC, O’Riordan D. Optimisation of the extraction and processing conditions of chamomile (Matricaria chamomilla L.) for incorporation into a beverage. Food Chem. 2009;115:15–19.
- Sharifi-Rad M, Nazaruk J, Polito L, et al. Matricaria genus as a source of antimicrobial agents: From farm to pharmacy and food applications. Microbiol Res. 2018;215:76–88.
- Sharma D, Thakur N, Vashistt J, et al. Antibacterial evaluation of cuprous oxide nanoparticles synthesized using leaf extract of Callistemon viminalis. IJPER. 2018;52:449–455.
- Sutradhar P, Saha A. Green synthesis of zinc oxide nanoparticles using tomato (Lycopersicon esculentum) extract and its photovoltaic application. J Exp Nanosci. 2016;11:314–327.
- Vassallo J, Besinis A, Boden R, et al. The minimum inhibitory concentration (MIC) assay with Escherichia coli: an early tier in the environmental hazard assessment of nanomaterials? Ecotoxicol Environ Safety. 2018;162:633–646.
- Hassan A, Usman J, Kaleem F, et al. Evaluation of different detection methods of biofilm formation in the clinical isolates. Braz J Infect Dis. 2011;15:305–311.
- Rashid MH, Kornberg A. Inorganic polyphosphate is needed for swimming, swarming, and twitching motilities of Pseudomonas aeruginosa. Proc Natl Acad Sci U S A. 2000;97:4885–4890.
- Helander IM, Nurmiaho-Lassila EL, Ahvenainen R, et al. Chitosan disrupts the barrier properties of the outer membrane of Gram-negative bacteria. Int J Food Microbiol. 2001;71:235–244.
- Kumar A, Pandey AK, Singh SS, et al. A flow cytometric method to assess nanoparticle uptake in bacteria. Cytometry A 2011;79A:707–712.
- Cai L, Chen JN, Liu ZW, et al. Magnesium oxide nanoparticles: effective agricultural antibacterial agent against Ralstonia solanacearum. Front Microbiol. 2018;9:1–19.
- Ogunyemi SO, Fang Y, Qiu W, et al. Role of type IV secretion system genes in virulence of rice bacterial brown stripe pathogen Acidovorax oryzae strain RS-2. Microb Pathog. 2019b;126:343–350.
- Umaralikhan L, Jaffar MJM. Green synthesis of MgO nanoparticles and it antibacterial activity. Iran J Sci Technol Trans Sci. 2018;42:477–485.
- Fouad H, Li HJ, Hosni D, et al. Controlling Aedes albopictus and Culex pipiens pallens using silver nanoparticles synthesized from aqueous extract of Cassia fistula fruit pulp and its mode of action. Artif Cells Nanomed Biotechnol. 2018;46:558–567.
- Ngoepe NM, Mbita Z, Mathipa M, et al. Biogenic synthesis of ZnO nanoparticles using Monsonia burkeana for use in photocatalytic, antibacterial and anticancer applications. Ceram Int. 2018;44:16999–17006.
- Moon SA, Salunke BK, Saha P, et al. Comparison of dye degradation potential of biosynthesized copper oxide, manganese dioxide, and silver nanoparticles using Kalopanax pictus plant extract. Korean J Chem Eng. 2018;35:702–708.
- Souri M, Hoseinpour V, Shakeri A, et al. Optimisation of green synthesis of MnO nanoparticles via utilising response surface methodology. IET Nanobiotechnol. 2018;12:822–827.
- Sharma G, Soni R, Jasuja ND. Phytoassisted synthesis of magnesium oxide nanoparticles with Swertia chirayaita. J Taibah Univ Sci. 2017;11:471–477.
- Moorthy SK, Ashok CH, Rao KV, et al. Synthesis and characterization of MgO nanoparticles by neem leaves through green method. Mater Today Proc. 2015;2:4360–4368.
- Lei H, Li DQ, Lin YJ, et al. Influence of nano-MgO particle size on bactericidal action against Bacillus subtilis var. niger. Chinscibull. 2005;50:514–519.
- Makhluf S, Dror R, Nitzan Y, et al. Microwave-assisted synthesis of nanocrystalline MgO and its use as a bacteriocide. Adv Funct Mater. 2005;15:1708–1715.
- Emami-Karvani Z, Chehrazi P. Antibacterial activity of ZnO nanoparticle on gram-positive and gram-negative bacteria. Afr J Microbiol Res. 2011;5:1368–1373.
- Krol A, Pomastowski P, Rafinska K, et al. Zinc oxide nanoparticles: synthesis, antiseptic activity and toxicity mechanism. Adv Colloid Interface Sci. 2018;249:37.
- Jones N, Ray B, Ranjit KT, et al. Antibacterial activity of ZnO nanoparticle suspensions on a broad spectrum of microorganisms. Fems Microbiol Lett. 2008;279:71–76.
- Wang LL, Hu C, Shao LQ. The antimicrobial activity of nanoparticles: present situation and prospects for the future. IJN. 2017;12:1227–1249.
- Rajkumari J, Busi S, Vasu AC, et al. Facile green synthesis of baicalein fabricated gold nanoparticles and their antibiofilm activity against Pseudomonas aeruginosa PAO1. Microb Pathog. 2017;107:261–269.
- Raghupathi KR, Koodali RT, Manna AC. Size-dependent bacterial growth inhibition and mechanism of antibacterial activity of zinc oxide nanoparticles. Langmuir. 2011;27:4020–4028.
- Capaldi Arruda SC, Diniz Silva AL, Galazzi RM, et al. Nanoparticles applied to plant science: a review. Talanta. 2015;131:693–705.
- Zabrieski Z, Morrell E, Hortin J, et al. Pesticidal activity of metal oxide nanoparticles on plant pathogenic isolates of Pythium. Ecotoxicology. 2015;24:1305–1314.
- Kim T, Momin E, Choi J, et al. Mesoporous silica-coated hollow manganese oxide nanoparticles as positive T-1 contrast agents for labeling and MRI tracking of adipose-derived mesenchyrnal stem cells. J Am Chem Soc. 2011;133:2955–2961.
- Na HB, Lee JH, An KJ, et al. Development of a T-1 contrast agent for magnetic resonance imaging using MnO nanoparticles. Angew Chem Int Ed. 2007;46:5397–5401.
- Shin JM, Anisur RM, Ko MK, et al. Hollow manganese oxide nanoparticles as multifunctional agents for magnetic resonance imaging and drug delivery. Angew Chem Int Ed. 2009;48:321–324.
- Yu X, Wadghiri YZ, Sanes DH, et al. In vivo auditory brain mapping in mice with Mn-enhanced MRI. Nat Neurosci. 2005;8:961–968.