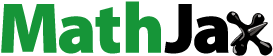
Abstract
The present study focuses on extraction of green synthesized silver nanoparticles (Ag-NPs) from Barleria longiflora L. leaves for antibacterial and photocatalytic activities. The extracted Ag-NPs have been characterized by XRD, FTIR, FE-SEM with EDX, HR-TEM accompanied SAED pattern and UV-Visible absorption spectroscopic techniques. Spectral studies confirmed the UV-Visible absorption spectrum of the Ag-NPs at a wavelength of 443 nm and a good crystalline nature with a face-centered cubic crystal structure using XRD spectrum. Surface topography and the presence of Ag in the prepared sample have been confirmed from SEM and EDX measurements. Various functional groups present in the sample have been examined using FT-IR spectroscopic analysis. A homogeneous dispersion of spherical form nanoparticles with a usual size of 2.4 nm was confirmed by visualization using FE-SEM and HR-TEM. Moreover, Ag-NPs stimulate a strong inhibition of Enterococcus sp., Streptococcus sp, Bacillus megaterium, Pseudomonas putida, Pseudomonas aeruginosa and Staphylocouus aureus; with a good catalytic reduction activity for degrading organic methylene blue (MB) dye. Therefore, silver nanoparticles obtained from Barleria longiflora L. have potential application in medicine and photocatalytic dye degradation processes.
Introduction
Development of nanoscience and technology has an intensive impact on various aspects of human life [Citation1] with particular advantages to fields of electronics and medical fields [Citation2], with a vital role played for biological markers [Citation3], catalysts [Citation4] and antimicrobials agents [Citation5]. Apart from various physical and chemical methods, the biological method is identified as crucial for the synthesis of nanoparticles [Citation6]. Variety of techniques have also been investigated by the researchers for the synthesis of silver nanoparticles (Ag-NPs) such as laser ablation [Citation7], photochemical reduction [Citation8], gamma ray and solar light irradiation [Citation9], UV photo reduction [Citation10], microwave-assisted [Citation11], electrochemical [Citation12], etc. In addition, an experimental setup can threaten the human health and the environment due to the excessive usage of toxic chemicals and their by-products during the synthesis process [Citation13]. Extraction of nanoparticles from plant leaves naturally makes the process simple, eco-friendly and an efficient method [Citation14–17]. Hence, it is very essential to focus on alternative techniques, which may lead to the consumption of fewer chemicals and natural sources (plant species), for the synthesis of Ag-NPs [Citation18–20].
Extraction of Ag-NPs from different parts of the plants (stem, root, fruit, seed, callus, peel and leaves) have been reported by the researchers, using different species such as Psidium guajava L, Convolvulus arvensis, Zanthoxylum armatum, Bauhinia tomentosa L, Thymbra spicata, Allium cepa, Citrus sinensis, Butea monosperma, Azadirachta indica, Diospyros paniculata, Gmelina arborea, Berberis vulgaris [Citation21–27]. These plant species provide a wide array of metabolites, phenolic acid, flavonoids, alkaloids and terpenoids that can enhance the activity of Ag-NPs synthesized to render antioxidant, antimicrobial, anti-inflammatory, anticancer, photo-catalytic and antidiabetic activities. The use of Barleria longiflora Lins (BL) has been primarily to cure kidney stone, cough, dropsy and inflammations. The objective of the present investigation focused on the synthesis of BL leaves mediated Ag-NPs and its application in antibacterial and photo-catalytic activities. In this inceptive study, a simple, eco-friendly and efficient biological route for synthesis of Ag-nanoparticles from BL leaves is reported.
Materials and methods
Plant collection and extraction
The BL leaves were collected from Karubayanahalli village, Dharmapuri, Western Ghats region of Tamil Nadu, India. Silver nitrate (AgNO3), nutrient agar (NA) and methylene blue (MB) of pure analytical grade were purchased from Hi Media Laboratories Pvt. Ltd., India. During the experiment distilled water (DW) was used to prepare aqueous solutions. About 20 g of fresh and good quality BL leaves were collected and thoroughly washed using DW. Washed leaves were grinded to a paste, mixed with 100 ml of DW and boiled at 80 °C for 15 min. This homogeneous suspension was filtered using Whatman filter paper No.1 grade and stored at 4 °C for further use.
Synthesis of Ag-NPs from plant extract
The Ag-NPs have been synthesized using the protocol which was already reported by Das et al. [Citation28] with slight modification. A volume of 90 ml of AgNO3 solution (with 1 mM concentration) and 10 ml of BL leaf extract were added. The suspension was then continuously stirred at 160 rpm on a magnetic stirrer until the colour of the suspension changed from pale yellow to yellowish dark brown. This colour change reflects the reduction of AgNO3 into Ag-NPs.
Characterization of the Ag-NPs
The Ag-NPs have been characterized by UV-visible spectroscopy (Shimadzu UV-1800, India). Phase formation and crystalline nature of the sample have also been examined by Rigaku X-ray diffractometer (XRD). Various functional groups exist in the Ag-NPs which have been analyzed by FTIR spectroscopic technique (Perkin Elmer Spectrum 100 FTIR Spectrometer) and the synthesized Ag-NPs were coated onto KBr pellet. The IR spectrum was recorded in mid-region wavelength of 4000–400 cm−1 at a resolution of 4.0 cm−1. The surface architectures and the average size of the particles were studied using field emission scanning electron microscope (FESEM) with EDX (JEOL 7401 F), and high-resolution scanning electron microscope (HRTEM; TecnaiTM G2 F30) with selected area electron diffraction (SAED) analyses.
Antibacterial activity
The antibacterial activity of the synthesized Ag-NPs was evaluated against pathogenic bacteria, which may cause infection to human beings: Enterococcus sp., Streptococcus sp, Bacillus megaterium, Pseudomonas putida, Pseudomonas aeruginosa and Staphylocouus aureus. The zone of inhibition was noted as per the protocol given by Roy et al. [Citation29] with a minor modification. Bacterial culture studies were maintained in nutrient broth (NB) medium; the experiments were performed on NA plates and the bacterial culture was inoculated with a volume of 100 μl of bacterial suspension with different concentrations of Ag-NPs (10 μg of Ag-NPs were dissolved in 1 ml volume of DMSO solvent to prepare single dilution). Approximately, 20 μl of the diluted Ag-NPs were added to the center of each well of 4 mm diameter. The control plates were made using a well consisting of BL extract alone. The antibiotic chloramphenicol was used as a positive control and the plates were incubated at 37 °C for 24 h. Plates were examined at the end of the incubation period and the zone of diameter was as recorded mean values (n = 3) and expressed in millimeter (mm).
Photocatalytic activity
To examine the photocatalytic activity of the as-prepared photocatalyst, 50 mg of catalyst was added into a volume of 100 ml solution (MB dye of 30 mg l−1). A high-pressure halogen lamp was used as a light source. The dye solution along with loaded catalyst was stirred for 30 min to ensure the absorption–desorption equilibrium of dye molecules on the surface of the catalyst [Citation29]. Thereafter the suspension container was placed under a halogen lamp for irradiation under continuous stirring. For every 15 min, about 4 ml of suspension was collected and centrifuged to separate the suspended catalyst for UV-Visible absorption spectroscopic analysis. As the irradiation time increases, gradual degradation in the colour which was observed was confirmed by UV-Visible absorption spectroscopy. The spectra showed a gradual decrease in the absorption intensity of MB dye. The final concentration of dye solution was calculated using the following equation
(1)
(1)
where, Co is the initial concentration of MB dye, C the final concentration of MB dye, At is the intensity of absorption band after any irradiated time (t), and Ao is the intensity of absorption band at time (t = 0). The photocatalytic dye degradation efficiency of the prepared Ag-NPs can be calculated using the following equation:
(2)
(2)
Results and discussion
UV visible spectroscopy analysis
The particle size distribution of Ag-NPs on an average is ∼2.4 ± 0.5 nm (). According to Veisi et al’s [Citation24] report, the Ag-NPs synthesized from Thymbra spicata also showed a spherical shape with an average size of 7 nm. UV-Vis spectroscopy was used to observe the formation of Ag-NPs by reduction of the aqueous Ag ions during the exposure of BL leaf extract. The colourless aqueous AgNO3 solution became yellowish dark brown few minutes’ after the addition of BL leaf extract. It was well known that this light-yellow colour of the reaction mixture owes to stimulation of SPR vibration in the Ag-NPs. shows that synthesized Ag-NPs maximum absorption spectrum at 443 nm was confirmed based on the SPR vibration range. This evidently confirmed the presence of reducing agents present in BL extract.
XRD analysis
The XRD pattern of green synthesized Ag-NPs is shown in . All the peaks positioned at 2θ values: 28.5°, 32.9°, 46.9°, 55.5°, 58.2°, 68.1° and 77.3° are assigned to the corresponding (111), (111), (220), (200), (220), (220) and (311) planes, respectively. Moreover, the spectrum strongly reveals high crystalline nature of prepared Ag-NPs. This observation is consistent with earlier reports [Citation23,Citation25].
FTIR analysis
showed that the FTIR spectrum of Ag-NPs. The peaks positioned at wave numbers 3291, 3924, 1642, 1516, 1451, 1372, 1152 and 1020 cm−1 are attributed to –OH (alcohol), >C=C (alkenes), =NH (amines) of flavonoids and amines groups, respectively. The peaks between 3291 and 3924 cm−1 correspond to the phenolic hydroxyl groups and secondary amines. The peaks from 1642 to 1451 cm−1 are due to C=O stretching vibrations of the carbonyl groups, while the peaks at 1372 cm−1 were amide peaks. Aromatic ring or C=C existence was confirmed by 1372 cm−1 band. Stretching mode C–O–C is observed from 502 to 1152 cm−1 by an aromatic ether linkage. FTIR spectroscopic analysis confirmed the strong affinity of Ag-NPs towards different functional groups in the BL compounds, which agrees with the earlier results [Citation30,Citation31]. Hence phenolic agents and proteins present in the BL extraction were responsible for successful reduction of AgNO3 into Ag-NPs.
FE-SEM-EDX and HR-TEM analysis
Surface architecture and presence of Ag element have been investigated using FE-SEM with EDX analysis respectively. shows spherical morphology with the little aggregation of Ag nanoparticles. The EDX spectrum confirms the presence of Ag elements, which was identical with an earlier report [Citation32]. HR-TEM analysis also revealed the mono-dispersion of spherical nanoparticles. Additionally, shows a thin layer of capping organic material obtained from Ag-NPs. The capping organic material effectively prevents the Ag-NPs from agglomeration and makes them more stable.
Antibacterial activity
Ag-NPs have good inhibition against the human pathogenic bacteria and the obtained results shown in . Zone of inhibitions in different concentration of Ag-NPs are tabulated in . The results illustrate that synthesized Ag-NPs can be an efficient antibacterial agent against human pathogens and it was enhanced with the increasing dose concentrations of Ag-NPs. The Ag-NPs show a very negligible zone of inhibition, and for bacterial strains, indicates low appreciable activity against Bacillus megaterium, Staphylocouus aureus, Pseudomonas putida and Pseudomonas aeruginosa. In this connection, the mechanism of antibacterial activities was realized by the nanoparticles which may affect the bacterial strains cell wall, cell membrane generation through hydrophobic, and the bioaccumulation mechanism [Citation33–37].
Figure 3. Antibacterial activity of Ag-NPs against human pathogens (a) Enterococcus sp., (b) Streptococcus sp, (c) Bacillus megaterium, (d) Pseudomonas putida (e) Pseudomonas aeruginosa, (f) Staphylocouus aureus. Concentration of Ag-NPs – 50, 75 and 100 µl; P.E- leaf extract.
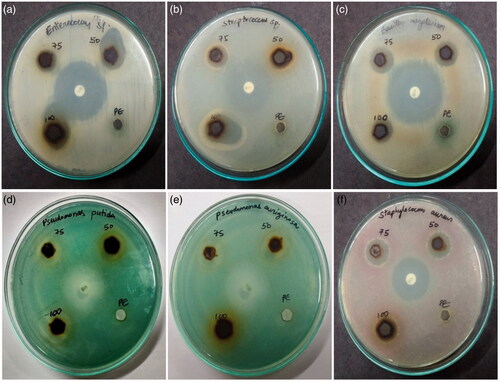
Table 1. Antibacterial activity of the synthesized nanoparticles from Barleria longiflora L leaf extracts.
Photocatalytic dye degradation
An aqueous solution of MB dye loaded with Ag-NPs catalyst is exposed to halogen lamp, and the irradiated solution is periodically collected for UV-Visible absorption spectroscopic analysis(). Within 45 min of exposure of dye solution under visible light Ag nanoparticles degraded the toxicity completely from the solution. This is due to the potential photosensitization behavior of Ag nanoparticles. The high photocatalytic activity can be correlated with the following factors: (i) enhanced light absorption ability; (ii) action of both e−/h+ traps so as to reduce the recombination rate of electron and hole pairs [Citation38–40].
Figure 4. (a) Adsorption spectrum of methylene blue under light irritation, (b) adsorption spectrum of Ag-NPs against methylene blue under light irritation and (c) photocatalytic degradation of methylene blue in the presence Ag-NPs.
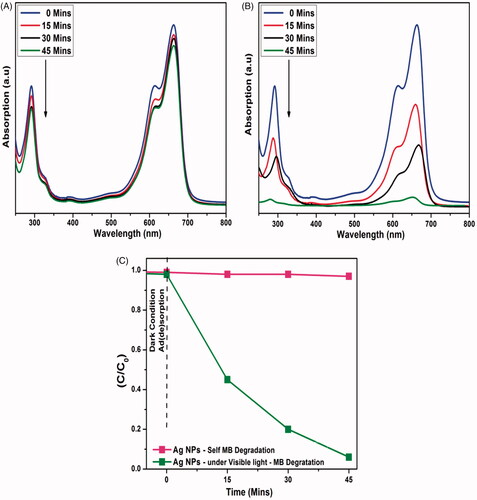
Using Ag-NPs as a catalyst, the concentration of MB dye was only reduced up to an optimum of 3% with 45 min of visible-light irradiation (). The remarkable important photocatalytic activity was observed where the concentration of MB dye was decreased by 93% on the same revelation time. The excellent photocatalytic behaviors caused by the synergistic Ag-NPs, which participated in charge transfer and bridge of separation efficiency. Similarly, in accordance with many reports with respect to the efficiency of green synthesized Ag-NPs and their biological activities [Citation41–48], our green synthesized Ag-NPs also have proven to be useful for wastewater remediation applications as well.
Conclusion
Eco-friendly green synthesis route used to prepare Ag-NPs from aqueous BL leaf extract was confirmed by XRD analysis and FTIR spectrum. Spherical surface morphology was examined using FE-SEM with EDX and HR-TEM with SAED analyses. The Ag-NPs showed as higher antibacterial activity against Enterococcus sp., and Pseudomonas aeruginosa and lesser antibacterial activity against Staphylocouus aureus and Bacillus megaterium compared to the pharmaceutical formulation of chloramphenicol. Complete degradation of MB dye occurred within 45 min with the help of Ag-NPs as catalyst. The results clearly revealed that the synthesized Ag-NPs catalyst has shown remarkable antibacterial and photocatalytic dye degradation activities rendering its use for societal applications.
Acknowledgement
All the authors gratefully acknowledge Sejong University, Seoul, Republic of Korea for providing instrumental analysis facility.
Disclosure statement
No potential conflict of interest was reported by the authors.
Additional information
Funding
References
- Nordberg J, Arnér E. Reactive oxygen species, antioxidants, and the mammalian thioredoxin. Free Radic Biol Med. 2001;31:1287–1312.
- Lee E, Kim DH, Woo Y, et al. Solution structure of peptide AG4 used to form silver nanoparticles. Biochem Biophys Res Commun. 2008;376:595–598.
- Edelstein R. The BARC biosensor applied to the detection of biological warfare agents. Biosens Bioelectron. 2000;14:805–813.
- Astruc D, Lu F, Aranzaes JR. Nanoparticles as recyclable catalysts: The frontier between homogeneous and heterogeneous catalysis. Angew Chem Int Ed Engl. 2005;44:7852–7872.
- Lara HH, Garza-Treviño EN, Ixtepan-Turrent L, et al. Silver nanoparticles are broad-spectrum bactericidal and virucidal compounds. J Nanobiotechnol. 2011;9:30.
- Ghosh S, Kaushik R, Nagalakshmi K, et al. Antimicrobial activity of highly stable silver nanoparticles embedded in agar-agar matrix as a thin film. Carbohydr Res. 2010;345:2220–2227.
- Abid JP, Wark AW, Brevet PF, et al. Preparation of silver nanoparticles in solution from a silver salt by laser irradiation. Chem Commun. 2002;2002;792–793.
- Kéki S, Török J, Deák G, et al. Silver nanoparticles by PAMAM-assisted photochemical reduction of Ag+. J Colloid Interface Sci. 2000;229:550–553.
- Wei X, Luo M, Li W, et al. Synthesis of silver nanoparticles by solar irradiation of cell-free Bacillus amyloliquefaciens extracts and AgNO3. Bioresour Technol. 2012;103:273–278.
- Yuan X, Wang H, Wu Y, et al. One-pot self-assembly and photoreduction synthesis of silver nanoparticle-decorated reduced graphene oxide/MIL-125(Ti) photocatalyst with improved visible light photocatalytic activity. Appl Organometal Chem. 2016;2016:289–296.
- Parveen M, Ahmad F, Malla AM, et al. Microwave-assisted green synthesis of silver nanoparticles from Fraxinus excelsior leaf extract and its antioxidant assay. Appl Nanosci. 2016;6:267–276.
- Yin B, Ma H, Wang S, et al. Electrochemical synthesis of silver nanoparticles under protection of poly (N -vinylpyrrolidone). J Phys Chem B. 2003;107:8898–8904.
- Padalia H, Moteriya P, Chanda S. Green synthesis of silver nanoparticles from marigold flower and its synergistic antimicrobial potential. Arab J Chem. 2015;8:732–741.
- Mittal AK, Chisti Y, Banerjee UC. Synthesis of metallic nanoparticles using plant extracts. Biotechnol Adv. 2013;31:346–356.
- Mittal AK, Kaler A, Banerjee UC. Free radical scavenging and antioxidant activity of silver nanoparticles synthesized from flower extract of Rhododendron dauricum. Nano Biomed Eng. 2012;4:118–124.
- Chitra K, Manikandan A, Arul Antony S. Effect of poloxamer on Zingiber officinale extracted green synthesis and antibacterial studies of silver nanoparticles. J Nanosci Nanotechnol. 2016;16:758–764.
- Chitra K, Reena K, Manikandan A, et al. Antibacterial studies and effect of poloxamer on gold nanoparticles by zingiber officinale extracted green synthesis. J Nanosci Nanotechnol. 2015;15:4984–4991.
- Jayanthi P, Gaithuilung R, Kazingmei P. Physicochemical analysis for reclamation of soils of Tingroi Hills in Lunghar, Ukhrul District, Manipur, India. Universal J Environ Res Technol 2015;5:101–111.
- Salari Z, Danafar F, Dabaghi S, et al. Sustainable synthesis of silver nanoparticles using macroalgae Spirogyra varians and analysis of their antibacterial activity. J Saudi Chem Soc. 2016;20:459–464.
- Gupta P, Kumar V, Usmani Z, et al. Phosphate solubilization and chromium (VI) remediation potential of Klebsiella sp. strain CPSB4 isolated from the chromium contaminated agricultural soil. Chemosphere 2018;192:318–327.
- Wang L, Lu F, Liu Y, et al. Photocatalytic degradation of organic dyes and antimicrobial activity of silver nanoparticles fast synthesized by flavonoids fraction of Psidium guajava L. leaves. J Mol Liq. 2018;263:187–192.
- Rasheed T, Bilal M, Li C, et al. Catalytic potential of bio-synthesized silver nanoparticles using Convolvulus arvensis extract for the degradation of environmental pollutants. J Photochem Photobiol B.2018;181:44–52.
- Jyoti K, Singh A. Green synthesis of nanostructured silver particles and their catalytic application in dye degradation. J Genet Eng Biotechnol. 2016;14:311–317.
- Veisi H, Azizi S, Mohammadi P. Green synthesis of the silver nanoparticles mediated by Thymbra spicata extract and its application as a heterogeneous and recyclable nanocatalyst for catalytic reduction of a variety of dyes in water. J Clean Prod. 2018;170:1536–1543.
- Pattanayak S, Mollick MMR, Maity D, et al. Butea monosperma bark extract mediated green synthesis of silver nanoparticles: characterization and biomedical applications. J Saudi Chem Soc. 2017;21:673–684.
- Saha J, Begum A, Mukherjee A, et al. A novel green synthesis of silver nanoparticles and their catalytic action in reduction of Methylene Blue dye. Sustainable Environ Res. 2017;27:245–250.
- Behravan M, Hossein Panahi A, Naghizadeh A, et al. Facile green synthesis of silver nanoparticles using Berberis vulgaris leaf and root aqueous extract and its antibacterial activity. Int J Biol Macromol. 2019;124:148–154.
- Das M, Mondal A, Patowary K, et al. Biosynthesis of AgNPs using aqueous leaf extract of Ipomoea eriocarpa and their anti-inflammatory effect on carrageenan-induced paw edema in male Wistar rats. IET Nanobiotechnol. 2017;11:225–229.
- Roy K, Sarkar CK, Ghosh CK. Photocatalytic activity of biogenic silver nanoparticles synthesized using yeast (Saccharomyces cerevisiae) extract. Appl Nanosci. 2015;5:953–959.
- Viamajala S, Peyton BM, Apel WA, et al. Chromate reduction in Shewanella oneidensis MR-1 is an inducible process associated with anaerobic growth. Biotechnol Prog. 2002;18:290–295.
- Kalaimurugan D, Vivekanandhan P, Sivasankar P, et al. Larvicidal activity of silver nanoparticles synthesized by Pseudomonas fluorescens YPS3 isolated from the Eastern Ghats of India. J Clust Sci. 2019;30:225–233.
- Patil S, Chaudhari G, Paradeshi J, et al. Instant green synthesis of silver-based herbo-metallic colloidal nanosuspension in Terminalia bellirica fruit aqueous extract for catalytic and antibacterial applications. 3 Biotech. 2017;7:36.
- Bondarenko O, Ivask A, Käkinen A, et al. Particle-cell contact enhances antibacterial activity of silver nanoparticles. PLoS ONE. 2013;8:e64060.
- Chitra K, Manikandan A, Moortheswaran S, et al. Zingiber officinale extracted green synthesis of copper nanoparticles: structural, morphological and antibacterial studies. Adv Sci Engng Med. 2015;7:710–716.
- Elayakumar K, Dinesh A, Manikandan A, et al. Structural, morphological, enhanced magnetic properties and antibacterial bio-medical activity of rare earth element (REE) cerium (Ce3+) doped CoFe2O4 nanoparticles. J Magn Magn Mater. 2019;476:157–165.
- Manikandan A, Manikandan E, Meenatchi B, et al. Rare earth element (REE) lanthanum doped zinc oxide (La: ZnO) nanomaterials: synthesis structural optical and antibacterial studies. J Alloy Comp. 2017;723:1155–1161.
- Ravichandran AT, Srinivas J, Karthick R, et al. Facile combustion synthesis, structural, morphological, optical and antibacterial studies of Bi1− xAlxFeO3 (0.0 ≤ x ≤ 0.15) nanoparticles. Ceram Int. 2018;44:13247–13252.
- Nagaraj P, Aradhana N, Shivakumar A, et al. Spectrophotometric method for the determination of chromium (VI) in water samples. Environ Monit Assess. 2009;157:575–582.
- Cittrarasu V, Balasubramanian B, Kaliannan D, et al. Fabrication and characterization of noble crystalline silver nanoparticles from Ceropegia bulbosa Roxb root tuber extract for antibacterial, larvicidal and histopathology applications. Nanosci Nanotech Lett. 2019;11:11–21.
- Kumar B, Vizuete KS, Sharma V, et al. Ecofriendly synthesis of monodispersed silver nanoparticles using Andean Mortiño berry as reductant and its photocatalytic activity. Vacuum 2019;160:272–278.
- Meena Kumari M, Philip D. Facile one-pot synthesis of gold and silver nanocatalysts using edible coconut oil. Spectrochim Acta A Mol Biomol Spectrosc. 2013;111:154–160.
- Vidhu VK, Philip D. Catalytic degradation of organic dyes using biosynthesized silver nanoparticles. Micron 2014;56:54–62.
- Kunnamareddy M, Diravidaman B, Rajendran R, et al. Synthesis of silver and sulphur codoped TiO2 nanoparticles for photocatalytic degradation of methylene blue. J Mater Sci: Mater Electron. 2018;29:18111–18119.
- Bomila R, Srinivasan S, Gunasekaran S, et al. Enhanced photocatalytic degradation of methylene blue dye, opto-magnetic and antibacterial behaviour of pure and La-doped ZnO nanoparticles. J Supercond Nov Magn. 2018;31:855–864.
- Maruthamani D, Vadivel S, Kumaravel M, et al. Fine cutting edge shaped Bi2O3 rods/reduced graphene oxide (RGO) composite for supercapacitor and visible-light photocatalytic applications. J Colloid Interface Sci. 2017;498:449–459.
- Thilagavathi P, Manikandan A, Sujatha S, et al. Sol–gel synthesis and characterization studies of NiMoO4 nanostructures for photocatalytic degradation of methylene blue dye. Nanosci Nanotechnol Lett. 2016;8:438–443.
- Shameem A, Devendran P, Siva V, et al. Preparation and characterization studies of nanostructured CdO thin films by SILAR method for photocatalytic applications. J Inorg Organomet Polym. 2017;27:692–699.
- Silambarasu A, Manikandan A, Balakrishnan K. Room-temperature superparamagnetism and enhanced photocatalytic activity of magnetically reusable spinel ZnFe2O4 nanocatalysts. J Supercond Nov Magn. 2017;30:2631–2640.