Abstract
Angiotensin I-Converting Enzyme (ACE, CD143) Gene plays a crucial role in the pathology of carcinomas in many cancers including colorectal cancer (CRC). However, the methylation of ACE was rarely reported. In this study, our purpose was to investigate the methylation status of ACE and explored its prognostic value in CRC. The expression of ACE was detected by quantitative real-time polymerase chain reaction (qRT-PCR) analysis while the methylation status of ACE was measured via methylation-specific polymerase chain reaction (MSP). The result demonstrated that ACE expression was up-regulated in tumour tissues and HT-29 cells compared with the controls. ACE was also confirmed to be hypomethylated in CRC. Next, we evaluated the influence of ACE hypomethylation on cell growth. It was proved to be a favourable factor for the cell proliferation, cell colony forming, but an inhibitor for the cell apoptosis of CRC cells according to MST assay, colony forming assay and flow cytometry assay. ACE hypomethylation was also considered to be related to the prognosis of CRC through Cox regression analysis. Taken together, the over-expression of ACE was regulated by its hypomethylation and the ACE hypomethylation might be an independent prognostic indicator in CRC.
Introduction
Colorectal cancer (CRC) is one of the most common malignancies and the third leading cause of cancer-related death, causing over 600,000 deaths every year all over the world [Citation1,Citation2]. The morbidity and mortality are increasing recently. In 2014, there are approximately 136,830 individuals newly diagnosed with colorectal cancer and 50,310 colorectal cancer deaths in the United States [Citation3]. And, its incidence is associated with changes in diet and environment closely. Although there are great achievements had got in the treatment of CRC, but the prognosis of CRC is still poor due to its frequent metastasis [Citation4]. Therefore, it is necessary to explore effective molecular marker for the prognosis of CRC.
Angiotensin I-Converting Enzyme (ACE, CD143) is a key enzyme in regulating the activity of renin angiotensin system (RAS) [Citation5]. It is expressed by many cell types of various organs and tissues, including vascular endothelial cells, epithelial cells of the small intestine, kidney tubular cells, mononuclear cells, and fibroblasts [Citation6]. ACE plays crucial roles in the pathology of carcinomas and influences many tumour processes such as cell proliferation, tumour cell migration, angiogenesis, and metastatic behaviour for its differentially expressed in several malignancies [Citation5–9]. The gene encoding ACE is located in chromosome 17 consists of 26 exons and 25 introns in humans [Citation10]. It has been cloned and found to be characterized in humans by a major polymorphism exhibiting presence (I allele, insertion) or absence (D allele, deletion) of a 287-base pair Alu repeat sequence in intron 16 of this gene [Citation11]. In previous studies, the association between ACE gene polymorphism and the occurrence risk has been investigated in various diseases. ACE insertion/deletion (I/D) polymorphism was also found to be involved in the risk colorectal cancer (CRC) development [Citation12]. A mete-analysis reported ACE I/D polymorphism was significantly associated with the risk of digestive cancer [Citation13]. The methylation of ACE had also been studied in some disease. Lam reported that AEC methylation may serve as a biomarker for cortisol and/or HPA axis activity in depression [Citation14]. Aberrant methylation in promoter region of ACE2 may contribute to essential hypertension (EH) risk [Citation15]. However, the methylation status of ACE and its function in CRC is never reported.
In this study, we detected the expression of ACE and its status of methylation. The effects of ACE methylation on cell growth and prognosis of CRC had also been estimated.
Materials and methods
Patients and samples
One hundred twelve patients who were diagnosed as CRC were collected from Southwest Hospital, Army Medical University and the study was permitted by the Ethnic Committee of the hospital. All of them had never received any therapy before sampling and signed written inform consent in advance. Besides, 87 healthy people were taken as healthy controls.
Tumour tissues and adjacent non-cancerous tissues from CRC patients as well as tissues from healthy people were obtained. Then all tissues samples were frozen by liquid nitrogen immediately and stored at −80 °C for DNA and RNA extraction. The clinicopathologic characteristics including age, gender, tumour size, tumour site, histology types, TNM stages, dukes classification system, invasion depth, Lymph node metastasis and distant metastasis were recorded in a database. A 5-years’ follow-up was performed via a telephone or questionnaire. Patients who died from unexpected events or other diseases were excluded from the study.
RNA extraction and quantitative real-time polymerase chain reaction (qRT-PCR)
Total RNA was isolated from all the tissues samples using TRIzol (Invitrogen, Carlsbad, CA, USA). The first chain of cDNA was synthesized by reverse transcription with TaqMan MicroRNA Reverse Transcription Kit (Applied Biosystems, Foster City, CA, USA). Then, RT-PCR reaction was performed in the Applied Biosystems 7900 Fast Real-Time PCR system (Applied Biosystems, Foster City, California, USA). β-Actin was taken as an endogenous control. The relative expression quantification of ACE gene at mRNA level was evaluated by comparative cycle threshold (CT) method.
DNA extraction and bisulfite modification
Genomic DNA was extracted from the tumour tissues, adjacent tissues and healthy controls with the DNA Mammalian Genomic Purification Kit purchased from Sigma-Aldrich Co. (St. Louis, MO) according to the manufacturer’s instruction. Then the DNA was treated with sodium bisulfite using the EZ DNA MethylationTM Kit (Zymo Research, Orange, CA) to conduct the conversion of cytosine to uracil. The modified DNA was resuspended in elution buffer and stored at −20 °C until next step.
Cell culture and transfection
Human CRC cell lines HT-29 and normal colorectal mucosa cell lines FHC were purchased from the Cell Bank of the Chinese Academy of Sciences (Shanghai, China) and grown in RPMI-1640 supplemented with 10% fetal bovine serum in humidified of 95% air and 5% CO2 at 37 °C.
The HT-29 cells were seeded in 96-well plates and cultured overnight. ACE gene was cloned from human genomic DNA into the pGL3 basic vector (Promega, E1751). Then, the cells were transfected with pGL3-ACE and pGL3-vector (negative control) using Lipofectamine 2000 (Invitrogen 11668027) according to the manufacturer’s instructions after the cells concentration reached 80–90%. The experiment was conducted in triplicate.
ACE methylation assay
The methylation level of ACE was detected using bisulfite polymerase chain reaction (PCR) and pyrosequencing. The bisulfite-converted DNA was amplified using PCR and the amplification system contained 2 μl bisulfite-converted DNA, 2.5 μl 10 × PCR buffer, 2.5 μl MgCI2 solution, 2.5 μl dNTPs, 2.5 μl forward and reverse primer, respectively, 0.1 μl 5 U/μl Taq Gold polymerase and sterile water which was supplied to total 25 μl. The procedure of PCR was as follows: initial denaturation at 95 °C for 15 min, 45 cycles of denaturation for 30 s at 95 °C, annealing at 55 °C for 45 s, extension at 72 °C for 30 s, and finally extension at 72 °C for 5 min. PCR amplification was performed in three duplicates for every participant sample. CpG sites of ACE promoter were performed the pyrosequencing analysis using a PyroMark System (Qiagen, Hilden, Germany).
Cell proliferation and apoptosis assay
The ability of cell proliferation was analyzed via MTS assay. After transfection, HT-29 cells viability at different time points (0, 24, 48, and 72 h) was measured at 450 nm with an enzyme immunoassay analyzer (Bio-Rad, Hercules, CA, USA). Each sample was in triplicate.
The cells were harvested and fixed with 2.5% glutaraldehyde for 30 min after treating with or without DDP for another 12 h. Then, the cells were conducted with routine embedment and section. The apoptosis rates were determined via Annexin V-FITC and PI staining flow cytometry.
Cell colony forming assay
HT-29 cells transfected with pGL3-ACE or empty vector were cultured in a 6-well plates for 12 h. Then, these cells were maintained in DMEM containing 10% FBS for 2 weeks. Finally, colonies were fixed with methanol and stained with 0.1% crystal violet in 20% methanol for 15 min.
Statistical analysis
The statistical analysis was conducted with SPSS 13.0 software. Graph pad primer 5 was used to design the relative figures. The differences between two groups were estimated by students’ t-test while three or more groups were analyzed with one way ANOVA assay. The relationship between ACE methylation and clinicopathologic characteristics was estimated by chi-square test. Kaplan–Meier analysis was used to distinguish the difference of overall survival of patients with different statue of ACE methylation. And, Cox regression was taken to predict the prognostic role of ACE methylation adjusting for clinicopathologic characteristics. p < .05 represented that the difference was significant.
Results
ACE was over-expression in CRC patients
The expression level of ACE at mRNA level in tissues and cell lines were both detected via qRT-PCR. It demonstrated that ACE expression was significantly higher in tumour tissues than in adjacent non-cancerous tissues and healthy tissues (, p < .05). Besides, ACE expression in HT-29 cell lines was also increased compared with those in cell lines FHC (, p < .05).
Figure 1. The expression of ACE at mRNA level in tumour tissues and cell lines. (A) The ACE expression was higher in tumour tissues compared with in adjacent normal tissues and healthy tissues. (B) The ACE expression was higher in HT-29 cell lines than in FHC cell lines. **p < .05 represented the significant difference between the two.
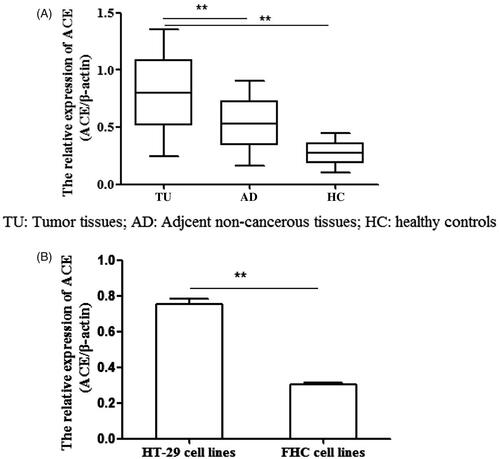
The status of ACE gene methylation in patients with CRC
We investigated the status of ACE methylation in patients with CRC through MSP. The result showed that the methylation level of ACE in tumour tissues was the lowest compared with that in adjacent non-cancerous tissues and healthy tissues (, p < .05) while it was also lower in HT-29 cells than in FHC cells (, p < .05). Namely, ACE was hypomethylation in CRC patients.
Figure 2. The methylation status of ACE in tumour tissues and cell lines. (A,B) The methylation of ACE was lower in tumour tissues than in adjacent normal tissues and healthy tissues. (B) The methylation of ACE was lower in HT-29cell lines than in FHC cell lines. **p < .05 represented the significant difference between the two.
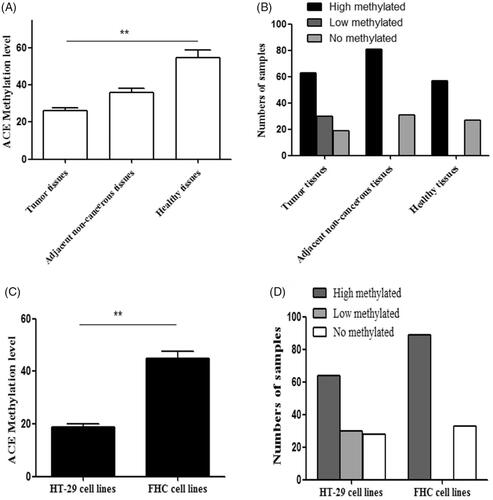
ACE hypomethylation promoted cell proliferation, inhibited cell apoptosis and increased the colony forming
To explore whether ACE hypomethylation could influence the tumour growth of CRC cells, MTS assay, flow cytometry and colony forming assay were used to estimate the cell proliferation, apoptosis and colony formation. MTS assay indicated that cell proliferation was promoted by ACE hypomethylation (). Cell apoptosis was reduced () while cell colony formation was increased by ACE hypomethylation () in CRC patients. These revealed that ACE hypomethylation contributed to the cell growth of CRC cells.
The relationship between ACE hypomethylation and clinicopathologic characteristics
To study the role of ACE hypomethylation in the development of CRC, we analyzed its relationship with clinicopathologic characteristics. As shown in , TNM stages (p = .010), invasion depth (p = .002), and lymph node metastasis (p = .015) were proved to important factors for the status of ACE methylation which manifested ACE methylation was involved in the development of CRC.
Table 1. The relationship between the methylation of ACE gene and clinicopathologic characteristics.
Association between ACE methylation and overall survival of patients with CRC
To make clear the prognostic value of ACE hypomethylation, we carried out a 5 years’ follow-up. During the follow-up, there were patients died. Kaplan–Meier analysis showed patients with ACE hypomethylation had a shorter overall survival than those with ACE hypermethylation (, log rank test, p < .001). This revealed that ACE hypomethylation was related to the prognosis of CRC. Then, we further analyzed the prognostic value of ACE hypomethylation using Cox regression analysis. The outcome indicated that ACE hypomethylation (HR = 8.231, 95CI% = 2.554–26.532, p < .001), tumor size (HR = 6.116, 95CI% = 2.372–15.757, p < .001) and invasion depth (HR = 8.371, 95CI% = 3.075–22.789, p < .001) were all vital influence factors in the prognosis of CRC ().
Figure 6. Kaplan–Meier analysis of ACE hypomethylation in patients with CRC. The patients with ACE hypomethylation had a shorter overall survival compared with those with ACE hypermethylation and unmethylation (Log-rank test, p < .001).
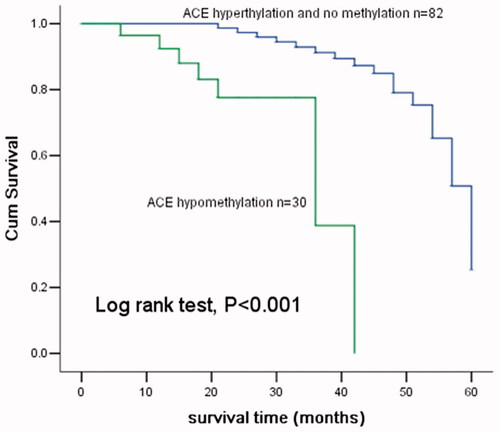
Table 2. Multivariate analysis for the prognostic factors in patients with CRC.
Discussion
Methylation is one of the most widely used epigenetic modification in human development and disease [Citation16–19]. It takes effect on the transcriptional regulation, chromosomal stability, genomic imprinting and X-inactivation [Citation20]. DNA methylation controls the availability of gene regulatory sequences towards transcription factors and enzymes via methylating the promoter regions and chromatin condensation state [Citation21]. Aberrant DNA methylation of various genes has been found in different tumour types and serves as a promising potential bio-marker and is widely studied in various types of cancers [Citation22–27].
There were many studies about genes methylation in CRC had been reported in the past years. For instance, the gene MT3-MMP was down-regulated and its low expression could inhibit cell migration via promoter hypermethylation [Citation28]. Tsai et al. considered the hypermethylation of SHISA3 could not only down-regulate the expression of this gene but also can influence the prognosis of CRC [Citation29]. The meta-analysis made by Ding et al. demonstrated that APC promoter methylation was significantly related to the risk of CRC and it might be a potential biomarker for the carcinogenesis of CRC [Citation30]. Numerous studies had confirmed the association between ACE polymorphism and risk of various cancers including CRC [Citation12,Citation31–33]. Moreover, the methylation of ACE had also been found in some diseases [Citation11,Citation34]. However, the relationship between ACE methylation and CRC remains unknown.
In the current study, we first measured the expression of ACE at mRNA level using qRT-PCR analysis. The data manifested it was over-expression in tumour tissues and cell lines compared with the controls. This might be revealed ACE was an oncogene in CRC. Then, we investigated the methylated status of ACE with MSP which showed a hypomethylation of ACE in CRC patients. So, we inferred that ACE hypomethylation might be an influencing factor for the aberrant expression of ACE. Next, we analyzed whether ACE hypomethylation was correlated with the development of CRC through evaluated the relationship between it and clinicopathologic characteristics. The result indicated that ACE hypomethylation was involved in the development of CRC.
As respect to the correlation between ACE hypomethylation and cell growth, we conducted MTS assay, flow cytometry as well as colony forming assay. As a result, ACE hypomethylation was found that it could reduce cell growth by promoting cell proliferation and colony formation, inhibiting cell apoptosis at same time. We also estimated the prognostic value of ACE hypomethylation with Kaplan–Meier and Cox regression analysis. Kaplan–Meier analysis showed that hypomethylation of ACE often leads to a shorter overall survival for patients with CRC. Meanwhile, Cox regression analysis proved ACE hypomethylation was an independent prognostic marker in CRC.
In conclusion, we find ACE is up-regulated expression, and it is also hypomethylation in CRC. This regulated manner is impacted by TNM stages, invasion depth and lymph node metastasis. The cell growth is promoted while apoptosis was inhibited by ACE hypomethylation. Besides, ACE hypomethylation could be an independent indicator in the prognosis of CRC.
Disclosure statement
No potential conflict of interest was reported by the authors.
Additional information
Funding
References
- Siegel R, Naishadham D, Jemal A. Cancer statistics, 2012. CA Cancer J Clin. 2012;62:10–29.
- Siegel R, Desantis C, Jemal A. Colorectal cancer statistics, 2014. CA Cancer J Clin. 2014;64:104–117.
- Siegel R, Ma J, Zou Z, et al. Cancer statistics, 2014. CA Cancer J Clin. 2014;64:9–29.
- Jemal A, Bray F, Center MM, et al. Global cancer statistics. CA Cancer J Clin. 2011;61:69–90.
- Abali H, Gullu IH, Engin H, et al. Old antihypertensives as novel antineoplastics: angiotensin-I-converting enzyme inhibitors and angiotensin II type 1 receptor antagonists. Med Hypotheses. 2002;59:344–348.
- Bauvois B. Transmembrane proteases in cell growth and invasion: new contributors to angiogenesis? Oncogene. 2004;23:317–329.
- Yoshiji H, Kuriyama S, Fukui H. Angiotensin-I-converting enzyme inhibitors may be an alternative anti-angiogenic strategy in the treatment of liver fibrosis and hepatocellular carcinoma. Possible role of vascular endothelial growth factor. Tumour Biol. 2002;23:348–356.
- Carl-McGrath S, Lendeckel U, Ebert M, et al. The ectopeptidases CD10, CD13, CD26, and CD143 are upregulated in gastric cancer. Int J Oncol. 2004;25:1223–1232.
- Deshayes F, Nahmias C. Angiotensin receptors: a new role in cancer? Trends Endocrinol Metab. 2005;16:293–299.
- Rieder MJ, Taylor SL, Clark AG, et al. Sequence variation in the human angiotensin converting enzyme. Nat Genet. 1999;22:59–62.
- Rangel M, dos Santos JC, Ortiz PH, et al. Modification of epigenetic patterns in low birth weight children: importance of hypomethylation of the ACE gene promoter. PloS One. 2014;9:e106138.
- Liu SY, Sima X, Wang CH, et al. The association between ACE polymorphism and risk of colorectal cancer in a Chinese population. Clin Biochem. 2011;44:1223–1226.
- Yang H, Cai C, Ye L, et al. The relationship between angiotensin-converting enzyme gene insertion/deletion polymorphism and digestive cancer risk: insights from a meta-analysis. J Renin Angiotensin Aldosterone Syst. 2015;16:1306–1313.
- Lam D, Ancelin ML, Ritchie K, et al. DNA methylation and genetic variation of the angiotensin converting enzyme (ACE) in depression. Psychoneuroendocrinology. 2018;88:1–8.
- Fan R, Mao SQ, Gu TL, et al. Preliminary analysis of the association between methylation of the ACE2 promoter and essential hypertension. Mol Med Rep. 2017;15:3905–3911.
- Rakyan VK, Down TA, Thorne NP, et al. An integrated resource for genome-wide identification and analysis of human tissue-specific differentially methylated regions (tDMRs). Genome Res. 2008;18:1518–1529.
- Esteller M. Cancer epigenomics: DNA methylomes and histone-modification maps. Nat Rev Genet. 2007;8:286–298.
- Ung MH, Varn FS, Lou S, et al. Regulators associated with clinical outcomes revealed by DNA methylation data in breast cancer. PLoS Comput Biol. 2015;11:e1004269.
- Amabile G, Di Ruscio A, Muller F, et al. Dissecting the role of aberrant DNA methylation in human leukaemia. Nat Commun. 2015;6:7091.
- Bird A. DNA methylation patterns and epigenetic memory. Genes Dev. 2002;16:6–21.
- Riviere G, Lienhard D, Andrieu T, et al. Epigenetic regulation of somatic angiotensin-converting enzyme by DNA methylation and histone acetylation. Epigenetics. 2011;6:478–489.
- Rawluszko-Wieczorek AA, Horbacka K, Krokowicz P, et al. Prognostic potential of DNA methylation and transcript levels of HIF1A and EPAS1 in colorectal cancer. Mol Cancer Res. 2014;12:1112–1127.
- Truong PK, Lao TD, Doan TP, et al. Loss of expression of cyclin d2 by aberrant DNA methylation: a potential biomarker in Vietnamese breast cancer patients. Asian Pac J Cancer Prev. 2015;16:2209–2213.
- Liang YX, Mo RJ, He HC, et al. Aberrant hypomethylation-mediated CD147 overexpression promotes aggressive tumor progression in human prostate cancer. Oncol Rep. 2015;33:2648–2654.
- Yang Y, Huang JQ, Zhang X, et al. MiR-129-2 functions as a tumor suppressor in glioma cells by targeting HMGB1 and is down-regulated by DNA methylation. Mol Cell Biochem. 2015;404:229–239.
- Tao L, Huang G, Chen Y, et al. DNA methylation of DKK3 modulates docetaxel chemoresistance in human nonsmall cell lung cancer cell. Cancer. Biother & Radiopharm. 2015;30:100–106.
- Moran A, Fernandez-Marcelo T, Carro J, et al. Methylation profiling in non-small cell lung cancer: clinical implications. Int J Oncol. 2012;40:739–746.
- Moon JW, Choi JH, Lee SK, et al. Promoter hypermethylation of membrane type 3 matrix metalloproteinase is associated with cell migration in colorectal adenocarcinoma. Cancer Genet. 2015;208:261–270.
- Tsai MH, Chen WC, Yu SL, et al. DNA hypermethylation of SHISA3 in colorectal cancer: an independent predictor of poor prognosis. Ann Surg Oncol. 2015;22:S1481–S1489.
- Ding Z, Jiang T, Piao Y, et al. Meta-analysis of the association between APC promoter methylation and colorectal cancer. Onco Targets Ther. 2015;8:211–222.
- Rocken C, Neumann K, Carl-McGrath S, et al. The gene polymorphism of the angiotensin I-converting enzyme correlates with tumor size and patient survival in colorectal cancer patients. Neoplasia. 2007;9:716–722.
- Toma M, Cimponeriu D, Apostol P, et al. Lack of association between ACE ID polymorphism and colorectal cancer in Romanian patients. Chirurgia (Bucur). 2009;104:553–556.
- van der Knaap R, Siemes C, Coebergh JW, et al. Renin-angiotensin system inhibitors, angiotensin I-converting enzyme gene insertion/deletion polymorphism, and cancer: the Rotterdam Study. Cancer 2008;112:748–757.
- Zill P, Baghai TC, Schule C, et al. DNA methylation analysis of the angiotensin converting enzyme (ACE) gene in major depression. PloS One. 2012;7:e40479.